- Department of Neurology, Affiliated Nanjing Brain Hospital, Nanjing Medical University, Nanjing, China
Introduction: Transcranial magnetic stimulation (TMS) is a non-invasive intervention that holds promise for improving cognitive function in individuals with Alzheimer's disease (AD). However, the effectiveness of this therapy and the optimal TMS parameters has not reached a consensus. The purpose of the meta-analysis was to systematically discern the effectiveness of different components of TMS protocols on cognitive improvement in patients with mild cognitive impairment (MCI) and AD.
Methods: The meta-analysis was preregistered on Prospero (registration number: CRD42022345482). PubMed, Web of Science, Science Direct, and Cochrane Library databases were used to search, screen and identify eligible studies with the following keywords: Transcranial Magnetic Stimulation OR TMS OR theta burst stimulation AND Alzheimer OR Alzheimers OR Alzheimer's OR mild cognitive impairment OR MCI. Randomized controlled trials (RCTs) of participants with accepted standardized diagnostic criteria were searched by two authors independently. The risk of bias was assessed using an adapted Cochrane Risk of Bias tool. Standardized mean difference (SMD) and 95% confidence interval (CI) were calculated using the random-effects models. Subgroup analyses were performed to investigate the influential factors.
Results: A total of 21 studies and 25 trials were included in this meta-analysis. The findings revealed a significant overall cognition improvement of real stimulation compared with sham stimulation (short-term effects: SMD, 0.91; 95% CI 0.44–1.38; P < 0.01; long-lasting effects: SMD, 0.91; 95% CI 0.27–1.55; P < 0.01). Subgroup analysis demonstrated that stimulation of the left dorsolateral prefrontal cortex and bilateral cerebellums, as well as moderate frequency stimulation (5 Hz and 10 Hz) on mild and moderate cognitive impairment patients, were more effective than other TMS protocols. However, the additional application of cognitive training showed no significant improvement.
Conclusion: Cognitive improvement effect of TMS was demonstrated in MCI and AD patients in both short-term assessment and long-lasting outcomes, and the efficiency of TMS is affected by the stimulation frequency, stimulation site, and participant characteristics. Further RCTs are needed to validate the findings of our subgroup analysis.
Systematic review registration: https://www.crd.york.ac.uk/prospero/display_record.php?ID=CRD42022345482, identifier: CRD42022345482.
Introduction
Alzheimer's disease is the most prevalent neurodegenerative disease, characterized by progressive deterioration of memory and other cognitive function, accompanied by abnormal neuropsychiatric behavior (1). AD pose a substantial healthcare challenge globally, affecting ~4% of the elderly population worldwide up to 2025 and developing in an estimated 6, 7 million people annually (2). However, currently approved clinical treatments for AD have limited efficacy (3) and development of pharmacological interventions has faced significant challenges over the past two decades (4). Consequently, novel therapeutic approaches have gained increasing attention. and non-invasive electrical brain stimulation (NIBS) has emerged as a potential alternative (5).
Transcranial magnetic stimulation (TMS), the most common form of NIBS, modulates cortical excitability and neuroplasticity by inducing electromagnetic pulses to the brain (6, 7). TMS can be classified into various forms according to the frequency and interval of stimulation. Among these, conventional low and high frequency repetitive transcranial magnetic stimulation (rTMS) and patterned rTMS, such as theta-burst stimulation (TBS) (8), are the most commonly used for therapeutic purposes and serve as the primary focus of our research. Previous studies have demonstrated that high-frequency (HF) rTMS (>1 Hz) or intermittent theta-burst stimulation induce an enhanced effect, whereas low-frequency (LF) rTMS ( ≤ 1 Hz) or continuous theta-burst stimulation suppress neural activity (9, 10). TMS has proven its safety and barely has contraindications (11), allowing for its widespread clinical application in the treatment of patients with mild cognitive impairment (MCI) and AD. However, the precise therapeutic effects and optimal TMS parameters remain debatable and thus necessitate further research. Therefore, this meta-analysis aimed to systematically analyze the effectiveness of different components of TMS protocols in enhancing cognitive function in patients with MCI and AD.
To date, 15 meta-analyses have summarized the effects of rTMS on patients with AD or MCI. Hovever, none of these meta-analyses included TBS as the treatment modality. Among the existing meta-analyses, five primarily focused on comparing the therapeutic effects of rTMS with other non-invasive interventions, such as transcranial direct current stimulation (tDCS) and cognitive training (CT) (12–16). These studies reported effective results for rTMS and controversial effects for DCS and CT in AD patients. Five studies evaluated the effects of different stimulation sites on the efficacy of rTMS. Most of these studies compared the effects of left and right dorsolateral prefrontal cortex (DLPFC) stimulation or DLPFC stimulation with other brain regions (17–20). Only one study explored the effects of specific brain regions, comparing memory and general cognition improvement in the DLPFC and temporo-parietal regions (21), concluded significant memory improvement only in the DLPF. However, these studies have yielded inconsistent conclusions and lack a comprehensive comparison of stimulation sites.
The present meta-analysis was strictly based on randomized controlled trials (RCTs) and included 21 studies (25 trials), surpassing the number of previous studies (The minimum number of included TMS studies was five and the maximum was 13). Notably, we incorporated one study that investigated a novel stimulation site not previously explored in AD patients (22). In addition, we performed comprehensive subgroup analyses considering stimulation parameters, trial designs and beneficiary groups to elucidate the appropriate TMS protocols and provide guidance for the clinical application of TMS in AD treatment.
Materials and methods
Search strategy
The meta-analysis was preregistered on Prospero (registration number: CRD42022345482, Available from: https://www.crd.york.ac.uk/prospero/display_record.php?ID=CRD42022345482) and conducted on October 1, 2022, using the PubMed, Web of Science, Science Direct, and Cochrane Library databases with the following keywords: Transcranial Magnetic Stimulation OR TMS OR theta burst stimulation AND Alzheimer OR Alzheimers OR Alzheimer's OR mild cognitive impairment OR MCI.
Inclusion and exclusion criteria
Two investigators (YY and TW) independently searched for RCTs that compared active TMS treatment with sham treatment in patients diagnosed with AD or MCI, based on accepted standardized diagnostic criteria. The inclusion criteria were as follows: (1) participants diagnosed with AD or MCI according to accepted standardized criteria (e.g.,—DSM, NIAAA, NINCDS-ADRDA, or Petersen's criteria for MCI); (2) presence of a sham-controlled condition with either parallel or cross-over design; (3) outcome measures based on cognitive function assessments; (4) studies published in English; and (5) studies limited to human subjects. The exclusion criteria were as follows: (1) non-primary studies, such as reviews, meta-analyses, editorials, conference abstracts, case studies, and protocols; (2) absence of TMS-sham-controlled groups; (3) TMS not intended as a treatment; (4) cognitive impairment due to non-AD conditions (e.g., Parkinson's disease, stroke); and (5) unavailability of the necessary data.
Data extraction
Two authors (YY and YW) independently extracted the data using a predesigned data extraction form, and any discrepancies were resolved through consensus. The extracted data included sample size, age, sample characteristics, TMS protocol, cognitive performance outcomes, and timing of the outcome assessments. In cases where the mean and standard deviation (SD) of cognitive outcomes were not provided directly, the corresponding authors were contacted or the values were calculated using formulas from the Cochrane Handbook 5.1.0, Chapter 16.1.3.2 (23). The Coefficient refers to the correlation coefficients, and the value Coefficient was imputed from another study (22) with complete data included in this meta-analysis (23), where the coefficient value was determined to be 0.8.
Evaluation of risk of bias
The risk of bias was assessed using items adapted from the Cochrane Risk of Bias tool (24). The assessment criteria included (1) use of accepted standardized criteria for AD/MCI diagnosis; (2) specific methods for random sequence generation; (3) blinding of personnel and participants; 4) blinding of outcome assessment; (5) similarity of characteristics between the active and sham groups; and (6) reporting of participant dropout numbers. Each study was assigned a quality score, with a score of 1 indicating compliance with the assessment, 0 indicating non-compliance, and “un” indicating that the information was not reported. A higher score indicates better quality. The methodological quality of each included study was independently assessed by two authors (YY and MT) and any disagreements were resolved through discussion.
Statistical analysis
Statistical analysis was performed using the Stata 16.0 statistical software (Stata Corp., College Station, TX, USA). The standardized mean difference (SMD) and 95% confidence interval (CI) were calculated to summarize the effect size of the clinical scores pre- and post-treatment in the experimental and control groups. We preferred using Hedges's g (25) for SMD estimation because of its reduced bias in small sample sizes. The DerSimonian-Laird method was used to synthesize SMD estimates (26). The heterogeneity of the included studies was assessed using Cochran's Q statistic and the I2 test. The random-effects model was applied to obtain a more conservative result.
Subgroup analyses
Additional subgroup comparisons were conducted to determine appropriate TMS protocols. we compared both short term and long-lasting efficacy of TMS in the following categories: (1) stimulation sites including the left and bilateral DLPFC, parietotemporal area, bilateral cerebellum and precuneus; (2) TMS frequency including 1 Hz, 5 Hz, 10 Hz, 20 Hz, and 50 Hz iTBS; (3) TMS with or without cognitive training; and 4) patients with mild, mederate or severe cognitive impairment.
Results
Information on the included studies
A total of 2,908 studies were initially identified through the primary search. After removing duplicates (n = 778), irrelevant studies (n = 1,595), and non-RCT studies (n = 486), 49 studies remained for the full-text screening. Following the application of exclusion criteria, including studies without cognitive assessment (n = 2), non-clinical studies (n = 2), lack of TMS-sham-controlled groups (n = 9), studies not intended for treatment (n = 7), unclear diagnostic criteria (n = 4), and unavailable data (n = 4),a total of 21 studies (25 trials) involving 806 MCI and AD patients were included in this analysis. A flow diagram illustrating the study selection process was conducted according the PRISMA statement (Figure 1).
Among the included studies, Most studies reported only one trial, with one reported two trials (27) and one reported four trials (28). Eighteen applied a parallel design (22, 27–41), whereas three studies utilized a crossover patterns (42–44). The majority of studies employed moderate-to high-frequency rTMS, with one study using low-frequency rTMS (LFrTMS) (28) and one using intermittent theta-burst stimulation (iTBS) (35). Single site TMS was applied in 11 studies, including left dorsolateral prefrontal cortex (L-DLPFC) (30–33, 35, 38, 40, 42, 43) and the left inferior parietal lobule (37, 45), Four studies used bilateral stimulation (22, 28, 44, 46) and six studies employed multiple stimulation sites: Broca and Wernicke area (29, 36, 39, 41), bilateral dorsolateral prefrontal cortex (DLPFC) (29, 36, 39, 41), bilateral parietal somatosensory association cortex (pSAC) (36, 41), bilateral inferior parietal lobule (IPL) (29, 39), parietal and posterior temporal area (27), and L-DLPFC and left lateral temporal lobe (LTL) (34). Cognitive outcomes assessed in these studies studies included Mini Mental Status Examination (MMSE) (22, 28, 30–32, 35, 37, 40, 43, 44), Alzheimer's disease Assessment Scale-Cognitive (ADAS-cog) (29, 34, 36, 39, 41, 47), Montreal Cognitive Assessment (MOCA) (38, 42), and Rivermead Behavioral Memory Test (RBMT) (33). The basic characteristics of these articles are summarized in Table 1.
Quality assessment of the studies
Table 2 shows that all the included studies had similar active and sham groups, and three-quarters of the studies described specific random allocation methods. Seven studies employed a triple-blind design, whereas four studies utilized a double-blind design. The dropout number of patients was reported in 18 studies. Studies with a high potential risk of bias (scores < 4) were removed for sensitivity analysis.
Effects of TMS on AD
Given that most of the included studies reported short-term results (< 3 days) as well as follow-up (>4 weeks) outcomes, the pooled effects of TMS on the cognitive improvement in MCI and AD patients were assessed for both outcome timings.
The short-term effects were evaluated in 24 trials (20 studies) and demonstrated an overall significant improvement (SMD, 0.91; 95% CI 0.44–1.38; P < 0.01) in the random-effects model analysis. Of the 24 trials, 10 showed statistically significant positive effects (Figure 2). Long-lasting effects (SMD, 0.91; 95% CI 0.27–1.55; P < 0.01) were observed in 17 trials (13 studies), with significant positive effects in 9 trials and negative effects in 2 trials. However, the difference in the effects between the short-term and long-lasting outcomes was not statistically significant (Q = 0.00, P < 1.00). Substantial heterogeneity was found in both short-term (H2 = 8.58, I2 = 88.34%) and long-lasting effects (H2 = 9.19, I2 = 89.21%). Therefore, subgroup analyses were performed explore the potential sources of heterogeneity and assess the efficacy of various factors on cognitive improvement.
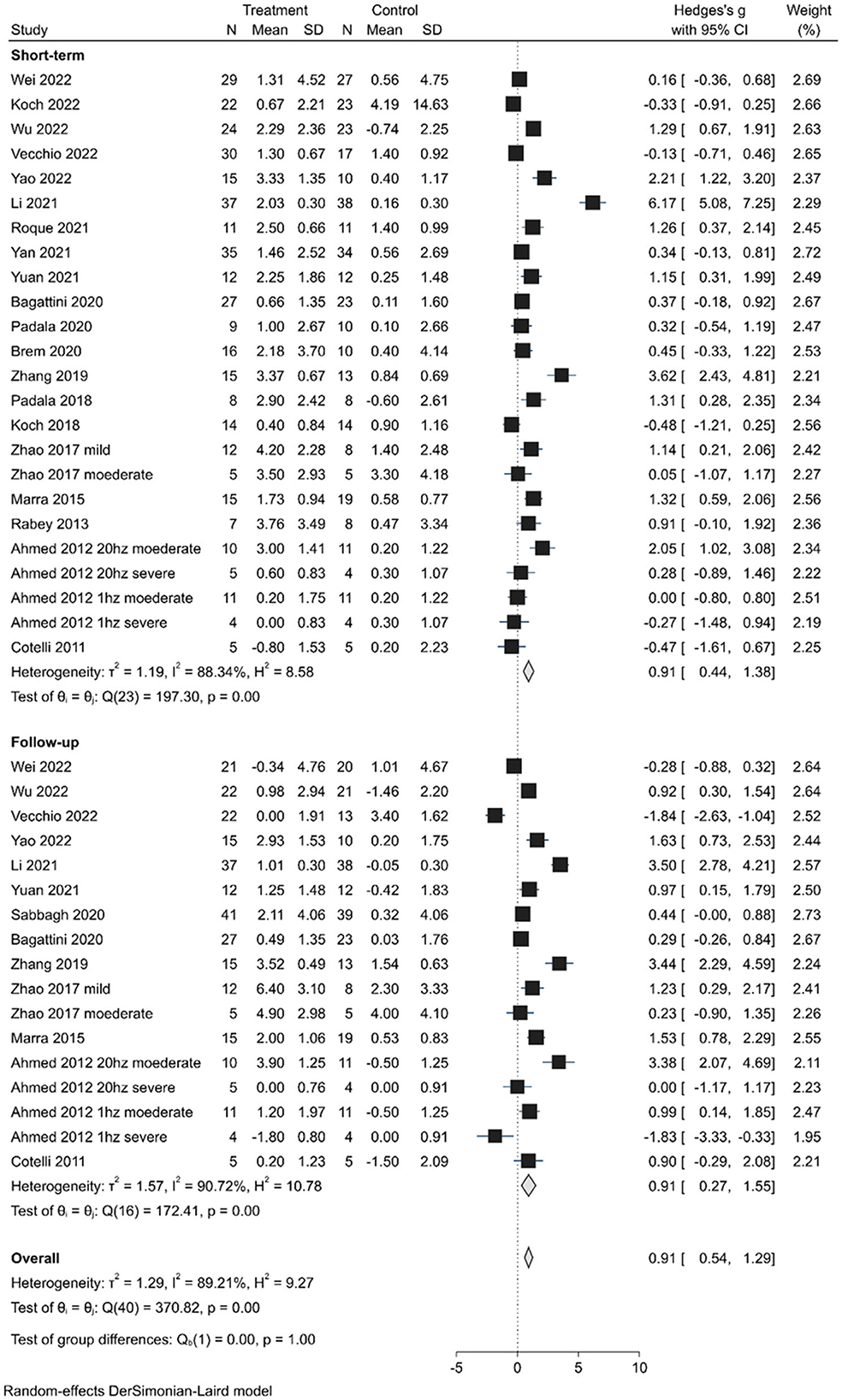
Figure 2. Forest plot depicting the pooled short-term and long-lasting effects of TMS on cognition outcomes.
Subgroup analyses
Figure 3 shows the subgroup analysis of the stimulation sites. The results indicate that global cognitive function could be significantly improved in the short- and long-lasting terms with left/bilateral DLPFC stimulation (short-term effects: SMD, 1.13; 95% CI 0.38–1.89; P < 0.01; long-lasting effects: SMD, 1.10; 95% CI 0.29–1.92; P < 0.01) and bilateral cerebellum stimulation (short-term effects: SMD, 2.21; 95% CI 1.22–3.20; P < 0.01; long-lasting effects: SMD, 1.63; 95% CI 0.73–2.53; P < 0.01). However, parietotemporal area stimulation (short-term effects: SMD, 0.36; 95% CI −0.03–0.71; P = 0.31; long-lasting effects: SMD, 0.35; 95% CI −0.60–1.30; P = 0.16) and precuneus stimulation (short-term effects: SMD, −0.39; 95% CI −0.84–0.07; P = 0.75) did not show significant improvement. Subgroup analyses comparing multiple site and bilateral site stimulation with single-site stimulation did not demonstrate larger responses (short-term effects: Test of group differences: Q = 1.02, P = 0.60; long-lasting effects: Test of group differences: Q = 0.41, P = 0.82) (Supplementary Figures 1, 2). Furthermore, stimulation of the left DLPFC alone, which showed the largest effect size (short-term effects: SMD, 1.39; 95% CI 0.43–2.36; long-lasting effects: SMD, 1.35; 95% CI 0.39–2.32), showed no difference (short-term effects: Test of group differences: Q = 3.61, P = 0.16; long-lasting effects: Test of group differences: Q = 0.45, P = 0.80) with bilateral DLPFC stimulation and multiple site stimulation involving the left/bilateral DLPFC (Supplementary Figures 3, 4). Additionally, although stimulation of the bilateral cerebellum showed larger responses (short-term effects: SMD, 2.21; 95% CI 1.22–3.20; long-lasting effects: SMD, 1.39; 95% CI 0.55–2.23) than left DLPFC stimulation, the difference was not statistically significant (short-term effects: Test of group differences: Q = 1.34, P = 0.25; long-lasting effects: Test of group differences: Q = 0.17, P = 0.68) (Supplementary Figures 5, 6).
The stimulation frequency of rTMS ranged from 1 Hz to 20 Hz, in addition to a study that applied 50 Hz intermittent theta-burst stimulation (iTBS). Subgroup analysis of stimulation frequency revealed that cognitive outcomes were improved in the short-term responses in patients receiving 5 Hz stimulation (SMD, 1.71; 95% CI 0.78–2.64; P < 0.01), 10 Hz stimulation (SMD, 0.84; 95% CI 0.33–1.35; P < 0.01) and iTBS (SMD, 1.29; 95% CI 0.67–1.91; P < 0.01), but not in 1 Hz stimulation (SMD, −0.08; 95% CI −0.75–0.59; P = 0.71) or 20 Hz stimulation (SMD, 0.96; 95% CI −0.20–2.11; P = 0.17). In terms of long-lasting effects, all stimulation frequencies achieved statistical significance (5 Hz: SMD, 1.63; 95% CI 0.73–2.53; P < 0.01; 10 Hz: SMD, 1.56; 95% CI 0.66–2.46; P < 0.01; 20 Hz: SMD, 1.36; 95% CI 0.19–2.52; P < 0.01; iTBS: SMD, 0.92; 95% CI 0.30–1.54; P < 0.01) expect for 1 Hz stimulation (SMD, −0.35; 95% CI −3.11–2.41; P = 0.84).
Furthermore, significant improvements in cognitive function were observed with TMS combined with CT (short-term effects: SMD, 0.86; 95% CI 0.26–1.47; P < 0.01; long-lasting effects: SMD, 1.08; 95% CI 0.34–1.82; P < 0.01) or without CT (short-term effects: SMD, 1.37; 95% CI 0.31–2.42; P < 0.01; long-lasting effects: SMD, 1.17; 95% CI 0.45–1.89; P < 0.01). The TMS combination with CT showed no significant difference with TMS without CT (short-term effects: Test of group differences: Q = 0.01, P = 0.91; long-lasting effects: Test of group differences: Q = 0.26, P = 0.61). Subgroup analysis of CT vs. no CT in MCI patients also did not reveal a significant difference between the groups (short-term effects: Test of group differences: Q = 0.09, P = 0.76; long-lasting effects: Test of group differences: Q = 0.07, P = 0.80) (Supplementary Figures 7, 8). Additionally, in the subgroup analysis of patient characteristics, patients with mild cognitive impairment (short-term effects: SMD, 0.64; 95% CI 0.26–1.02; P < 0.01; long-lasting effects: SMD, 0.99; 95% CI 0.47–1.50; P < 0.01) or moderate cognitive impairment (short-term effects: SMD, 1.67; 95% CI 0.50–2.84; P < 0.01; long-lasting effects: SMD, 1.85; 95% CI 0.47–3.23; P < 0.01) showed greater improvement than participants with severe cognitive impairment (short-term effects: SMD, 0.01; 95% CI −0.83–0.86; P = 0.52; long-lasting effects: SMD, −0.85; 95% CI −2.64–0.4; P = 0.06).
Publication bias and sensitivity analysis
Figure 4, displays the results of Egger's test (P = 0.115) and visual inspection of the funnel plot, which did not indicate any significant publication bias in the primary outcome. Therefore, no fill-and-trim procedure was performed to adjust the effect sizes. Additionally, sensitivity analysis was conducted to examine the impact of each included study on the overall results. Figure 5 illustrates the results of omitting each study. It can be observed that the exclusion of any specific study did not lead to substantial changes in the overall findings, indicating the robustness and reliability of our results.
Discussion
This meta-analysis revealed the following: (1) Stimulation of the DLPFC and cerebellum had short-term and long-lasting positive effects on general cognitive function. (2) TMS protocols using moderate frequency stimulation (5 Hz and 10 Hz) and iTBS demonstrated larger therapeutic effects. (3) CT did not yield any additional effects. (4) The population with mild to moderate cognitive impairment responded better to stimulation than patients with severe AD.
Our study is the first to demonstrate that the cerebellum, in addition to the DLPFC, is an effective TMS site for AD treatment. Previous studies predominantly focused on bilateral or left DLPFC stimulation as the TMS site (28, 30–33, 35, 38, 40, 42, 43). Other reported stimulation sites, such as the parietotemporal area (27, 37) and the precuneus (44) showed limited improvement in global cognition. Existing meta-analyses on TMS efficacy in AD treatment primarily compared single and multiple stimulation sites (16, 48, 49) or left, right, and bilateral hemisphere stimulation (17, 50). Our subgroup analyses revealed no significant difference between 10 trials using single-site stimulation and 6 trials using bilateral site stimulation or 6 trials using multiple site stimulation. Jiang et al. (17) reported positive effects of left/bilateral DLPFC stimulation. Our comparisons of the left DLPFC, bilateral DLPFC, and multiple site stimulation (including the left/bilateral DLPFC) indicated that the left DLPFC showed the largest improvement. However, bilateral cerebellum stimulation had a larger effect size than left DLPFC stimulation, although the difference was not statistically significant. Subgroup analyses of four trials using parietotemporal stimulation and two trials using precuneus stimulation showed no significant therapeutic effects. Thus, among all reported stimulation sites, the left DLPFC and cerebellum stimulation were more effective. Although only one study (22) applied bilateral cerebellum stimulation, the potential of cerebellar stimulation in AD treatment deserves more attention, and further investigation is needed in future studies.
Regarding stimulation frequency, our subgroup analyses indicated that moderate frequency stimulation (5 Hz and 10 Hz) and theta-burst stimulation might have superior efficacy for general cognition compared to low-frequency (1 Hz) and high-frequency (20 Hz) stimulation. Among these frequencies, the 5 Hz stimulation demonstrated the highest effect size for both short-term and long-lasting effects. The 20 Hz stimulation was only significant for the long-lasting effects, and its effect size was lower than that of the 10 Hz stimulation. Theta-burst stimulation exhibited high short-term effects but the lowest long-lasting effects. Generally, low-frequency ( ≤ 1 Hz) is considered to suppres excitability within the targeted brain region, while higher frequency stimulation is considered enhancing, but the inhibitory or excitatory effects on brain areas do not directly correspond to a decrease or improvement in cognitive function. The improved performance may be observed with an optimum frequency depending on the task demands and stimulation area (51). Most studies considered 5, 10, and 20 Hz as high-frequency stimulation and concluded that high-frequency stimulation has excitatory effects (12, 17, 21, 50, 52). Wang et al. (15) considered 10 Hz as a moderate frequency and 20 Hz as a high-frequency and their findings contradicted our research, suggesting that 20 Hz stimulation induced better cognitive improvement than 10 Hz rTMS. Several studies have also reported blockade or disruption of brain function with high-frequency TMS over certain brain regions (53, 54). Regarding the duration of treatment required for effectiveness, all frequencies of rTMS demonstrated positive significance for long-lasting effects, except for 1 Hz, probably due to the short-lived block effects of high-frequency TMS. Thus, this meta-analysis is the first to demonstrate that moderate-frequency (5 Hz and 10 Hz) rTMS may be more suitable than high- and low- frequency rTMS for AD treatment.
The combination of CT with multisite rTMS has shown potential effectiveness in enhancing cognition (55). However, our findings did not provide evidence for additional cognitive enhancement through CT, independent of TMS. Previous meta-analyses have reported both positive (14) and not positive additional effects (12, 16). Wang et al. proposed that the positive effects of combining CT can be confounded by the number of stimulation sessions (14). Chu et al. (12) also observed a lack of efficacy when CT was combined with TMS in different cognitive domains. Considering that CT may be more effective in the early stages of AD, we conducted a subgroup analysis comparing rTMS combined with CT and rTMS alone in individuals with mild cognitive impairment (MCI) and mild AD. No significant differences were observed between the two subgroups. However, it is important to note that the effect of CT cannot be deemed null because the recovery effect of CT may not have been sufficient to induce a significant additive effect in conjunction with TMS.
Regarding participant characteristics, our subgroup analysis revealed that patients with severe AD may not experience as much improvement as individuals with mild-to-moderate AD through TMS, which is consistent with previous meta-analyses (12, 16, 21).
As research on TMS progress, novel methodologies have emerged (22) and previous studies have been enriched, leading to new changes in the analysis of TMS treatment effects on MCI and AD patients. The inclusion of recently published RCTs comprehensive comparisons of factors influencing TMS efficacy, have resulted in different conclusions compared with previous studies. This meta-analysis is the first meta-analysis to demonstrate that the cerebellum may be a potent TMS site for improving cognitive function, whereas previous studies lacked convincing evidence for cognition enhancement through TMS targets other than the DLPFC (55). Additionally, instead of generalizing stimulation higher than 1 Hz as high-frequency stimulation, we investigated the effect size of 5 Hz, 10 Hz, 20 Hz stimulation and proposed that moderate frequency (5 Hz and 10 Hz) rTMS may be more effective for general cognition than high-frequency (20 Hz) rTMS. Comprehensive comparisons conducted through four subgroup analyses are helpful in identifying the optimal stimulation protocols and appropriate patient characteristics. Moreover, our analysis included 21 TMS RCTs based on rigorous inclusion criteria, enriching existing studies and providing a higher level of evidence in this field. The absence of significant publication bias and consistent results in the sensitivity analysis further validate the reliability of our findings. In summary, our study demonstrates that the cerebellum is a potential novel TMS target for improving cognition function, in addition to the DLPFC, and suggests that 5 Hz and 10 Hz stimulation may be more effective than 20 Hz rTMS. Future studies should focus on identifying the optimal combination of TMS parameters, including the stimulation site and frequency, as well as tailoring TMS treatment protocols to individual patients.
However, it is important to acknowledge several limitations of this study. First, although 21 studies and 25 trials were included, the sample size was relatively small and uneven, highlighting the need for further RCTs to provide more robust evidence. Second, our meta-analysis focused only on global cognitive outcomes, whereas other domains, such as behavioral and psychological changes associated with cognitive impairment, require further research. Third, the inclusion of both MCI and AD patients, representing distinct clinical stages of cognitive impairment, along with the heterogeneity of the study subjects, was intended to increase the statistical reliability and broaden the general applicability. However, studies specifically focusing on patients with MCI or pure AD may yield less interference. Finally, certain findings were obtained from a single study with a small sample size, necessitating confirmation through large-scale clinical trials.
Conclusion
Our data suggested that stimulation of the left DLPFC or bilateral cerebellum, along with moderate frequency stimulation (5 Hz and 10 Hz), may yield more favorable outcomes than other TMS protocols in terms of improving global cognition. Additionally, and patients with mild-to-moderate AD appear to have better responses to TMS than those with severe AD. However, the additional benefits of combining CT or multiple site stimulation lack sufficient supportive evidence. The cognitive improvement effects of TMS persisted at the 4-week follow-up.
Data availability statement
The original contributions presented in the study are included in the article/Supplementary material, further inquiries can be directed to the corresponding author.
Author contributions
YY: writing - original draft, methodology, investigation, formal analysis, and visualization. MT: validation, writing - original draft, and investigation. TW: investigation and resources. XW: data curation. YW: investigation. JS: conceptualization, funding acquisition, resources, supervision, writing - reviewing, and editing.
Funding
This study was supported by Nanjing Medical Science and Technology Development Key Project (No. ZKX21034).
Conflict of interest
The authors declare that the research was conducted in the absence of any commercial or financial relationships that could be construed as a potential conflict of interest.
Publisher's note
All claims expressed in this article are solely those of the authors and do not necessarily represent those of their affiliated organizations, or those of the publisher, the editors and the reviewers. Any product that may be evaluated in this article, or claim that may be made by its manufacturer, is not guaranteed or endorsed by the publisher.
Supplementary material
The Supplementary Material for this article can be found online at: https://www.frontiersin.org/articles/10.3389/fneur.2023.1209205/full#supplementary-material
Supplementary Figure 1. Forest plot: the subgroup analysis of short-term effects of single site, bilateral site, and multiple site stimulation.
Supplementary Figure 2. Forest plot: the subgroup analysis of long-lasting effects of single site, bilateral site, and multiple site stimulation.
Supplementary Figure 3. Forest plot: the subgroup analysis of short-term effects of left DLPFC stimulation, bilateral DLPFC stimulation, and multiple sites including DLPFC stimulation.
Supplementary Figure 4. Forest plot: the subgroup analysis of long-lasting effects of left DLPFC stimulation, bilateral DLPFC stimulation, and multiple sites including DLPFC stimulation.
Supplementary Figure 5. Forest plot: the subgroup analysis of short-term effects of left DLPFC and bilateral cerebellum stimulation.
Supplementary Figure 6. Forest plot: the subgroup analysis of long-lasting effects of left DLPFC and bilateral cerebellum stimulation.
Supplementary Figure 7. Forest plot: the subgroup analysis of short-term effects on mild cognitive impairment patients with CT or without CT.
Supplementary Figure 8. Forest plot: the subgroup analysis of long-lasting effects on mild cognitive impairment patients with CT or without CT.
Supplementary Figure 9. Forest plot: the subgroup analysis of the stimulation sites in the short-term effects.
Supplementary Figure 10. Forest plot: the subgroup analysis of the stimulation sites in the long-lasting effects.
Supplementary Figure 11. Forest plot: the subgroup analysis of the TMS frequency in the short-term effects.
Supplementary Figure 12. Forest plot: the subgroup analysis of the TMS frequency in the long-lasting effects.
Supplementary Figure 13. Forest plot: the subgroup analysis of the with/without CT in the short-term effects.
Supplementary Figure 14. Forest plot: the subgroup analysis of the with/without CT in the long-lasting effects.
Supplementary Figure 15. Forest plot: the subgroup analysis of patient characteristics in the short-term effects (Above) and in the long-lasting effects (Below).
References
1. Alzheimer's Association. 2016 Alzheimer's disease facts and figures. Alzheimer's Dementia. (2016) 12:459–509. doi: 10.1016/j.jalz.2016.03.001
2. WHO. Global Action Plan on the Public Health Response to Dementia 2017–2025. (2017). Available online at: https://www.who.int/publications/i/item/9789241513487 (accessed July 23, 2022).
3. Livingston G, Sommerlad A, Orgeta V, Costafreda SG, Huntley J, Ames D, et al. Dementia prevention, intervention, and care. Lancet. (2017) 390:2673–734. doi: 10.1016/S0140-6736(17)31363-6
4. Kim CK, Lee YR, Ong L, Gold M, Kalali A, Sarkar J. Alzheimer's disease: key insights from two decades of clinical trial failures. J Alzheimer's Dis. (2022) 87:83–100. doi: 10.3233/JAD-215699
5. Polanía R, Nitsche MA, Ruff CC. Studying and modifying brain function with non-invasive brain stimulation. Nat Neurosci. (2018) 21:174–87. doi: 10.1038/s41593-017-0054-4
6. Valero-Cabré A, Amengual JL, Stengel C, Pascual-Leone A, Coubard OA. Transcranial magnetic stimulation in basic and clinical neuroscience: a comprehensive review of fundamental principles and novel insights. Neurosci Biobehav Rev. (2017) 83:381–404. doi: 10.1016/j.neubiorev.2017.10.006
7. Ni Z, Chen R. Transcranial magnetic stimulation to understand pathophysiology and as potential treatment for neurodegenerative diseases. Translat Neurodegen. (2015) 3:1–2. doi: 10.1186/s40035-015-0045-x
8. Rossi S, Antal A, Bestmann S, Bikson M, Brewer C, Brockmöller J, et al. Safety and recommendations for TMS use in healthy subjects and patient populations, with updates on training, ethical and regulatory issues: expert Guidelines. Clinical Neurophysiol. (2021) 132:269–306. doi: 10.1016/j.clinph.2020.10.003
9. Chen RM, Classen J, Gerloff C, Celnik P, Wassermann EM, Hallett M, et al. Depression of motor cortex excitability by low-frequency transcranial magnetic stimulation. Neurology. (1997) 48:1398–403. doi: 10.1212/WNL.48.5.1398
10. George MS, Aston-Jones G. Noninvasive techniques for probing neurocircuitry and treating illness: vagus nerve stimulation (VNS), transcranial magnetic stimulation (TMS) and transcranial direct current stimulation (tDCS). Neuropsychopharmacology. (2010) 35:301–16. doi: 10.1038/npp.2009.87
11. Brunoni AR, Sampaio-Junior B, Moffa AH, Aparício LV, Gordon P, et al. Noninvasive brain stimulation in psychiatric disorders: a primer. Braz J Psychiatry. (2018) 41:70–81. doi: 10.1590/1516-4446-2017-0018
12. Chu CS Li CT, Brunoni AR, Yang FC, Tseng PT, Tu YK, et al. Cognitive effects and acceptability of non-invasive brain stimulation on Alzheimer's disease and mild cognitive impairment: a component network meta-analysis. JNeurol Neurosur Psychiatry. (2021) 92:195–203. doi: 10.1136/jnnp-2020-323870
13. Teselink J, Bawa KK, Koo GK, Sankhe K, Liu CS, Rapoport M, et al. Efficacy of non-invasive brain stimulation on global cognition and neuropsychiatric symptoms in Alzheimer's disease and mild cognitive impairment: a meta-analysis and systematic review. Ageing Res Rev. (2021) 72:101499. doi: 10.1016/j.arr.2021.101499
14. Wang X, Mao Z, Yu X. The role of noninvasive brain stimulation for behavioral and psychological symptoms of dementia: a systematic review and meta-analysis. Neurol Sci. (2020) 41:1063–74. doi: 10.1007/s10072-020-04245-4
15. Wang X, Mao Z, Ling Z, Yu X. Repetitive transcranial magnetic stimulation for cognitive impairment in Alzheimer's disease: a meta-analysis of randomized controlled trials. J Neurol. (2020) 267:791–801. doi: 10.1007/s00415-019-09644-y
16. Lin Y, Jiang WJ, Shan PY, Lu M, Wang T, Li RH, et al. The role of repetitive transcranial magnetic stimulation (rTMS) in the treatment of cognitive impairment in patients with Alzheimer's disease: a systematic review and meta-analysis. J Neurol Sci. (2019) 398:184–91. doi: 10.1016/j.jns.2019.01.038
17. Jiang L, Cui H, Zhang C, Cao X, Gu N, Zhu Y, et al. Repetitive transcranial magnetic stimulation for improving cognitive function in patients with mild cognitive impairment: a systematic review. Front Aging Neurosci. (2021) 12:593000. doi: 10.3389/fnagi.2020.593000
18. Hsu WY, Ku Y, Zanto TP, Gazzaley A. Effects of noninvasive brain stimulation on cognitive function in healthy aging and Alzheimer's disease: a systematic review and meta-analysis. Neurobiol Aging. (2015) 36:2348–59. doi: 10.1016/j.neurobiolaging.2015.04.016
19. Menardi A, Dotti L, Ambrosini E, Vallesi A. Transcranial magnetic stimulation treatment in Alzheimer's disease: a meta-analysis of its efficacy as a function of protocol characteristics and degree of personalization. J Neurol. (2022) 269:5283–301. doi: 10.1007/s00415-022-11236-2
20. Zhang T, Sui Y, Lu Q, Xu X, Zhu Y, Dai W, et al. Effects of rTMS treatment on global cognitive function in Alzheimer's disease: A systematic review and meta-analysis. Front Aging Neurosci. (2022) 14:984708. doi: 10.3389/fnagi.2022.984708
21. Chou YH, That VT, Sundman M A. systematic review and meta-analysis of rTMS effects on cognitive enhancement in mild cognitive impairment and Alzheimer's disease. Neurobiol Aging. (2020) 86:1–0. doi: 10.1016/j.neurobiolaging.2019.08.020
22. Yao Q, Tang F, Wang Y, Yan Y, Dong L, Wang T, et al. Effect of cerebellum stimulation on cognitive recovery in patients with Alzheimer disease: a randomized clinical trial. Brain Stimul. (2022) 15:910–20. doi: 10.1016/j.brs.2022.06.004
23. Cochrane Handbook for Systematic Reviews of Interventions Version 5.1.0. (2011) Available online at: www.handbook.cochrane.org (accessed August 1, 2022).
24. Higgins JP, Altman DG, Gøtzsche PC, Jüni P, Moher D, Oxman AD, et al. The Cochrane Collaboration's tool for assessing risk of bias in randomised trials. Bmj. (2011) 343. doi: 10.1136/bmj.d5928
25. Hedges LV. Distribution theory for Glass's estimator of effect size and related estimators. J Edu Stat. (1981) 6:107–28. doi: 10.3102/10769986006002107
26. DerSimonian R, Laird N. Meta-analysis in clinical trials. Control Clin Trials. (1986) 7:177–88. doi: 10.1016/0197-2456(86)90046-2
27. Zhao J, Li Z, Cong Y, Zhang J, Tan M, Zhang H, et al. Repetitive transcranial magnetic stimulation improves cognitive function of Alzheimer's disease patients. Oncotarget. (2017) 8:33864. doi: 10.18632/oncotarget.13060
28. Ahmed MA, Darwish ES, Khedr EM, El Serogy YM, Ali AM. Effects of low versus high frequencies of repetitive transcranial magnetic stimulation on cognitive function and cortical excitability in Alzheimer's dementia. J Neurol. (2012) 259:83–92. doi: 10.1007/s00415-011-6128-4
29. Sabbagh M, Sadowsky C, Tousi B, Agronin ME, Alva G, Armon C, et al. Effects of a combined transcranial magnetic stimulation (TMS) and cognitive training intervention in patients with Alzheimer's disease. Alzheimer's Dementia. (2019) 24:3. doi: 10.1016/j.jalz.2019.08.197
30. Li X, Qi G, Yu C, Lian G, Zheng H, Wu S, et al. Cortical plasticity is correlated with cognitive improvement in Alzheimer's disease patients after rTMS treatment. Brain Stimulation. (2021) 14:503–10. doi: 10.1016/j.brs.2021.01.012
31. Bagattini C, Zanni M, Barocco F, Caffarra P, Brignani D, Miniussi C, et al. Enhancing cognitive training effects in Alzheimer's disease: rTMS as an add-on treatment. Brain Stimul. (2020) 13:1655–64. doi: 10.1016/j.brs.2020.09.010
32. Padala PR, Boozer EM, Lensing SY, Parkes CM, Hunter CR, Dennis RA, et al. Neuromodulation for apathy in Alzheimer's disease: a double-blind, randomized, sham-controlled pilot study. Journal of Alzheimer's Dis. (2020) 77:1483–93. doi: 10.3233/JAD-200640
33. Drumond Marra HL, Myczkowski ML, Maia Memória C, Arnaut D, Leite Ribeiro P, Sardinha Mansur CG, et al. Transcranial magnetic stimulation to address mild cognitive impairment in the elderly: a randomized controlled study. Behav Neurol. (2015) 22:15. doi: 10.1155/2015/287843
34. Zhang F, Qin Y, Xie L, Zheng C, Huang X, Zhang M. High-frequency repetitive transcranial magnetic stimulation combined with cognitive training improves cognitive function and cortical metabolic ratios in Alzheimer's disease. J Neural Transm. (2019) 126:1081–94. doi: 10.1007/s00702-019-02022-y
35. Wu X, Ji GJ, Geng Z, Wang L, Yan Y, Wu Y, et al. Accelerated intermittent theta-burst stimulation broadly ameliorates symptoms and cognition in Alzheimer's disease: A randomized controlled trial. Brain Stimulation. (2022) 15:35–45. doi: 10.1016/j.brs.2021.11.007
36. Vecchio F, Quaranta D, Miraglia F, Pappalettera C, Di Iorio R., L'Abbate F, et al. Neuronavigated magnetic stimulation combined with cognitive training for Alzheimer's patients: an EEG graph study. Geroscience. (2022) 1:1–4. doi: 10.1007/s11357-021-00508-w
37. Jia Y, Xu L, Yang K, Zhang Y, Lv X, Zhu Z, et al. Precision repetitive transcranial magnetic stimulation over the left parietal cortex improves memory in Alzheimer's disease: a randomized, double-blind, sham-controlled study. Front Aging Neurosci. (2021) 13:693611. doi: 10.3389/fnagi.2021.693611
38. Yuan LQ, Zeng Q, Wang D, Wen XY, Shi Y, Zhu F, et al. Neuroimaging mechanisms of high-frequency repetitive transcranial magnetic stimulation for treatment of amnestic mild cognitive impairment: a double-blind randomized sham-controlled trial. Neural Regeneration Research. (2021) 16:707. doi: 10.4103/1673-5374.295345
39. Brem AK, Di Iorio R, Fried PJ, Oliveira-Maia AJ, Marra C, Profice P, et al. Corticomotor plasticity predicts clinical efficacy of combined neuromodulation and cognitive training in Alzheimer's disease. Front Aging Neurosci. (2020) 12:200. doi: 10.3389/fnagi.2020.00200
40. Cotelli M, Calabria M, Manenti R, Rosini S, Zanetti O, Cappa SF, et al. Improved language performance in Alzheimer disease following brain stimulation. J Neurol Neurosurg Psychiatry. (2011) 82:794–7. doi: 10.1136/jnnp.2009.197848
41. Rabey JM, Dobronevsky E. Repetitive transcranial magnetic stimulation (rTMS) combined with cognitive training is a safe and effective modality for the treatment of Alzheimer's disease: clinical experience. J Neural Transm. (2016) 123:1449–55. doi: 10.1007/s00702-016-1606-6
42. Gy RR, Reyes-López JV, Garcell R, AR CM NA AG. Effect of transcranial magnetic stimulation as an enhancer of cognitive stimulation sessions on mild cognitive impairment: preliminary results. Psychiatry Res. (2021) 304:114151. doi: 10.1016/j.psychres.2021.114151
43. Padala PR, Padala KP, Lensing SY, Jackson AN, Hunter CR, Parkes CM, et al. Repetitive transcranial magnetic stimulation for apathy in mild cognitive impairment: a double-blind, randomized, sham-controlled, cross-over pilot study. Psychiatry Res. (2018) 261:312–8. doi: 10.1016/j.psychres.2017.12.063
44. Koch G, Bonnì S, Pellicciari MC, Casula EP, Mancini M, Esposito R, et al. Transcranial magnetic stimulation of the precuneus enhances memory and neural activity in prodromal Alzheimer's disease. Neuroimage. (2018) 169:302–11. doi: 10.1016/j.neuroimage.2017.12.048
45. Wei L, Zhang Y, Wang J, Xu L, Yang K, Lv X, et al. Parietal-hippocampal rTMS improves cognitive function in Alzheimer's disease and increases dynamic functional connectivity of default mode network. Psychiatry Research. (2022) 315:114721. doi: 10.1016/j.psychres.2022.114721
46. Koch G, Casula EP, Bonnì S, Borghi I, Assogna M, Minei M, et al. Precuneus magnetic stimulation for Alzheimer's disease: a randomized, sham-controlled trial. Brain. (2022) 145:3776–86. doi: 10.1093/brain/awac285
47. Zhou X, Wang Y, Lv S, Li Y, Jia S, Niu X, et al. Transcranial magnetic stimulation for sleep disorders in Alzheimer's disease: a double-blind, randomized, and sham-controlled pilot study. Neurosci Lett. (2022) 766:136337. doi: 10.1016/j.neulet.2021.136337
48. Zhang X, Lan X, Chen C, Ren H, Guo Y. Effects of repetitive transcranial magnetic stimulation in patients with mild cognitive impairment: a meta-analysis of randomized controlled trials. Front Hum Neurosci. (2021) 15:723715. doi: 10.3389/fnhum.2021.723715
49. Cheng CP, Wong CS, Lee KK, Chan AP, Yeung JW, Chan WC. Effects of repetitive transcranial magnetic stimulation on improvement of cognition in elderly patients with cognitive impairment: a systematic review and meta-analysis. Int J Geriatr Psychiatry. (2018) 33:e1–3. doi: 10.1002/gps.4726
50. Liao X, Li G, Wang A, Liu T, Feng S, Guo Z, et al. Repetitive transcranial magnetic stimulation as an alternative therapy for cognitive impairment in Alzheimer's disease: a meta-analysis. J Alzheimer's Dis. (2015) 48:463–72. doi: 10.3233/JAD-150346
51. Miniussi C, Ruzzoli M, Walsh V. The mechanism of transcranial magnetic stimulation in cognition. cortex. (2010) 2010:128–30. doi: 10.1016/j.cortex.2009.03.004
52. Dong X, Yan L, Huang L, Guan X, Dong C, Tao H, et al. Repetitive transcranial magnetic stimulation for the treatment of Alzheimer's disease: a systematic review and meta-analysis of randomized controlled trials. PLoS ONE. (2018) 13:e0205704. doi: 10.1371/journal.pone.0205704
53. Epstein CM, Lah JJ, Meador K, Weissman JD, Gaitan LE, Dihenia B. Optimum stimulus parameters for lateralized suppression of speech with magnetic brain stimulation. Neurology. (1996) 47:1590–3. doi: 10.1212/WNL.47.6.1590
54. Pascual-Leone A, Gates JR, Dhuna A. Induction of speech arrest and counting errors with rapid-rate transcranial magnetic stimulation. Neurology. (1991) 41:697–702. doi: 10.1212/WNL.41.5.697
Keywords: transcranial magnetic stimulation, Alzheimer's disease, mild cognitive impairment, cognition, meta-analysis, cerebellum
Citation: Yan Y, Tian M, Wang T, Wang X, Wang Y and Shi J (2023) Transcranial magnetic stimulation effects on cognitive enhancement in mild cognitive impairment and Alzheimer's disease: a systematic review and meta-analysis. Front. Neurol. 14:1209205. doi: 10.3389/fneur.2023.1209205
Received: 20 April 2023; Accepted: 28 June 2023;
Published: 17 July 2023.
Edited by:
Pengxu Wei, National Research Center for Rehabilitation Technical Aids, ChinaReviewed by:
Grigorios Nasios, University of Ioannina, GreeceElias Paolo Casula, University of Rome Tor Vergata, Italy
Copyright © 2023 Yan, Tian, Wang, Wang, Wang and Shi. This is an open-access article distributed under the terms of the Creative Commons Attribution License (CC BY). The use, distribution or reproduction in other forums is permitted, provided the original author(s) and the copyright owner(s) are credited and that the original publication in this journal is cited, in accordance with accepted academic practice. No use, distribution or reproduction is permitted which does not comply with these terms.
*Correspondence: Jingping Shi, cHJvZnNoaWpwQDE2My5jb20=