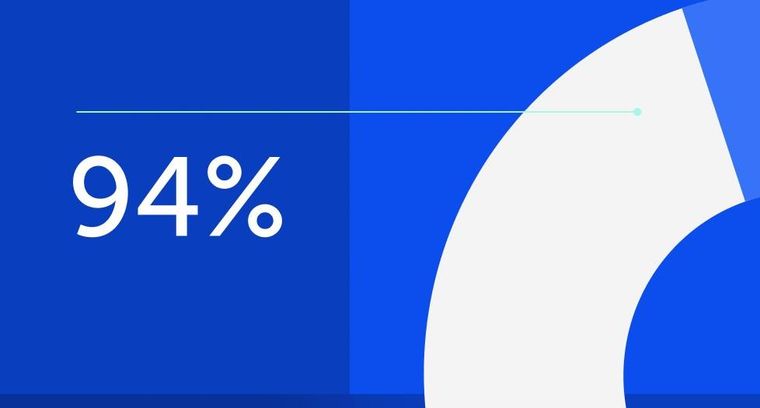
94% of researchers rate our articles as excellent or good
Learn more about the work of our research integrity team to safeguard the quality of each article we publish.
Find out more
REVIEW article
Front. Neurol., 30 June 2023
Sec. Multiple Sclerosis and Neuroimmunology
Volume 14 - 2023 | https://doi.org/10.3389/fneur.2023.1207626
This article is part of the Research TopicAging in multiple sclerosis: from childhood to old age, in women and menView all 13 articles
Multiple Sclerosis (MS) is a complex neurological disorder that involves demyelination, lesions and atrophy in both white and gray matter. Such changes in the central nervous system are diagnostic in MS and has a strong relationship with both physical and cognitive symptoms. As a result, magnetic resonance imaging (MRI) scans as a metric of brain atrophy have emerged as an important outcome measure in MS studies. Recently, research has begun to focus on the contribution of aging to the structural changes in the brain associated with MS; prompting questions about whether there is an amplifying effect of aging superimposed on MS-related brain atrophy. To examine current evidence of how the brain ages in individuals with MS, a systematic review of the literature was performed. Specific questions were focused on how aging affects gray and white matter structure, whether patterns of brain atrophy differ in younger and older cohorts and if there are structural differences in the brain as a function of sex in aging people with MS. This review considered studies that used MRI to examine the effects of aging in adults with MS. Twenty-one studies met eligibility criteria. Findings across these studies revealed that gray matter atrophy was more pronounced in older adults with MS, particularly in subcortical regions such as the thalamus; that the rates of atrophy were similar but varied by region for younger and older cohorts; and that males may experience more brain atrophy than females. Further studies that use multimodal MRI acquisition methods are needed to capture changes in both males and females over time, particularly in middle to older adulthood.
Globally, the population is aging at an unprecedented rate (1). Given the shifts in population demographics, it is essential to better understand the neurobiological aging process in both healthy conditions and in people with neurological disorders. Recent research has used magnetic resonance imaging (MRI), a non-invasive and easily repeatable technique, to depict normative trajectories of aging in gray and white matter. Specifically, Bethlehem et al. (2) used MRI data from more than 100,000 brain scans to create brain charts revealing patterns of gray and white matter atrophy in both males and females throughout middle and late adulthood (age 40 years onwards). These findings were largely in line with previous research indicating that after the age of 40 years, overall brain volume begins to decline by a rate of 5% per decade, with increased rates of decline at 70 years onwards (3–6). These are expected changes in the brain with normative aging. Although a better understanding of the neurobiological underpinnings of aging is being developed, relatively less is known about how the brain changes with age in people with chronic neurological conditions, such as multiple sclerosis.
Multiple Sclerosis (MS) is an inflammatory demyelinating disorder which predominantly affects the central nervous system (CNS). Approximately 90% of MS cases initially present as relapsing–remitting (RRMS), with acute inflammation and demyelinating lesions associated with relapsing symptoms (7). Over time, the majority of people with MS (pwMS) develop secondary progressive symptoms (SPMS), and although less common, some individuals present with primary progressive symptoms from onset (PPMS). Notably, MS tends to affect women more than men at an approximate 3:1 ratio, although, men who develop MS tend to move toward progressive stages of the disease faster (8). Regardless of the subtype, MS is characterized by chronic and cumulative changes in the brain that are evident on magnetic resonance imaging (MRI) and represent a key diagnostic criterion. Specifically, diagnoses are based on MRI-detected lesions (which tend to occur in periventricular regions) disseminated in time and space, in conjunction with characteristic symptoms. From a research perspective, common MRI findings for pwMS include decreased whole brain volume, enlarged ventricles, and a measurable white matter lesion load, as well as a decreased white matter integrity on diffusion tensor imaging (DTI) (9). MRI metrics have emerged as important outcome measures in MS studies, given that brain atrophy and white matter lesions are strong predictors of motor impairment and cognitive dysfunction (10). Unlike many other neurological disorders, MS is typically diagnosed in young to middle adulthood. Yet, most pwMS live into older adulthood, with life expectancy at approximately 75 years (11). Along with the population as a whole, the proportion of older adults with MS is growing and many older adults with MS did not have access to disease modifying treatments at the time of their diagnosis (12).
Age is an important factor when considering the disease course of MS, especially given that pwMS over the age of 65 are more commonly in the progressive form of the disease (13). Reduced remyelinating capacity is observed independently in aging populations and in pwMS. It has been shown in animal models of MS that as age increases, oligodendrocyte precursor cells, the primary cells responsible for remyelination, have reduced capacity for differentiation into remyelinating oligodendrocytes (14). Further, iron accumulation and deposition are hallmarks of not only normal aging, but older pwMS tend to show exacerbated iron toxicity that can cause increased neurodegeneration (15). Additionally, it has been suggested that inflammation related to previous viral infections (e.g., Epstein–Barr virus) can result in decreased immune system function and reduced capacity for tissue repair (12, 16).
Essentially, emerging research has begun to focus on the contribution of normal aging to the structural changes in the brain associated with MS; prompting questions about whether there is an amplifying effect of aging superimposed on MS-related neurodegeneration. To examine current evidence, a systematic review of the literature that used MRI to examine aging in pwMS was performed. This is the first study to synthesize the literature on the effects of aging on brain structure in pwMS. The following questions were specifically examined and will be discussed in this review:
1. How does brain structure (e.g., volume, white matter microstructure) differ or change with age in pwMS?
2. Are there specific structural MRI findings in older pwMS compared to younger pwMS?
3. Are there structural differences in the brain as a function of sex in aging pwMS?
It was hypothesized that there would be differences in brain structure that were specific to pwMS beyond the changes associated with typical aging and that older pwMS would have greater whole brain atrophy than younger pwMS. Sex differences were examined because of the known differences in prevalence and progression of MS between females and males, although this question was purely exploratory.
Specific eligibility criteria included studies that were peer-reviewed, in English, focused on adult (>18 years of age) human subjects and included an MS group (any subtype: RRMS, SPMS, PPMS) with full-text availability. Given the research questions of interest, studies must have also used MRI to examine brain structure. Any acquisition sequence was acceptable (e.g., T1-weighted, T2-weighted, diffusion weighted, FLAIR) because each provides different information about the brain. For example, T1-weighted scans can be used to examine gray matter volume, and diffusion-weighted scans can be used to examine white matter microstructure. Exclusion criteria included interventional studies and any studies focused on participants with other neurodegenerative conditions with comorbid MS. This review was registered on PROSPERO (CRD42021287667).
The literature search was conducted using two databases, PubMed and PsycINFO. Several preliminary search strategies were tested to determine the strategy that would be inclusive yet precisely able to identify papers of interest. The search strategy used a combination of terms relevant to the research questions and agreed upon by the authors (see Supplementary material for search terms in full). In summary, search terms included variables of interest including “aging,” “multiple sclerosis,” “white matter” “gray matter,” and “MRI.” Different variations of search terms were used to account for differing spellings and key words across articles. The reference sections of several articles were also screened to ensure that the search strategy was sufficient.
Titles and abstracts of search results were extracted and uploaded into systematic review management software, Covidence. Duplicates were removed. An initial screening of the titles and abstracts was conducted based on the eligibility criteria stated previously to exclude ineligible articles. The suitability of each article based on title and abstract was reviewed by two randomly selected authors (two of NT, IT, CB, JG) such that no more than one quarter of the articles were reviewed by the same two reviewers. Where disagreements occurred regarding eligibility of an article, a randomly selected third author would break the tie and determine the final eligibility of the article. Full-text articles were then uploaded and a second screening was conducted by two authors based on the entire research article using the same eligibility criteria. As per the initial screening, a randomly chosen third author resolved any conflicts.
To assess the quality of the articles included in this systematic review, the well-established critical appraisal tool for cross-sectional studies (AXIS) was used to rate each study. The AXIS tool uses a set of 20 questions that evaluate study design quality and quality of reporting. Studies were randomly assigned to authors, with each study assessed by a single author (one of NT, IT, CB, JG). Each study was assessed and interpreted for each of the 20 questions to evaluate its overall quality. To maintain consistency, both cross-sectional and longitudinal studies were assessed using the AXIS tool to rate the quality.
Studies were assigned to authors randomly (one of NT, IT, CB, JG) to extract relevant data. For each study, the information extracted included sample size, MS group subtypes at baseline, mean age at baseline, sex ratio, geography of study participants, study type (cross-sectional or longitudinal), acquisition/analysis measurement methods used in the study, and summarized results relevant to the research questions identified for this review.
The search for studies was conducted on September 22, 2021. Initial search results yielded 318 studies. Covidence software automatically removed 133 duplicate studies based on titles. A total of 185 articles remained and their titles and abstracts were manually reviewed and screened further for inclusion and exclusion criteria, after which 144 irrelevant articles were excluded. Manual retrieval of full-text articles was conducted for the remaining 41 studies. These 41 full-text articles were then reviewed in-depth for inclusion. Each article was screened by two authors and any discrepancies for inclusion were resolved by a third author who was not involved in the initial voting for that article. Exclusions primarily resulted when studies did not have appropriate outcomes for this review (e.g., interventional studies, studies in which participants had concurrent neurodegenerative disorders, etc.). Supplementary Figure S1 details the papers that underwent full-text review but were ultimately excluded. The selection process is detailed in Figure 1 using a PRISMA flow diagram. A total of 21 studies published between 2003 and 2021 were included in the final review. Table 1 provides all the information extracted from the 21 full-text articles including sample size, MS groups, mean age, sex, geography, study type, acquisition/analysis, and summary of study results.
Figure 1. PRISMA flow diagram detailing the steps of literature collection for this systematic review (created in BioRender).
The AXIS tool (38) was used to assess study quality in both cross-sectional and longitudinal studies included in this review. Quality was assessed by 20 questions that allow for evaluation of any pitfalls that studies may have. Table 2 shows the breakdown of quality analysis across each of the 20 questions for the 21 included studies.
Table 2. AXIS tool responses to assess qualitative features of papers that were used in this review.
Overall, 21 studies were included in the final analyses. A description of each study, including sample size, MS group breakdown, mean age and sex of participants, study geography, study type and duration, type of scans acquired/analyzed, and a summary of study results, are included in Table 1. The findings are discussed further in the context of the research questions guiding this review in the discussion section below.
MS is a chronic neurological disorder that is characterized by lesions in the CNS and typically diagnosed in young to mid-adulthood. Relatively less research has focused on older adults with MS, although recent questions have emerged about whether there is an accelerated aging effect for pwMS. The current study involved a systematic review of the literature that used structural MRI methods to examine changes in the brain specific to aging with MS. This review considered cross sectional studies that examined differences between pwMS and healthy controls, as well as longitudinal studies tracking changes in the brain in pwMS over time with an aim to answer several specific questions. Herein, we pose each of the pre-determined questions along with conditional answers based on the extant literature.
With an aim to answer this overarching question, the search terms of the current review included both gray and white matter as well as specific imaging techniques, such as diffusion tensor imaging (DTI), which provides metrics of white matter microstructure. However, there were no studies that focused on white matter using DTI and although several studies examined total white matter volume, the vast majority of studies focused on gray matter volume using high resolution T1-weighted anatomical MRI scans.
Of the 21 identified studies, 13 compared pwMS and healthy controls and all of these revealed significant structural brain differences. Notably, some studies focused on broad metrics such as whole brain volume, while other studies extracted values representing gray and white matter volume or white matter lesion load. Several studies also extracted volumes for particular regions or carried out voxel-based morphometry analyses. Although there was variability in the regions examined across studies, 4 studies reported decreased whole brain volume in pwMS (27, 29–31, 39), 6 reported decreased gray matter volume (18, 24, 25, 29, 30, 36, 39), 6 reported decreased volume in subcortical gray matter structures (17, 18, 20, 24, 25, 29, 30) (particularly in the thalamus and basal ganglia) and 3 reported decreases in white matter based on volume or lesion load (19, 29, 30, 33). Notably, 6 of these studies examined how atrophy rates in healthy controls compared to pwMS. These studies suggested that pwMS showed accelerated rates of atrophy in the thalamus (17, 18) and other gray matter regions (24, 25, 39).
Three studies investigated predicted “brain age” using various algorithms and found that pwMS had significantly larger predicted brain age differences (higher biological age compared to chronological age) in comparison to healthy controls which also suggests accelerated aging in pwMS (22, 23, 28). Finally, a unique study from Krysko et al. (32) established that short leukocyte telomere length was associated with disability and brain atrophy (measured as total brain volume) in pwMS. The authors suggest that based on this finding, biological aging may contribute to disability worsening in MS (32).
Taken together, the studies included in this review provide evidence that supports acceleration of whole brain and gray matter atrophy as pwMS age when compared to normal aging in people without MS. Gray matter and subcortical regions including the thalamus appear particularly vulnerable to faster atrophy with age in pwMS as compared to healthy normal aging controls, although there were limited numbers of studies that focused on specific regions and on white matter which limits the conclusions that can be drawn from the literature.
Of the 21 studies reviewed, 6 used MRI to examine brain structure differences in younger and older pwMS using a mix of cross-sectional and longitudinal approaches (18, 20, 21, 34, 35, 37). Using a cross-sectional approach, Newbould et al. (35) compared younger (30.4 years) and older (49.7 years) pwMS and found strong magnetization transfer ratio (MTR) differences between the two age groups within white matter lesions. This suggests decreased myelin integrity in the older age group. Bishop et al. (20) also took a cross-sectional approach and compared both younger (30.4 years) and older (48.7 years) pwMS to age-matched healthy controls. They found similar patterns of atrophy for each age group with MS in the putamen, thalamus and nucleus accumbens, but significantly more atrophy in the hippocampus and caudate in the younger group. A retrospective, cross-sectional approach was used by Tortorella et al. (37) to examine gadolinium-enhancing lesions in MS populations. They found that enhanced lesions were more frequently found in younger patients with lower disability. They also showed that the change in risk for gadolinium-enhancing lesions occurs at 35 years of age in pwMS. The authors proposed that these lesions may contribute to disease mechanisms in younger pwMS, whereas there are likely age-related neurodegenerative mechanisms occurring in older pwMS. Bove et al. (21) used a mixed design with a longitudinal component to examine changes in slopes of MRI extracted metrics including total brain volume and white matter lesion load over 2 years in pwMS who were under (younger group) or over (older group) 50 years of age (42 years and 58 years, respectively). The authors found no significant differences in slope of change as a function of age for either metric. Martola et al. (34) also used longitudinal observations to examine changes in brain volume at three time points over a decade in pwMS (ranging in age from 24–65 years) and found uniform progression of atrophy over time. Lastly, an interesting study by Azevedo et al. (18) examined annual MRI scans from 520 participants with RRMS over 5 years and found that the contributions of normal aging and MS-related atrophy differed by brain region. Findings revealed that the contributions of aging became greater with each decade from 30 to 60 years of age, while the contribution of MS-related atrophy lessened. In contrast, the contributions of aging and MS-related atrophy were relatively more stable over time in the putamen and caudate. These findings underscore the need for more comprehensive regional analyses to better understand patterns of brain atrophy in aging pwMS.
The various approaches to study design and analyses create challenges and limitations in synthesizing the results to date. Although there is some evidence that aging may have differential effects on brain atrophy in pwMS across the lifespan as compared to normal aging, more research is needed. In particular, the use of regional approaches in older age groups (e.g., 60+ years) that have not been well captured require further examination.
Typically, MS affects 3 times more females than males, although disability progression occurs quicker in males (40). Of the 21 studies included, 2 examined aging as a function of sex in pwMS (21, 29, 30). Although Bove et al. (21) did not find differences in rate of whole brain atrophy as a function of age, their findings did reveal significantly greater disease severity (based on EDSS scores) and whole brain atrophy in males compared to females. Further, Jakimovski et al. (29, 30) highlighted similar findings with males demonstrating lower whole brain and gray matter volumes as well as increased ventricular volume compared to females. However, this paper also showed that after the age of 60, there were no evident differences in brain structure between males and females with progressive MS (29, 30).
As evidenced by our literature search, there are a limited number of studies examining sex differences in aging pwMS. However, the evidence to date suggests that males have greater brain atrophy than females with age. One potential factor that may be attributed to the differences seen between sexes is menopause as it has been suggested to affect brain atrophy patterns in aging females with MS (41). Another factor may relate to the course of MS in males, as males have been shown to have more rapid disability accumulation and cognitive decline than females (42). More research including longitudinal studies that capture changes from age 40 to 60 in both females and males are essential to understanding the potential effects of sex on age-related atrophy over time and whether such differences are also stable over time.
The current review represents the first synthesis of the literature examining brain aging in pwMS. It was hypothesized that there would be age-related differences in brain structure that were specific to pwMS and beyond the changes associated with typical aging, with older adults showing greater atrophy. Indeed, the literature revealed that cortical and subcortical gray matter are vulnerable to excess atrophy with age in pwMS. Predicted brain age was also consistently higher and atrophy rates were accelerated in pwMS compared to healthy controls. There were also indications that certain brain regions may become more prone to atrophy as pwMS age and that males may experience greater atrophy than females. However, more research is imperative to fully address each of the research questions posed in this review and there are several strengths and limitations within the current review, as well as within the literature that should be addressed.
With regards to the current review, strengths included the broad approach taken to search terms in combination with the focused questions on age related changes as measured by MRI in pwMS. The regional patterns and characteristics that influence brain atrophy for pwMS have important implications for clinical practice. In particular, understanding expected changes related to aging and whether there is accelerated neurodegeneration in MS can assist with interpretation of MRI findings for older adults with MS.
Conversely, a limitation was that studies examining correlations between structural brain changes with age and clinical variables were beyond the scope of the current review. Several studies that met the criteria for review happened to include comparisons of brain atrophy patterns and clinical outcomes (e.g., disease severity) with significant findings. Specifically examining such relationships between age related atrophy and symptoms of MS could form the topic of a separate focused systematic review.
Within the literature, the quality of the reviewed studies was consistently high. Assessment of study quality with the AXIS tool revealed that all of the studies in the current review clearly stated their objectives and designed their study appropriately, using proper outcome variables. The majority of studies were also clear on how significance was determined, and the conclusions made within each study were consistent with the presented results. The main limitations of the literature became apparent when synthesizing the findings. Specifically, there was heterogeneity in methods, including widely variable sample sizes (ranging from 19 to 2,199 pwMS), acquisition techniques (e.g., field strength, imaging sequences, parameters), analysis approaches (e.g., whole brain, gray matter volume, white matter lesion load, voxel-based morphometry, various brain age algorithms), and study designs (e.g., different age groups, study protocols) that yielded findings that are difficult to weigh and integrate across studies. As mentioned previously, there was a distinct lack of studies that examined aging pwMS using diffusion tensor imaging derived metrics of white matter microstructure. Given that changes in myelination and axonal integrity are characteristic of MS and that DTI is sensitive to both lesions and changes in normal appearing white matter, this technique could provide important information on the aging brain for pwMS. Additionally, in most of the included studies, the majority of participants had RRMS, although SPMS most commonly develops over the disease course of people with RRMS and should be better represented in studies on aging. Relatedly, people with different subtypes of MS may be more or less likely to be on disease modifying treatments (DMTs). Many, but not all, of the studies reviewed reported on DMTs, which was higher in people with RRMS than other subtypes. The differences and changes in brain volume detected in these studies were largely present in the context of DMT use. Although it is beyond the scope of the current review to determine whether DMT use has a modifying effect on neurodegeneration, ongoing research should report DMT use so that future reviews can examine whether any accelerated aging effects are muted by treatment (as current cohorts on these treatments age).
Importantly, previous research has shown that African Americans exhibit more rapid neurodegeneration than Caucasian Americans (43); however, most studies did not report on race or ethnicity and those that did report these characteristics had a majority of white participants. Moving forward, it will be important for research to be inclusive and involve transparent reporting.
In terms of other directions for future research, there is a clear need for large scale studies that use multimodal MRI acquisition methods to capture changes in both males and females over time, particularly in middle to older adulthood. Such studies would essentially aid the understanding of how the brain ages in pwMS, which will have implications for both the conceptualization of the disease course of MS and the interpretation of intervention related changes in the brain as pwMS age.
NT and IT drafted the initial manuscript. All authors were involved in conceptualizing the review, reviewing the literature, and critical revisions.
This project was supported by the endMS Scholar Program for Researchers IN Training (SPRINT) program by the Multiple Sclerosis Society of Canada.
The authors declare that the research was conducted in the absence of any commercial or financial relationships that could be construed as a potential conflict of interest.
All claims expressed in this article are solely those of the authors and do not necessarily represent those of their affiliated organizations, or those of the publisher, the editors and the reviewers. Any product that may be evaluated in this article, or claim that may be made by its manufacturer, is not guaranteed or endorsed by the publisher.
The Supplementary material for this article can be found online at: https://www.frontiersin.org/articles/10.3389/fneur.2023.1207626/full#supplementary-material
1. World Health Organization. (2023). Aging and health. Available at: https://www.who.int/news-room/facts-in-pictures/detail/aging.
2. Bethlehem, RAI, Seidlitz, J, White, SR, Vogel, JW, Anderson, KM, Adamson, C, et al. Brain charts for the human lifespan. Nature. (2022) 604:525–33. doi: 10.1038/s41586-022-04554-y
3. Liu, H, Yang, Y, Xia, W, Zhu, W, Leak, RK, Wei, Z, et al. Aging of cerebral white matter. Aging Res Rev. (2017) 34:64–76. doi: 10.1016/j.arr.2016.11.006
4. Nyberg, L, Salami, A, Andersson, M, Eriksson, J, Kalpouzos, G, Kauppi, K, et al. Longitudinal evidence for diminished frontal cortex function in aging. Proc Natl Acad Sci U S A. (2010) 107:22682–6. doi: 10.1073/pnas.1012651108
5. Scahill, RI, Frost, C, Jenkins, R, Whitwell, JL, Rossor, MN, and Fox, NC. A longitudinal study of brain volume changes in normal aging using serial registered magnetic resonance imaging. Arch Neurol. (2003) 60:989–94. doi: 10.1001/archneur.60.7.989
6. Svennerholm, L, Boström, K, and Jungbjer, B. Changes in weight and compositions of major membrane components of human brain during the span of adult human life of swedes. Acta Neuropathol. (1997) 94:345–52. doi: 10.1007/s004010050717
7. Browne, P, Chandraratna, D, Angood, C, Tremlett, H, Baker, C, Taylor, BV, et al. Atlas of multiple sclerosis 2013: a growing global problem with widespread inequity. J Neurol. (2014) 83:1022–4. doi: 10.1212/WNL.0000000000000768
8. Dunn, SE, Gunde, E, and Lee, H. Sex-Based Differences in Multiple Sclerosis (MS): Part II: Rising Incidence of Multiple Sclerosis in Women and the Vulnerability of Men to Progression of this Disease. In: A La Flamme and J Orian, editors. Emerging and Evolving Topics in Multiple Sclerosis Pathogenesis and Treatments. Current Topics in Behavioral Neurosciences Vol 26. Cham: Springer. (2015). 57–86.
9. Hemond, CC, and Bakshi, R. Magnetic resonance imaging in multiple sclerosis. Cold Spring Harb Perspect Med. (2018) 8:a028969. doi: 10.1101/cshperspect.a028969
10. DeLuca, GC, Yates, RL, Beale, H, and Morrow, SA. Cognitive impairment in multiple sclerosis: clinical, radiologic and pathologic insights. Brain Pathol. (2014) 25:79–98. doi: 10.1111/bpa.12220
11. Lunde, HMB, Assmus, J, Myhr, K, Bø, L, and Grytten, N. Survival and cause of death in multiple sclerosis: a 60-year longitudinal population study. J Neurol Neurosurg Psychiatry. (2017) 88:621–5. doi: 10.1136/jnnp-2016-315238
12. Balusha, AAK, and Morrow, SA. Multiple sclerosis in people over age 55. Pract Neurol. (2021) 41:41–43.
13. Sanai, SA, Saini, V, Benedict, RHB, Zivadinov, R, Teter, BE, Ramanathan, M, et al. Aging and multiple sclerosis. Mult Scler J. (2016) 22:717–25. doi: 10.1177/1352458516634871
14. Neumann, B, Segel, M, Chalut, KJ, and Franklin, RJM. Remyelination and aging: reversing the ravages of time. Mult Scler J. (2019) 25:1835–41. doi: 10.1177/1352458519884006
15. Stankiewicz, JM, Neema, M, and Ceccarelli, A. Iron and multiple sclerosis. Neurobiol Aging. (2014) 35:S51–8. doi: 10.1016/j.neurobiolaging.2014.03.039
16. Bjornevik, K, Cortese, M, Healy, BC, Kuhle, J, Mina, MJ, Leng, Y, et al. Longitudinal analysis reveals high prevalence of Epstein-Barr virus associated with multiple sclerosis. Science. (2022) 375:296–301. doi: 10.1126/science.abj8222
17. Azevedo, CJ, Cen, SY, Khadka, S, Liu, S, Kornak, J, Shi, Y, et al. Thalamic atrophy in multiple sclerosis: a magnetic resonance imaging marker of neurodegeneration throughout disease. Ann Neurol. (2018) 83:223–34. doi: 10.1002/ana.25150
18. Azevedo, CJ, Cen, SY, Jaberzadeh, A, Zheng, L, Hauser, SL, and Pelletier, D. Contribution of normal aging to brain atrophy in MS. Neurol Neuroimmunol Neuroinflamm. (2019) 6:e617. doi: 10.1212/NXI.0000000000000616
19. Baird, JF, and Motl, RW. Cognitive function and whole-brain MRI metrics are not associated with mobility in older adults with multiple sclerosis. Int J Environ Res Public Health. (2021) 18:4232. doi: 10.3390/ijerph18084232
20. Bishop, CA, Newbould, RD, Lee, JSZ, Honeyfield, L, Quest, R, Colasanti, A, et al. Analysis of aging-associated grey matter volume in patients with multiple sclerosis shows excess atrophy in subcortical regions. Neuroimage Clin. (2017) 13:9–15. doi: 10.1016/j.nicl.2016.11.005
21. Bove, R, Musallam, A, Healy, BC, Houtchens, M, Glanz, BI, Khoury, S, et al. No sex-specific difference in disease trajectory in multiple sclerosis patients before and after age 50. BMC Neurol. (2013) 13:73. doi: 10.1186/1471-2377-13-73
22. Cole, JH, Raffel, J, Friede, T, Eshanghi, A, Brownlee, WJ, Chard, D, et al. Longitudinal assessment of multiple sclerosis with the brain-age paradigm. Ann Neurol. (2020) 88:93–105. doi: 10.1002/ana.25746
23. Erramuzpe, A, Schurr, R, Yeatman, JD, Gotlib, IH, Sacchet, MD, Travis, KE, et al. A comparison of quantitative R1 and cortical thickness in identifying age, lifespan dynamics, and disease states of the human cortex. Cereb Cortex. (2021) 31:1211–26. doi: 10.1093/cercor/bhaa288
24. Eshaghi, A, Bodini, B, Ridgway, GR, García-Lorenzo, D, Tozer, DJ, Sahraian, MA, et al. Temporal and spatial evolution of grey matter atrophy in primary progressive multiple sclerosis. Neuroimage. (2014) 86:257–64. doi: 10.1016/j.neuroimage.2013.09.059
25. Eshaghi, A, Prados, F, Brownlee, WJ, Altmann, DR, Tur, C, Cardoso, MJ, et al. Deep grey matter volume loss drives disability worsening in multiple sclerosis. Ann Neurol. (2018) 83:210–22. doi: 10.1002/ana.25145
26. Fisher, E, Lee, J-C, Nakamura, K, and Rudick, RA. Gray matter atrophy in multiple sclerosis: A longitudinal study. Ann Neurol. (2008) 64:255–65. doi: 10.1002/ana.21436
27. Ghione, E, Bergsland, N, Dwyer, MG, Hagermeier, J, Jakimovski, D, Paunkoski, I, et al. Aging and brain atrophy in multiple sclerosis. Neuroimage. (2019) 29:527–35. doi: 10.1111/jon.12625
28. Høgestøl, EA, Kaufmann, T, Nygaard, GO, Beyer, MK, Sowa, P, Nordvik, JE, et al. Cross-sectional and longitudinal MRI brain scans reveal accelerated brain aging in multiple sclerosis. Front Neurol. (2019) 10:450. doi: 10.3389/fneur.2019.00450
29. Jakimovski, D, Bergsland, N, Dwyer, MG, Hagemeier, J, Ramasamy, DP, Szigeti, K, et al. Long-standing multiple sclerosis neurodegeneration: volumetric magnetic resonance imaging comparison to Parkinson’s disease, mild cognitive impairment, Alzheimer’s disease, and elderly healthy controls. Neurobiol Aging. (2020) 90:84–92. doi: 10.1016/j.neurobiolaging.2020.02.002
30. Jakimovski, D, Zivadinov, R, Bergsland, N, Ramasamy, DP, Hagemeier, J, Weinstock-Guttman, B, et al. Sex-specific differences in life span brain volumes in multiple sclerosis. Neuroimage. (2020) 30:342–50. doi: 10.1111/jon.12709
31. Kassubek, J, Tumani, H, Ecker, D, Kurt, A, Ludolph, AC, and Juengling, FD. Age-related brain parenchymal fraction is significantly decreased in young multiple sclerosis patients: a quantitative MRI study. Neuroreport. (2003) 14:427–30. doi: 10.1097/00001756-200303030-00026
32. Krysko, KM, Henry, RG, Cree, BAC, Lin, J, University of California, San Francisco MS EPIC Team, et al. Telomere length is associated with disability progression in multiple sclerosis. Ann Neurol. (2019) 86:671–82. doi: 10.1002/ana.25592
33. Kuusisto, H, Wu, X, Dastidar, P, Luukkaala, T, and Elovaara, I. Volumetric MRI assessment of brain and spinal cord in Finnish twins discordant for multiple sclerosis. Medicina. (2012) 48:437–41.23168917. doi: 10.3390/medicina48090065
34. Martola, J, Bergström, J, Fredrikson, S, Stawiarz, L, Hillert, J, Zhang, Y, et al. A longitudinal observational study of brain atrophy rate reflecting four decades of multiple sclerosis: a comparison of serial 1D, 2D and volumetric measurements from MRI images. Neuroradiology. (2010) 52:109–17. doi: 10.1007/s00234-009-0593-9
35. Newbould, RD, Nicholas, R, Thomas, CL, Quest, R, Lee, JSZ, Honeyfield, L, et al. Age independently affects myelin integrity as detected by magnetization transfer magnetic resonance imaging in multiple sclerosis. Neuroimage Clin. (2014) 4:641–8. doi: 10.1016/j.nicl.2014.02.004
36. Tiberio, M, Chard, DT, Altmann, DR, Davies, G, Griffin, CM, Rashid, W, et al. Gray and white matter volume changes in early RRMS: a 2-year longitudinal study. Neurology. (2005) 64:1001–7. doi: 10.1212/01.WNL.0000154526.22878.30
37. Tortorella, C, Bellacosa, A, Paolicelli, D, Fuiani, A, Di Monte, E, Simone, IL, et al. Age-related gadolinium-enhancement of MRI brain lesions in multiple sclerosis. J Neurol Sci. (2005) 239:95–9. doi: 10.1016/j.jns.2005.08.006
38. Downes, MJ, Brennan, ML, Williams, HC, and Dean, RS. Development of a critical appraisal tool to assess the quality of cross-sectional studies (AXIS). BMJ Open. (2016) 6:e011458. doi: 10.1136/bmjopen-2016-011458
39. Fischer, E, Lee, J, Nakamura, K, and Rudick, RA. Gray matter atrophy in multiple sclerosis: a longitudinal study. Ann Neurol. (2008) 64:255–65. doi: 10.1002/ana.21436
40. Voskuhl, RR, Patel, K, Paul, F, Gold, SM, Scheel, M, Kuchling, J, et al. Sex differences in brain atrophy in multiple sclerosis. Biol Sex Differ. (2020) 11:49. doi: 10.1186/s13293-020-00326-3
41. Bove, R, Okai, A, Houtchens, M, Elias-Hamp, B, Lugaresi, A, Hellwig, K, et al. Effects of menopause in women with multiple sclerosis: an evidence-based review. Front Neurol. (2012) 12:554375. doi: 10.3389/fneur.2021.554375
42. Alvarez-Sanchez, N, and Dunn, SE. Potential biological contributers to the sex difference in multiple sclerosis progression. Front Immunol. (2023) 14:1175874. doi: 10.3389/fimmu.2023.1175874
Keywords: multiple sclerosis, aging, magnetic resonance imaging, systematic review, brain
Citation: Tokarska N, Tottenham I, Baaklini C and Gawryluk JR (2023) How does the brain age in individuals with multiple sclerosis? A systematic review. Front. Neurol. 14:1207626. doi: 10.3389/fneur.2023.1207626
Received: 17 April 2023; Accepted: 13 June 2023;
Published: 30 June 2023.
Edited by:
Kristen Marie Krysko, St Michael’s Hospital, CanadaReviewed by:
Omar Al-Louzi, Cedars Sinai Medical Center, United StatesCopyright © 2023 Tokarska, Tottenham, Baaklini and Gawryluk. This is an open-access article distributed under the terms of the Creative Commons Attribution License (CC BY). The use, distribution or reproduction in other forums is permitted, provided the original author(s) and the copyright owner(s) are credited and that the original publication in this journal is cited, in accordance with accepted academic practice. No use, distribution or reproduction is permitted which does not comply with these terms.
*Correspondence: Jodie R. Gawryluk, Z2F3cnlsdWtAdXZpYy5jYQ==
†These authors have contributed equally to this work
Disclaimer: All claims expressed in this article are solely those of the authors and do not necessarily represent those of their affiliated organizations, or those of the publisher, the editors and the reviewers. Any product that may be evaluated in this article or claim that may be made by its manufacturer is not guaranteed or endorsed by the publisher.
Research integrity at Frontiers
Learn more about the work of our research integrity team to safeguard the quality of each article we publish.