- 1Department of Rehabilitation, National Rehabilitation Center, Ewha Womans University, Seoul, Republic of Korea
- 2Department of Music Therapy, Graduate School, Ewha Womans University, Seoul, Republic of Korea
Attention training is the primary step in the rehabilitation for patients with acquired brain injury (ABI). While active music performance has been reported to aid neural and functional recovery, its efficacy for patients with ABI remains uncertain due to methodological concerns. The purpose of the study is to develop a virtual reality-based music attention training (VR-MAT), which utilizes a visually guided, bilateral drumming in an immersive environment to train attention and executive functions. We also aims to examine the feasibility and effectiveness of the VR-MAT with a small sample size of participants (3–60 months after ABI, N = 20 approximately). Participants will be randomly assigned to either a waitlist control or music group, in which VR-MAT will take place five times weekly over 4 weeks (randomized crossover design). The evaluation of VR-MAT performance will include accuracy and response time in music responses. Neurocognitive outcome measures will be administered to quantify pre-post changes in attention, working memory, and executive functions. Additionally, functional near-infrared spectroscopy will be employed to explore the relationships between musical behavior, neurocognitive function, and neurophysiological responses.
1. Introduction
Acquired brain injury (ABI) is the damage to the brain that occurs due to certain events after birth (1) and includes traumatic injury and/or stroke (2, 3). Many individuals with ABI experience temporary or permanent neurological sequelae which is not limited to psychomotor, cognitive, and communication difficulties (4–7). The prevalence of ABI-related changes in cognition, including attention, working memory, memory, and executive function, is high (8–12), leading to a lower quality of life, accelerated functional decline, and a higher risk of dependence and mortality (5, 7). Cognitive impairments can decrease therapeutic potential and clinical benefits, since verbal and non-verbal information given in therapeutic settings is hardly recognized. Attention impairment should be a priority in the rehabilitation agenda for patients with ABI because dysfunctions in the fundamental mechanism of attention are pervasively involved in cognitive impairment (13–19). More importantly, auditory attention appears to be more challenging in patients with ABI (20, 21). These patients often have difficulty attuning their attention to relevant auditory information and distinguishing target sounds from distracting sounds (20, 22–24).
Auditory information processing is challenging because it is both sequential and simultaneous. Listening to music can enhance attentional function, which is required to process complex auditory information. Music automatically activates the attention system, even when actively ignoring presented melodies and voluntarily attending to target melodies (25). In addition, music processing in different textures can lead to activation in neural regions that house different types of attention, such as selective attention and divided attention, depending on the manner of listening to music (i.e., holistic, selective, or divided) (26–28). Music error/change detection tasks can be utilized to investigate and train auditory attention (26, 29–33). More recently, active music performance and listening have been applied to cognitive rehabilitation. An initial study investigated the effects of a music attention training program on five adults with TBI (34), which was developed based on a hierarchy of attention (35). Furthermore, one study examined the effect of a music attention training program on alternating attention using a dichotic listening test (36), and another study suggested that music therapy is an effective early intervention for cognitive rehabilitation (37).
More recently, active music performances have been applied to various populations, such as juveniles, adolescents with neurodevelopmental disorders, and psychiatric patients. Abrahams and van Dooren (38) compared music attention control training (MACT) with non-standardized music therapy (NSMT) and treatment as usual (TAU) in terms of the attentional ability of juveniles (N = 6). With a six-week training of 45 min weekly, some participants in the MACT group showed an extensive increase in selective, sustained, and divided attention. Pasiali et al. (39) investigated the effect of the MACT on the attentional function of adolescents with neurodevelopmental delays using a single-group pre-and post-test design. With a six-week training of 45 min weekly, participants showed considerable improvements in selective attention and divided attention. van Alphen et al. (40) conducted a randomized controlled trial to determine the effect of MACT on attention skills in a group of adult psychiatric patients. After a 6 week training of 30 min weekly, single-blind pre-and post-intervention showed a marked enhancement in selective, sustained, and alternating attention in the MACT group. Jones (14) conducted a randomized controlled trial (RCT) to examine the effect of MACT compared to that of Attention Process Training (APT). The findings showed significant values and partial support from nonparametric testing, suggesting the potential of MACT in favor of APT.
Despite rigorous attempts, the efficacy of active music performance for patients with ABI is still under debate. For instance, in a systematic review of music and rehabilitation, Bradt et al. (41) reported that psychomotor rehabilitation effectively improves gait regulation, yet the success of cognitive rehabilitation remains uncertain. Similarly, Magee et al. (42) provided evidence supporting the positive influence of music interventions on upper and lower mobility functions as well as speech. Nonetheless, only two studies (43, 44) demonstrated the efficacy in music-based cognitive rehabilitation. Both reviews agreed on the urgent need for high-quality RCTs focusing on cognitive rehabilitation, along with a lack of replicable therapeutic protocols for music-based training designed to aid recovery from cognitive deficits (34, 36, 45). Most studies relied solely on functional outcome measures without quantifying musical responses, potentially missing key relationships between musical and functional behaviors, as well as changes uniquely evident through musical behaviors. To address the limitations, we developed a replicable therapeutic protocol of active music performance and integrated the protocol with a virtual reality system. The efficacy of VR-MAT will be evaluated through randomized cross-over trials, using a comprehensive data collection strategy that incorporates neuropsychological, neurophysiological and music responses measures.
2. Materials and methods
2.1. Study design
The present study will be conducted as an assessor-blinded, randomized, controlled trial with a crossover design at a rehabilitation hospital. Participants will be randomly allocated to either the treatment sequence: VR-MAT first and second with conventional cognitive training (CT) (VC group), or in the opposite order (CV group) with an allocation ratio of 1:1 using a computer-generated randomization table. The crossover design is selected because the participants need to serve as their own control group to minimize confounding effects on cognition, which might be markedly affected by a person’s traits, which is not intended by the intervention. In addition, it might be beneficial for the statistical efficiency of this pilot trial, in which VR-MAT had not been attempted for patients with ABI.
Each VR-MAT or CT training period will last 4 weeks with five 30-min intervention sessions per week, resulting in a total of 20 sessions. Therefore, each participant will have received a total of 40 sessions of the intervention, comprising 20 sessions of VR-MAT and 20 sessions of CT. The randomized group assignments were concealed in consecutively numbered sealed opaque envelopes placed in a plastic container. The allocated group will then be determined by the sequential opening of the envelopes after obtaining written informed consent from the participants. Participants will receive a conventional inpatient rehabilitation program (1 h of physical therapy or 30 min of occupational therapy); however, they will not receive a cognitive intervention. Outcome measurements will be performed by a researcher blinded to the group assignment.
2.2. Participants
Participants will be recruited among patients who have been admitted to the neurorehabilitation unit of the rehabilitation hospital. The inclusion criteria are as follows: (1) cognitive impairment secondary to a first-ever ABI, as evidenced by a Clinical Dementia Scale (CDR) score of 0.5 – 1; (2) a diagnosis of ABI > 3 months ago with medical records and/or brain imaging; (3) no history of prior ABI; (4) upper limb muscle strength of ≥ 3 on the medical research council scale, indicating suitability for training and assessment; (5) age >19 years. The exclusion criteria are: (1) known cognitive impairment prior to ABI; (2) severe aphasia or cognitive communication disorder precluding cognitive intervention, including MAT and CCT, or assessment; (3) neurological or psychiatric disorders other than ABI; (4) severe depression (Geriatric Depression Scale-short form score of ≥8); (5) uncontrolled medical illnesses, such as severe cardiac or respiratory disease. The exclusion criteria are as follows: (1) known cognitive impairment prior to ABI; (2) severe aphasia or cognitive communication disorder which precluded cognitive intervention including MAT and CCT or assessment; (3) neurological or psychiatric disorder other than ABI; (4) severe depression [Geriatric Depression Scale-short form ≥8]; (5) uncontrolled medical illness such as severe cardiac or respiratory disease. The experimental protocol was approved by the ethics committee of the rehabilitation hospital (IRB no. NRC-2015-04-032). Sample size calculation was not possible because the effects of the VR-MAT on cognitive function have not yet been reported. Therefore, we have set the sample size to 12 for each arm, in accordance with the minimum number recommended for pilot trials (46, 47). In total, considering a potential participant attrition rate of 20%, we aim to recruit 24 participants for this pilot study.
2.3. Virtual reality-based music attention training
VR-MAT comprises virtual reality-based, visually guided, bilateral drumming on electronic instruments. The VR-MAT is designed to fit individual therapeutic sessions and employs the patients’ preferred songs given in a multisensory environment (i.e., visual, auditory, and haptic). The VR-MAT consists of evaluation and training sessions. The VR-MAT-Evaluation includes baseline tempo and visuomotor response assessment tasks, aiming to ascertain individual variations, providing baseline information for VR-MAT-Training. In the baseline tempo assessment, participants will be asked to play the drums at a comfortable tempo for 10 s using their dominant and non-dominant hands independently. The baseline tempo assessment will be repeated thrice. Once the baseline tempo of the patients’ drumming is calculated, the participants will be asked to play a visually cued rhythmic pattern on the given electronic drum. The rhythm patterns will consist of four-beat rhythmic patterns with a binary meter, representative of four popular music genres, Rock, Swing, Shuffle, Bossa-Nova. Each genre will encompass rhythmic patterns that vary in the level of difficulty. Figure 1 presents examples of rhythm pattern varied across difficulty level within the Rock genre. Visual cues will be presented at a comfortable tempo via flashes on a computer screen, positioned 70–80 cm in front of the patients. In the VR-MAT-Evaluation, we will also collect information about participants’ previous musical experiences, including years of music training, types of musical activities they have participated in, and their musical preferences.
VR-MAT-Training consists of four levels of visually guided drumming tasks designed to restore various attention types in a hierarchical manner: (1) MAT-Level 1: One hand drumming, (2) MAT-Level 2: Two hand drumming, (3) MAT-Level 3: Reversed drumming, and (4) MAT-Level 4: Alternating drumming. In VR-MAT Training Level 1, a four-beat rhythm pattern is visually presented on the computer screen, first on the left side, and then independently on the right side. In VR-MAT Training Level 2, the visual cues used in Session Level 1 are combined and presented concurrently. VR-MAT-Training Level 3 is a two-hand drumming task that employs reversed rhythm patterns compared with those used in Level 2. For example, the visual patterns presented on the right side are presented on the left side and vice versa. Because individuals already possess habituated body patterns, they must inhibit previously learned patterns while learning new reverse patterns. Finally, VR MAT Training Level 4 provides the rhythm patterns of Levels 2 and 3 in a randomly alternating manner. Each task includes 20–21 trials, and the completion time is approximately 40 min.
Given the nature of bilateral drumming, the VR-MAT utilizes a familiar four-beat rhythmic pattern with a binary meter. Rhythmic patterns are carefully designed to vary the level of difficulty based on the VR-MAT-Evaluation results. Background music (BGM), originally composed of a binary meter, is selected from the patient’s preferred song list. The BGM can be played by a therapist or recorded without a vocal track. In both cases, adjustment of the BGM tempo is necessary to maintain the baseline tempo of the patients. With the auditory feedback (either a kicking or snaring drum sound), three types of visual cues are given: (a) if the drum stroke is falling within a time range of ±150 millisecond, a flashlight is given (i.e., matched), (b) if the drum stroke is hit earlier than the exact target point, ranging between −300 to −150 millisecond, the ball is exploded (i.e., mismatched), (c) if the ball is missed in the time range mentioned above, no visual cue is presented (i.e., unmatched). Figure 2 shows the user interface of VR-MAT, and Figure 3 presents example rhythm patterns for each of the four training levels.
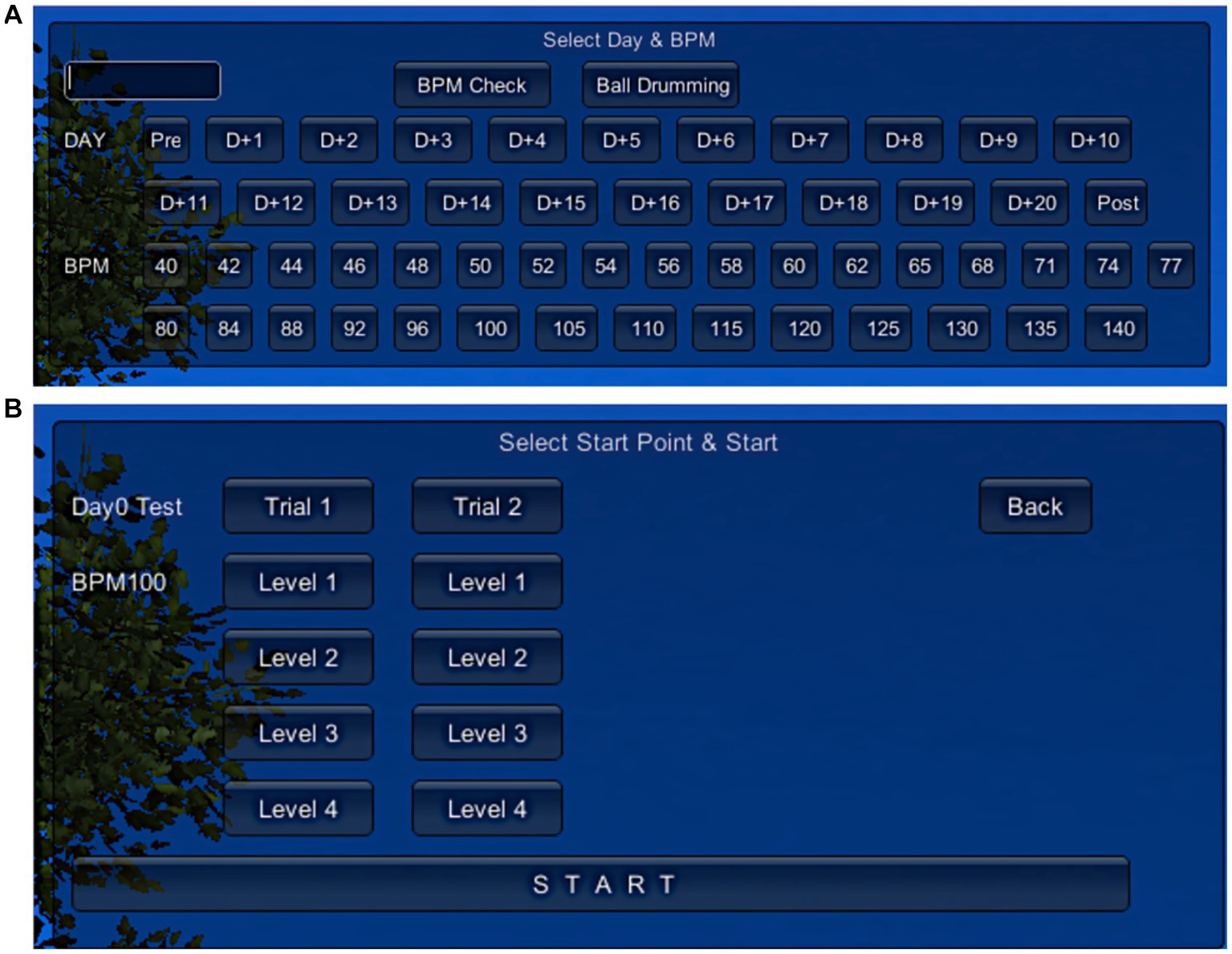
Figure 2. User interface of VR-MAT. (A) The first page of the UI includes four sections; User ID input, Warm-up exercise (BPM check, Free ball drumming), Day, and BPM selection; (B) The second page of the UI includes selection of trials and levels.
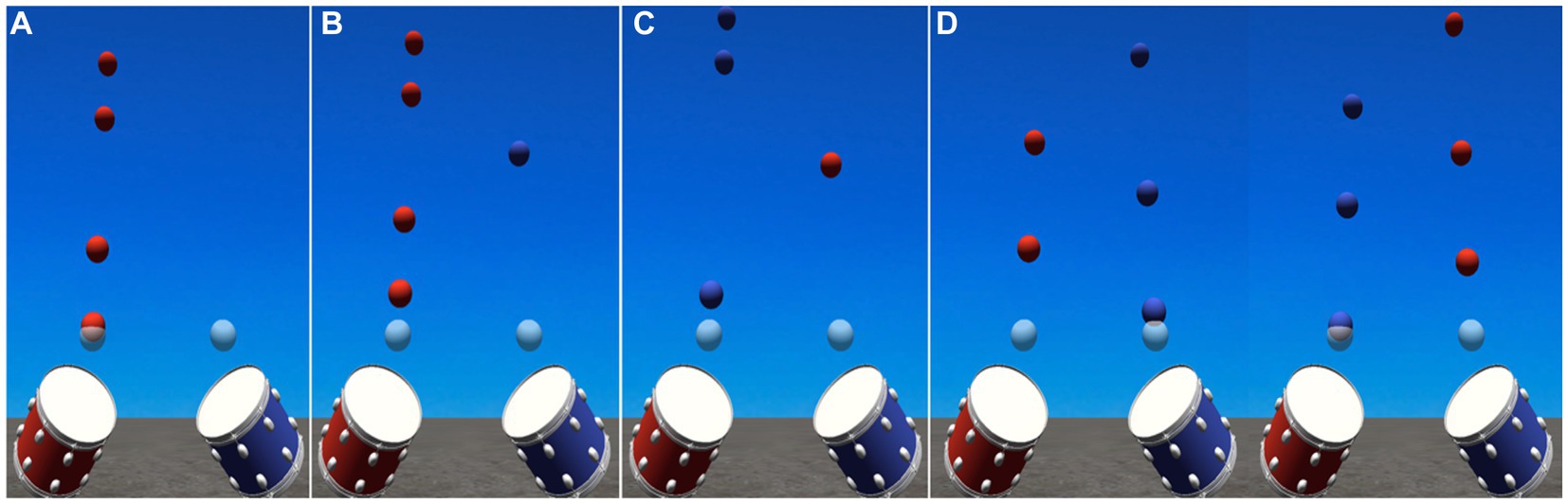
Figure 3. Examples of each of the four levels of training. (A) VR-MAT Level 1; One-hand drumming; (B) VR-MAT Level 2; Two-hand drumming, (C) VR-MAT Level 3; Two-hand drumming-reversed, (D) VR-MAT Level 4; Two-hand drumming-randomized.
2.3.1. VR-MAT behavioral data
We will evaluate the VR-MAT performance four times [pre (day 1), mid1 (day 8), mid2 (day 15), and post (day 22) sessions]. We will obtain (1) the VR-MAT performance accuracy, which is defined as the number of matched strokes divided by the total number of strokes, and (2) the VR-MAT response time (RT), which is the time required for the matched strokes. No background music will be played during the evaluation sessions.
2.3.2. Equipment set-up
All musical stimuli will be generated using Logic Pro X (Apple Inc., California, United States) and combined with the visual stimuli using Unity (Unity Technologies, San Francisco, United States). The electronic drum consists of tom-toms on an adjustable stand, which is connected to a screen, speakers, and a desktop computer. A total minimum area of two meters squared will be required to set up the tom-toms on the stand, including space for the participant to sit (Figure 4).
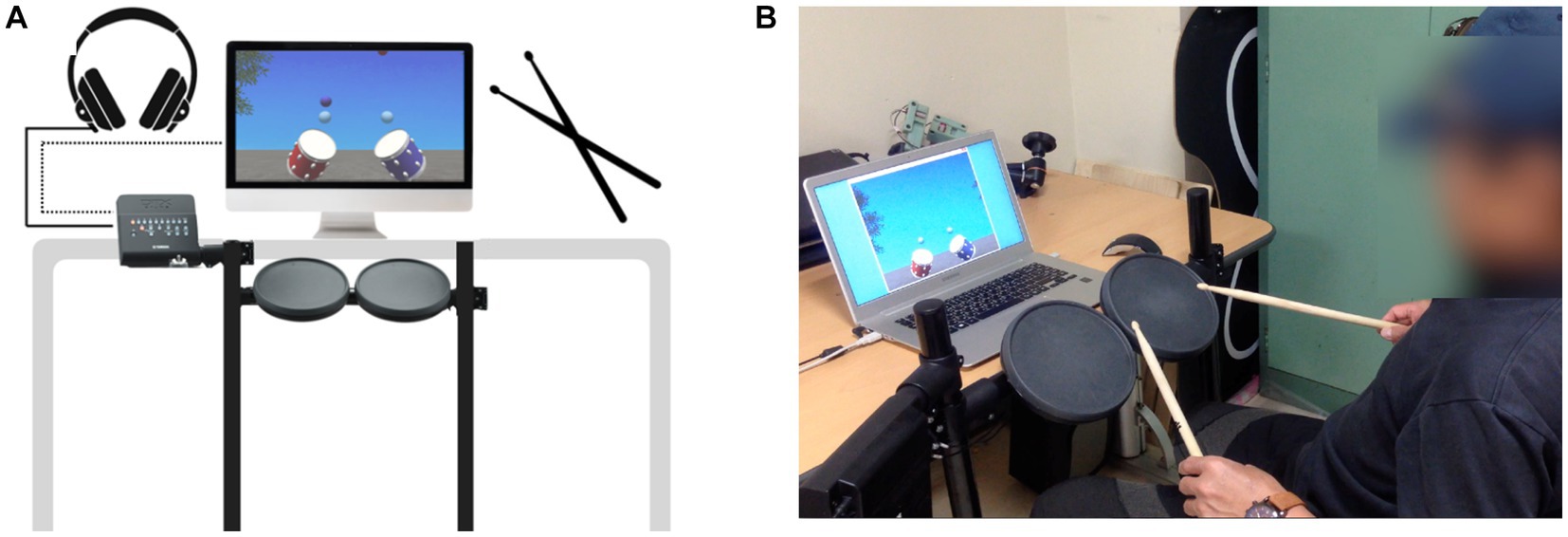
Figure 4. (A) Example of equipment set-up. A control panel of the electronic drum is connected to a desktop computer and a monitor. Accompaniment and sound feedback will be provided via a headphone that is connected to the control panel. (B) An individual demonstrating VR-MAT.
2.4. Conventional cognitive training
The CCT was designed to mimic attention process training, which has proven to be effective in patients with ABI(48, 49). Therefore, the CCT encompasses various levels of attention, such as focused, sustained, selective, alternating, and divided attention, and progresses with the participants’ levels. The training difficulty level is adjusted by modifying tasks, such as altering the number of presented stimuli. Feedback is provided after each task, and the therapist provides a summary at the end of each session. The CCT is administered by an experienced occupational therapist, utilizing diverse training methods including cards, dictation, buzzer, paper-and-pencil tasks, or computerized cognitive training.
2.5. Outcome measurement
Baseline demographic characteristics, including sex, age, diagnosis of ABI, duration after ABI, side of brain lesion, and education, will be collected. Additionally, neuropsychological outcomes will be measured for each participant at baseline, after 20 sessions (4 weeks), and after 40 sessions of intervention (after 20 sessions of alternative intervention; 8 weeks) by a blinded neuropsychologist. We will collect data on neuropsychological outcomes in two ways: global cognitive function and executive function, which are specific targets of VR-MAT. All of the cognitive function tests will be administered in Korean. Additionally, we will obtain VR-MAT behavioral data, which is previously explained, and functional infrared spectroscopy (fNIRS) data (Table 1).
2.5.1. Global neuropsychological assessment
We will administer the mini-mental status examination (MMSE) (48), clinical dementia rating (CDR) (49), and global deterioration scale (GDS) (50) for the global cognitive functions and subjective memory complaint questionnaires (SMCQ) (51) for subjective cognitive function by an experienced research neuropsychologist. The MMSE is the most widely used screening tool for cognitive deficits. It comprises 30 items that provide information about orientation for time and place, memory (encoding and delayed recall), attention, calculation, language, and visuospatial construction. The CDR is a semi-structured informant-based clinical interview that rates global cognitive performance and functioning. It comprises six domains (memory, orientation, judgment and problem-solving, community affairs, home and hobbies, and personal care) (49). The global CDR score (range 0–3) will be calculated using an established algorithm indicating dementia severity: 0, no dementia; 0.5, questionable dementia; 1, mild dementia; 2, moderate dementia; and 3, severe dementia. The CDR sum of the box (CDR-SOB; range, 0–18) will be obtained as the sum of each domain score. A higher global CDR or CDR-SOB score indicates a greater severity of dementia. The Korean version of the CDR has also been validated (52). The GDS, which emphasizes memory function and the ability to complete activities of daily living, is used to rate the severity of cognitive deterioration in seven stages ranging from “no global impairment” (stage 1) to “very severe global impairment” (stage 7). Stages 1–3 indicate pre-dementia and stages 4–7 represent dementia (8). In addition, we will measure subjective cognitive function (beliefs about one’s own memory) using a 14-item of SMCQ (subjective memory complaint questionnaire). The total SMCQ score ranges from 0 to 14, with higher scores indicating more severe subjective cognitive decline (51).
2.5.2. Executive function assessment
Targeting executive function specifically, we will perform Trail Making Test (TMT) (53), TMT-Black & White (TMT-B&W) (54) as paper-and-pencil tests, and computerized cognitive function tests (Attention-Switching Task [AST], Stop Signal Task [SST], Spatial Working Memory [SWM]) from the Cambridge Neuropsychological Test Automated Battery (CANTAB) (55). Participants will be seated comfortably in front of a desk and will be asked to perform the tests. The TMT consists of parts A (TMT A) and B (TMT B), in which TMT A is related to visual attention skills and TMT-B is related to executive functions. The TMT-A requires participants to draw lines to connect randomly distributed numbers in ascending order, whereas the TMT-B requires participants to connect numbers and letters alternatively (56, 57). The TMT-B&W is similarly constructed. The TMT-B&W A will prompt the participants to connect the circles containing numbers in ascending order. In contrast, TMT-B&W B will prompt the participants to connect the numbers in consecutive order, alternating between the two color sets (black and white) (54). TMT is measured as the time taken to complete the task in parts A (TMT A) and B (TMT B), and we obtained TMT A, TMT B, TMT-B&W A, and TMT-B&W B from both tests. In addition, we obtained TMT B-TMT A by subtracting TMT A from TMT B, which reflects greater executive function, sequestering psychomotor speed, and visual search (58, 59).
The researcher will administer the CANTAB tests using a two-button press pad connected to a touchscreen tablet computer, following the administration guide. The AST reflects selective attention and cognitive flexibility, along with top-down executive function, measuring cued attentional set-shifting, which manages conflicting information and inhibits task-irrelevant information. In each trial, the participants will see instructions presented on the monitor about which stimulus they should respond to, either the placement (right or left on the screen) or the direction (to the right or left) of an arrow. The participants will then be asked to press the right or left button according to the presented arrow. Thus, some trials will present congruent stimuli (placement and direction are identical), whereas others will display incongruent stimuli (the side on which the arrow appeared), thereby assessing the ability to switch attention between the placement and direction of an arrow. There are two different conditions between the two consecutive trials: non-switched trials (previous and present trials are identical) and switched trials (previous and present trials are not identical). AST outcome measures include response time (RT) and the number of correct trials; latency in congruent, incongruent, and all conditions; and percent correct (rate of correct response). A lower RT or a higher number of correct trials indicate better performance. In addition, we will obtain the following variables: congruency cost (ms, RT in incongruent condition – RT in congruent condition) and switch cost (ms, RT in switched trial – Rt in non-switched trial), in which a lower cost indicates better executive function.
SWM reflects spatial working memory or executive function through a search task, which is the ability to retain and manipulate visuospatial information. In each trial, the participants will be presented with randomly located colored boxes on the computer screen and will be asked to search through those boxes by touching each box to reveal its contents and find all the hidden tokens, in which the number of hidden tokens corresponded to the number of boxes. When they find a yellow token inside the box, it will be moved to, and fill the column on, the right side of the screen. The yellow token is never hidden in the same box twice; thus, the participants will be told to try to find the next hidden token, open the unselected box, and not revisit the previously opened box until they find the token. It is considered an error if the participant opens any box that is already opened. When the participants find a token in each box, the trial ends. As the participants complete each trial, the number of boxes increases from four to six to eight boxes. To reduce errors, the participants must remember where the tokens were previously hidden. The outcomes of SWM are “between errors” and “strategy.” Between errors is defined as the number of revisits to the box in which the participants already found a yellow token in the previous search, with a lower value indicating higher performance. The strategy is the effectiveness of the participants’ searching, in which participants follow a pre-specified sequence beginning with a specific box and return to the same box to start a new sequence. It ranges from 8 to 56, with a higher score indicating noncompliance with a specific strategy and a poor strategy.
The SST measures an individual’s ability to inhibit a response that has already been initiated, in two stages (go and stop trials). (1) Go trial: The subjects are requested to make a speeded response by pressing a button depending on the direction of an arrow pointing (left button for a left-pointing arrow and right button for a right-pointing arrow). (2) Stop trial: If an audio tone (a beep signal) is presented, participants should withhold any response to the stimulus and not press the button. The SST outcome measures include stopped signal reaction time (SSRT) and the proportion of successful stops, which are calculated from the last half of the trials. The SSRT is an indirect estimate of the inhibitory response speed, indicating the time required to abort a pre-potent action in the presence of a stop signal (stop signal delay), which is calculated by subtracting the RT on the stop trial from the mean RT on the go trial. A lower SSRT indicates a faster response and better performance. The proportion of successful stops is obtained with the number of times of successful stops, in which the participant stopped successfully, divided by the total number of stop signals, with higher indicating better performance.
2.6. Functional near-infrared spectroscopy
We will also examine the hemodynamic changes using fNIRS. The hemodynamic responses will be obtained at a sampling rate of 0.65 s, and the data will be converted to concentrated changes of hemoglobin using the modified Beer–Lambert law. Subsequently, a zero-phase low-and high-pass filter with a cut-off frequency of 0.01–0.09 Hz will be applied using a matrix laboratory (MATLAB) (60–62). Additionally, we will employ vector-based phase analysis to examine multifaceted aspects of cerebral hemodynamic changes (63–66). Vector-based phase analysis is based on an orthogonal vector coordinate plane defined by four indices: oxygenated hemoglobin, deoxygenated hemoglobin, total hemoglobin concentration, and oxygen level changes in the blood vessels. The four indices will be subjected to the calculation of angle k, which indicates the degree of oxygen exchange (66–68), and a scalar L, which indicates the amount of hemoglobin variation (69), and will be further used to calculate the phase-associated response intensity (PRI).
2.7. Statistical analysis
We will perform descriptive statistical analysis of the general demographics of the study participants. We define completers as participants who finish 20 sessions of either intervention (VR-MAT or CT) and undergo measurements at 4 weeks of intervention. The statistical analysis will be conducted on an intention to treat basis and the last observation carried forward method will be used to complete the data. Adherence will be reported as the number of completers among enrolled participants. For neuropsychological assessments, a comparative analysis between two different intervention sequences (VC or CV) will be performed and the carry-over effects will be tested using t-tests on the change in each sequence for all crossover endpoints (70, 71). For variables with insignificant carry-over effects, the changes in variables during specific interventions from either sequence will be combined and compared. For patients with significant carry-over effects, we will compare the changes in specific interventions during the first period (20 sessions of either intervention). Comparisons between interventions will be performed using a two-tailed t-test or the Mann–Whitney U test, depending on normality. For music behavior responses obtained from the VR-MAT data, we will perform a two-way repeated-measures analysis of variance (ANOVA) on level (levels 1, 2, 3, and 4) and time (pre, day 8, day 15, and post) with Bonferroni post-hoc comparisons.
Repeated measures of correlation between executive function assessment and VR-MAT variables will be examined to determine the linear relationship between paired repeated data using the R package rmcorr from R.3.6.1 (72). The independent variables will remain the same for the analysis of fNIRS data, and the dependent variables will be the PRIs obtained from all channels. All analyses will be performed using the software package R.3.6.1,1 and the level of significance will be set to 0.05.
3. Discussion
Instrumental music playing combined with VR technology might be a promising strategy for increasing the effectiveness of MAT by providing individualized and systematic interventions. This study aims to develop a replicable therapeutic protocol using an active music-playing platform. This topic is essential, considering that individuals need customized training programs suited to their functional levels and musical preferences. In addition, an increasing number of individuals require remote access to music-based attention programs, specifically those with restricted mobility. Moreover, our system can be used as a cognitive function measurement tool because music behavior data will be recorded with regard to accuracy and response time across all sessions. The data will be used to set up an individualized rhythm, determine whether to proceed to the next level of trial, and track changes over time.
The effectiveness of the system will be explored using cognitive outcome measures, including global and specific cognitive function test, especially executive function. Attention is a key component of executive function, as attention direct cognitive resources toward more important stimuli in order to sustain information processing and engaging and disengaging attention is linked to flexibility or inhibitory control [3; 4]. Thus, our cognitive outcomes could demonstrate how improvements in attentional abilities impact executive function, moreover global cognitive function. In addition, fNIRS data employ multifaceted variables of cerebral hemodynamics to indicate a PRI, which can provide interpretable information in terms of relationships and intensity changes over time. Therefore, the present study will contribute to increasing the scientific rigor of understanding how active music playing can affect cognitive function and cerebral hemodynamic responses, thus demonstrating its efficacy in cognitive rehabilitation. This specific study protocol enables music therapists to measure and quantify musical responses and clearly indicates how changes in musical responses are related to and can lead to cognitive enhancement. In addition, the evaluation and training protocols that we designed are readily applicable to music therapy in medical and rehabilitation settings if the use of electronic media and information technologies readily gains acceptance.
Author contributions
EJ contributed to the conception and design of the VR-MAT. J-HS contributed to the clinical design and clinical assessments. EJ and J-HS drafted the manuscript, critically reviewed the manuscript for important intellectual content. All authors have read and approved the final manuscript.
Funding
This work was supported by the Ministry of Education of the Republic of Korea and the National Research Foundation of Korea (NRF-2018S1A5A2A03034582) and the Technology Innovation Program (or Industrial Strategic Technology Development Program-Technology Innovation Program) (20014480, A light-weight wearable upper limb rehabilitation robot system and untact self-training and assessment platform customizable for individual patient) funded By the Ministry of Trade, Industry & Energy (MOTIE, Korea).
Conflict of interest
The authors declare that the research was conducted in the absence of any commercial or financial relationships that could be construed as a potential conflict of interest.
Publisher’s note
All claims expressed in this article are solely those of the authors and do not necessarily represent those of their affiliated organizations, or those of the publisher, the editors and the reviewers. Any product that may be evaluated in this article, or claim that may be made by its manufacturer, is not guaranteed or endorsed by the publisher.
Footnotes
References
1. van Velzen, JM, van Bennekom, CA, van Dormolen, M, Sluiter, JK, and Frings-Dresen, MH. Factors influencing return to work experienced by people with acquired brain injury: a qualitative research study. Disabil Rehabil. (2011) 33:2237–46. doi: 10.3109/09638288.2011.563821
2. Kashluba, S, Hanks, RA, Casey, JE, and Millis, SR. Neuropsychologic and functional outcome after complicated mild traumatic brain injury. Arch Phys Med Rehabil. (2008) 89:904–11. doi: 10.1016/j.apmr.2007.12.029
3. Ciuffreda, KJ, Kapoor, N, Rutner, D, Suchoff, IB, Han, ME, and Craig, S. Occurrence of oculomotor dysfunctions in acquired brain injury: a retrospective analysis. Optom Am Optom Assoc. (2007) 78:155–61. doi: 10.1016/j.optm.2006.11.011
4. Barrow, IM, Collins, JN, and Britt, LD. The influence of an auditory distraction on rapid naming after a mild traumatic brain injury: a longitudinal study. J Trauma Acute Care Surg. (2006) 61:1142–9. doi: 10.1097/01.ta.0000241238.70269.c1
5. Holmqvist, K, Kamwendo, K, and Ivarsson, A-B. Occupational therapists’ descriptions of their work with persons suffering from cognitive impairment following acquired brain injury. Scand J Occup Ther. (2009) 16:13–24. doi: 10.1080/11038120802123520
6. Scheibel, RS, Newsome, MR, Steinberg, JL, Pearson, DA, Rauch, RA, Mao, H, et al. Altered brain activation during cognitive control in patients with moderate to severe traumatic brain injury. Neurorehabil Neural Repair. (2007) 21:36–45. doi: 10.1177/1545968306294730
7. Messinis, L, Kosmidis, MH, Nasios, G, Dardiotis, E, and Tsaousides, T. Cognitive neurorehabilitation in acquired neurological brain injury. Behav Neurol. (2019) 2019:1–4. doi: 10.1155/2019/8241951
8. Cicerone, KD, Goldin, Y, Ganci, K, Rosenbaum, A, Wethe, JV, Langenbahn, DM, et al. Evidence-based cognitive rehabilitation: systematic review of the literature from 2009 through 2014. Arch Phys Med Rehabil. (2019) 100:1515–33. doi: 10.1016/j.apmr.2019.02.011
9. Loetscher, T, Potter, KJ, Wong, D, and das Nair, R. Cognitive rehabilitation for attention deficits following stroke. Cochrane Database Syst Rev. (2019) 2019:CD002842. doi: 10.1002/14651858.CD002842.pub3
10. Sinclair, KL, Ponsford, JL, Rajaratnam, SMW, and Anderson, C. Sustained attention following traumatic brain injury: use of the psychomotor vigilance task. J Clin Exp Neuropsychol. (2013) 35:210–24. doi: 10.1080/13803395.2012.762340
11. Slovarp, L, Azuma, T, and Lapointe, L. The effect of traumatic brain injury on sustained attention and working memory. Brain Inj. (2012) 26:48–57. doi: 10.3109/02699052.2011.635355
12. Lempke, LB, Lynall, RC, Hoffman, NL, Devos, H, and Schmidt, JD. Slowed driving-reaction time following concussion-symptom resolution. J Sport Heal Sci. (2021) 10:145–53. doi: 10.1016/j.jshs.2020.09.005
13. Kelly, C, Foxe, JJ, and Garavan, H. Patterns of normal human brain plasticity after practice and their implications for neurorehabilitation. Arch Phys Med Rehabil. (2006) 87:S20–9. doi: 10.1016/j.apmr.2006.08.333
14. Jones, C. The use of therapeutic music training to remediate cognitive impairment following an acquired brain injury: the theoretical basis and a case study. Healthcare. (2020) 8:327. doi: 10.3390/healthcare8030327
15. Cicerone, KD, and Giacino, JT. Remediation of executive function deficits after traumatic brain injury. Neurorehabilitation. (1992) 2:12–22. doi: 10.3233/NRE-1992-2304
16. Cicerone, KD, Dahlberg, C, Kalmar, K, Langenbahn, DM, Malec, JF, Bergquist, TF, et al. Evidence-based cognitive rehabilitation: recommendations for clinical practice. Arch Phys Med Rehabil. (2000) 81:1596–615. doi: 10.1053/apmr.2000.19240
17. Cicerone, KD, Dahlberg, C, Malec, JF, Langenbahn, DM, Felicetti, T, Kneipp, S, et al. Evidence-based cognitive rehabilitation: updated review of the literature from 1998 through 2002. Arch Phys Med Rehabil. (2005) 86:1681–92. doi: 10.1016/j.apmr.2005.03.024
18. Gray, JR, Chabris, CF, and Braver, TS. Neural mechanisms of general fluid intelligence. Nat Neurosci. (2003) 6:316–22. doi: 10.1038/nn1014
19. Levine, B, Robertson, IH, Clare, L, Carter, G, Hong, J, Wilson, BA, et al. Rehabilitation of executive functioning: an experimental-clinical validation of goal management training. J Int Neuropsychol Soc. (2000) 6:299–312. doi: 10.1017/S1355617700633052
20. Kaipio, M-L, Cheour, M, Čeponienė, R, Öhman, J, Alku, P, and Näätänen, R. Increased distractibility in closed head injury as revealed by event-related potentials. Neuroreport Rapid Commun Neurosci Res. (2000) 11:1463–8. doi: 10.1097/00001756-200005150-00021
21. Schaefer, M, Tewes, U, Münte, TF, and Johannes, S. Lateralized irrelevant speech alters visuospatial selective attention mechanisms. Biol Psychol. (2006) 72:51–8. doi: 10.1016/j.biopsycho.2005.07.007
22. Kaipio, ML, Cheour, M, Öhman, J, Salonen, O, and Näätänen, R. Mismatch negativity abnormality in traumatic brain injury without macroscopic lesions on conventional MRI. Neuroreport. (2013) 24:440–4. doi: 10.1097/WNR.0b013e32836164b4
23. Barker-Collo, S, Feigin, V, Lawes, C, Parag, V, and Senior, H. Attention deficits after incident stroke in the acute period: frequency across types of attention and relationships to patient characteristics and functional outcomes. Top Stroke Rehabil. (2010) 17:463–76. doi: 10.1310/tsr1706-463
24. Solbakk, A-K, Reinvang, I, and Andersson, S. Assessment of P3a and P3b after moderate to severe brain injury. Clin Electroencephalogr. (2002) 33:102–10. doi: 10.1177/155005940203300306
25. Trainor, LJ, McDonald, KL, and Alain, C. Automatic and controlled processing of melodic contour and interval information measured by electrical brain activity. J Cogn Neurosci. (2002) 14:430–42. doi: 10.1162/089892902317361949
26. Janata, P, Tillmann, B, and Bharucha, JJ. Listening to polyphonic music recruits domain-general attention and working memory circuits. Cogn Affect Behav Neurosci. (2002) 2:121–40. doi: 10.3758/CABN.2.2.121
27. Satoh, M, Takeda, K, Nagata, K, Hatazawa, J, and Kuzuhara, S. Activated brain regions in musicians during an ensemble: a PET study. Cogn Brain Res. (2001) 12:101–8. doi: 10.1016/S0926-6410(01)00044-1
28. Uhlig, M, Fairhurst, MT, and Keller, PE. The importance of integration and top-down salience when listening to complex multi-part musical stimuli. Neuroimage. (2013) 77:52–61. doi: 10.1016/j.neuroimage.2013.03.051
29. Bigand, E, McAdams, S, and Foret, S. Divided attention in music. Int J Psychol. (2000) 35:270–8. doi: 10.1080/002075900750047987
30. Byo, JL. The effects of texture and number of parts on the ability of music majors to detect performance errors. J Res Music Educ. (1997) 45:51–66. doi: 10.2307/3345465
31. Crawley, EJ, Acker-Mills, BE, Pastore, RE, and Weil, S. Change detection in multi-voice music: the role of musical structure, musical training, and task demands. J Exp Psychol Percept Perform. (2002) 28:367–78. doi: 10.1037//0096-1523.28.2.367
32. Fujioka, T, Trainor, LJ, Ross, B, Kakigi, R, and Pantev, C. Automatic encoding of polyphonic melodies in musicians and nonmusicians. J Cogn Neurosci. (2005) 17:1578–92. doi: 10.1162/089892905774597263
33. Davison, LL, and Banks, WP. Selective attention in two-part counterpoint. Music Percept. (2003) 21:3–20. doi: 10.1525/mp.2003.21.1.3
34. Wit, V, Knox, R, Jutai, J, and Loveszy, R. Music therapy and rehabilitation of attention in brain injury: a pilot study. Can J Music Ther. (1994) 21:99–104.
35. Sohlberg, MM, and Mateer, CA. Effectiveness of an attention-training program. J Clin Exp Neuropsychol. (1987) 9:117–30. doi: 10.1080/01688638708405352
36. Knox, R, Yokota-Adachi, H, Kershner, J, and Jutai, J. Musical attention training program and alternating attention in brain injury: an initial report. Music Ther Perspect. (2003) 21:99–104. doi: 10.1093/mtp/21.2.99
37. Bower, J, Catroppa, C, Grocke, D, and Shoemark, H. Music therapy for early cognitive rehabilitation post-childhood TBI: an intrinsic mixed methods case study. Dev Neurorehabil. (2014) 17:339–46. doi: 10.3109/17518423.2013.778910
38. Abrahams, TP, and van Dooren, JC. Musical attention control training (MACT) in secure residential youth care: a randomised controlled pilot study. Arts Psychother. (2018) 57:80–7. doi: 10.1016/j.aip.2017.10.008
39. Pasiali, V, LaGasse, AB, and Penn, SL. The effect of musical attention control training (MACT) on attention skills of adolescents with neurodevelopmental delays: a pilot study. J Music Ther. (2014) 51:333–54. doi: 10.1093/jmt/thu030
40. van Alphen, R, Stams, GJJM, and Hakvoort, L. Musical attention control training for psychotic psychiatric patients: an experimental pilot study in a forensic psychiatric hospital. Front Neurosci. (2019) 13:570. doi: 10.3389/fnins.2019.00570
41. Bradt, J, Magee, WL, Dileo, C, Wheeler, BL, and McGilloway, E. Music therapy for acquired brain injury. Cochrane Database Syst Rev. (2010) 7:CD006787. doi: 10.1002/14651858.CD006787.pub2
42. Magee, WL, Clark, I, Tamplin, J, and Bradt, J. Music interventions for acquired brain injury. Cochrane Database Syst Rev. (2017) 2017:CD006787. doi: 10.1002/14651858.CD006787.pub3
43. Särkämö, T, Tervaniemi, M, Laitinen, S, Forsblom, A, Soinila, S, Mikkonen, M, et al. Music listening enhances cognitive recovery and mood after middle cerebral artery stroke. Brain. (2008) 131:866–76. doi: 10.1093/brain/awn013
44. Pool, J. Brief group music therapy for acquired brain injury: Cognition and emotional needs (PhD thesis). Cambridge, UK: Anglia Ruskin University. (2013).
45. Thaut, MH, Gardiner, JC, Holmberg, D, Horwitz, J, Kent, L, Andrews, G, et al. Neurologic music therapy improves executive function and emotional adjustment in traumatic brain injury rehabilitation. Ann N Y Acad Sci. (2009) 1169:406–16. doi: 10.1111/j.1749-6632.2009.04585.x
46. Julious, SA. Sample size of 12 per group rule of thumb for a pilot study. J Appl Stat Pharm Ind. (2005) 4:287–91. doi: 10.1002/pst.185
47. Hertzog, MA. Considerations in determining sample size for pilot studies. Res Nurs Health. (2008) 31:180–91. doi: 10.1002/nur.20247
48. Folstein, MF, Robins, LN, and Helzer, JE. The mini-mental state examination. Arch Gen Psychiatry. (1983) 40:812. doi: 10.1001/archpsyc.1983.01790060110016
49. Morris, JC. The clinical dementia rating (CDR): current version and scoring rules. Young. (1991) 41: 1588–1592.
50. Reisberg, B, Ferris, SH, de Leon, MJ, and Crook, T. The global deterioration scale for assessment of primary degenerative dementia. Am J Psychiatry. (1982) 139:1136–9. doi: 10.1176/ajp.139.9.1136
51. Youn, JC, Kim, KW, Lee, DY, Jhoo, JH, Lee, SB, Park, JH, et al. Development of the subjective memory complaints questionnaire. Dement Geriatr Cogn Disord. (2009) 27:310–7. doi: 10.1159/000205512
52. Choi, SH, Na, DL, Lee, BH, Hahm, D-S, Jeong, JH, Yoon, SJ, et al. Estimating the validity of the Korean version of expanded clinical dementia rating (CDR) scale. J Korean Neurol Assoc. (2001) 19:585–91.
53. Reitan, RM, and Wolfson, D. A selective and critical review of neuropsychological deficits and the frontal lobes. Neuropsychol Rev. (1994) 4:161–98. doi: 10.1007/BF01874891
54. Kim, HJ, Baek, MJ, and Kim, S. Alternative type of the trail making test in nonnative English-speakers: the trail making test-black & white. PLoS One. (2014) 9:e89078. doi: 10.1371/journal.pone.0089078
55. Robbins, TW, James, M, Owen, AM, Sahakian, BJ, McInnes, L, and Rabbitt, P. Cambridge neuropsychological test automated battery (CANTAB): a factor analytic study of a large sample of normal elderly volunteers. Dement Geriatr Cogn Disord. (2004) 5:266–81. doi: 10.1159/000106735
56. Corrigan, JD, and Hinkeldey, NS. Relationships between parts a and B of the trail making test. J Clin Psychol. (1987) 43:402–9. doi: 10.1002/1097-4679(198707)43:4<402::AID-JCLP2270430411>3.0.CO;2-E
57. Katherine ArbuthnottFrank, J. Trail making test, part B as a measure of executive control: validation using a set-switching paradigm. J Clin Exp Neuropsychol. (2000) 22:518–28. doi: 10.1076/1380-3395(200008)22:4;1-0;FT518
58. Strauss, E, Sherman, EMS, and Spreen, O. A compendium of neuropsychological tests: administration, norms, and commentary. New York, NY: Oxford University Press (2006).
59. Bowie, CR, and Harvey, PD. Administration and interpretation of the trail making test. Nat Protoc. (2006) 1:2277–81. doi: 10.1038/nprot.2006.390
60. Akgul Sankur, B, and Akin, ACB. Spectral analysis of event-related hemodynamic responses in functional near infrared spectroscopy. J Comput Neurosci. (2005) 18:67–83. doi: 10.1007/s10827-005-5478-2
61. Bauernfeind, G, Scherer, R, Pfurtscheller, G, and Neuper, C. Single-trial classification of antagonistic oxyhemoglobin responses during mental arithmetic. Med Biol Eng Comput. (2011) 49:979–84. doi: 10.1007/s11517-011-0792-5
62. Morren, G, Wolf, M, Lemmerling, P, Wolf, U, Choi, JH, Gratton, E, et al. Detection of fast neuronal signals in the motor cortex from functional near infrared spectroscopy measurements using independent component analysis. Med Biol Eng Comput. (2004) 42:92–9. doi: 10.1007/BF02351016
63. Herff, C, Heger, D, Putze, F, Hennrich, J, Fortmann, O, and Schultz, T. Classification of mental tasks in the prefrontal cortex using fNIRS. Annu Int Conf IEEE Eng Med Biol Soc. (2013):2160–3. doi: 10.1109/EMBC.2013.6609962
64. Cui, X, Bray, S, and Reiss, AL. Functional near infrared spectroscopy (NIRS) signal improvement based on negative correlation between oxygenated and deoxygenated hemoglobin dynamics. Neuroimage. (2010) 49:3039–46. doi: 10.1016/j.neuroimage.2009.11.050
65. Tachtsidis, I, and Scholkmann, F. False positives and false negatives in functional near-infrared spectroscopy: issues, challenges, and the way forward. Neurophotonics. (2016) 3:031405. doi: 10.1117/1.NPh.3.3.031405
66. Yoshino, K, Oka, N, Yamamoto, K, Takahashi, H, and Kato, T. Functional brain imaging using near-infrared spectroscopy during actual driving on an expressway. Front Hum Neurosci. (2013) 7:882. doi: 10.3389/fnhum.2013.00882
67. Oka, N, Yoshino, K, Yamamoto, K, Takahashi, H, Li, S, Sugimachi, T, et al. Greater activity in the frontal cortex on left curves: a vector-based fNIRS study of left and right curve driving. PLoS One. (2015) 10:e0127594. doi: 10.1371/journal.pone.0127594
68. Zafar, A, Hong, K-S, and Khan, MJ. “Initial dip detection based on both HbO and HbR vector-based phase analysis,” in Proceedings of the 2016 55th Annual Conference of the Society of Instrument and Control Engineers of Japan (SICE), pp. 543–548, Tsukuba, Japan. (2016).
69. Yoshino, K, and Kato, T. Vector-based phase classification of initial dips during word listening using near-infrared spectroscopy. Neuroreport. (2012) 23:947–51. doi: 10.1097/WNR.0b013e328359833b
70. Jones, B, and Kenward, MG. Design and analysis of cross-over trials. New York: Chapman and Hall/CRC (1989).
71. Wellek, S, and Blettner, M. On the proper use of the crossover design in clinical trials. Dtsch Arztebl Int. (2012) 109:276–81. doi: 10.3238/arztebl.2012.0276
Keywords: music therapy, attention deficit, acquired brain injury, virtual reality, attention evaluation and training, neurocognitive rehabilitation
Citation: Shin J-H and Jeong E (2023) Virtual reality-based music attention training for acquired brain injury: A protocol for randomized cross-over trial. Front. Neurol. 14:1192181. doi: 10.3389/fneur.2023.1192181
Edited by:
Stephanie Clarke, Centre Hospitalier Universitaire Vaudois (CHUV), SwitzerlandReviewed by:
Concetta Maria Tomaino, Institute for Music and Neurologic Function, United StatesShantala Hegde, National Institute of Mental Health and Neurosciences (NIMHANS), India
Copyright © 2023 Shin and Jeong. This is an open-access article distributed under the terms of the Creative Commons Attribution License (CC BY). The use, distribution or reproduction in other forums is permitted, provided the original author(s) and the copyright owner(s) are credited and that the original publication in this journal is cited, in accordance with accepted academic practice. No use, distribution or reproduction is permitted which does not comply with these terms.
*Correspondence: Eunju Jeong, ZWplb25nQGV3aGEuYWMua3I=