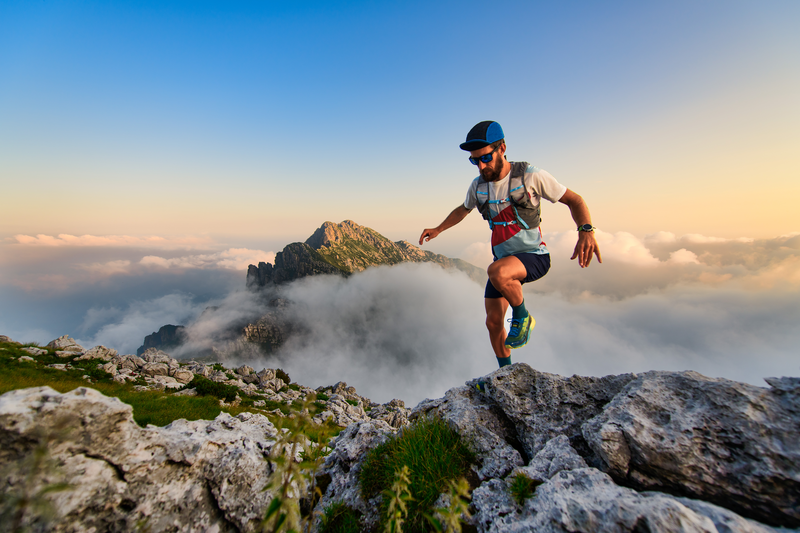
95% of researchers rate our articles as excellent or good
Learn more about the work of our research integrity team to safeguard the quality of each article we publish.
Find out more
SYSTEMATIC REVIEW article
Front. Neurol. , 21 June 2023
Sec. Neurorehabilitation
Volume 14 - 2023 | https://doi.org/10.3389/fneur.2023.1189034
This article is part of the Research Topic Combining a non-invasive transcranial stimulation technique with another therapeutic approach: mechanisms of action, therapeutic interest and tolerance View all 10 articles
Objective: This study aimed to elucidate the efficacy, safety, and long-term implications of vagus nerve stimulation (VNS) as a viable therapeutic option for patients with upper limb dysfunction following a stroke.
Methods: Data from the following libraries were searched from inception to December 2022: PubMed, Wanfang, Scopus, China Science and Technology Journal Database, Embase, Web of Science, China Biology Medicine Disc, Cochrane Library, and China National Knowledge Infrastructure. Outcomes included indicators of upper limb motor function, indicators of prognosis, and indicators of safety (incidence of adverse events [AEs] and serious AEs [SAEs]). Two of the authors extracted the data independently. A third researcher arbitrated when disputes occurred. The quality of each eligible study was evaluated using the Cochrane Risk of Bias tool. Meta-analysis and bias analysis were performed using Stata (version 16.0) and RevMan (version 5.3).
Results: Ten trials (VNS combined with rehabilitation group vs. no or sham VNS combined with rehabilitation group) with 335 patients were included in the meta-analysis. Regarding upper extremity motor function, based on Fugl–Meyer assessment scores, VNS combined with other treatment options had immediate (mean difference [MD] = 2.82, 95% confidence interval [CI] = 1.78–3.91, I2 = 62%, p < 0.00001) and long-term (day-30 MD = 4.20, 95% CI = 2.90–5.50, p < 0.00001; day-90 MD = 3.27, 95% CI = 1.67–4.87, p < 0.00001) beneficial effects compared with that of the control treatment. Subgroup analyses showed that transcutaneous VNS (MD = 2.87, 95% CI = 1.78–3.91, I2 = 62%, p < 0.00001) may be superior to invasive VNS (MD = 3.56, 95% CI = 1.99–5.13, I2 = 77%, p < 0.0001) and that VNS combined with integrated treatment (MD = 2.87, 95% CI = 1.78–3.91, I2 = 62%, p < 0.00001) is superior to VNS combined with upper extremity training alone (MD = 2.24, 95% CI = 0.55–3.93, I2 = 48%, p = 0.009). Moreover, lower frequency VNS (20 Hz) (MD = 3.39, 95% CI = 2.06–4.73, I2 = 65%, p < 0.00001) may be superior to higher frequency VNS (25 Hz or 30 Hz) (MD = 2.29, 95% CI = 0.27–4.32, I2 = 58%, p = 0,03). Regarding prognosis, the VNS group outperformed the control group in the activities of daily living (standardized MD = 1.50, 95% CI = 1.10–1.90, I2 = 0%, p < 0.00001) and depression reduction. In contrast, quality of life did not improve (p = 0.51). Safety was not significantly different between the experimental and control groups (AE p = 0.25; SAE p = 0.26).
Conclusion: VNS is an effective and safe treatment for upper extremity motor dysfunction after a stroke. For the functional restoration of the upper extremities, noninvasive integrated therapy and lower-frequency VNS may be more effective. In the future, further high-quality studies with larger study populations, more comprehensive indicators, and thorough data are required to advance the clinical application of VNS.
Systematic review registration: https://www.crd.york.ac.uk/prospero/, identifier: CRD42023399820.
Stroke is a severe health risk that represents a great burden to society and healthcare systems. Approximately 60% of the individuals who experience a stroke have long-lasting upper extremity dysfunction that hinders their activities of daily living and compromises their mental wellbeing (1–4). It has been predicted that, by 2050, there will be ~200 million stroke victims worldwide. Hence, it is paramount that more effective treatment strategies are developed (5). Alternative approaches are required because standard rehabilitation therapy may not successfully restore function after a stroke (6). Ideally, future therapies for stroke should combine thrombolysis with antithrombotic, neuroprotective, and neuroplasticity-enhancing interventions (7). One possible treatment for enhancing neuroplasticity of the upper limb following a stroke is vagus nerve stimulation (VNS). VNS is an adjunctive therapy approved by the Food and Drug Administration for the treatment of partial epilepsy, depression, and primary headache disorders (8). VNS refers to any method that stimulates the vagus nerve. The methods are divided into invasive VNS (iVNS) and transcutaneous VNS (tVNS). Furthermore, tVNS can be further divided into transcutaneous cervical VNS (tcVNS) and transcutaneous auricular VNS (taVNS) (9, 10). The number of publications related to VNS has tripled in the last 10 years. In particular, the number of published studies has exponentially increased over the last few years (11). Numerous preclinical studies have documented positive poststroke recovery following a combination of VNS and physical therapy. Numerous clinical studies have also produced encouraging findings (12).
Animal studies involving rats with cerebral ischemia have suggested that VNS combined with rehabilitation can significantly alleviate neurological impairment, reduce cerebral infarction volume, and improve forelimb function, as well as memory and cognition (13–17). The mechanism of action may include enhancing angiogenesis, controlling blood-brain barrier permeability, minimizing the spread of depolarization, preventing neuroinflammation, and facilitating poststroke axonal plasticity (18–22). An increasing number of clinical trials have also demonstrated the beneficial effects of VNS combined with rehabilitation for patients with stroke. However, most clinical trials have been limited by small sample sizes (23, 24). Several meta-analyses have concluded based on the available clinical trials that VNS may improve the recovery of upper limb function following a stroke (25–32). Additionally, some researchers have reported that tVNS may be more effective than iVNS (26, 28, 29, 31, 32). These published meta-analyses had a risk of publication bias due to the absence of funnel plots. Many of these meta-analyses have also highlighted the need for more welldesigned studies to verify the long-term efficacy of VNS, including the stimulation settings, prognostic scores, integrated rehabilitation training methods, adverse events (AEs), and other factors. The analyses conducted by Liu et al. (28) and Zhao et al. (32) also restricted the studies to specific languages. In light of the potential clinical significance of VNS and the currently weak evidence from quantitative analyses, this study aimed to conduct a comprehensive and up-to-date meta-analysis on VNS for upper limb dysfunction after a stroke, including the efficacy, safety, and long-term implications.
This study was a systematic review of previously published studies. Therefore, both patient consent and ethical approval were not required (33). The meta-analysis was conducted according to the Preferred Reporting Items for Systematic Reviews and Meta-Analyses guidelines and previously published protocols (34). The detailed protocol used to perform this systematic evaluation has been registered in PROSPERO (reference number: CRD42023399820).
The following databases were searched from the time of inception to December 2022: PubMed, Scopus, Embase, Web of Science, Cochrane Library, China National Knowledge Infrastructure, Wanfang, China Science and Technology Journal Database, and China Biology Medicine. The following search terms were used: (Stroke OR Cerebrovascular Accident OR CVA OR Cerebrovascular Apoplexy OR Vascular Accident OR Cerebral Stroke) AND (VNS OR Vagal Nerve Stimulation OR Vagal Nerve Stimulation). To identify further relevant articles, we traced the references included in the identified articles and conducted manual searches.
We searched for studies without language restrictions. The inclusion criteria were as follows: (1) Studies with patients with stroke and upper limb disorders; (2) the experimental group received VNS combined with other treatment approaches, and the control group received no VNS or sham stimulation combined with other treatment approaches. The other treatment approaches were the same in the experimental and control groups; (3) studies that were randomized controlled trials (RCT); and (4) the study included at least one of the following pretraining or follow-up outcome indicators: motor function, quality of life, activities of daily living (ADL), and/or AEs. The exclusion criteria were as follows: (1) Patients who experienced a non-primary stroke; (2) relevant data required for meta-analysis were not available; (3) the full text of the paper could not be obtained even after contacting the corresponding author.
The following data were gathered: author, location, publication year, disease course, disease type, the number of samples, intervention modes, the type of combined therapy, stimulus parameters, stimulus time, evaluation time, and outcomes. Two researchers independently screened the papers and extracted and crosschecked the data. Any dispute was resolved through discussion or negotiation with an independent researcher. We used the Java program GetData Graph Digitizer (http://www.getdata-graph-digitizer.com) to determine the numerical values from the plotted data if no values were originally provided. If there were no pre- and post-treatment differences in the included randomized controlled trials or post-treatment data, the corresponding author was contacted to obtain the missing details. Where necessary, we manually calculated the mean and standard deviation (SD) using the Cochrane Handbook formulas based on the available baseline and outcome data.
The outcome measures in this study were the efficacy and safety of VNS for the treatment of upper limb dysfunction after a stroke. Efficacy referred to the improvement of upper limb motor function and its impact on patient prognosis, while safety included the number of AEs and serious AEs (SAEs). The main indicator of upper limb motor function was the Fugl–Meyer Assessment for Upper Extremity (FMA-UE) score after VNS treatment at different frequencies combined with different treatment methods. The secondary indicators were the Wolf Motor Function Test (WMFT) score and FMA-UE effective rate. The prognosis was defined as improvement in ADL, quality of life, and mental wellbeing (e.g., mood).
The quality of all the articles was assessed independently by the two researchers who reviewed the findings. When a disagreement occurred, a third researcher was consulted for arbitration. The quality of the included RCTs was assessed using the Cochrane risk-of-bias tool (35). This involved evaluating seven different types of biases: attrition bias (incomplete outcome data), selection bias (unbiased sequence generation and allocation concealment), reporting bias (selective result reporting), blinding bias (unbiased performance and detection), and other bias. The risk of bias for each item was rated as low, unclear, or high.
The evaluation index data of the included studies were processed using RevMan software (version 5.3; Cochrane Collaboration, Software Update, Oxford, UK). The mean difference (MD) and 95% confidence interval (CI) were used to express continuous variables. For continuous variables with different units, the standardized MD (SMD) and 95% CI were applied to exclude the influence of units (36). Dichotomous variables were expressed as risk ratios using the Mantel–Haenszel method. The degree of study heterogeneity was represented using I2. A random-effects model was applied if I2 exceeded 50%. Otherwise, a fixed-effects model was used. Values >75% indicated high heterogeneity. Sensitivity and subgroup analyses were utilized to pinpoint the source of heterogeneity and also to examine the stability of the results, as well as compare the effects of different clinical factors. Descriptive analysis was performed if the cause of the heterogeneity was not identified. Stata software (version 16.0, http://www.stata.com) was used to construct a funnel plot to determine publication bias. Finally, we used GRADE profiler software (https://gradeprofifiler.software.informer.com/) to evaluate the quality of the evidence based on the analyzed outcome indicators of the present study.
The flowchart of the search and article selection process is shown in Figure 1. Initially, 1,335 articles were identified as potentially relevant. Ten articles (three written in Chinese and seven in English) (23, 24, 37–44), involving 335 participants, were finally included in this study. The basic details of the 10 included articles are shown in Table 1. All 10 articles were RCTs with an experimental group and a control group. The experimental groups underwent VNS with different stimulus parameters combined with other treatment approaches. Eight of the 10 studies utilized a placebo in the control group, while the other two were blank controls (no VNS) (Table 1). The combined treatment methods employed with VNS included upper limb therapy alone in six studies and comprehensive therapy in three studies. The interventions lasted for 2–4 weeks, and evaluations were performed 1–90 days after the treatment. Wu et al. (41) and Dawson et al. (23) did not blind the outcome assessments (detection bias), but the remaining eight studies were blinded (Figure 2).
Nine articles reported the FMA-UE scores a day after treatment (immediate effect). The available results indicated that the VNS group significantly improved upper limb motor function compared with the control group (MD = 2.84, 95% CI = 1.78–3.91, I2 = 62%, p < 0.00001; Figure 3) (23, 24, 37, 39–44). Three articles reported FMA-UE scores at 30- and 90-day posttreatment (long-term effects) (24, 41, 43). The pooled findings indicated that the scores in the VNS group were significantly higher than those in the control group at 30- (MD = 4.20, 95% CI = 2.90–5.50) and 90-day posttreatment (MD = 3.27, 95% CI = 1.67–4.87) (Figure 3). Based on these results, VNS demonstrated immediate and long-term effects on upper limb motor function.
A subgroup analysis was conducted to compare various aspects that may influence efficacy, such as disease stage, combination protocol, stimulation modality, and other stimulation parameters. The results revealed that tVNS (MD = 2.87, 95% CI = 1.78–3.91, I2 = 62%, p < 0.00001) may be superior to iVNS (MD = 3.56, 95% CI = 1.99–5.13, I2 = 77%, p < 0.0001, Figure 4A). Moreover, VNS in conjunction with combination therapy (MD = 2.87, 95% CI = 1.78–3.91, I2 = 62%, p < 0.00001) outperforms VNS in conjunction with upper extremity training alone (MD = 2.24, 95% CI = 0.55–3.93, I2 = 48%, p = 0.009, Figure 4B). Furthermore, lower frequency VNS (< 25 Hz) (MD = 3.39, 95% CI = 2.06–4.73, I2 = 65%, p < 0.00001) may be superior to higher frequency VNS (≥25 Hz) (MD = 2.29, 95% CI = 0.27–4.32, I2 = 58%, p = 0.03) (Figure 4C).
Figure 4. (A) Subgroup analysis of different modes of VNS in FMA-UE scores. (B) Subgroup analysis of VNS combined with different treatments. tVNS, transcutaneous vagus nerve stimulation; iVNS, invasive vagus nerve stimulation. (C) Subgroup analysis of VNS with different frequencies in FMA-UE scores.
FMA-UE efficiency was defined as an increase in the FMA-UE score by >6. Three articles (23, 24, 39) reported the FMA-UE scores at 1 day posttreatment (immediate effect), and two articles (39, 43) reported the FMA-UE scores at 90 days posttreatment (long-term effect) (Figure 5). Pooled analyses indicated that both the immediate (MD = 4.06, 95% CI = 1.18–13.89) and long-term (MD = 3.37, 95% CI = 1.56–7.28) effects had little heterogeneity. The fixed-effects model was employed, and the results indicated that FMA-UE efficiency was higher in the experimental group than that in the control group.
Three articles (39, 41, 43) reported the immediate effect as indicated by the WMFT score. The pooled analysis indicated that the WMFT score was higher in the experimental group than that in the control group (MD = 0.37, 95% CI = 0.06–0.81, I2 = 89%) (Figure 6A). A subgroup analysis was conducted due to high heterogeneity. The results showed that lower frequency VNS (< 25 Hz) (MD = 3.59, 95% CI = 1.97–5.51) was more effective than higher frequency VNS (≥25 Hz) (MD = 0.17, 95% CI = 0.07–0.27) (Figure 6B). Three articles (39, 43, 44) reported the long-term effect as indicated by the WMFT score in the absence of heterogeneity (i.e., I2 = 0) and found that the VNS group had significantly higher scores than the control group at 90-day posttreatment (MD = 0.30, 95% CI = 0.19–0.47) (Figure 6A).
Figure 6. (A) Forest plot of WMFT. (B) Subgroup analysis of VNS with different frequencies in WMFT scores.
To determine the prognosis, we examined the ADL, quality of life, and depression status scores. Four studies (37, 38, 40, 41) included indicators that assessed ADL, including BI, MBI, and FIM. After aggregation, it was found that I2 was 88% (Figure 7A), indicating excessive heterogeneity. The sensitivity analysis indicated that after excluding the study of Zhang et al. (37), the heterogeneity decreased to 0%. Thus, this indicator was excluded from the analysis. Subsequently, the reanalyzed results indicated that the ADL score was significantly higher after VNS (SMD = 1.50, 95% CI = 1.10–1.90, I2 = 0%, p < 0.00001) (Figure 7B). Two articles (42, 43) included life quality assessment scales, including the Stroke-Specific Quality of Life Scale and EuroQol five-dimensional questionnaire. No significant difference was found between the experimental and control groups after the summary analysis (SMD = 0.10; 95% CI = −0.2 to 0.41, I2 = 0%; p = 0.51) (Figure 7C). Two articles included a scale for assessing depression status, namely, the Beck Depression Inventory (BDI) and the depression domain of the Hospital Anxiety and Depression Scale (HADS). Li et al. (42) found that the HADS score decreased in the experimental group after VNS, while Dawson et al. (43) found a decrease in the BDI score 1 day (−1.6 [SD = 6.2] vs. 0.8 [SD = 5.0]) and 90 days posttreatment (−1.8 [SD = 5.6] vs. 0.2 [SD = 4.1]) compared with that of the control group.
Figure 7. (A) Forest map of ADL score before elimination. (B) Forest map of ADL score after elimination. (C) Forest map of life quality.
The AE incidence was reported in seven papers (23, 24, 40–44), and the SAE incidence was reported in three papers (23, 39, 43). As shown in the pooled analysis in Figure 8, there was no significant difference between the experimental and control groups in the incidence of AEs (p = 0.25, Figure 8A) or SAEs (p = 0.26) (Figure 8B). These results indicate that VNS combined with rehabilitation therapy is safe for the treatment of upper limb dysfunction after stroke.
Figure 8. (A) Forest plot for the meta-analysis of atrial fibrillations (AEs). (B) Forest plot for the meta-analysis of several atrial fibrillations (SAEs).
Quantitative evaluation of the FMA-UE scores using Egger's test indicated no bias (p = 0.266). The publication bias chart is shown in Figure 9. Sensitivity analysis was performed by excluding publications one by one. The heterogeneity decreased significantly after removing the study by Chang et al. (44). The bias was presumably related to the intervention duration, which differed from those of other studies. However, Chang et al.'s study did not affect the pooled results or the subgroup analysis results, which were stable.
The key outcome indicators (FMA-UE score, ADL score, and the number of AEs and SAEs) of the 10 included studies were evaluated using the GRADE software. The GRADE system evaluates five factors: risk of bias, inconsistency, imprecision, indirectness, and publication bias, and divides the quality of the evidence into four categories: high, medium, low, and very poor. The results according to the GRADE system indicated that the evidence was of high quality for the FMA-UE score, medium quality for the number of AEs and ADL, and low quality for the number of SAEs.
This study included 10 RCTs, which is a larger sample size than the previous meta-analyses that examined the use of VNS in stroke treatment. Publication bias, stimulation parameters, combination regimens, long-term efficacy, and prognosis were integrated and discussed in this study. The results and differences were as follows: (1) Regarding motor function, VNS exerted immediate and long-term effects when combined with comprehensive treatment as indicated by the FMA-UE score, WMFT score, and FMA-UE efficiency, which was consistent with the findings of other meta-analyses. However, the results showed that the FMA-UE pre- and post-treatment difference after 90 days of treatment was lower than that after 1 day of treatment, whereas the WMFT score was the opposite. Therefore, we could only confirm that VNS combined with rehabilitation therapy had a long-term effect and were unable to pinpoint the specific long-term changes. To further investigate this aspect, further clinical studies are warranted. The results of the subgroup analyses suggest that the tVNS mode combined combination therapy and lower frequency of VNS resulted in better outcomes. (2) Prognosis in terms of quality of life was not significant in this study. This result is consistent with the findings of previous studies. In contrast, the increased ability to perform ADL and the remission of depression contradicted the results of Gao et al. (26). For depression, the same two articles were included. The present study used a qualitative analysis considering its excessive heterogeneity. Hence, the effect of VNS on depression needs to be further demonstrated by including future studies. Regarding ADL, Gao et al. (26) included two articles that used the Stroke Impact Scale (hand function) only. In this study, we included more articles and assessed additional indicators, resulting in less heterogeneity. Our results indicated that VNS improved ADL. (3) The present study quantified the occurrence of AEs and SAEs separately to evaluate safety. This has not been considered in previous studies. Based on our results, VNS used in stroke treatment is safe.
Although supported by numerous preclinical and clinical trials, treatment with VNS-targeted plasticity remains challenging due to various factors (6). In the present study, a subgroup analysis of multiple factors was performed. First, we resulted that tVNS was superior to iVNS, which is consistent with the findings of earlier meta-analyses. In this study, all tVNS were taVNS, as tcVNS clinical trials were sparse. Hence, in the future, further investigation of tcVNS is necessary. The results stayed the same after removing the 2016 article by Dawson et al. (23) to eliminate the placebo effect. Second, we conducted a subgroup analysis of the combined VNS protocols, an important factor overlooked in previous meta-analyses, and found that VNS combined with comprehensive training had a better effect on upper limb function than upper limb training alone. We hypothesized that there is a mutually beneficial relationship between different neuroprotective and neuroplastic treatment modalities, suggesting that VNS is a suitable adjunctive therapy for stroke treatment. Furthermore, VNS combined with comprehensive training is recommended in clinical practice. Finally, the ideal parameters for optimizing VNS have long been a highly controversial issue. Optimizing these parameters is crucial for efficacy comparisons (12). One of the major limitations of VNS is its large parameter variations. Variable pulse widths, frequencies, and stimulation currents make it difficult to determine what parameters are more important and which are the best matches or combinations (45). Currently, of the many different parameters, the current intensity is the most studied. Several studies have shown that intensity and plasticity have an inverted U-shape relationship, with medium intensity being superior, and that intensity is inversely proportional to pulse width, with low intensity compensated by wide pulses (46–49). Compared with intensity, frequency is less affected by individualization; therefore, it is easier to optimize, although little research has been conducted to date (10). Buell et al. (50) in their biological experiments verified that frequency and plasticity have an inverted U-shape relationship and that frequencies of ~30 Hz are more effective. Currently, VNS at 20–30 Hz is commonly used in clinical practice. Results from our subgroup analyses demonstrated that VNS at lower frequencies (< 25 Hz) may be more effective than higher frequencies (≥25 Hz), which complements the findings of Buell et al. (50). Additionally, in a study that utilized taVNS for migraines (51), 1 Hz was shown to be more effective than 25 Hz (51). Taken together, these findings imply that lower frequencies may produce superior clinical outcomes. However, owing to diverse clinical applications and the limited number of frequency studies, we cannot exclude the influence of other parameters or factors on frequency. Interestingly, a study (52) has suggested that lower frequencies in tcVNS can be compensated by higher intensities. However, this is inconsistent with our subgroup analysis results on iVNS and taVNS. In the future, more vigilant investigations, including basic experiments and clinical trials, are warranted to verify and validate current findings. In summary, each of these parameters may contribute to the therapeutic effect, and one or more parameters may be altered according to the optimization of the clinical effects in individual patients.
Of note, previous studies on VNS have consistently suggested that the results obtained are influenced by individual differences that inhibit the optimization of stimulation parameters. Theoretically, advanced age is associated with reduced neuroplasticity. Moreover, stroke is dichotomized according to sex and different underlying diseases or drugs may change the effects of VNS through neuroregulatory pathway activation (4). However, preclinical trials have demonstrated that age does not limit the use of VNS in stroke treatment (16); Dawson et al. (53) have conducted further detailed subgroup analyses of their patients after their clinical trials in 2021 and found that differences among different subgroups, including age, sex, residence location, stroke severity, stroke duration, side of the palsy, and cortical involvement, did not affect patient outcomes. An exploratory study by David et al. (54) examined various predictors in combination with two clinical trials and led to the hypothesis that VNS provides additional benefits for patients with more severe upper extremity disability at baseline and unfavorable imaging outcomes (e.g., higher cerebrospinal fluid volume), with no other findings inconsistent with previous speculations. These studies were restricted to specific baseline ranges, and the between-group differences and sample sizes were small. As such, further investigations, especially clinical studies, are needed to justify the above hypothesis and optimize stimulation parameters. Other studies have suggested that relationships exist between stimulation parameters and side effects that may also influence stimulation parameter optimization. These relationships require further clarification. Hence, further studies are needed (55).
This study had some limitations. First, VNS and stroke are both intrinsically heterogeneous situations. Therefore, the variables considered in the subgroup analyses were not entirely homogeneous, which could have interfered with the results. Second, only a few prognostic studies have been published, leading to insufficient evidence for drawing definitive conclusions. Future studies should include a greater number of welldesigned RCTs with high-quality samples. Third, because we did not analyze any objective indicators, future studies should consider the evaluation of neuroimaging and neurophysiological technologies.
VNS for poststroke upper extremity dysfunction is effective and safe in the long term. It improves upper extremity motor function, increases daily activity capacity, and improves mental state. The results of the subgroup analyses showed that tVNS, combined with integrated rehabilitation and a lower frequency of VNS are superior for the management of poststroke upper extremity function. This study had some limitations that need a comprehensive index and uniform stimulation parameters to further explore the use of VNS in patients with upper limb dysfunction following a stroke.
The original contributions presented in the study are included in the article/supplementary material, further inquiries can be directed to the corresponding author.
QD and YFL accepted accountability for their analysis's accuracy and the data's reliability. The study's inception, design, and manuscript writing were all assisted by XW and WZ. The tables and figures were created by TL and WL. The literature search, data extraction and analysis, data interpretation, and quality assessment were significantly aided by YKL and JY. All authors reviewed and approved the article's submission.
This paper was funded by Henan Province Medical Science and Technology Research Program (SBGJ20210203).
We would like to express our heartfelt gratitude to WZ and WL for their valuable suggestions on the meta-analysis. We also hope to express our appreciation to the experts for editing and proofreading this manuscript.
The authors declare that the research was conducted in the absence of any commercial or financial relationships that could be construed as a potential conflict of interest.
All claims expressed in this article are solely those of the authors and do not necessarily represent those of their affiliated organizations, or those of the publisher, the editors and the reviewers. Any product that may be evaluated in this article, or claim that may be made by its manufacturer, is not guaranteed or endorsed by the publisher.
1. Chun HY, Ford A, Kutlubaev MA, Almeida OP, Mead GE. Depression, anxiety, and suicide after stroke: a narrative review of the best available evidence. Stroke. (2022) 53:1402–10. doi: 10.1161/STROKEAHA.121.035499
2. Collaborators GBDS. Global, regional, and national burden of stroke and its risk factors, 1990–2019: a systematic analysis for the global burden of disease study 2019. Lancet Neurol. (2021) 20:795–820. doi: 10.1016/S1474-4422(21)00252-0
3. Crichton SL, Bray BD, McKevitt C, Rudd AG, Wolfe CD. Patient outcomes up to 15 years after stroke: survival, disability, quality of life, cognition and mental health. J Neurol Neurosurg Psychiatry. (2016) 87:1091–8. doi: 10.1136/jnnp-2016-313361
4. Engineer ND, Kimberley TJ, Prudente CN, Dawson J, Tarver WB, Hays SA. Targeted vagus nerve stimulation for rehabilitation after stroke. Front Neurosci. (2019) 13:280. doi: 10.3389/fnins.2019.00280
5. Brainin M, Feigin VL, Norrving B, Martins SCO, Hankey GJ, Hachinski V, et al. Global prevention of stroke and dementia: the wso declaration. Lancet Neurol. (2020) 19:487–8. doi: 10.1016/S1474-4422(20)30141-1
6. Li L, Wang D, Pan H, Huang L, Sun X, He C, et al. Non-invasive vagus nerve stimulation in cerebral stroke: current status and future perspectives. Front Neurosci. (2022) 16:820665. doi: 10.3389/fnins.2022.820665
7. Moretti A, Ferrari F, Villa RF. Neuroprotection for ischaemic stroke: current status and challenges. Pharmacol Ther. (2015) 146:23–34. doi: 10.1016/j.pharmthera.2014.09.003
8. Ma J, Qiao P, Li Q, Wang Y, Zhang L, Yan LJ, et al. Vagus nerve stimulation as a promising adjunctive treatment for ischemic stroke. Neurochem Int. (2019) 131:104539. doi: 10.1016/j.neuint.2019.104539
9. Howland RH. Vagus nerve stimulation. Curr Behav Neurosci Rep. (2014) 1:64–73. doi: 10.1007/s40473-014-0010-5
10. Farmer AD, Strzelczyk A, Finisguerra A, Gourine AV, Gharabaghi A, Hasan A, et al. International consensus based review and recommendations for minimum reporting standards in research on transcutaneous vagus nerve stimulation (Version 2020). Front Hum Neurosci. (2020) 14:215. doi: 10.3389/fnhum.2020.568051
11. Li R, Hu H, Luo N, Fang J. Bibliometric analysis of publication trends and research hotspots in vagus nerve stimulation: a 20-year panorama. Front Neurol. (2022) 13:1045763. doi: 10.3389/fneur.2022.1045763
12. Cheng K, Wang Z, Bai J, Xiong J, Chen J, Ni J. Research advances in the application of vagus nerve electrical stimulation in ischemic stroke. Front Neurosci. (2022) 16:1043446. doi: 10.3389/fnins.2022.1043446
13. Sun Z, Baker W, Hiraki T, Greenberg JH. The effect of right vagus nerve stimulation on focal cerebral ischemia: an experimental study in the rat. Brain Stimul. (2012) 5:1–10. doi: 10.1016/j.brs.2011.01.009
14. Khodaparast N, Kilgard MP, Casavant R, Ruiz A, Qureshi I, Ganzer PD, et al. Vagus nerve stimulation during rehabilitative training improves forelimb recovery after chronic ischemic stroke in rats. Neurorehabil Neural Repair. (2016) 30:676–84. doi: 10.1177/1545968315616494
15. Khodaparast N, Hays SA, Sloan AM, Hulsey DR, Ruiz A, Pantoja M, et al. Vagus nerve stimulation during rehabilitative training improves forelimb strength following ischemic stroke. Neurobiol Dis. (2013) 60:80–8. doi: 10.1016/j.nbd.2013.08.002
16. Hays SA, Ruiz A, Bethea T, Khodaparast N, Carmel JB, Rennaker RL, et al. Vagus nerve stimulation during rehabilitative training enhances recovery of forelimb function after ischemic stroke in aged rats. Neurobiol Aging. (2016) 43:111–8. doi: 10.1016/j.neurobiolaging.2016.03.030
17. Hays SA, Khodaparast N, Hulsey DR, Ruiz A, Sloan AM, Rennaker RL, et al. Vagus nerve stimulation during rehabilitative training improves functional recovery after intracerebral hemorrhage. Stroke. (2014) 45:3097–100. doi: 10.1161/STROKEAHA.114.006654
18. Ay I, Nasser R, Simon B, Ay H. Transcutaneous cervical vagus nerve stimulation ameliorates acute ischemic injury in rats. Brain Stimul. (2016) 9:166–73. doi: 10.1016/j.brs.2015.11.008
19. Du L, Yang Z, Sheng H, Liu M, Sun Q. Effects of long-term vagus nerve electrical stimulation therapy on acute cerebral infarction and neurological function recovery in post mcao mice. Oxid Med Cell Longev. (2022) 2022:8131391. doi: 10.1155/2022/8131391
20. Lindemann J, Rakers C, Matuskova H, Simon BJ, Kinfe T, Petzold GC. Vagus nerve stimulation reduces spreading depolarization burden and cortical infarct volume in a rat model of stroke. PLoS One. (2020) 15:e0236444. doi: 10.1371/journal.pone.0236444
21. Meyers EC, Solorzano BR, James J, Ganzer PD, Lai ES, Rennaker RL, et al. Vagus nerve stimulation enhances stable plasticity and generalization of stroke recovery. Stroke. (2018) 49:710–7. doi: 10.1161/STROKEAHA.117.019202
22. Yang Y, Yang LY, Orban L, Cuylear D, Thompson J, Simon B, et al. Non-invasive vagus nerve stimulation reduces blood-brain barrier disruption in a rat model of ischemic stroke. Brain Stimul. (2018) 11:689–98. doi: 10.1016/j.brs.2018.01.034
23. Dawson J, Pierce D, Dixit A, Kimberley TJ, Robertson M, Tarver B, et al. Safety, feasibility, and efficacy of vagus nerve stimulation paired with upper-limb rehabilitation after ischemic stroke. Stroke. (2016) 47:143–50. doi: 10.1161/STROKEAHA.115.010477
24. Capone F, Miccinilli S, Pellegrino G, Zollo L, Simonetti D, Bressi F, et al. Transcutaneous vagus nerve stimulation combined with robotic rehabilitation improves upper limb function after stroke. Neural Plast. (2017) 2017:6. doi: 10.1155/2017/7876507
25. Ananda R, Roslan MHB, Wong LL, Botross NP, Ngim CF, Mariapun J. Efficacy and safety of vagus nerve stimulation in stroke rehabilitation: a systematic review and meta-analysis. Cerebrovasc Dis. (2022). doi: 10.1159/000526470
26. Gao Y, Zhu Y, Lu X, Wang N, Zhu S, Gong J, et al. Vagus nerve stimulation paired with rehabilitation for motor function, mental health and activities of daily living after stroke: a systematic review and meta-analysis. J Neurol Neurosurg Psychiatry. (2022) 22:2975. doi: 10.1136/jnnp-2022-329275
27. Li H, Yan L, Qian YL. Transcutaneous vagus nerve stimulation combined with rehabilitation training in the intervention of upper limb movement disorders after stroke: a systematic review. Neuropsychiatr Dis Treat. (2022) 18:2095–106. doi: 10.2147/NDT.S376399
28. Liu YL, Zhang LP, Zhang X, Ma JX, Jia GW. Effect of combined vagus nerve stimulation on recovery of upper extremity function in patients with stroke: a systematic review and meta-analysis. J Stroke Cerebrovasc Dis. (2022) 31:11. doi: 10.1016/j.jstrokecerebrovasdis.2022.106390
29. Ramos-Castaneda JA, Barreto-Cortes CF, Losada-Floriano D, Sanabria-Barrera SM, Silva-Sieger FA, Garcia RG. Efficacy and safety of vagus nerve stimulation on upper limb motor recovery after stroke. A Systematic Review and Meta-Analysis Front Neurol. (2022) 13:12. doi: 10.3389/fneur.2022.889953
30. Wei J, Zhang C, Wang JX, Sun FH, Xie YJ, Ou X, et al. The effect of Vns on the rehabilitation of stroke: a meta-analysis of randomized controlled studies. J Clin Neurosci. (2020) 81:421–5. doi: 10.1016/j.jocn.2020.09.022
31. Xie YL, Wang S, Wu Q, Chen X. Vagus nerve stimulation for upper limb motor impairment after ischemic stroke a meta-analysis. Medicine (Baltimore). (2021) 100:10. doi: 10.1097/MD.0000000000027871
32. Zhao KH, Yang JE, Huang JP, Zhao ZQ, Qu Y. Effect of vagus nerve stimulation paired with rehabilitation for upper limb function improvement after stroke: a systematic review and meta-analysis of randomized controlled trials. Int J Rehabil Res. (2022) 45:99–108. doi: 10.1097/MRR.0000000000000509
33. Higgins JP, Thompson SG. Quantifying heterogeneity in a meta-analysis. Stat Med. (2002) 21:1539–58. doi: 10.1002/sim.1186
34. Moher D, Liberati A, Tetzlaff J, Altman DG. Preferred reporting items for systematic reviews and meta-analyses: the prisma statement. Annals of internal medicine. (2009) 151:264–9. doi: 10.7326/0003-4819-151-4-200908180-00135
35. Sterne JAC, Savović J, Page MJ, Elbers RG, Blencowe NS, Boutron I, et al. Rob 2: A revised tool for assessing risk of bias in randomised trials. Bmj. (2019) 366:l4898. doi: 10.1136/bmj.l4898
36. Murad MH, Wang Z, Chu H, Lin L. When continuous outcomes are measured using different scales: guide for meta-analysis and interpretation. BMJ. (2019) 364:k4817. doi: 10.1136/bmj.k4817
37. Liping Z MY, Sanrong W, Botao T, Lang J, Gongwei J. Effects of transcutaneous vagus nerve stimulation on the recovery of upper limb motor function in patients with ischemic stroke. Chin J Rehabil Med. (2020) 35:1316–20. doi: 10.3969/j.issn.1001-1242.2020.11.007
38. Zhenguo H LS, Wen Y, Qiaoli D. Effect of vagus nerve stimulation and chinese medicine comprehensive physiotherapy on the recovery of limb function in patients with hemiplegia after stroke. Chinese J Integrat Med Cardio-Cerebrovascul Dis. (2018) 16:1974–6.
39. Kimberley TJ, Pierce D, Prudente CN, Francisco GE, Yozbatiran N, Smith P, et al. Vagus nerve stimulation paired with upper limb rehabilitation after chronic stroke: a blinded randomized pilot study. Stroke. (2018) 49:2789–92. doi: 10.1161/STROKEAHA.118.022279
40. Xing W. Effect of trausauricular vagus nerve stimulation combined with limb training on upper limb motor function and brain plasticity in stroke patient. [Master's degree] [China(Tianjin)]Tianjin Institute of Physical Education (2020).
41. Wu DD, Ma JX, Zhang LP, Wang SR, Tan BT, Jia GW. Effect and safety of transcutaneous auricular vagus nerve stimulation on recovery of upper limb motor function in subacute ischemic stroke patients: a randomized pilot study. Neural Plast. (2020) 2020:9. doi: 10.1155/2020/8841752
42. Li JN, Xie CC Li CQ, Zhang GF, Tang H, Jin CN, et al. Efficacy and safety of transcutaneous auricular vagus nerve stimulation combined with conventional rehabilitation training in acute stroke patients: a randomized controlled trial conducted for 1 year involving 60 patients. Neural Regen Res. (2022) 17:1809–13. doi: 10.4103/1673-5374.332155
43. Dawson J, Liu CY, Francisco GE, Cramer SC, Wolf SL, Dixit A, et al. Vagus nerve stimulation paired with rehabilitation for upper limb motor function after ischaemic stroke (vns-rehab): a randomised, blinded, pivotal, device trial. Lancet. (2021) 397:1545–53. doi: 10.1016/S0140-6736(21)00475-X
44. Chang JL, Coggins AN, Saul M, Paget-Blanc A, Straka M, Wright J, et al. Transcutaneous auricular vagus nerve stimulation (tavns) delivered during upper limb interactive robotic training demonstrates novel antagonist control for reaching movements following stroke. Front Neurosci. (2021) 15:11. doi: 10.3389/fnins.2021.767302
45. Badran BW, Yu AB, Adair D, Mappin G, DeVries WH, Jenkins DD, et al. Laboratory administration of transcutaneous auricular vagus nerve stimulation (Tavns): technique, targeting, and considerations. J Vis Exp. (2019) doi: 10.3791/58984
46. Borland MS, Engineer CT, Vrana WA, Moreno NA, Engineer ND, Vanneste S, et al. The interval between Vns-Tone pairings determines the extent of cortical map plasticity. Neuroscience. (2018) 369:76–86. doi: 10.1016/j.neuroscience.2017.11.004
47. Hulsey DR, Riley JR, Loerwald KW, Rennaker RL. 2nd, Kilgard MP, Hays SA. Parametric characterization of neural activity in the locus coeruleus in response to vagus nerve stimulation. Exp Neurol. (2017) 289:21–30. doi: 10.1016/j.expneurol.2016.12.005
48. Morrison RA, Hulsey DR, Adcock KS, Rennaker RL. 2nd, Kilgard MP, Hays SA. Vagus nerve stimulation intensity influences motor cortex plasticity. Brain Stimul. (2019) 12:256–62. doi: 10.1016/j.brs.2018.10.017
49. Pruitt DT, Danaphongse TT, Lutchman M, Patel N, Reddy P, Wang V, et al. Optimizing dosing of vagus nerve stimulation for stroke recovery. Transl Stroke Res. (2021) 12:65–71. doi: 10.1007/s12975-020-00829-6
50. Buell EP, Loerwald KW, Engineer CT, Borland MS, Buell JM, Kelly CA, et al. Cortical map plasticity as a function of vagus nerve stimulation rate. Brain Stimul. (2018) 11:1218–24. doi: 10.1016/j.brs.2018.07.045
51. Andreas Straube1* JE, 3, Eren1 O, Blum B, Ruscheweyh R. Treatment of Chronic Migraine with Transcutaneous Stimulation of the Auricular Branch of the Vagal Nerve (Auricular T-Vns): A Randomized, Monocentric Clinical Trial. (2015).
52. Loerwald KW, Borland MS, Rennaker RL, Hays SA, Kilgard MP. The interaction of pulse width and current intensity on the extent of cortical plasticity evoked by vagus nerve stimulation. Brain Stimul. (2018) 11:271–7. doi: 10.1016/j.brs.2017.11.007
53. Dawson J, Liu C, Francisco GE, Cramer SC, Wolf SL, Dixit A, et al. Vagus nerve stimulation paired with rehabilitation for upper limb motor function after ischaemic stroke: sub-group analysis of the randomised, blinded, pivotal, vns-rehab device trial. Stroke. (2022) 53:2. doi: 10.1161/str.53.suppl_1.1
54. Dickie DA, Kimberley TJ, Pierce D, Engineer N, Tarver WB, Dawson J. An exploratory study of predictors of response to vagus nerve stimulation paired with upper-limb rehabilitation after ischemic stroke. Sci Rep. (2019) 9:15902. doi: 10.1038/s41598-019-52092-x
Keywords: vagus nerve stimulation (vns), stroke, rehabilitation, meta-analysis, upper limb dysfunction
Citation: Wang X, Ding Q, Li T, Li W, Yin J, Li Y, Li Y and Zhuang W (2023) Application of vagus nerve stimulation on the rehabilitation of upper limb dysfunction after stroke: a systematic review and meta-analysis. Front. Neurol. 14:1189034. doi: 10.3389/fneur.2023.1189034
Received: 18 March 2023; Accepted: 16 May 2023;
Published: 21 June 2023.
Edited by:
Jean-Pascal Lefaucheur, Univ Paris-Est Créteil, AP-HP, FranceReviewed by:
Robert Andrew Morrison, The University of Texas at Dallas, United StatesCopyright © 2023 Wang, Ding, Li, Li, Yin, Li, Li and Zhuang. This is an open-access article distributed under the terms of the Creative Commons Attribution License (CC BY). The use, distribution or reproduction in other forums is permitted, provided the original author(s) and the copyright owner(s) are credited and that the original publication in this journal is cited, in accordance with accepted academic practice. No use, distribution or reproduction is permitted which does not comply with these terms.
*Correspondence: Weisheng Zhuang, emh1YW5nMjAwNjI2MzRAMTYzLmNvbQ==
Disclaimer: All claims expressed in this article are solely those of the authors and do not necessarily represent those of their affiliated organizations, or those of the publisher, the editors and the reviewers. Any product that may be evaluated in this article or claim that may be made by its manufacturer is not guaranteed or endorsed by the publisher.
Research integrity at Frontiers
Learn more about the work of our research integrity team to safeguard the quality of each article we publish.