- 1Neurology Service, Department of Diagnostic and Therapeutic Services, IRCCS ISMETT (Istituto Mediterraneo per i Trapianti e Terapie ad Alta Specializzazione), University of Pittsburgh Medical Center (UPMC), Palermo, Italy
- 2Department of Research, IRCCS ISMETT, UPMC, Palermo, Italy
- 3Rehabilitation Service, IRCCS ISMETT, Palermo, Italy
- 4Department of Anesthesiology and Intensive Care, IRCCS ISMETT, UPMC, Palermo, Italy
- 5Radiology Unit, Department of Diagnostic and Therapeutic Services, IRCCS ISMETT, Palermo, Italy
- 6Cardiac Surgery Unit, Department for the Treatment and Study of Cardiothoracic Diseases and Cardiothoracic Transplantation, IRCCS ISMETT, Palermo, Italy
- 7Salvator Mundi International Hospital, UPMC, Rome, Italy
- 8Discovery Center for Musculoskeletal Recovery, Schoen Adams Research Institute at Spaulding, Boston, MA, United States
- 9Department of Physical Medicine and Rehabilitation, Spaulding Rehabilitation Hospital, Charlestown, MA, United States
- 10Department of Physical Medicine and Rehabilitation, Harvard Medical School, Boston, MA, United States
Objective: Mechanisms of neurocognitive injury as post-operative sequelae of coronary artery bypass grafting (CABG) are not understood. The systemic inflammatory response to surgical stress causes skeletal muscle impairment, and this is also worsened by immobility. Since evidence supports a link between muscle vitality and neuroprotection, there is a need to understand the mechanisms by which promotion of muscle activity counteracts the deleterious effects of surgery on long-term cognition.
Methods: We performed a clinical trial to test the hypothesis that adding neuromuscular electrical stimulation (NMES) to standard rehabilitation care in post-CABG patients promotes the maintenance of skeletal muscle strength and the expression of circulating neuroprotective myokines.
Results: We did not find higher serum levels of neuroprotective myokines, except for interleukin-6, nor better long-term cognitive performance in our intervention group. However, a greater increase in functional connectivity at brain magnetic resonance was seen between seed regions within the default mode, frontoparietal, salience, and sensorimotor networks in the NMES group. Regardless of the treatment protocol, patients with a Klotho increase 3 months after hospital discharge compared to baseline Klotho values showed better scores in delayed memory tests.
Significance: We confirm the potential neuroprotective effect of Klotho in a clinical setting and for the first time post-CABG.
Introduction
Cognitive decline is still a common neurologic complication following coronary artery bypass grafting (CABG). Post-CABG cognitive decline affects 43% of patients acutely; this reduces to 19% at 4–6 months but then increases again to 25% of patients between 6 months to 1 year post-operatively (1). The mechanisms of neurocognitive injury are not fully understood. It is known that the systemic inflammation induced by surgery causes blood–brain barrier (BBB) dysfunction, resulting in an influx of inflammatory mediators (2). The increased release of pro-inflammatory cytokines also causes muscle proteolysis, resulting in the loss of skeletal muscle mass. Furthermore, skeletal muscle protein synthesis decreases after surgery since amino acids are directed toward acute phase protein production (3). Altered protein metabolism occurs within 48 h of surgery, the phase when patients have mobility restrictions from being in the intensive care unit (ICU) (4). Bedrest, in turn, exacerbates muscle impairment. Skeletal muscle wasting triggers a devastating cascade of decline in both physical and cognitive functioning. In addition to the critical role of skeletal muscle function in physical mobility, increased attention is being paid to the endocrine function of skeletal muscle as an important secretor of circulating factors (myokines) that play a role in a myriad of systemic functions, including cardiovascular and cognitive health (5, 6). Sarcopenia has been reported to be associated with cognitive decline caused by imbalanced myokine secretion, which leads to upregulation of pro-inflammatory cytokines responsible for memory dysfunction (7). The positive role of physical exercise on brain health suggests that muscle-brain crosstalk, at least in part, may be mediated by myokine signaling. Indeed, exercise promotes hippocampal neurogenesis and blood flow to this part of the brain and, more in general, an improvement in multidomain cognition [reviewed in Severinsen et al. (8)]. Potential activity-induced candidate myokines that have been associated with cognitive function include: Klotho and brain-derived neurotrophic factor (BDNF), which regulate neuronal survival and growth and protect from cardiovascular or cognitive impairment (9–12); fibroblast growth factor 23 (FGF23) and Klotho co-receptor, which may impact brain function, resulting in hippocampal-dependent cognitive impairment if lacking (13–15); and interleukin-6 (IL-6), a pro-inflammatory marker involved in neuroinflammation, which during exercise has an anti-inflammatory effect (5, 16–18). We found this set of physical activity-induced biomarkers interesting because of their differentiated neuroprotective activities: neurogenesis (Klotho, BDNF and FGF-23) and anti-inflammatory effects (IL-6).
In light of this and other evidence supporting a link between muscle vitality and cognitive function, there is a need to preserve skeletal muscle mass and strength during hospitalization for surgical procedures such as CABG. Early mobilization after surgery has multiple benefits for CABG patients, although there is no consensus on the best type, intensity, or duration of rehabilitation (19). Soon after surgery, patients often have no ability to produce effective voluntary muscle contraction because of post-operative pain, hemodynamic instability, the need for mechanical ventilation, sedation, analgesic treatment, and/or tube and catheter placement. For all these reasons, early standard rehab in patients admitted to ICU or semi-intensive units after cardiac surgery is challenging. Conversely, neuromuscular electrical stimulation (NMES) enhances muscle activity without requiring active effort. NMES has also been recognized as a promising tool in cardiovascular rehabilitation (4).
Iwatsu et al. reported the safe use of NMES soon after cardiac surgery (20). Later in the course of recovery, NMES may even be used to attenuate muscle proteolysis and strength loss after cardiac surgery (21). However, data on the post-operative effects of NMES in cardiac surgery patients are conflicting. A randomized clinical trial conducted by Fontes Cerqueira et al. (4) reported that the use of NMES had no effect on walking ability, strength, quality of life or functional outcome in the post-operative period for patients who underwent regular valve replacement. Similarly, Kitamura et al. demonstrated no significant effects of NMES on muscle proteolysis or physical function after cardiovascular surgery (22).
The aim of the present single-center pilot clinical trial was to test our central hypothesis that the addition of an NMES protocol combined with usual care can preserve and enhance skeletal muscle mass in CABG patients. Moreover, we aimed to verify whether the enhanced muscle activity induced by NMES promotes the secretion of myokines important for the preservation of cognitive outcomes.
Materials and methods
Study design
A randomized, 2-arm, controlled trial was performed at a single center between February 1st 2019 and July 31st 2020. This study was approved by the Institutional Research Review Board (approval number: 32/17) and the Ethical Committee. Written informed consent was obtained from each participant. Immediately after CABG surgery, adults (≥ 18 years old) were enrolled in the study and randomly assigned to the intervention (NMES + standard rehab) or control group (standard rehab). Randomization was performed using the electronic randomization system provided by RedCap,1 the same web application used to collect and analyze the study data. Investigators involved in all assessments were blinded to group assignment.
Surgical procedure
CABG was performed under general anesthesia. Cardiopulmonary bypass was established through the cannulation of the ascending aorta and the right atrium after full heparin administration. Myocardial protection was achieved by warm blood cardioplegia delivered every 15 min during aortic cross-clamp.
Participants
Adult patients of both sexes affected with critical coronary artery disease (CAD) and scheduled for elective or urgent CABG surgery were selected if they had been hospitalized for less than 6 days prior to the enrolment date. Participants were recruited during their ICU stay or once they had been transferred to the cardiothoracic unit (CTU), as soon as possible after surgery, and after having signed the informed consent form. Study exclusion criteria were: current use of neuromuscular blocking drugs or vasopressors; fraction of inspired oxygen ≥60%, or positive end-expiratory pressure ≥ 10 cm H20; severe respiratory failure or neurologic disorder with lower extremity function or cognition impairment; blindness; deafness; illiteracy; history of quadriceps or patellar tendon rupture; skin lesions that precluded the application of NMES surface electrodes; current lower extremity fractures; and prisoners. Cognitive and motor impairment was identified by reviewing patients’ medical charts or interviewing a family member when available. Moreover, in order to exclude pre-existing cognitive impairment, a Brief Intelligence Test (TIB) was administered.
Interventions
For both the NMES and usual care groups, 30-min sessions were conducted twice daily from the enrolment date for 15 days maximum, starting as soon as possible after surgery and continuing during the hospital stay.
Usual care group
Subjects received standard care, which included passive mobilization of the limbs, active assisted and/or active exercises (with or without resistance, according to strength level), and cycle ergometry, according to the patient’s consciousness and compliance. Usually, the cycle ergometry setup was as follows: 18–20 revolutions per minute (rpm), resistance 1 or 2 for 30–40 min. When feasible, further rehabilitation included chair sitting and progressive gait training. Intensity of physical activity was decided after a multidisciplinary group discussion and considering clinical status.
NMES group
In addition to usual care, subjects in this group completed a low-intensity electrical stimulation protocol to the quadriceps, tibialis anterior (TA), and gastrocnemius muscles bilaterally using the electra stimulator (physioled) (23). Stimulation began on the day of enrolment following baseline assessment. Surface electrode placement and NMES device set-up were performed daily by a trained physical therapist who also checked periodically for skin irritation during the initiation of the NMES protocol. Muscles were stimulated at a pulse frequency of 50 hertz (Hz), with a pulse width of 200 microseconds (μs). The alternating stimulation protocol consisted of a 12-s stimulation of bilateral lower extremity muscles, followed by a 6-s rest, with a 1.6 s ramp-up time and a 1.6 s ramp-down time (24). The intensity of stimulation was gradually increased until a slight muscle contraction was observed; then it was decreased to just subcontractile. This protocol has already been safely used in patients in ICU with no reported major complications (24). Heart rate (HR), systolic blood pressure (SBP), diastolic blood pressure (DBP), saturation of peripheral oxygen (SpO2), and respiratory rate were monitored continuously during sessions. Sessions were stopped if patients had an abnormal physiologic response: HR > 70% of predicted maximum, >20% decrease in HR, SBP > 180 mm Hg, > 20% decrease in SBP or DBP, SpO2 < 90%, or clinical signs and symptoms of cardiorespiratory distress.
Outcome measures
The primary outcome variables were levels of the circulating biomarkers: Klotho, FGF23, IL-6 and BDNF. The serum levels of FGF23, IL-6 and BDNF were determined using magnetic bead technology from LuminexTM with the Human Custom ProcartaPlex 3-plex (Affymetrix, Vienna, Austria) according to the manufacturer’s instructions. In addition, the levels of serum α-Klotho were determined using the Human soluble α-Klotho ELISA kit (Immuno-Biological Labs, Japan), following the manufacturer’s instructions, and analyzed by the Spark Microplate Reader (Tecan, Männedorf, Switzerland). The concentration of each factor was calculated from standard curves.
Secondary outcome variables were: (a) Clinical (physical and cognitive function): bilateral tibialis anterior (TA) and quadriceps muscle strength measured using a hand-held dynamometer (Sauter, Kern, FK Digital dynamometer), Repeatable Battery for the Assessment of Neuropsychological Status (RBANS) test, Trail Making Test (TMT) and Mini-Mental State Examination (MMSE) scores; (b) Neuroradiological: structural and functional brain magnetic resonance imaging (MRI) features performed on a 3 T MRI scanner (Discovery 750w, General Electric Medical System) utilizing a 32-channel head coil. Participants were positioned in the scanner with their heads comfortably restrained by foam padding to reduce head movement. Earplugs were used to reduce the noise of the scanner.
Muscle strength and biomarker data were collected at baseline (before starting intervention, t0) and 3 months after hospital discharge (t1). Baseline brain MRI studies were performed at the earliest feasible time after enrolment, mostly within 2 days (mean 2.13 ± standard deviation 2.68) and at t1. Finally, cognitive assessments were performed only at t1. A more detailed description of the demographic and clinical data collected, serum analysis, muscle strength evaluation, cognitive testing and imaging analysis is provided in the Supplementary material.
Data analysis
Sample size and power
We performed this pilot study to generate a formal sample size and power computations for planning a larger and more comprehensive prospective study.
Statistical analysis
Qualitative variables are described by frequency distributions and percentages, while quantitative variables are expressed by mean ± standard deviation (SD) or median ± interquartile range. To compare the two treatment groups, the chi-squared test and Fisher’s exact test were used for categorical variables, while the two-sample t-test and Wilcoxon-Mann–Whitney test were used for continuous variables when appropriate. Comparisons between two temporal observations of biomarkers were assessed with paired t-tests. Since the data were repeated, a generalized estimating equations approach was applied to describe the effect of treatment on biomarkers and on muscle strength. Moreover, a generalized linear model was used to describe the effect of education on cognitive assessment on the basis of treatment. P-values < 0.05 were considered statistically significant. All statistical analyses were performed using SAS software, version 9.4 (SAS Institute Inc., Cary, NC, United States).
Results
The analyses included only patients who completed the entire protocol (55 patients in total, NMES group: 23; control group: 32). The subjects’ demographic and clinical data are reported in Table 1. The two groups (NMES and control) were homogenous in relation to baseline variables, length of stay (LOS) and number of rehabilitation sessions. None of the patients enrolled in the study experienced delirium during the hospital stay or perioperative stroke. Baseline muscle strength evaluations, biomarker values and structural brain MRI features are reported in Table 2.
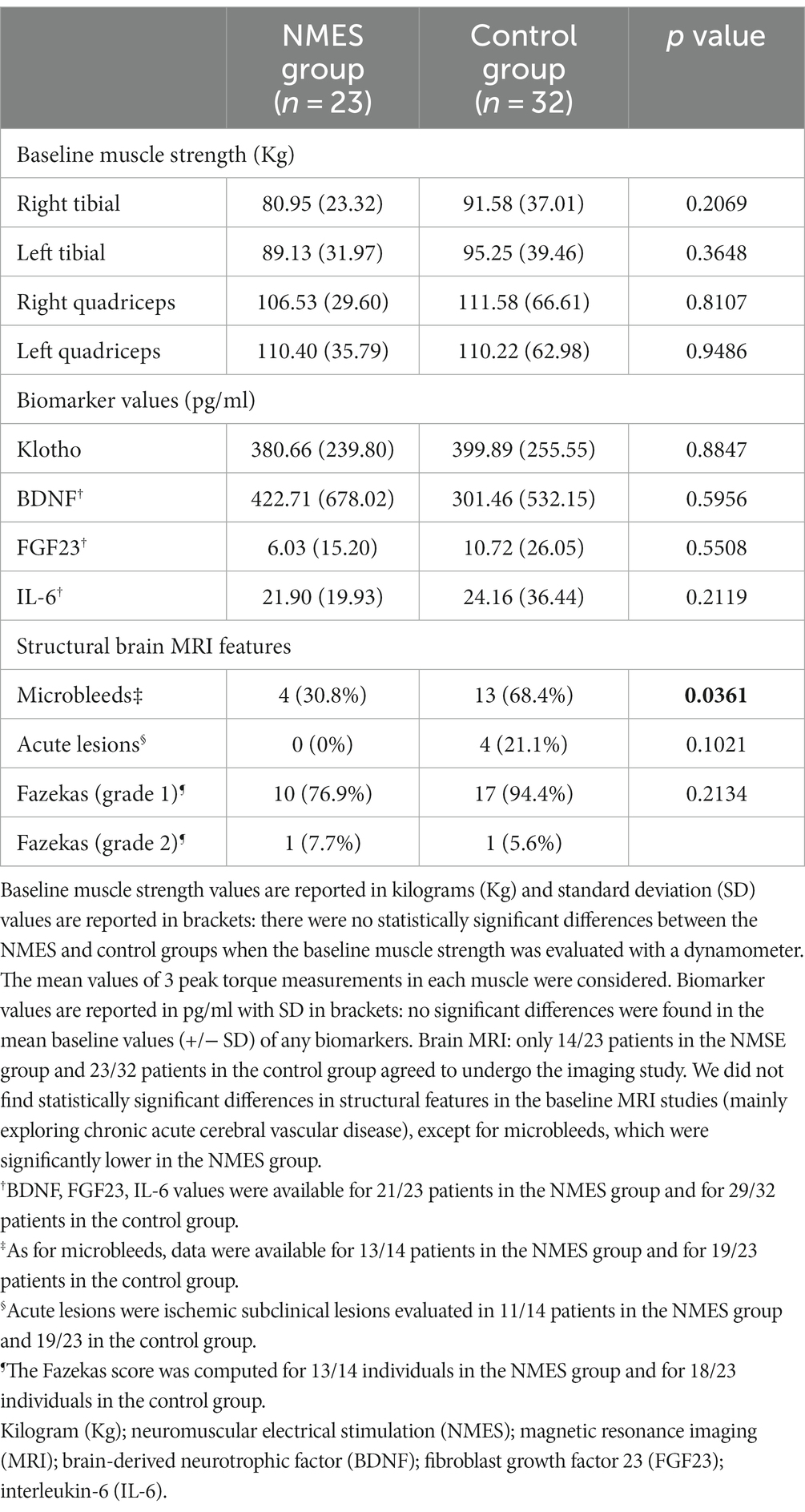
Table 2. Baseline muscle strength evaluations (Kg), biomarker values (pg/ml) and structural brain MRI features.
The primary outcome was the difference in biomarker values between the interventional and control groups 3 months after hospital discharge (mean values are reported in Table 3). There was no statistically significant difference between the two groups for Klotho, BDNF or FGF23, but IL-6 was significantly higher in the NMES group (Table 3).
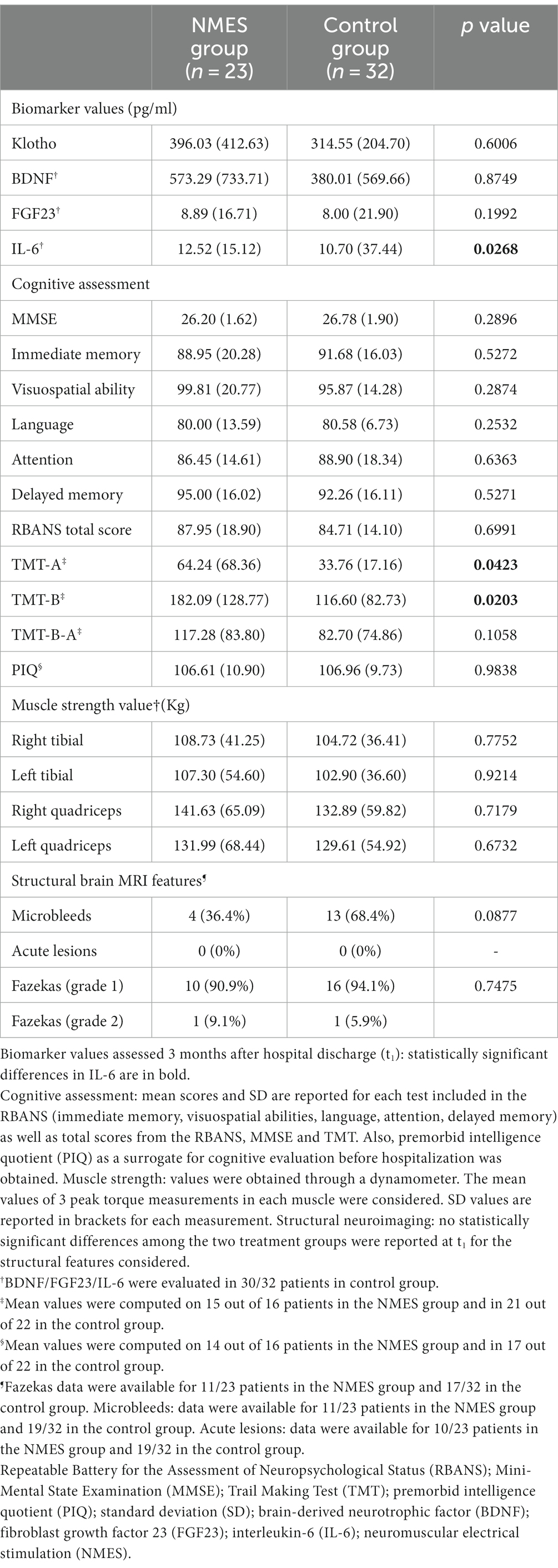
Table 3. Biomarker values, cognitive assessment, muscle strength values and neuroimaging analysis 3 months after hospital discharge.
Secondary outcome variables, the cognitive assessment performance scores obtained at MMSE, TMT A and B, and RBANS, are reported in Table 3. No significant differences were found in scores computed for any test exploring different cognitive functions. A statistically significant difference was reported in TMT B, a test of attention, with the control group displaying lower values (i.e., better attention). No statistically significant differences between either group was observed in muscle strength values or brain MRI structural features when evaluated 3 months after hospital discharge (Table 3).
Functional brain MRI imaging was fully acquired in 16 subjects (eight in each group). MRI exams not fully acquired or not suitable for image post-processing were excluded. The remaining patients eligible for MRI examination were not imaged due to lack of consent for imaging or the presence of metallic foreign bodies.
There was a statistically significant (p 0.05 false discovery rate (FDR)) medium to large (Cohen’s d = 0.5 medium; Cohen’s d = 0.8 large) increase in functional connectivity in the default mode, frontoparietal, salience, and sensorimotor networks in patients after usual care treatment compared to pre-treatment functional connectivity in the same networks (Figures 1, 2).
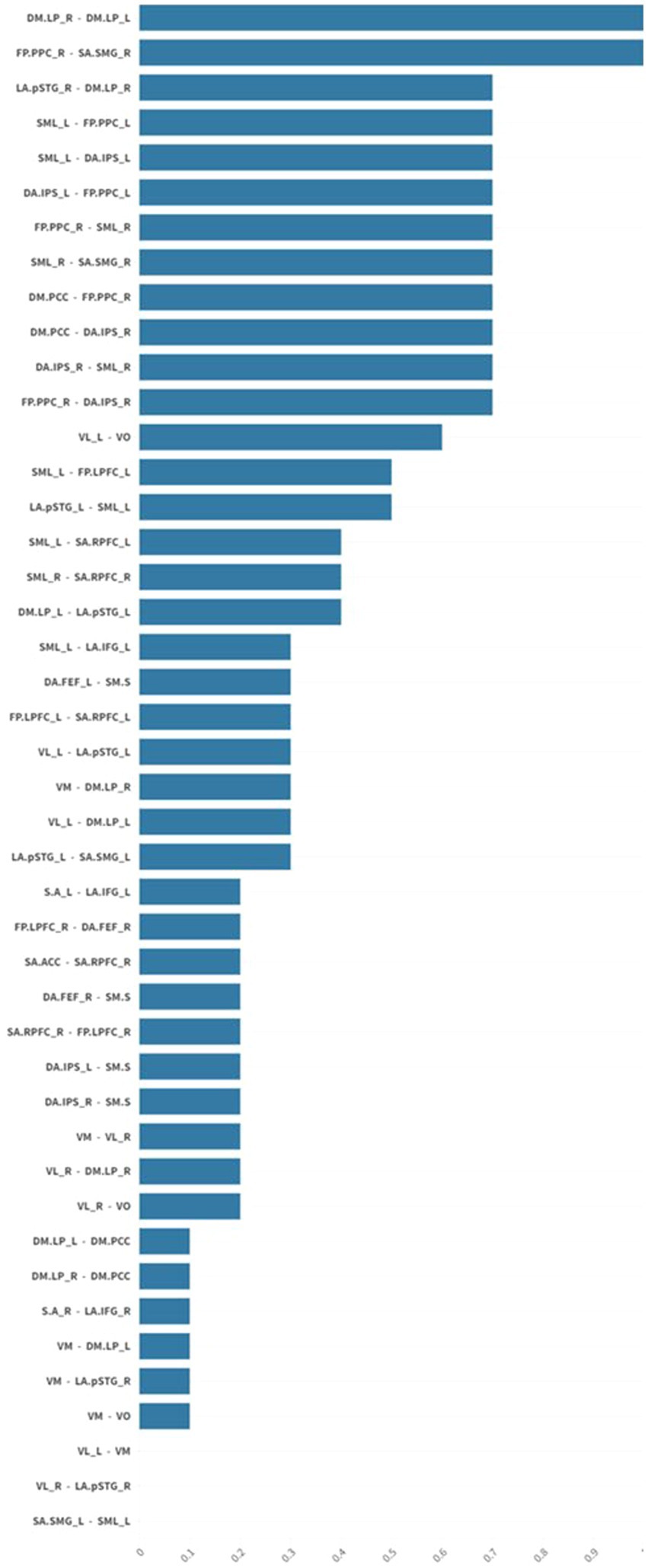
Figure 1. The graph shows mean effect size differences in resting-state network connectivity before and after usual care treatment. Mean effect sizes are represented as average normalized differences in Cohen’s d. There was a statistically significant (p 0.05 FDR) medium to large (Cohen’s d = 0.5 medium; Cohen’s d = 0.8 large) increase in functional connectivity in the default mode, frontoparietal, salience, and sensorimotor networks in patients after usual care treatment. False discovery rate (FDR).
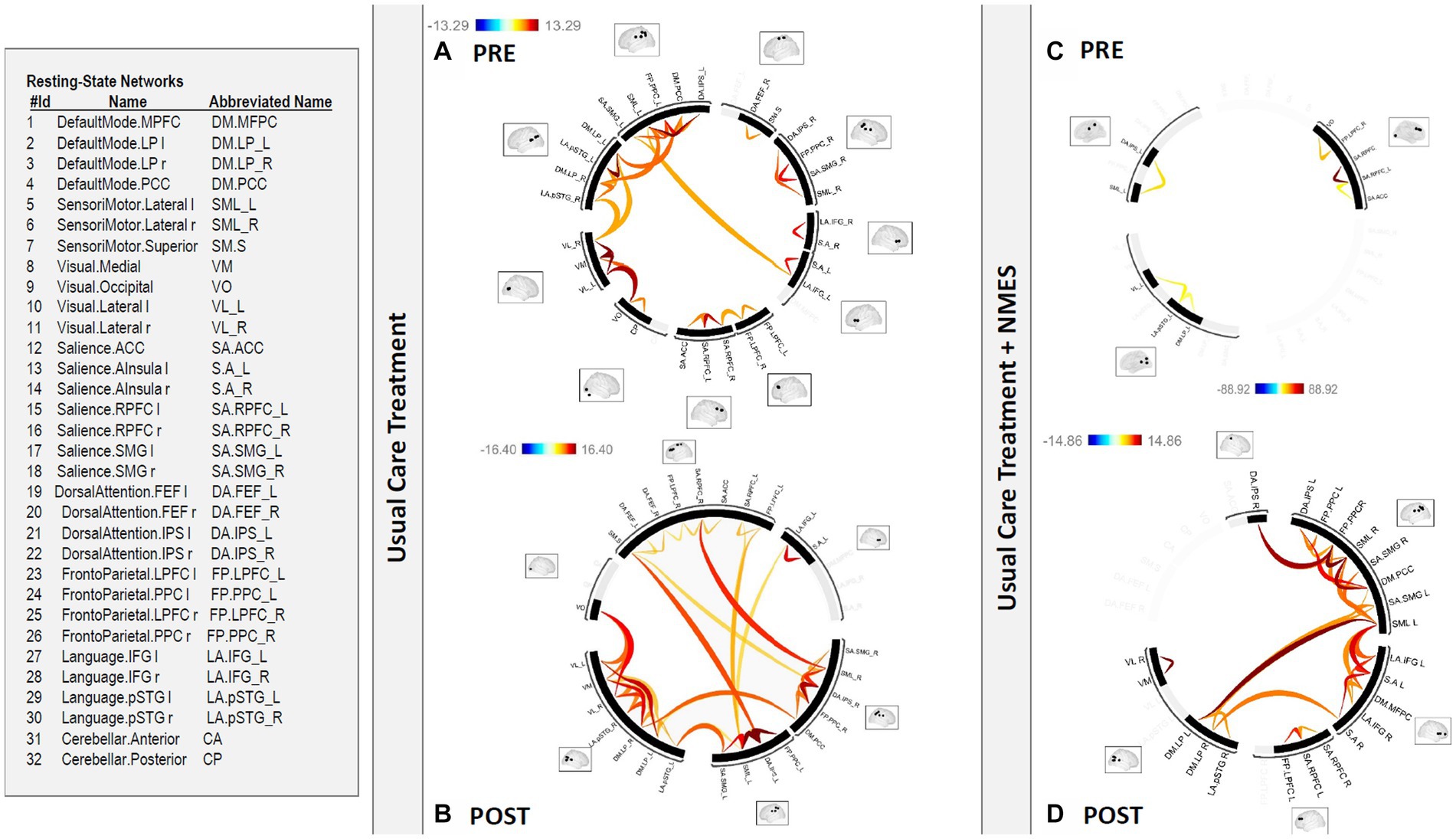
Figure 2. Functional connectome in patients (A) PRE and (B) POST usual care treatment versus (C) PRE and (D) POST NMES treatment. There was a statistically significant (p < 0.05 FDR) increase in functional connectivity in the default mode, frontoparietal, salience, and sensorimotor networks in patients after usual care treatment compared to pre-treatment functional connectivity in the same networks. This increased functional connectivity in the default mode, frontoparietal, salience, and sensorimotor networks was even greater for individuals in the usual care plus NMES group, compared to pre-treatment functional connectivity in the same networks, since the pre-treatment functional connectivity showed a prominent reduction in global functional connectivity in this group. Neuromuscular electrical stimulation (NMES); false discovery rate (FDR).
There was a statistically significant (p 0.05 FDR) medium to large (Cohen’s d = 0.5 medium; Cohen’s d = 0.8 large) increase in functional connectivity in the default mode, frontoparietal, salience, and sensorimotor networks for individuals in the NMES group compared to pre-treatment functional connectivity in the same networks (Figures 2, 3). This finding was even more evident as the pre-treatment functional connectivity assessment showed a prominent reduction in global functional connectivity in this group.
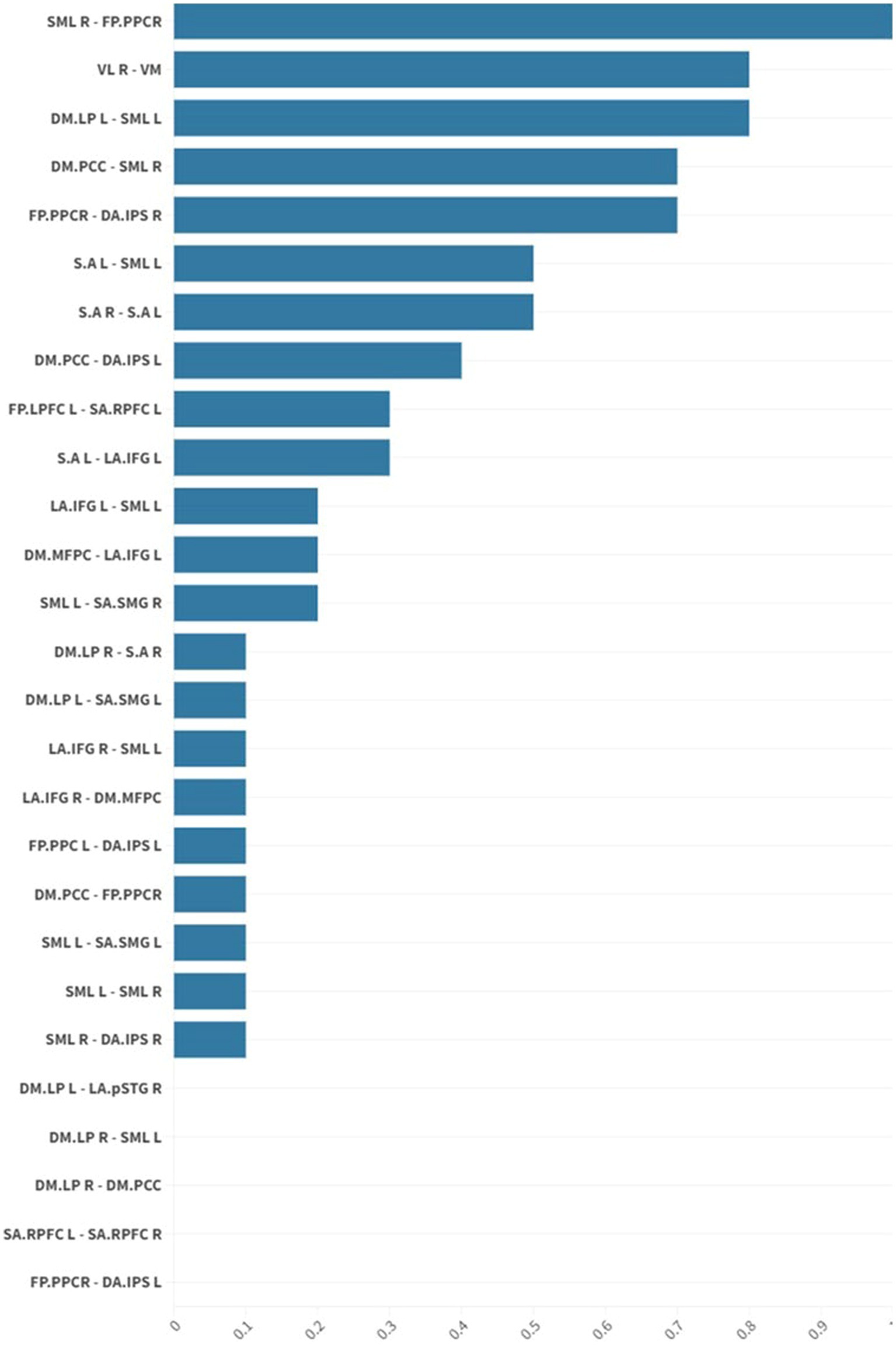
Figure 3. The graph shows mean effect size differences in resting-state network connectivity before and after intervention in the NMES group. Mean effect sizes are represented as average normalized differences in Cohen’s d. There was a statistically significant (p 0.05 FDR) medium to large (Cohen’s d = 0.5 medium; Cohen’s d = 0.8 large) increase in functional connectivity in the default mode, frontoparietal, salience, and sensorimotor networks for individuals in the usual care plus NMES treatment group. Neuromuscular electrical stimulation (NMES); false discovery rate (FDR).
Looking at the baseline muscle strength data, the NMES group seemed weaker than the control; for this reason, we performed an additional statistical analysis normalizing for baseline muscle strength, BMI and Klotho levels. There were no statistically significant differences in the primary outcome (Klotho levels at 3 months) between the two groups (NMES vs. usual care) (data not shown).
The above findings suggest the NMES protocol used in this study was likely to be insufficient to exert a significant additive effect over standard care alone on muscle and cognitive measures. For the next stage, we performed a supplementary analysis, stratifying patients according to the change (Δ) in Klotho (or other biomarker levels) from pre-to post-treatment [3 months after discharge (t1) relative to baseline assessment (t0)], regardless of the physical therapy protocol performed. Subjects were classified as “responders” (n°22 patients) if t1 Klotho values were increased compared to t0 assessment (Δ > 0; Δ range: 1.8–1099.32 pg/mL), and “non-responders” (n°31 patients) if follow-up values were decreased or unchanged from baseline (Δ ≤ 0; Δ range: −508.81 – −8.3). In Table 4, we report the demographic characteristics of both groups as well as the baseline muscle strength values and baseline biomarker values for responders and non-responders. We confirmed that the two groups of patients, responders and non-responders for Klotho, did not differ in most of the baseline clinical features, muscle strength or biomarker values. We only found that Klotho-responders had significantly lower baseline Klotho values than non-responders (p 0.002), a significantly lower percentage of diabetes (p 0.029) and finally that non-responders were predominantly male (p 0.0385).
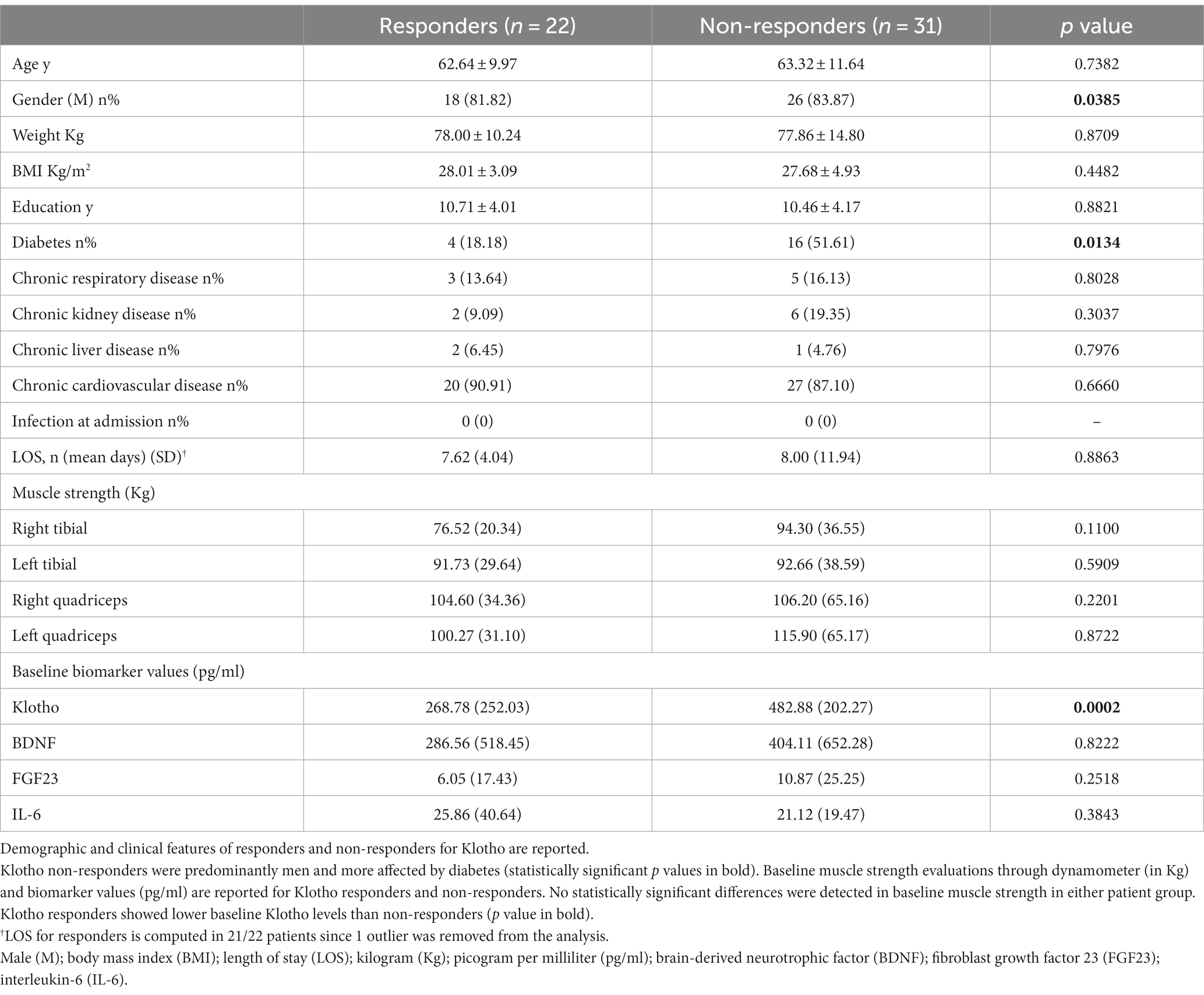
Table 4. Demographic, clinical features and baseline physical and laboratory evaluations of responders and non-responders for Klotho.
We performed the same classification (responders/non-responders) according to the other myokine trend values (data shown in Supplementary material, Results section: Supplementary Tables S2, S3); however, we mainly focused on Klotho, in consideration of the interesting findings reported below, which confirm the well-established neuroprotective role of this myokine (25, 26).
No significant differences were detected in cognitive performance between responders and non-responders when this classification was based on myokines other than Klotho (BDNF, FGF23, and IL-6 groups) (data shown in Supplementary material, Table S3). Klotho responders instead performed significantly better than non-responders on the delayed memory test (p 0.02). A similar trend was also observed for all the other cognitive tests included in RBANS, though the values did not reach statistical significance (Figure 4).
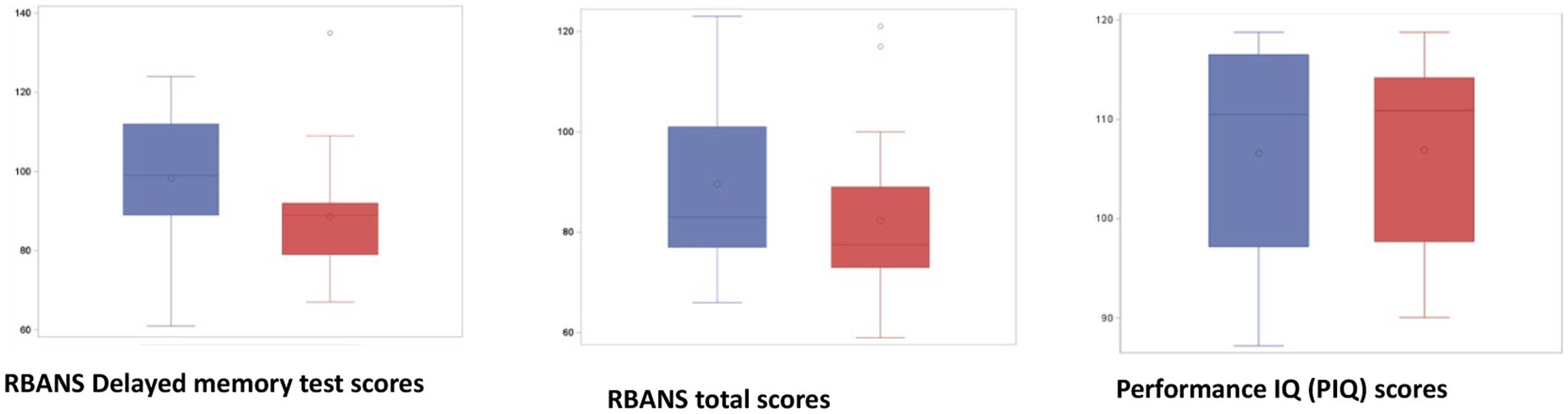
Figure 4. Partial and total RBANS scores in responders (blue) and non-responders for Klotho (red). Responders (blue) performed significantly better than non-responders (red) on the delayed memory test (p value = 0.02). A similar trend was also observed for all other cognitive tests included in RBANS without reaching significance. Performance of Premorbid Intelligence Quotient (PIQ) scores: The absence of differences between the PIQ of the two groups indicates that the differences in the NP tests are not attributable to different IQs. Repeatable Battery for the Assessment of Neuropsychological Status (RBANS); premorbid intelligence quotient (PIQ).
Discussion
According to the results of this study, the addition of an NMES protocol to the normal standard of care during hospitalization only increased IL-6 levels when compared to standard care alone. Many biological roles have been described for muscle-derived IL-6, such as regulation of glucose homeostasis, regulation of fat metabolism, as well as positive immunological suppressive effects (27–29), but we did not find any direct impact on cognition consistent with our results. None of the other neuroprotective myokines were significantly different between groups. Nor were there differences between groups when considering the long-term cognitive function, muscle strength, or structural changes according to brain MRI of CABG patients. However, we did find that both groups yielded improvements in the functional connectome according to functional brain MRI, and NMES amplified these effects. Neuromuscular electrical stimulation (NMES) has been extensively adopted in rehabilitation settings as a complement and/or substitute for voluntary strength training when this is unfeasible (30). A positive role of NMES has been demonstrated in healthy seniors for increasing muscle size and strength, inducing molecular changes like upregulation of insulin-like growth factor (IGF-1) and modulation of the muscle RING-finger protein-1 (MuRF-1) in the skeletal muscle (31); upregulation of blood BDNF and Klotho in the hippocampus of normal rats has also been observed (32). Conversely, in our subjects NMES did not modulate secretion into the blood of potential neuroprotective myokines (Klotho, BDNF, FGF23), except for IL-6, whose pleiotropic functions in different tissues and organs have been described (33). In this human clinical setting, we cannot verify if there are some local effects in upregulation of Klotho expression in the hippocampus through an additional pathway of neurogenesis induced by the peripheral nervous system and activated by muscle stimulation (32). Since our protocol of NMES intervention did not produce any macroscopic changes in terms of cognitive performance, we can indirectly hypothesize that this intervention paradigm does not induce any significant local molecular changes in the brain either.
In the second part of this study, we stratified subjects according to those who demonstrated a positive response to intervention (“responders”), as determined by circulating biomarker levels, compared to those who did not demonstrate a biomarker response to intervention (“non-responders”), independent of the type of rehabilitation intervention (standard or standard + NMES). We found that, of all the biomarkers evaluated, only patients with a change in Klotho levels performed better on all cognitive tests, significantly on the delayed memory index of the RBANS test, which is a measure of delayed recall and recognition of verbal and visual information, indicating good functioning of the long-term memory store. This result supports, in a clinical research setting, the neuroprotective role of Klotho as a cognitive-enhancing protein (22). Increased endocrine function of the active muscle is one hypothesis to explain the anti-aging effects of physical activity (muscle-brain endocrine axis) (9), but it is still unknown whether skeletal muscle contractile activity itself results in the local production and secretion of Klotho into the bloodstream, or if some other myokine is responsible for inducing Klotho expression in other tissues, such as the brain. In murine and human models, exercise has been shown to be a potent stimulus for increased plasma Klotho levels, but that response may be dependent on physical fitness level as well as age (9). There must be additional factors, other than age and muscle strength, influencing Klotho expression or upregulation since we did not find any differences in those parameters between the two groups (responders and non-responders). According to our results, diabetes and male gender were more frequent in the non-responder group; therefore, metabolic factors, sex factors and other additional factors are worth exploring as triggers or modulators of Klotho expression. Also, baseline levels of Klotho, which may depend on gene polymorphism, influence post-operative and post-treatment Klotho levels.
It has been suggested that the Klotho protein may protect against cognitive decline in aging and neurodegenerative disorders through multiple mechanisms: such as promoting optimal synaptic function via activation of N-methyl-d-aspartate (NMDA) receptor signaling, stimulating the antioxidant defense system, reducing inflammation, promoting autophagy and enhancing clearance of amyloid-β (34). A number of epidemiological studies have associated elevated Klotho levels with better health outcomes and even extended life span in humans. Higher plasma Klotho concentrations were associated with lower risk of meaningful decline and a smaller average decline in the Mini Mental State Examination (MMSE), but not in executive function or motor skills tests (35). Zhao et al. showed that Klotho overexpression inhibited PYD domain-containing protein 3 (NLRP3) inflammasome activation and promoted Aβ clearance through an increase in M2 type microglia and the regulation of Aβ transporters in amyloid precursor protein/presenilin 1 (APP/PS1) mice, with effectively relieved neuroinflammation and Aβ burden and ameliorated Alzheimer’s Disease (AD)-like phenotypes (36). Like our findings, previous in vivo studies in mice showed that upregulation of Klotho improves aging-related memory deficits (37).
We recognize that our study has several limitations, mainly concerning the small sample size, the lack of cognitive assessment before surgery, and the limited time of follow-up. Since only a few brain MRI scans were obtained, we could not explore any plausible laboratory-clinical and imaging (anatomical and functional) correlations, nor could we explore functional neuroimaging differences between the two groups of Klotho responders and non-responders. Unfortunately, neither the timing (candidate for urgent surgery or in the post-operative status) nor the setting (hospitalization as in-patients) were appropriate for a baseline cognitive assessment. Many patients had contraindications to MRI scans or refused a time-consuming neuroimaging assessment for their post-operative status. On the other hand, we demonstrated a cognition enhancing role of Klotho in a surgical setting with the potential to reverse, or at least reduce, post-operative cognitive decline after cardiac surgery. We can further speculate that having lower pre-operative plasma levels of Klotho can be a biomarker of lower post-operative values, potentially associated with worse memory performances. We still need to understand which factors induce Klotho expression, particularly modifiable factors, like a specific rehabilitation program, in order to design appropriate exercise protocols to counteract the deleterious effects of surgery, above all in high-risk populations (CABG candidates with low baseline Klotho levels). In addition, it is worth investigating in future studies whether specific cognitive function improvement is associated with concomitant anatomical or functional changes in specific brain regions.
The main achievement of our study is the confirmation, in a clinical setting, that Klotho has potential neuroprotective effects, preserving or improving memory function not only in physiological aging but also in this post-operative setting characterized by an increased risk of cognitive impairment.
It paves the way for further research questions, for example, relating to how an exercise program and factors other than physical exercise, for instance sex and age, influence the endocrine function of skeletal muscle, or testing the potential treatment application of Klotho as a drug in this patient category.
Data availability statement
The raw data supporting the conclusions of this article will be made available by the authors, without undue reservation.
Ethics statement
The studies involving humans were approved by Comitato Etico IRCCS Sicilia-Sezione ISMETT IRCCS srl. The studies were conducted in accordance with the local legislation and institutional requirements. The participants provided their written informed consent to participate in this study.
Author contributions
VL and FAm developed the ideas in the study, defined goals and endpoints, conceived the study design, and drafted the manuscript. GRu coordinated the research activities and data management. EL, MB, GI, DT, AS, FAv, GS, GeM, GPan, GRa, and MP collected the data and participated in data acquisition and interpretation of results. RA and FT contributed to the study methods and performed the data analysis. SA contributed with data collection and Tables/Figures drafting. GPar contributed with analysis and explanation of functional imaging. GV supported with the acquisition of funding and writing. PC was responsible for and supervised all research activities and edited the manuscript. All authors participated in drafting the manuscript sections and reviewing them critically, contributed to scientific and intellectual content, and approved the final manuscript.
Funding
This work was supported by the Italian Ministry of Economic Development (Horizon 2020 PON I&C 2014-2020, Lifestyle4Health F/050408/01-03/X32) and partially funded by the Italian Health Ministry, Ricerca Corrente 2023.
Acknowledgments
We acknowledge all patients as well as the team of physical therapists, the cardiac surgery unit, and UPMC Italy Language Services.
Conflict of interest
The authors declare that the research was conducted in the absence of any commercial or financial relationships that could be construed as a potential conflict of interest.
Publisher’s note
All claims expressed in this article are solely those of the authors and do not necessarily represent those of their affiliated organizations, or those of the publisher, the editors and the reviewers. Any product that may be evaluated in this article, or claim that may be made by its manufacturer, is not guaranteed or endorsed by the publisher.
Supplementary material
The Supplementary material for this article can be found online at: https://www.frontiersin.org/articles/10.3389/fneur.2023.1209905/full#supplementary-material
Footnotes
References
1. Greaves, D, Psaltis, PJ, Ross, TJ, Davis, D, Smith, AE, Boord, MS, et al. Cognitive outcomes following coronary artery bypass grafting: a systematic review and meta-analysis of 91, 829 patients. Int J Cardiol. (2019) 289:43–9. doi: 10.1016/j.ijcard.2019.04.065
2. McDonagh, DL, Berger, M, Mathew, JP, Graffagnino, C, Milano, CA, and Newman, MF. Neurological complications of cardiac surgery. Lancet Neurol. (2014) 13:490–502. doi: 10.1016/S1474-4422(14)70004-3
3. Caso, G, Vosswinkel, JA, Garlick, PJ, Barry, MK, Bilfinger, TV, and McNurlan, MA. Altered protein metabolism fgollowin coronary artery bypass graft (CABG) surgery. Clin Sci. (2008) 114:339–46. doi: 10.1042/CS20070278
4. Fontes Cerqueira, TC, Cerqueira Neto, ML, Cacau, LAP, Oliveira, GU, Silva Júnior, WMD, Carvalho, VO, et al. Ambulation capacity and functional outcome in patients undergoing neuromuscular electrical stimulation after cardiac valve surgery: a randomised clinical trial. Medicine. (2018) 97:e13012. doi: 10.1097/MD.0000000000013012
6. Pratesi, A, Tarantini, F, and Di Bari, M. Skeletal muscle: an endocrine organ. Clin Cases Miner Bone Metab. (2013) 10:11–4. doi: 10.11138/ccmbm/2013.10.1.011
7. Jo, D, Yoon, G, Kim, OY, and Song, J. A new paradigm in sarcopenia: cognitive impairment caused by imbalanced myokine secretion and vascular dysfunction. Biomed Pharmacother. (2022) 147:112636. doi: 10.1016/j.biopha.2022.112636
8. Severinsen, MCK, and Pedersen, BK. Muscle-organ crosstalk: the emerging roles of myokines. Endocr Rev. (2020) 41:594–609. doi: 10.1210/endrev/bnaa016. Erratum in: Endocr Rev. 2021; 42(1): 97-99
9. Avin, KG, Coen, PM, Huang, W, Stolz, DB, Sowa, GA, Dubé, JJ, et al. Skeletal muscle as a regulator of the longevity protein, Klotho. Front Physiol. (2014) 5:189. doi: 10.3389/fphys.2014.00189
10. Jensen, CS, Hasselbalch, SG, Waldemar, G, and Simonsen, AH. Biochemical markers of physical exercise on mild cognitive impairment and dementia: systematic review and perspectives. Front Neurol. (2015) 6:187. doi: 10.3389/fneur.2015.00187
11. Loprinzi, PD, and Frith, E. A brief primer on the mediational role of BDNF in the exercise-memory link. Clin Physiol Funct Imaging. (2019) 39:9–14. doi: 10.1111/cpf.12522
12. Prud'homme, GJ, Kurt, M, and Wang, Q. Pathobiology of the klotho antiaging protein and therapeutic considerations. Front Aging. (2022) 3:931331. doi: 10.3389/fragi.2022.931331
13. Drew, DA, Katz, R, Kritchevsky, S, Ix, JH, Shlipak, M, Newman, AB, et al. Fibroblast growth factor 23 and cognitive impairment: the health, aging, and body composition study. PLoS One. (2020) 15:e0243872. doi: 10.1371/journal.pone.0243872
14. Laszczyk, AM, Nettles, D, Pollock, TA, Fox, S, Garcia, ML, Wang, J, et al. FGF-23 deficiency impairs hippocampal-dependent cognitive function. eNeuro. (2019) 6:ENEURO.0469-18. 2019. doi: 10.1523/ENEURO.0469-18.2019
15. Li, DJ, Fu, H, Zhao, T, Ni, M, and Shen, FM. Exercise-stimulated FGF23 promotes exercise performance via controlling the excess reactive oxygen species production and enhancing mitochondrial function in skeletal muscle. Metabolism. (2016) 65:747–56. doi: 10.1016/j.metabol.2016.02.009
16. Iizuka, K, Machida, T, and Hirafuji, M. Skeletal muscle is an endocrine organ. J Pharmacol Sci. (2014) 125:125–31. doi: 10.1254/jphs.14R02CP
17. Oudbier, SJ, Goh, J, Looijaard, SMLM, Reijnierse, EM, Meskers, CGM, and Maier, AB. Pathophysiological mechanisms explaining the association between low skeletal muscle mass and cognitive function. J Gerontol A Biol Sci Med Sci. (2022) 77:1959–68. doi: 10.1093/gerona/glac121
18. Serna-Rodríguez, MF, Bernal-Vega, S, de la Barquera, JAO, Camacho-Morales, A, and Pérez-Maya, AA. The role of damage associated molecular pattern molecules (DAMPs) and permeability of the blood-brain barrier in depression and neuroinflammation. J Neuroimmunol. (2022) 371:577951. doi: 10.1016/j.jneuroim.2022.577951
19. Chen, B, Xie, G, Lin, Y, Chen, L, Lin, Z, You, X, et al. A systematic review and meta-analysis of the effects of early mobilization therapy in patients after cardiac surgery. Medicine. (2021) 100:e25314. doi: 10.1097/MD.0000000000025314
20. Iwatsu, K, Yamada, S, Iida, Y, Sampei, H, Kobayashi, K, Kainuma, M, et al. Feasibility of neuromuscular electrical stimulation immediately after cardiovascular surgery. Arch Phys Med Rehabil. (2015) 96:63–8. doi: 10.1016/j.apmr.2014.08.012
21. Iwatsu, K, Iida, Y, Kono, Y, Yamazaki, T, Usui, A, and Yamada, S. Neuromuscular electrical stimulation may attenuate muscle proteolysis after cardiovascular surgery: a preliminary study. J Thorac Cardiovasc Surg. (2017) 153:373–379.e1. doi: 10.1016/j.jtcvs.2016.09.036
22. Kitamura, H, Yamada, S, Adachi, T, Shibata, K, Tamaki, M, Okawa, Y, et al. Effect of perioperative neuromuscular electrical stimulation in patients undergoing cardiovascular surgery: a pilot randomized controlled trial. Semin Thorac Cardiovasc Surg. (2019) 31:361–7. doi: 10.1053/j.semtcvs.2018.10.019
23. Myers, J, Prakash, M, Froelicher, V, Do, D, Partington, S, and Atwood, JE. Exercise capacity and mortality among men referred for exercise testing. N Engl J Med. (2002) 346:793–801. doi: 10.1056/NEJMoa011858
24. Ojima, M, Takegawa, R, Hirose, T, Ohnishi, M, Shiozaki, T, and Shimazu, T. Hemodynamic effects of electrical muscle stimulation in the prophylaxis of deep vein thrombosis for intensive care unit patients: a randomized trial. J Intensive Care. (2017) 5:9. doi: 10.1186/s40560-016-0206-8
25. Ji, N, Luan, J, Hu, F, Zhao, Y, Lv, B, Wang, W, et al. Aerobic exercise-stimulated klotho upregulation extends life span by attenuating the excess production of reactive oxygen species in the brain and kidney. Exp Ther Med. (2018) 16:3511–7. doi: 10.3892/etm.2018.6597
26. Karizmeh, MS, Shabani, M, Shabani, M, Sardari, M, Babaei, JF, Nabavizadeh, F, et al. Preconditioning exercise reduces hippocampal neuronal damage via increasing klotho expression in ischemic rats. Brain Res Bull. (2022) 188:133–42. doi: 10.1016/j.brainresbull.2022.07.022
27. Febbraio, MA, and Pedersen, BK. Muscle-derived interleukin-6: mechanisms for activation and possible biological roles. FASEB J. (2002) 16:1335–47. doi: 10.1096/fj.01-0876rev
28. Priest, C, and Tontonoz, P. Inter-organ cross-talk in metabolic syndrome. Nat Metab. (2019) 1:1177–88. doi: 10.1038/s42255-019-0145-5
29. Febbraio, MA, and Pedersen, BK. Who would have thought - myokines two decades on. Nat Rev Endocrinol. (2020) 16:619–20. doi: 10.1038/s41574-020-00408-7
30. Maffiuletti, NA, Minetto, MA, Farina, D, and Bottinelli, R. Electrical stimulation for neuromuscular testing and training: state-of-the art and unresolved issues. Eur J Appl Physiol. (2011) 111:2391–7. doi: 10.1007/s00421-011-2133-7
31. Kern, H, Barberi, L, Löfler, S, Sbardella, S, Burggraf, S, Fruhmann, H, et al. Electrical stimulation counteracts muscle decline in seniors. Front Aging Neurosci. (2014) 6:189. doi: 10.3389/fnagi.2014.00189
32. Dalise, S, Cavalli, L, Ghuman, H, Wahlberg, B, Gerwig, M, Chisari, C, et al. Biological effects of dosing aerobic exercise and neuromuscular electrical stimulation in rats. Sci Rep. (2017) 7:10830. doi: 10.1038/s41598-017-11260-7
33. Muñoz-Cánoves, P, Scheele, C, Pedersen, BK, and Serrano, AL. Interleukin-6 myokine signaling in skeletal muscle: a double-edged sword? FEBS J. (2013) 280:4131–48. doi: 10.1111/febs.12338
34. Hanson, K, Fisher, K, and Hooper, NM. Exploiting the neuroprotective effects of α-klotho to tackle ageing-and neurodegeneration-related cognitive dysfunction. Neuronal. Signals. (2021) 5:NS20200101. doi: 10.1042/NS20200101
35. Shardell, M, Semba, RD, Rosano, C, Kalyani, RR, Bandinelli, S, Chia, CW, et al. Plasma klotho and cognitive decline in older adults: findings from the InCHIANTI study. J Gerontol A Biol Sci Med Sci. (2016) 71:677–82. doi: 10.1093/gerona/glv140
36. Zhao, Y, Zeng, CY, Li, XH, Yang, TT, Kuang, X, and Du, JR. Klotho overexpression improves amyloid-β clearance and cognition in the APP/PS1 mouse model of Alzheimer’s disease. Aging Cell. (2020) 19:e13239. doi: 10.1111/acel.13239
37. Zhou, HJ, Zeng, CY, Yang, TT, Long, FY, Kuang, X, and Du, JR. Lentivirus-mediated klotho up-regulation improves aging-related memory deficits and oxidative stress in senescence-accelerated mouse prone-8 mice. Life Sci. (2018) 200:56–62. doi: 10.1016/j.lfs.2018.03.027
38. Behzadi, Y, Restom, K, Liau, J, and Liu, TT. A component based noise correction method (Comp Cor) for BOLD and perfusion based fMRI. Neuroimage. (2007) 37:90–101. doi: 10.1016/j.neuroimage.2007.04.042
39. Colombo, L, Sartori, G, and Brivio, C. Stima del quoziente intellettivo tramite l'applicazione del TIB (Test Breve di Intelligenza). Giornale Italiano di Psicologia. (2002) 3:613–38. doi: 10.1421/1256
40. Nieto-Castanon, A, and Whitfield-Gabrieli, S. CONN functional connectivity toolbox: RRID SCR_009550, release 22. Boston, MA: Hilbert Press (2022).
41. Randolph, C, Tierney, MC, Mohr, E, and Chase, TN. The repeatable battery for the assessment of neuropsychological status (RBANS): preliminary clinical validity. J Clin Exp Neuropsychol. (1998) 20:310–9. doi: 10.1076/jcen.20.3.310.823
42. Talwar, N, Churchill, NW, Hird, MA, Tam, F, Graham, SJ, and Schweizer, TA. Functional magnetic resonance imaging of the trail-making test in older adults. PLoS One. (2020) 15:e0232469. doi: 10.1371/journal.pone.0232469
43. Wood, MD, Maslove, DM, Muscedere, J, Scott, SH, and Boyd, JG. Canadian critical care trials group. Robotic technology provides objective and quantifiable metrics of neurocognitive functioning in survivors of critical illness: a feasibility study. J Crit Care. (2018) 48:228–36. doi: 10.1016/j.jcrc.2018.09.011
Glossary
Keywords: post-operative cognitive decline (POCD), neuromuscular electrical stimulation (NMES), coronary artery bypass grafting (CABG), myokines, klotho
Citation: Lo Re V, Russelli G, Lo Gerfo E, Alduino R, Bulati M, Iannolo G, Terzo D, Martucci G, Anzani S, Panarello G, Sparacia G, Parla G, Avorio F, Raffa G, Pilato M, Speciale A, Agnese V, Mamone G, Tuzzolino F, Vizzini GB, Conaldi PG and Ambrosio F (2023) Cognitive outcomes in patients treated with neuromuscular electrical stimulation after coronary artery bypass grafting. Front. Neurol. 14:1209905. doi: 10.3389/fneur.2023.1209905
Edited by:
Jimmy Thomas Efird, The University of Newcastle, AustraliaReviewed by:
Zhi-Yong Wang, First Affiliated Hospital of Fujian Medical University, ChinaIrina Tarasova, Research Institute for Complex Issues of Cardiovascular Diseases, Russia
Copyright © 2023 Lo Re, Russelli, Lo Gerfo, Alduino, Bulati, Iannolo, Terzo, Martucci, Anzani, Panarello, Sparacia, Parla, Avorio, Raffa, Pilato, Speciale, Agnese, Mamone, Tuzzolino, Vizzini, Conaldi and Ambrosio. This is an open-access article distributed under the terms of the Creative Commons Attribution License (CC BY). The use, distribution or reproduction in other forums is permitted, provided the original author(s) and the copyright owner(s) are credited and that the original publication in this journal is cited, in accordance with accepted academic practice. No use, distribution or reproduction is permitted which does not comply with these terms.
*Correspondence: Vincenzina Lo Re, dmxvcmVAaXNtZXR0LmVkdQ==