- 1Department of Cardiology, Dokkyo Medical University Nikkyo Medical Center, Mibu, Japan
- 2Department of Neurology, Dokkyo Medical University, Mibu, Japan
- 3Department of Pharmacology and Toxicology, Dokkyo Medical University School of Medicine, Mibu, Japan
- 4Stroke Center, Dokkyo Medical University, Mibu, Japan
Hyperuricemia often accompanies hypertension, diabetes, dyslipidemia, metabolic syndrome, and chronic renal disease; it is also closely related to cardiovascular disease. Moreover, several epidemiological studies have linked hyperuricemia and ischemic stroke. However, uric acid may also have neuroprotective effects because of its antioxidant properties. An association between low uric acid levels and neurodegenerative diseases has been suggested, which may be attributed to diminished neuroprotective effects as a result of reduced uric acid. This review will focus on the relationship between uric acid and various neurological diseases including stroke, neuroimmune diseases, and neurodegenerative diseases. When considering both the risk and pathogenesis of neurological diseases, it is important to consider the conflicting dual nature of uric acid as both a vascular risk factor and a neuroprotective factor. This dual nature of uric acid is important because it may help to elucidate the biological role of uric acid in various neurological diseases and provide new insights into the etiology and treatment of these diseases.
1. Introduction
Serum uric acid levels are determined by the balance between uric acid production and its excretion by the kidneys and intestinal tract. When this balance is disrupted, both hyperuricemia and hypouricemia can occur. The upper limit of the reference value of serum uric acid levels for both sexes and all ages is 7.0 mg/dL; hyperuricemia is defined as exceeding this level (1). Lifestyle-related diseases such as obesity, metabolic syndrome, cardiovascular disease, chronic kidney disease (CKD), gout, and urolithiasis are associated with hyperuricemia (2). Hyperuricemia is also strongly associated with hypertension, which is the greatest risk factor for stroke and may have an important impact on stroke incidence. In contrast to hyperuricemia, there is no clear definition of hypouricemia; however, a serum uric acid level of 2.0 mg/dL or less is generally used as the reference value (3). Hypouricemia is commonly observed in patients with several neurological diseases, including neurodegenerative diseases. Many epidemiological studies have indicated a link between hypouricemia and an increased risk of developing neurological diseases, suggesting that uric acid may exert neuroprotective effects as an antioxidant (4). Nevertheless, patients with neurological diseases are often undernourished, and hyponutrition may cause hypouricemia. Thus, the causal relationship between uric acid levels and neurological diseases needs to be better understood. When considering brain and neurological diseases, we must consider the conflicting dual nature of uric acid: It has both a vascular risk factor aspect and a neuroprotective aspect. Thus, the effects of uric acid in neurological diseases are likely to vary greatly between stroke, which is a vascular disease, and Parkinson's and Alzheimer's diseases, which are neurodegenerative diseases. Individuals with low uric acid levels are more likely to develop neurodegenerative diseases, whereas hyperuricemia is a risk factor for stroke. Together, these findings indicate that the impact of uric acid is likely to vary depending on the characteristics of the neurological disease (Figure 1). In the present review, we provide evidence for the involvement of uric acid in neurological diseases—including stroke, neuroimmune diseases, and neurodegenerative diseases—and suggest that maintaining appropriate uric acid levels will facilitate future management decisions for neurological diseases.
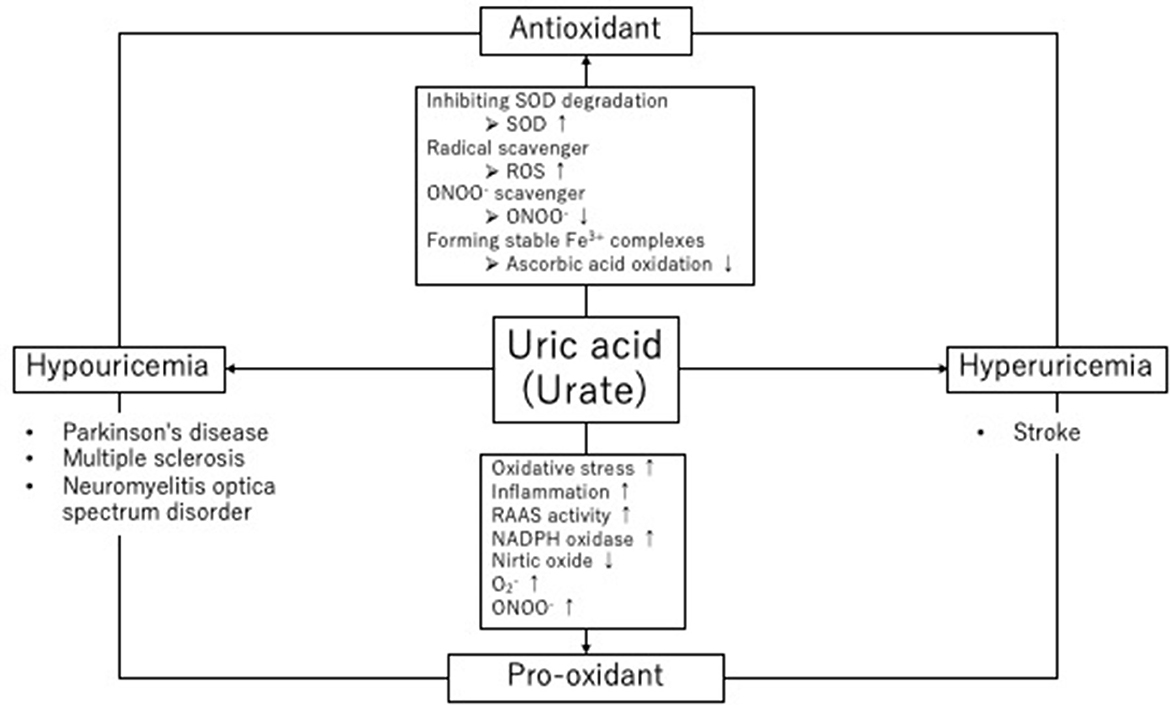
Figure 1. Role of uric acid in oxidative stress and its antioxidant properties. Uric acid has a dual nature, with both antioxidant and pro-oxidant effects, in neurological diseases. NO, nitric oxide; SOD, superoxide dismutase; ROS, radical oxygen species; ONOO−, peroxynitrite; RAAS, renin–angiotensin–aldosterone system; NADPH, nicotinamide adenine dinucleotide phosphate.
2. Methods
A literature search from August 2022 to November 2022 was performed for this narrative review using PubMed/Medline. English-language articles were identified using the term “uric acid” combined with the terms “neurological diseases,” “neurodegenerative diseases,” “stroke,” “dementia,” “Parkinson's disease,” “amyotrophic lateral sclerosis,” “neuromyelitis optica,” and “multiple sclerosis.”
3. Pathogenic role of uric acid in oxidative stress and its neuroprotective effects as an antioxidant
During evolution, mutations in genes involved in ascorbic acid synthesis resulted in their loss of function, and the antioxidant that replaced ascorbic acid was replaced by uric acid because of mutations in uricase. This replacement may be advantageous for survival because ascorbic acid is limited by its propensity for oxidation, producing oxygen radicals and associated mutagens (4). Uric acid and ascorbic acid are considered the most important water-soluble antioxidants, and the plasma uric acid level is approximately six times that of ascorbic acid (5). In addition, Yeum et al. measured antioxidant capacity and found that urate accounted for the majority of the total antioxidant capacity in plasma. This finding suggests that uric acid may be a major endogenous defense against oxidative damage in the body (6). Uric acid can scavenge free radicals under hydrophilic conditions and inhibits lipid peroxidation; however, it loses its antioxidant ability under hydrophobic conditions (7).
4. Uric acid regulation in the brain
Xanthine oxidoreductase metabolizes hypoxanthine to xanthine and then to uric acid. Uric acid excretion is performed by several uric acid transporters; approximately two-thirds is excreted by the kidneys, and one-third is excreted by the small intestine. In the brain, xanthine oxidoreductase levels are very low or absent. Thus, very little uric acid is produced in the brain. In addition, uric acid does not penetrate the blood–brain barrier or travel to the brain via any uric acid transporters, indicating that uric acid levels in the brain are much lower than those in the blood. Although there is a correlation between the blood and cerebrospinal fluid (CSF) levels, uric acid levels in the CSF in healthy individuals are reportedly only 5–6% of those in the blood. It has therefore been questioned whether uric acid can have any meaningful effect on brain cells (8).
5. Uric acid and stroke
According to data from the U.S. National Health and Nutrition Examination Survey (NHANES) obtained in 2015–2018, the prevalence of cardiovascular disease (including coronary artery disease, heart failure, stroke, and hypertension) among adults aged 20 years and older is 49.2% overall (126.9 million in 2018) and increases with age in both men and women (9). Stroke is the second most common cause of death in the world. Ischemic stroke is defined as necrosis of nerve cells because of the sudden cessation of blood in the brain, ultimately leading to brain inflammation (10). Stroke is clinically classified as lacunar stroke, atherosclerotic brain infarction is caused by atherosclerosis, cardiogenic stroke is caused by embolism, and other stroke types such as embolic stroke are caused by an undetermined source. The risk factors common to other cardiovascular diseases, such as hypertension, diabetes, dyslipidemia, hyperuricemia, CKD, and smoking, are involved in the development of stroke. Hyperuricemia may also play a role in the development of cardiovascular disease, stroke, and death; however, insufficient evidence has been provided to date.
5.1. Uric acid as a risk factor for stroke
In 2009, Kim et al. reported a systematic review and meta-analysis of the association between hyperuricemia and stroke incidence and death. In this study, hyperuricemia was associated with the risk of both stroke incidence and death, and an analysis adjusting for known risk factors such as age, hypertension, diabetes, and cholesterol also found a similar association (11). In 2014, Li et al. reported a meta-analysis of the association between hyperuricemia and stroke incidence and mortality and similarly found that hyperuricemia was correlated with both stroke incidence and mortality (12). This relationship was more pronounced in women. In 2017, a meta-analysis revealed a significant dose–response relationship between increased serum uric acid levels and stroke risk, with an ~10% increase in stroke risk for every 1 mg/dL increase in serum uric acid levels (13). In addition, the Reasons for Geographic and Racial Differences in Stroke (REGARDS) study in 2020 was a case–cohort study with a large data set. This study also concluded that hyperuricemia may be a risk factor for stroke and noted that hypertension severity may be a confounding factor in the association between serum uric acid levels and stroke (14). Together, these findings suggest that hyperuricemia is strongly associated with hypertension and may have a significant impact on stroke incidence. However, because negative results also exist regarding the relationship between serum uric acid levels and stroke, serum uric acid levels may not be a clear risk factor for stroke (15–17). These inconsistent results may derive from differences in study settings and participants. Table 1 summarized the evidence of investigating the relationship between stroke and serum uric acid levels (11–17, 22, 23).
5.2. Stroke and hyperuricemia associated with CKD
In CKD, uric acid excretion decreases, and serum uric acid levels increase as the glomerular filtration rate decreases. Although CKD is considered an independent risk factor for stroke, there are no clear reports on the association between uric acid levels and stroke in CKD patients. However, treatment with allopurinol, which inhibits uric acid synthesis by blocking xanthine oxidase activity, has been reported to reduce the progression of CKD (18). In addition, allopurinol treatment is associated with lower rates of stroke in older patients with hypertension, particularly at higher doses of allopurinol (19). However, because no prospective clinical trials have evaluated whether allopurinol improves stroke, there is not yet sufficient evidence for its use in stroke patients.
CKD may also increase the risk of stroke in patients with non-valvular atrial fibrillation. A meta-analysis by Zeng et al. showed that atrial fibrillation patients with an estimated glomerular filtration rate of less than 60 mL/min had a significantly higher risk of developing thromboembolic events than patients with an estimated glomerular filtration rate of over 60 mL/min (relative risk: 1.62, 95% confidence interval: 1.40 to 1.87, p < 0.001). The annual incidence of thromboembolic events increased by 0.41% (95% confidence interval: 0.17% to 0.65%) with a 10 mL/min decrease in renal function (20). Furthermore, Singh et al. demonstrated an association between allopurinol use and the risk of incident atrial fibrillation in the elderly. In a multivariate-adjusted analysis, allopurinol use was associated with the development of atrial fibrillation (hazard ratio: 0.83, 95% confidence interval: 0.74 to 0.93). In addition, prolonged allopurinol use was associated with a lower hazard ratio for atrial fibrillation (21).
5.3. Hypouricemia and stroke
Several epidemiologic studies have shown a J-shaped association between serum uric acid and cardiovascular events. In the Progetto Ipertensione Umbria Monitoraggio Ambulatoriale (PIUMA) study of a large cohort of patients with essential hypertension, the relationship between serum uric acid and cardiovascular events and all-cause mortality was J-shaped in both men and women, with a nadir in the second quartile (4.5 to 5.2 mg/dL in men, 3.2 to 3.9 mg/dL in women). These results indicate that cardiovascular events increase with hypouricemia (≤ 4.5 mg/dL in men, ≤ 3.2 mg/dL in women). (22). Moreover, in a longitudinal national cohort study by Kamei et al., serum uric acid levels above 7.1 mg/dL in men and 5.5 mg/dL in women were independently associated with the incidence of non-fatal stroke. Similarly, the risk of stroke was increased in this study, even in the group with lower uric acid levels, with a J-shaped curve (23). In other studies, the relationship between serum uric acid levels and stroke risk differed by sex, with a J-shaped relationship in men but a nearly linear trend in women (13, 15). Thus, although a wellestablished relationship between serum uric acid levels and stroke seems to exist, a causal relationship remains controversial because of a lack of solid evidence (24).
5.4. Uric acid localization in vessels
Using carotid endarterectomy specimens, Patetsios et al. found the presence of uric acid, along with cholesterol and xanthine oxidase, in atherosclerotic plaques (25). In addition, Park et al. found that monosodium urate (MSU) was present in the coronary arteries of gout patients. They suggested that uric acid crystals in the intima of blood vessels in gout patients may cause inflammation that leads to plaque rupture, thereby posing a risk of cardiovascular disease (26). This mechanism is thought to be the same mechanism by which gouty arthritis develops. In gouty arthritis, activation of gout salt microcrystals induces multiple inflammatory mediators, including interleukin-1β in the NALP3 inflammasome pathway (27). Similarly, in blood vessels, MSU crystal deposits in coronary arteries can trigger a corresponding inflammatory cascade leading to thrombosis and infarction. Several studies have used dual-energy computed tomography to reveal intravascular MSU deposits. For example, Klauser et al. identified MSU deposits in vessels by polarized light microscopy and dual-energy computed tomography; intravascular MSU deposition was detected frequently in gout patients and was associated with higher coronary calcium scores (28).
5.5. Effects of uric acid-lowering drugs in stroke
Hyperuricemia is associated with stroke events, but it is necessary to evaluate whether the drug-induced reduction of uric acid decreases the risk of these events. Larsen et al. conducted a cohort study of patients with serum uric acid levels of 6 mg/dL or higher in Denmark from 1992 to 2010. Among the 65,971 hyperuricemic patients surveyed, 7,127 patients were taking allopurinol. Compared with non-users, allopurinol users had a hazard ratio of 0.89 (95% confidence interval: 0.81 to 0.97) for cardiovascular outcomes and a hazard ratio of 0.68 (95% confidence interval: 0.62 to 0.74) for all-cause mortality. These results indicate that oral allopurinol is associated with a risk reduction of major cardiovascular events, including stroke (29). Moreover, in a study examining the association between allopurinol treatment initiation and stroke from 2006 to 2012, allopurinol treatment reduced the hazard ratio for stroke by 9% (95% confidence interval: 0.83 to 0.99). The beneficial effect of starting allopurinol treatment was seen at 6 months after initiation and was greater with longer use (30). MacIsaac et al. followed cardiovascular outcomes for 10 years in hypertensive patients aged 65 years and older in the United Kingdom. Allopurinol reduced the hazard ratio for stroke by 0.50 (95% confidence interval: 0.32 to 0.80) and for cardiovascular events by 0.61 (95% confidence interval: 0.43 to 0.87); this result was particularly true for high doses (300 mg/day or higher) (19).
A few prospective trials have also been conducted in patients with acute ischemic stroke. In a randomized, double-blind, controlled study, 70 patients (45 women and 25 men) with acute ischemic stroke received allopurinol (200 mg/day) for 3 months and were evaluated for functional outcomes using the modified Rankin scale. After 3 months, the rate of good functional status (modified Rankin scale score = 0–2) was 40.0% in the placebo group and 65.7% in the allopurinol group. A strong association was observed between good functional status and allopurinol administration (odds ratio = 4.646, p = 0.014) (31). By contrast, in a large observational study that compared probenecid with allopurinol in patients aged 65 years or older with gout, the gout patients initiating treatment with probenecid had a lower risk of hospitalization for myocardial infarction or stroke than those treated with allopurinol (hazard ratio: 0.80, 95% confidence interval: 0.69 to 0.93). These results thus indicate no beneficial effects of allopurinol (32). Similarly, the Febuxostat Vs. Placebo Randomized Controlled Trial Regarding Reduced Renal Function in Patients With Hyperuricemia Complicated by Chronic Kidney Disease Stage 3 (FEATHER) study—a multicenter, randomized, controlled trial in Japan—reported no difference in all-cause mortality, cardiac disease, or stroke events after 108 weeks of treatment with febuxostat vs. placebo (33). The multicenter, prospective, randomized, open-label Febuxostat for Cerebral and CaRdiorenovascular Events PrEvEntion StuDy (FREED) trial also showed no difference in the primary composite endpoint (death from cerebral, cardiovascular, or renal disease) between the febuxostat and non-febuxostat groups at 36 months of observation (34). Table 2 summarized the evidence for pharmacological intervention with uric acid lowering drugs for stroke (29–34).
6. Parkinson's disease
Parkinson's disease is the second most common neurodegenerative disease after Alzheimer's disease and is the most common neurodegenerative disease-causing movement disorder. The incidence of new onset is 5–35 per 1,00,000 persons, and the prevalence is 100–300 per 1,00,000 persons (35). The most common age of Parkinson's disease onset is 50–65 years, but the incidence usually increases with advancing age. Parkinson's disease is generally twice as common in men as in women, although some reports in Japan suggest that it is somewhat more common in women (36). Parkinson's disease is characterized by the loss of neurons in specific areas of the substantia nigra as well as extensive intracellular protein (alpha-synuclein) accumulation (35). Clinical diagnosis is made by identifying characteristic motor signs such as resting tremor and bradykinesia. Additionally, non-motor symptoms such as olfactory impairment, constipation, sleep disturbances, and cognitive impairment commonly occur at all stages of the disease.
6.1. Uric acid levels in Parkinson's disease
Elevated blood uric acid levels have been linked to a decreased risk of developing Parkinson's disease. Analyses adjusted for age, smoking, and caffeine use showed that higher uric acid quartiles were associated with lower odds ratios for Parkinson's disease. The odds ratio for the highest quartile compared with the lowest quartile was 0.43 (95% confidence interval: 0.18 to 1.02). A significant dose–response relationship was also observed when uric acid levels were analyzed as a continuous variable. The odds ratio for Parkinson's disease decreased by 0.76 for every 1 mg/dL increase in uric acid concentration (95% confidence interval: 0.61 to 0.95; p = 0.017) (37).
The Parkinson Research Examination of CEP-1347 Trial (PRECEPT), including 84 patients with early-stage Parkinson's disease (mean follow-up 21.4 months), was conducted from April 2002 to August 2005 to evaluate the efficacy of neuroprotective agents on the rate of Parkinson's disease progression. The primary outcome was progression to clinical disability requiring dopaminergic therapy. Parkinson's disease progression decreased with increasing serum uric acid levels; this association was stronger in men than in women. This study also evaluated changes in striatal uptake of the presynaptic dopamine transporter marker, iodine I 123-labeled 2-beta-carbomethoxy-3-beta-(4-iodophenyl) tropane. The rate of loss of this uptake also improved with increasing serum uric acid levels (38). Similarly, in the Deprenyl and Tocopherol Antioxidative Therapy of Parkinsonism (DATATOP) study, higher baseline serum and CSF uric acid levels were linked to a slower progression of Parkinson's disease (39).
Moccia et al. showed a relationship between serum uric acid levels and the presence and progression of non-motor symptoms in new-onset Parkinson's disease patients (40). In drug-naïve patients with Parkinson's disease, serum uric acid levels were correlated with the severity of dopaminergic impairment in the caudate, putamen, and striatum on dopamine transporter scans (41). Sleeman et al. assessed movement severity with the Movement Disorder Society Unified Parkinson's Disease Rating Scale Part III and demonstrated that lower serum uric acid levels were associated with worse motor function in patients with Parkinson's disease (42). These results were reviewed, and lower plasma uric acid levels in men were added as a new biomarker in the Movement Disorder Society study criteria for prodromal Parkinson's disease (43), a condition in which patients do not yet exhibit typical motor signs. Together, these findings suggest a strong epidemiological association between Parkinson's disease and uric acid; low serum uric acid is associated with the risk of development, progression, and severity of Parkinson's disease.
Although the exact pathogenesis of Parkinson's disease is still unclear, oxidative stress because of mitochondrial dysfunction, neuroinflammation, and alpha-synuclein misfolding/aggregation and impaired protein clearance triggered by multifactorial factors such as aging, genetic factors, and environmental/lifestyle factors could be involved (44). Uric acid is a potent antioxidant and is considered a potential neuroprotective agent. If healthy individuals have neuroprotection through uric acid, older individuals who develop Parkinson's disease may lack this defense and be more vulnerable to oxidative stress (45); in vitro and in vivo studies have shown that uric acid protects dopaminergic neurons (4). The prevailing view is that low uric acid levels increase oxidative stress, which causes dopaminergic neuron degeneration and triggers disease onset. Meanwhile, reverse causality suggests that symptoms and conditions associated with Parkinson's disease—such as mitochondrial dysfunction, inherently low purine metabolism, dietary habits, changes in gastrointestinal motility and microbiomes, and reduced physical activity—may lead to low uric acid levels (8). Table 3 summarized the evidence of investigating the relationship between Parkinson's disease and serum uric acid levels (37–42).
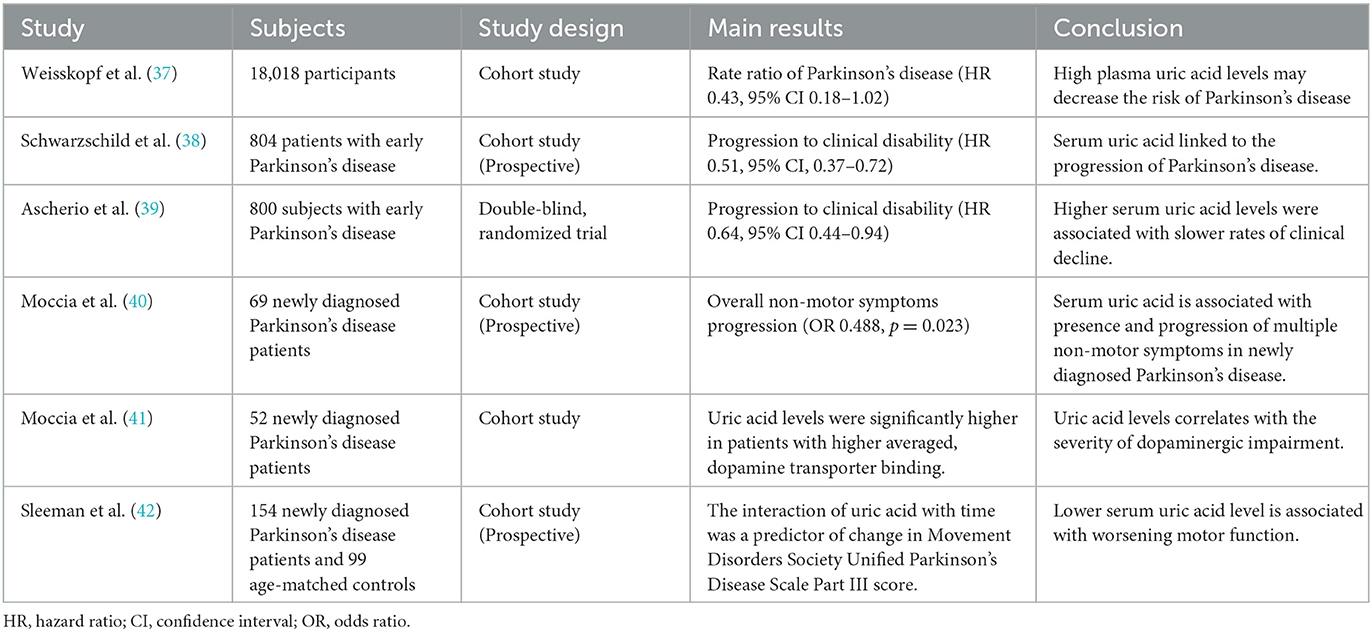
Table 3. Evidence of investigating the relationship between Parkinson's disease and serum uric acid levels.
6.2. Inosine treatment in Parkinson's disease
Oral administration of uric acid cannot increase serum uric acid levels because uric acid is rapidly degraded by the bacterial flora in the intestinal tract. However, oral ingestion of its precursor, inosine, quickly increases serum urate levels (46). Therefore, a randomized, double-blind, placebo-controlled, dose-ranging study of inosine (Safety of Urate Elevation in Parkinson's Disease [SURE-PD]) was conducted. Seventy-five outpatients attending an American Parkinson Study Group-accredited clinical trial facility between 2009 and 2011 were enrolled and received a placebo or inosine (up to two 500 mg capsules orally, up to three times a day for up to 24 months, median 18 months) to produce mildly (6.1–7.0 mg/dL) or moderately (7.1–8.0 mg/dL) high serum uric acid levels. The primary endpoints were the absence of unacceptable serious adverse events (“safety”), continued treatment without adverse events requiring dose reduction (“tolerability”), and elevated serum and CSF uric acid levels. Inosine was shown to be safe, well tolerated, and effective in increasing serum and CSF uric acid levels (47). In addition, Bhattacharyya et al. found a dose-dependent increase in serum uric acid levels with an increase in antioxidant capacity as assessed using urine 8-hydroxydeoxyguanosine (48). These results prompted a randomized, double-blind, placebo-controlled, phase III study of oral inosine in early Parkinson's disease. The primary endpoint was the rate of change in the Movement Disorders Society Unified Parkinson's Disease Rating Scale prior to the initiation of dopaminergic therapy; however, the rate of parkinsonism progression did not differ between the placebo and inosine groups (49). Although this study included Parkinson's disease patients who would be expected to benefit more from elevated uric acid treatment, the increase in uric acid levels with inosine did not seem to support a protective effect of uric acid. This may be because the urate precursor inosine, rather than uric acid, was received; this may have had detrimental effects that offset the benefits of uric acid elevation, such as mediating the production of xanthine oxidase. However, it may also be that uric acid is more useful as a biomarker for the severity and progression of Parkinson's disease than as a neuroprotective agent. Table 4 summarized the evidence of investigating the relationship between receiving inosine and neurodegenerative diseases (38, 47, 48, 59–62, 75).
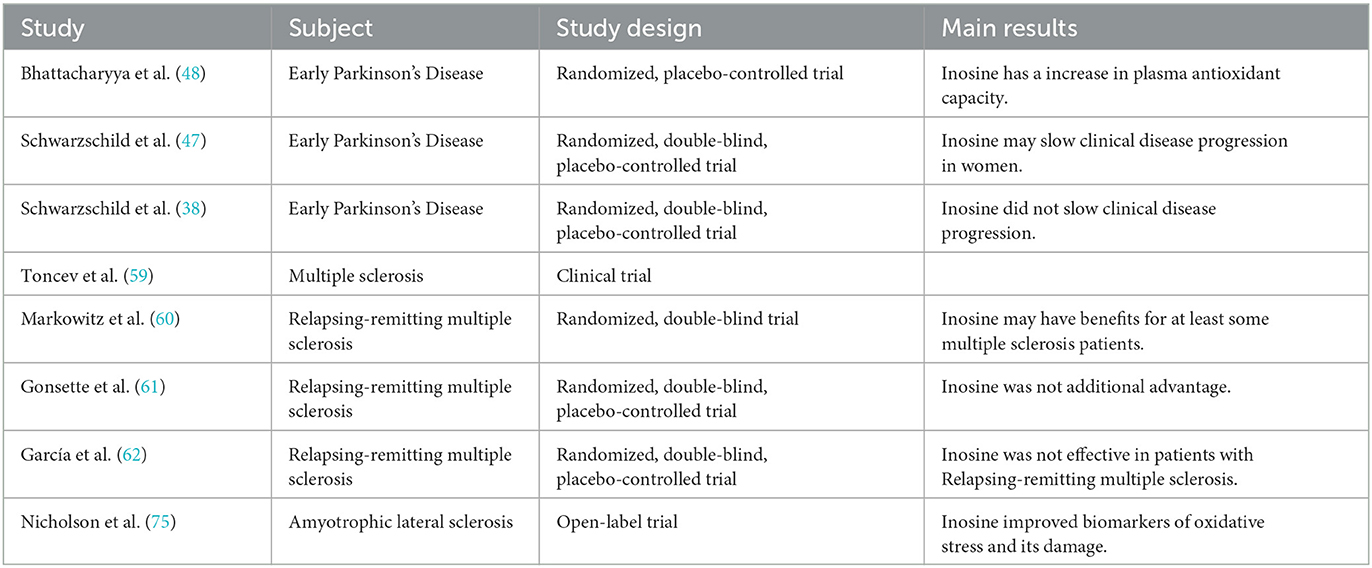
Table 4. Evidence of investigating the relationship between receiving inosine and neurodegenerative diseases.
7. Multiple sclerosis
Multiple sclerosis is a chronic inflammatory demyelinating disease that affects the central nervous system and is most frequently associated with disability in young adults. Multiple sclerosis affects an estimated 2.8 million people worldwide but has a heterogeneous prevalence. The majority of multiple sclerosis patients are diagnosed between the ages of 20 and 50 years, but the disease can develop at any age. The incidence of disease is higher in women than in men. The cause of multiple sclerosis is multifactorial, with both genetic and environmental risk factors contributing to disease risk. In terms of genetic risk, the association between HLA-DRB1*15:01 and a high risk of multiple sclerosis has been known for decades. The most commonly considered environmental risk factor is infection with Epstein–Barr virus; other factors that may be involved include smoking, vitamin D levels, ultraviolet light, vaccines, and obesity (50). However, the specific causes of multiple sclerosis remain largely unknown, and there are currently no wellestablished factors that can help to prevent the disease.
7.1. Uric acid levels in multiple sclerosis
A meta-analysis by Wang et al. showed that serum uric acid levels in patients with multiple sclerosis were significantly lower than in healthy individuals, which supports an association between multiple sclerosis and uric acid (51). Moreover, Drulović et al. reported that serum uric acid levels tended to be lower in multiple sclerosis patients than in patients with other neurological diseases although this difference was not significant (P = 0.068). However, the mean serum uric acid level in active multiple sclerosis patients was significantly lower than that in inactive multiple sclerosis patients (P = 0.046) and patients with other neurological diseases (P = 0.007). These results indicate that serum uric acid might act as a biomarker of disease activity in multiple sclerosis (52). Supporting this idea, Simental-Mendía et al. suggested that elevated uric acid levels are likely linked to the presence of benign multiple sclerosis (53). Furthermore, in patients with relapsing-remitting multiple sclerosis, Moccia et al. reported a link between the progressive reduction of uric acid levels and relapse risk, disability progression, and cognitive function (54). They also examined serum uric acid levels in patients with relapsing-remitting and secondary-progressive forms of multiple sclerosis and healthy individuals; their findings suggested the importance of serum uric acid as a biomarker of multiple sclerosis disability and progression (55). Niu et al. performed a meta-analysis of 110,347 patients; their estimate based on the single-nucleotide polymorphism rs12498742, which explained the largest proportion of variance, revealed that the odds ratio of uric acid for multiple sclerosis was 1.00 (95% confidence interval: 0.90 to 1.11; p = 0.96). Mendelian randomization analysis showed that the pooled odds ratio was 1.05 (95% confidence interval: 0.92 to 1.19; p = 0.50). The findings of this study, therefore, did not indicate that serum uric acid levels are causally associated with the risk of multiple sclerosis (56).
In vivo studies of multiple sclerosis have demonstrated that inosine or inosinic acid can increase serum uric acid levels and has good efficacy (57). Furthermore, oral administration of uric acid did not increase serum uric acid levels, but oral administration of its precursor, inosine, increased serum uric acid levels and was generally well tolerated (58). Toncev conducted a clinical study to evaluate the safety and efficacy of oral inosine as a monotherapy in patients with multiple sclerosis. A total of 32 patients with multiple sclerosis were administered 1–2 g of inosine daily; inosine administration was associated with a significantly lower recurrence rate (P = 0.001) and a greater increase in mean Expanded Disability Status Scale (EDSS) scores (P = 0.025) (59). Markowitz et al. conducted a randomized, double-blind trial evaluating relapse rate, disability assessed by EDSS, and magnetic resonance imaging features in 16 patients with relapsing-remitting multiple sclerosis who received oral inosine for 1 year. Elevated serum uric acid levels were correlated with fewer gadolinium-enhanced lesions on magnetic resonance imaging as well as improved EDSS. These findings suggest that the use of inosine to increase serum uric acid levels may be beneficial for at least some multiple sclerosis patients (60). Similarly, the ASsociation of Inosine and IFNβ in RRMS (ASIIMS) trial was a multicenter, double-blind, placebo-controlled trial designed to investigate whether inosine supplementation to standard therapy was effective in patients with relapsing-remitting multiple sclerosis. This study did not find any benefits associated with the addition of inosine (61). Muñoz García et al. conducted a trial in which 36 patients with relapsing-remitting multiple sclerosis who started treatment were randomly assigned either inosine (3 g/day) or placebo, with a follow-up period of 12 months. The study concluded that inosine had no neuroprotective role because it had no effect on patients with relapsing-remitting multiple sclerosis. However, they also suggested that phase III trials with a longer follow-up period, of more than 1 year, may be needed to evaluate the efficacy of inosine in preventing disease recurrence and progression (62). Given the results of preclinical and clinical studies of inosine use, the most important properties of inosine seem to be its antioxidant actions and its potential to increase energy resources by improving adenosine triphosphate availability (63). Table 5 summarized the evidence of investigating the relationship between multiple sclerosis and serum uric acid levels (40–42, 51, 52, 56).
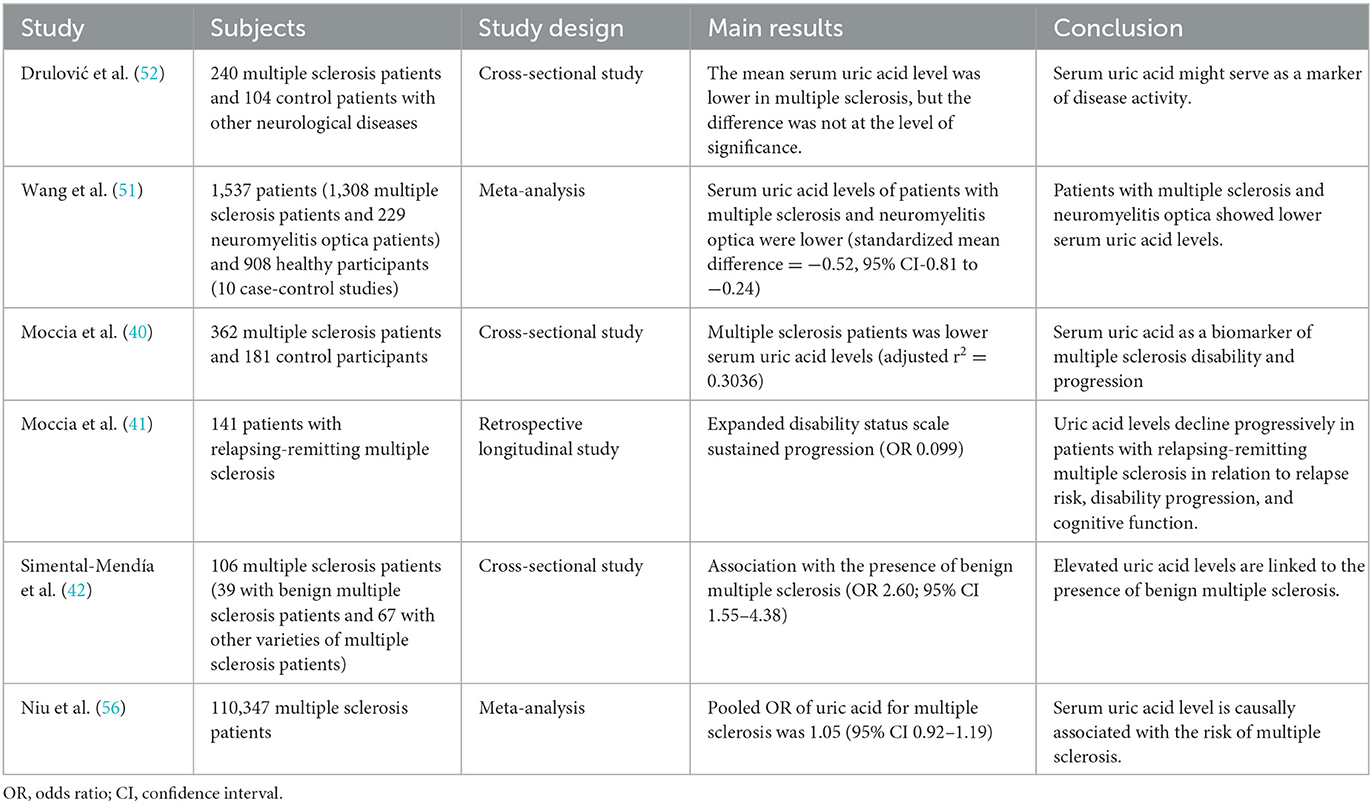
Table 5. Evidence of investigating the relationship between multiple sclerosis and serum uric acid levels.
8. Neuromyelitis optica spectrum disorder
Neuromyelitis optica spectrum disorder (NMOSD) is an inflammatory demyelinating disease of the central nervous system that primarily involves the optic nerve and spinal cord (64). After the identification of the aquaporin-4 immunoglobulin G antibody, NMOSD was differentiated from multiple sclerosis. NMOSD-related brain lesions, such as those in the thalamus, periependymal surfaces of the third ventricle, hypothalamus, dorsal medulla, and area postrema, have also been described. In the absence of spinal cord involvement or optic neuropathy, the diagnosis of NMOSD can be established by the presence of aquaporin-4 antibody positivity and NMOSD-associated brain lesions.
8.1. Uric acid levels in NMOSD
Several studies have suggested that uric acid and NMOSD are associated. Shu et al. reported that CSF uric acid levels in NMOSD were significantly higher than in control individuals (with non-inflammatory and non-neurodegenerative diseases), but that serum uric acid levels were not significantly different between the two groups. Additionally, they showed that CSF uric acid levels were higher in patients with relapsing NMOSD than in those with NMOSD in the inactive phase. Furthermore, CSF uric acid levels were significantly higher in patients with an impaired blood–brain barrier than in those with an intact blood–brain barrier, suggesting that CSF uric acid levels are affected by blood–brain barrier integrity and may be used as a marker of disease activity (65). In contrast, another study found that serum uric acid levels in NMOSD patients during relapses were significantly lower than those in healthy individuals and that these reduced uric acid levels during NMOSD relapse were normalized during remission (66). In addition, in the differentiation of myelin oligodendrocyte glycoprotein antibody disease and NMOSD, serum uric acid is higher in myelin oligodendrocyte glycoprotein antibody disease, suggesting that serum uric acid may be a potential marker to differentiate these two diseases (67). Liu et al. reported that serum uric acid levels were correlated with NMOSD disease duration and inversely correlated with disability scores, suggesting that serum uric acid may be a useful biomarker for monitoring disease activity (68). Furthermore, a meta-analysis revealed that, similar to in multiple sclerosis, serum uric acid levels are lower in NMOSD patients than in healthy individuals; serum uric acid may thus be a potential diagnostic biomarker for both multiple sclerosis and NMOSD (51). Table 6 summarized the evidence of investigating the relationship between NMOSD and uric acid levels (51, 65–68).
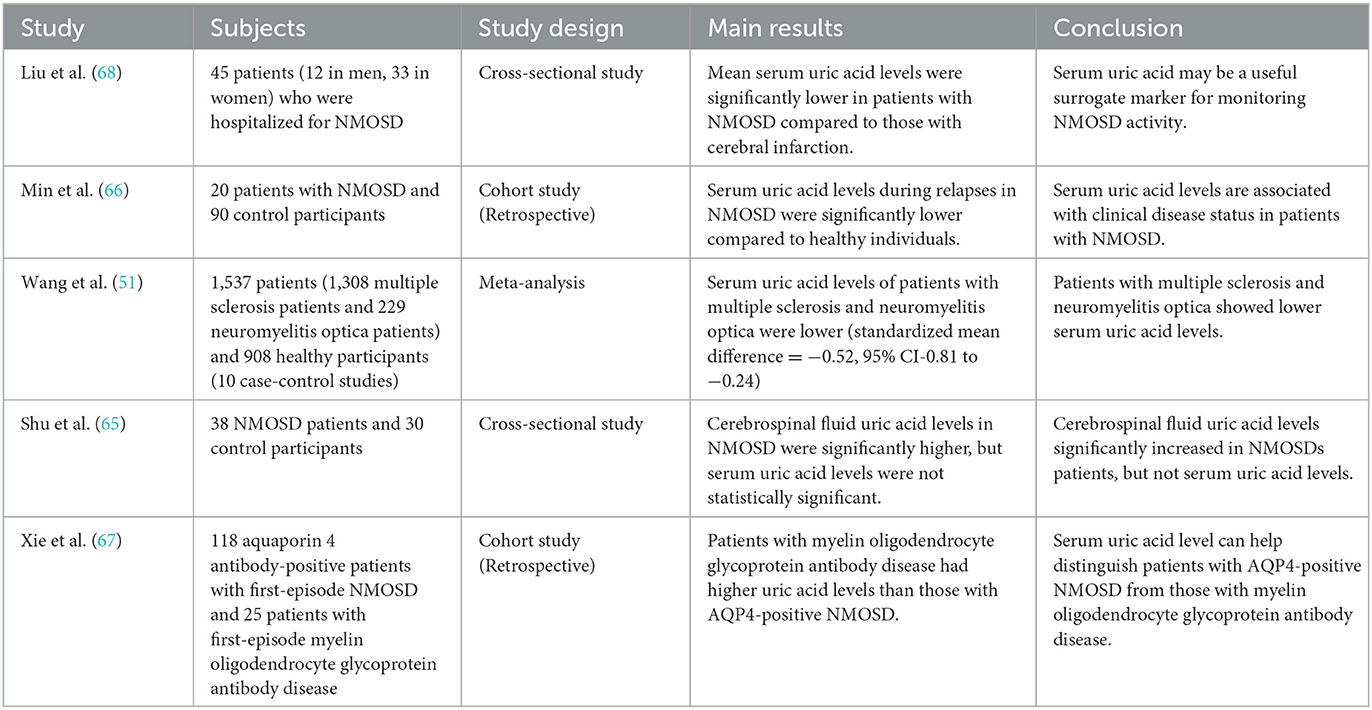
Table 6. Evidence of investigating the relationship between neuromyelitis optica spectrum disorders (NMOSD) and uric acid levels.
9. Amyotrophic lateral sclerosis
Amyotrophic lateral sclerosis (ALS) is a motor neuron disease that involves both upper and lower motor neurons. It is characterized by progressive degeneration of motor neurons, resulting in dysarthria, dysphagia, tetraplegia, and respiratory impairment. Approximately 90–95% of ALS cases are sporadic. The etiology of ALS is unknown, but oxidative stress and glutamate-mediated toxicity are suggested to be involved. The average life expectancy of patients with ALS is 11.3 years with a ventilator and 4.6 years without a ventilator (69). Therefore, disease-modifying therapies that improve patient prognosis are desperately needed.
9.1. Uric acid levels and inosine treatment in ALS
It has been suggested that increased uric acid levels may have a beneficial effect against ALS. Additionally, preclinical studies have reported the neuroprotective function of inosine in this disease. Inosine supplementation in fibroblasts from patients with ALS is bioenergetically favorable and may protect against age-related metabolic dysfunction in ALS (70). A descriptive study comprising patients diagnosed with gout from 2011 to 2018 revealed that ALS prevalence was lower in patients with gout than in those without gout (71). In addition, low uric acid levels are reportedly associated with cognitive decline and mortality in ALS (72, 73). The results of a clinical study including 40 patients who received edaravone for ALS suggested that ALS progression was slower in patients with high baseline uric acid levels and in those whose uric acid levels did not decrease (74). Based on these results, an open-label pilot study was conducted that included 25 patients with ALS. Inosine was titrated to increase serum uric acid levels to 7–8 mg/dL and was administrated for 12 weeks. The primary endpoints were safety and tolerability, while the secondary endpoints were biomarkers of oxidative stress and its damage. The results of this clinical trial showed no serious adverse events and no incidence of urinary tract stones or gout, and the administration of inosine resulted in a significant improvement in biomarkers. Inosine thus appears to be safe, well tolerated, and effective in increasing serum uric acid levels in ALS patients. This observation indicates the potential for a large clinical trial to investigate inosine as a therapy for ALS (75). Table 7 summarized the evidence of investigating the relationship between Amyotrophic lateral sclerosis and serum uric acid levels (71–74).
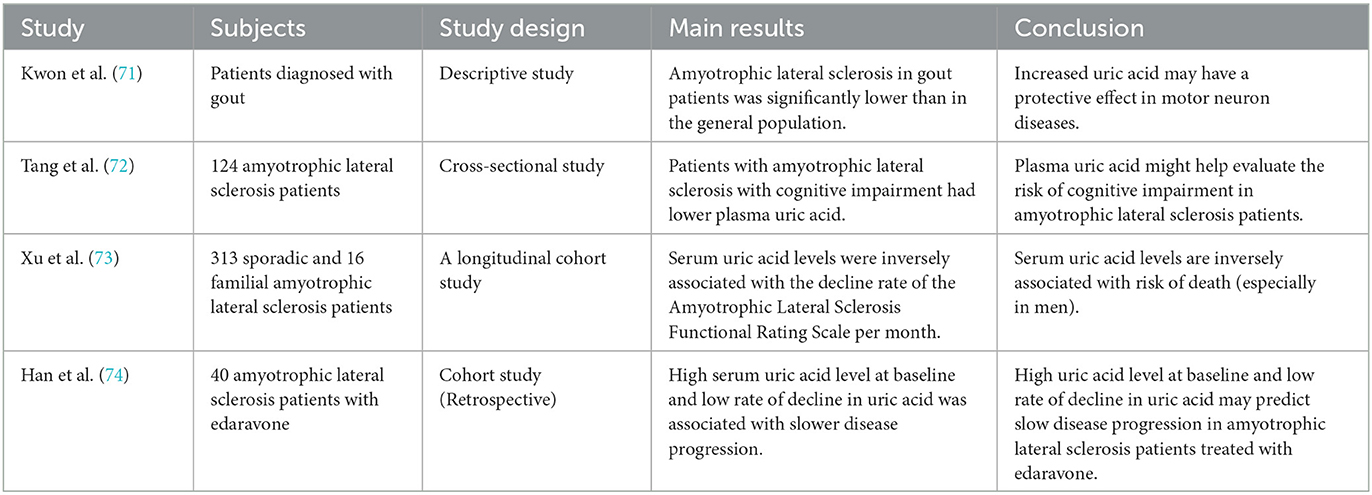
Table 7. Evidence of investigating the relationship between Amyotrophic lateral sclerosis and serum uric acid levels.
10. Relationship between uric acid levels and risk of dementia (Alzheimer's disease and vascular dementia)
In a prospective study of 4,618 participants aged 55 years or older, Euser et al. found a decreased risk of dementia in patients with high serum uric acid levels after correcting for several cardiovascular risk factors (hazard ratio: 0.89, 95% confidence interval: 0.80 to 0.99) (76). Lu et al. used data from the Health Improvement Network in the United Kingdom to evaluate the impact of gout on the risk of developing Alzheimer's disease in a cohort matched for age, sex, time of entry, and body mass index. In this study, 59,224 patients with gout and 238,805 controls were followed for 5 years; 309 new patients with Alzheimer's disease were found among patients with gout (1.0 per 1,000 person-years), and 1,942 new patients were found among controls (1.5 per 1,000 person-years). These results indicate that gout is inversely associated with the risk of developing Alzheimer's disease and support a possible neuroprotective role for uric acid (77). Du et al. analyzed 24 studies, including 21 case–control studies and 3 cohort studies, and found that patients with Alzheimer's disease had lower uric acid levels than healthy individuals (weighted mean difference = −0.77 mg/dL, 95% confidence interval: −2.28 to −0.36, p = 0.0002), and that higher serum uric acid levels were correlated with a lower risk of developing Alzheimer's disease (risk ratio: 0.66, 95% confidence interval: 0.52 to 0.85, p = 0.001) (78). Furthermore, Cascalheira et al. found that uric acid was an independent predictor of Alzheimer's disease in a case–control study in Portugal of 19 patients diagnosed with Alzheimer's disease and 36 older adult volunteers without symptoms of memory impairment or neurological diseases (odds ratio: 2.42, p < 0.02). They also reported a positive correlation between uric acid and Alzheimer's disease; however, the authors stated that this result was not caused by the antioxidant effect of uric acid but rather because reduced renal function is associated with Alzheimer's disease (79).
Khan et al. conducted a systematic review and meta-analysis examining serum uric acid levels in relation to dementia subtypes such as Alzheimer's disease, vascular dementia, and Parkinson's disease-related dementia. Overall, patients diagnosed with dementia had lower serum uric acid levels than controls; however, differences were observed by dementia type. Alzheimer's disease and Parkinson's disease-related dementia showed a strong association with serum uric acid levels, but no correlation was found in patients with vascular or mixed dementia, suggesting a different relationship with serum uric acid levels, especially in patients with vascular dementia compared with other dementia subtypes (80). Moreover, in a cohort of healthy older adults (Three-City Dijon cohort) with a mean age of 72 years, 1,598 patients were followed for 10 years, and 110 developed dementia (8.2/1,000 person-years). The multivariate hazard ratio of the highest to lowest serum uric acid level was 1.79 (95% confidence interval: 1.17 to 2.73, p = 0.007), and this association was stronger for vascular or mixed dementia (hazard ratio: 3.66, 95% confidence interval: 1.29 to 10.41, p = 0.015) than for Alzheimer's disease (hazard ratio: 1.55, 95% confidence interval: 0.92 to 2.61, p = 0.10) (81). These results indicate that high serum uric acid levels are protective against the development of dementia because of the antioxidant effects of uric acid. However, hyperuricemia promotes arteriosclerosis and adversely affects cognitive function, suggesting that both effects should be considered.
Interestingly, the relationship between serum uric acid levels and cognitive function may be stronger in the early stages of cognitive dysfunction. In a study comparing 58 patients with mild cognitive impairment (MCI) with 57 healthy older adults, serum uric acid levels were significantly lower in MCI patients (292.3 μmol/L) than in healthy older adults (322.5 μmol/L; p < 0.05) (82). Furthermore, although the sample size was small, there were significant positive correlations between Mini Mental State Examination scores and serum uric acid levels, including orientation, memory, computation, language, and five visual spatial dimensions (P < 0.05). In addition, a cross-sectional survey of 2,102 patients was conducted in Beijing, China. The prevalence rates of hyperuricemia and MCI in this community population were 16.7% and 15.9%, respectively, and prevalence rates increased linearly with age (P < 0.001) (83).
11. Discussion
The relationship between serum uric acid levels and neurological diseases remains controversial. Regarding the reasons, the hypothesis of a toxic effect in atherosclerotic diseases and a protective effect in neurodegenerative diseases and dementia is currently supported. However, the biological mechanisms underlying these relationships have not yet been firmly established. Low uric acid may be a biomarker of neurological diseases, and the possibility that uric acid alterations are a consequence rather than a cause of neurological disease cannot be ruled out. This possibility is supported by the relatively poor results of treatment with inosine, which elevates uric acid. However, it should also be noted that uric acid is not a biologically inert substance but has a wide range of actions including antioxidant, neurostimulatory, inflammation-inducing, and innate immune response-activating activities. In particular, uric acid has two opposing actions—namely antioxidant and oxidative effects—and it has been reported that both hypouricemia and hyperuricemia can lead to the development of cardiovascular diseases. When assessing uric acid levels in the future, it is, therefore, necessary to consider individuals with high and low uric acid separately, limit target diseases, and promote further research.
Author contributions
NO, EH, and MO: writing—original draft preparation. HT and KS: writing—reviewing and editing. All authors have read and agreed to the published version of the manuscript.
Acknowledgments
We thank Lisa Kreiner, Ph.D., and Bronwen Gardner, Ph.D., from Edanz (https://jp.edanz.com/ac) for editing a draft of this manuscript.
Conflict of interest
The authors declare that the research was conducted in the absence of any commercial or financial relationships that could be construed as a potential conflict of interest.
Publisher's note
All claims expressed in this article are solely those of the authors and do not necessarily represent those of their affiliated organizations, or those of the publisher, the editors and the reviewers. Any product that may be evaluated in this article, or claim that may be made by its manufacturer, is not guaranteed or endorsed by the publisher.
References
1. Japanese Society of Gout and Nucleic Acid Metabolism. Japanese Guideline for the Management of Hyperuricemia and Gout. 3rd edn. Tokyo, SHINDAN TO CHIRYO SHA, Inc (2018). p. 18–23.
2. Johnson RJ. I Why focus on uric acid? Curr Med Res Opin. (2015) 8:14181. doi: 10.1185/03007995.2015.1087979
3. Otani N, Ouchi M, Misawa K, Hisatome I, Anzai N. Hypouricemia and urate transporters. Biomedicines. (2022) 10:652. doi: 10.3390/biomedicines10030652
4. Crotty GF, Ascherio A, Schwarzschild MA. Targeting urate to reduce oxidative stress in Parkinson disease. Exp Neurol. (2017) 298:210–24. doi: 10.1016/j.expneurol.2017.06.017
5. Gersch C, Palii SP, Imaram W, Kim KM, Karumanchi SA, Angerhofer A, et al. Reactions of peroxynitrite with uric acid: formation of reactive intermediates, alkylated products and triuret, and in vivo production of triuret under conditions of oxidative stress. Nucleosides Nucleotides Nucleic Acids. (2009) 28:118–49. doi: 10.1080/15257770902736400
6. Yeum KJ, Russell RM, Krinsky NI, Aldini G. Biomarkers of antioxidant capacity in the hydrophilic and lipophilic compartments of human plasma. Arch Biochem Biophys. (2004) 430:97–103. doi: 10.1016/j.abb.2004.03.006
7. Sautin YY, Nakagawa T, Zharikov S, Johnson RJ. Adverse effects of the classic antioxidant uric acid in adipocytes: NADPH oxidase-mediated oxidative/nitrosative stress. Am J Physiol Cell Physiol. (2007) 293:C584–96. doi: 10.1152/ajpcell.00600.2006
8. Seifar F, Dinasarapu AR, Jinnah HA. Uric Acid in Parkinson's Disease: what is the connection? Mov Disord. (2022) 37:2173–83. doi: 10.1002/mds.29209
9. Virani SS, Alonso A, Aparicio HJ, Benjamin EJ, Bittencourt MS, Callaway CW, et al. Heart disease and stroke statistics-2021 update: a report from the american heart association. Circulation. (2021) 143:e254–743. doi: 10.1161/CIR.0000000000000950
10. Maida CD, Norrito RL, Daidone M, Tuttolomondo A, Pinto A. Neuroinflammatory mechanisms in ischemic stroke: focus on cardioembolic stroke, background, and therapeutic approaches. Int J Mol Sci. (2020) 21:6454. doi: 10.3390/ijms21186454
11. Kim SY, Guevara JP, Kim KM, Choi HK, Heitjan DF, Albert DA. Hyperuricemia and risk of stroke: a systematic review and meta-analysis. Arthritis Rheum. (2009) 61:885–92. doi: 10.1002/art.24612
12. Li M, Hou W, Zhang X, Hu L, Tang Z. Hyperuricemia and risk of stroke: a systematic review and meta-analysis of prospective studies. Atherosclerosis. (2014) 232:265–70. doi: 10.1016/j.atherosclerosis.2013.11.051
13. Zhong C, Zhong X, Xu T, Xu T, Zhang Y. Sex-specific relationship between serum uric acid and risk of stroke: a dose-response meta-analysis of prospective studies. J Am Heart Assoc. (2017) 6:5042. doi: 10.1161/JAHA.116.005042
14. Chaudhary NS, Jr SLB, Saag KG, Rahn EJ, Curtis JR, Gaffo A, et al. Severity of hypertension mediates the association of hyperuricemia with stroke in the REGARDS case cohort study. Hypertension. (2020) 75:246–56. doi: 10.1161/HYPERTENSIONAHA.119.13580
15. Zhang W, Iso H, Murakami Y, Miura K, Nagai M, Sugiyama D, et al. Serum uric acid and mortality form cardiovascular disease: EPOCH-JAPAN Study. J Atheroscler Thromb. (2016) 23:692–703. doi: 10.5551/jat.Er31591
16. Zhang M, Wang Y, Wang K, Yin R, Pan X, Ma A. Association between uric acid and the prognosis of acute ischemic stroke: a systematic review and meta-analysis. Nutr Metab Cardiovasc Dis. (2021) 31:3016–23. doi: 10.1016/j.numecd.2021.07.031
17. Li J, Muraki I, Imano H, Cui R, Yamagishi K, Umesawa M, et al. CIRCS investigators Serum uric acid and risk of stroke and its types: the Circulatory Risk in Communities Study (CIRCS). Hypertens Res. (2020) 43:313–21. doi: 10.1038/s41440-019-0385-5
18. Siu YP, Leung KT, Tong MK, Kwan TH. Use of allopurinol in slowing the progression of renal disease through its ability to lower serum uric acid level. Am J Kidney Dis. (2006) 247:51–9. doi: 10.1053/j.ajkd.2005.10.006
19. MacIsaac RL, Salatzki J, Higgins P. Allopurinol and cardiovascular outcomes in adults with hypertension. Hypertension. (2016) 67:535–40. doi: 10.1161/HYPERTENSIONAHA.115.06344
20. Zeng WT, Sun XT, Tang K, Mei WY, Liu LJ, Xu Q, et al. Risk of thromboembolic events in atrial fibrillation with chronic kidney disease. Stroke. (2015) 46:157–63. doi: 10.1161/STROKEAHA.114.006881
21. Singh JA, Yu S. Allopurinol and the risk of atrial fibrillation in the elderly: a study using Medicare data. Ann Rheum Dis. (2017) 76:72–8. doi: 10.1136/annrheumdis-2015-209008
22. Verdecchia P, Schillaci G, Reboldi G, Santeusanio F, Porcellati C, Brunetti P. Relation between serum uric acid and risk of cardiovascular disease in essential hypertension. The PIUMA study Hypertension. (2000) 36:1072–8. doi: 10.1161/01.HYP.36.6.1072
23. Kamei K, Konta T, Hirayama A. Associations between serum uric acid levels and the incidence of nonfatal stroke: a nationwide community-based cohort study. Clin Exp Nephrol. (2017) 21:497–503. doi: 10.1007/s10157-016-1311-7
24. Ae R, Kanbay M, Kuwabara M. The causality between the serum uric acid level and stroke. Hypertens Res. (2020) 43:354–6. doi: 10.1038/s41440-019-0346-z
25. Patetsios P, Song M, Shutze WP, Pappas C, Rodino W, Ramirez JA, et al. Identification of uric acid and xanthine oxidase in atherosclerotic plaque. Am J Cardiol. (2001) 88:188–91. doi: 10.1016/S0002-9149(01)01621-6
26. Park JJ, Roudier MP, Soman D, Mokadam NA, Simkin PA. Prevalence of birefringent crystals in cardiac and prostatic tissues, an observational study. BMJ Open. (2014) 4:e005308. doi: 10.1136/bmjopen-2014-005308
27. Martinon F, Pétrilli V, Mayor A, Tardivel A, Tschopp J. Gout-associated uric acid crystals activate the NALP3 inflammasome. Nature. (2006) 440:237–41. doi: 10.1038/nature04516
28. Klauser AS, Halpern EJ, Strobl S, Gruber J, Feuchtner G, Bellmann-Weiler R, et al. Dual-energy computed tomography detection of cardiovascular monosodium urate deposits in patients with gout. JAMA Cardiol. (2019) 4:1019–28. doi: 10.1001/jamacardio.2019.3201
29. Larsen, K. S., Pottegård, A., Lindegaard, H. M., and Hallas, J. (2016). Effect of allopurinol on cardiovascular outcomes in hyperuricemic patients: a cohort study. Am J Med. 129:299–306. doi: 10.1016/j.amjmed.2015.11.003
30. Singh JA, Yu S. Allopurinol and the risk of stroke in older adults receiving medicare. BMC Neurol. (2016) 16:164. doi: 10.1186/s12883-016-0692-2
31. Taheraghdam AA, Sharifipour E, Pashapour A, Namdar S, Hatami A, Houshmandzad S, et al. Allopurinol as a preventive contrivance after acute ischemic stroke in patients with a high level of serum uric acid: a randomized, controlled trial. Med Princ Pract. (2014) 23:134–9. doi: 10.1159/000355621
32. Kim SC, Neogi T, Kang EH, Liu J, Desai RJ, Zhang M, et al. Cardiovascular risks of probenecid vs. allopurinol in older patients with gout. J Am Coll Cardiol. (2018) 71:994–1004. doi: 10.1016/j.jacc.2017.12.052
33. Kimura K, Hosoya T, Uchida S, Inaba M, Makino H, Maruyama S, et al. Febuxostat therapy for patients with stage 3 ckd and asymptomatic hyperuricemia: a randomized trial. Am J Kidney Dis. (2018) 72:798–810. doi: 10.1053/j.ajkd.2018.06.028
34. Kojima S, Matsui K, Hiramitsu S, Hisatome I, Waki M, Uchiyama K, et al. Febuxostat for cerebral and cardiorenovascular events prevention study. Eur Heart J. (2019) 40:1778–86. doi: 10.1093/eurheartj/ehz119
35. Simon DK, Tanner CM, Brundin P. Parkinson disease. Nat Rev Dis Primers. (2017) 3:17013. doi: 10.1038/nrdp.2017.14
36. Baldereschi M, Di Carlo A, Rocca WA, Vanni P, Maggi S, Perissinotto E, et al. Parkinson's disease and parkinsonism in a longitudinal study: two-fold higher incidence in men. ILSA Working Group Ital. Longitud. Study Aging Neurol. (2000) 55:1358–63. doi: 10.1212/WNL.55.9.1358
37. Weisskopf MG, O'Reilly E, Chen H, Schwarzschild MA, Ascherio A. Plasma urate and risk of Parkinson's disease. Am J Epidemiol. (2007) 166:561–7. doi: 10.1093/aje/kwm127
38. Schwarzschild MA, Schwid SR, Marek K, Watts A, Lang AE, Oakes D, et al. Serum urate as a predictor of clinical and radiographic progression in Parkinson disease. Arch Neurol. (2008) 65:716–23. doi: 10.1001/archneur.2008.65.6.nct70003
39. Ascherio A, LeWitt PA, Xu K, Eberly S, Watts A, Matson WR, et al. Urate as a predictor of the rate of clinical decline in Parkinson disease. Arch Neurol. (2009) 66:1460–8. doi: 10.1001/archneurol.2009.247
40. Moccia M, Picillo M, Erro R, Vitale C, Longo K, Amboni M, et al. Presence and progression of non-motor symptoms in relation to uric acid in de novo Parkinson's disease. Eur J Neurol. (2015) 22:93–8. doi: 10.1111/ene.12533
41. Moccia M, Pappatà S, Erro R, Picillo M, Vitale C, Amboni M, et al. Uric acid relates to dopamine transporter availability in Parkinson's disease. Acta Neurol Scand. (2015) 131:127–31. doi: 10.1111/ane.12295
42. Sleeman I, Lawson RA, Yarnall AJ, Duncan GW, Johnston F, Khoo TK, et al. Urate and homocysteine: predicting motor and cognitive changes in newly diagnosed Parkinson's Disease. J Parkinsons Dis. (2019) 9:351–9. doi: 10.3233/JPD-181535
43. Heinzel S, Berg D, Gasser T, Chen H, Yao C, Postuma RB, et al. Update of the MDS research criteria for prodromal Parkinson's disease. Mov Disord. (2019) 34:1464–70. doi: 10.1002/mds.27802
44. Jankovic J, Tan EK. Parkinson's disease: etiopathogenesis and treatment. J Neurol Neurosurg Psychiatry. (2020) 91:795–808. doi: 10.1136/jnnp-2019-322338
45. Schirinzi T, Di Lazzaro G, Sancesario GM, Summa S, Petrucci S, Colona VL, et al. Young-onset and late-onset Parkinson's disease exhibit a different profile of fluid biomarkers and clinical features. Neurobiol Aging. (2020) 90:119–24. doi: 10.1016/j.neurobiolaging.2020.02.012
46. Yamamoto T, Moriwaki Y, Cheng J, Takahashi S, Tsutsumi Z, Ka T, et al. Effect of inosine on the plasma concentration of uridine and purine bases. Metabolism. (2002) 51:438–42. doi: 10.1053/meta.2002.31322
47. Schwarzschild MA, Macklin EA, Bakshi R, Battacharyya S, Logan R, Espay AJ, et al. Sex differences by design and outcome in the Safety of Urate Elevation in PD (SURE-PD) trial. Neurology. (2019) 93:e1328–38. doi: 10.1212/WNL.0000000000008194
48. Bhattacharyya S, Bakshi R, Logan R, Ascherio A, Macklin EA, Schwarzschild MA. Oral inosine persistently elevates plasma antioxidant capacity in Parkinson's disease. Mov Disord. (2016) 31:417–21. doi: 10.1002/mds.26483
49. Investigators PSGS-P, Schwarzschild MA, Ascherio A, Casaceli C, Curhan GC, Fitzgerald R, et al. Effect of urate-elevating inosine on early parkinson disease progression: The SURE-PD3 randomized clinical trial. JAMA. (2021) 326:926–39.
50. McGinley MP, Goldschmidt CH, Rae-Grant AD. Diagnosis and treatment of multiple sclerosis: a review. JAMA. (2021) 325:765–79. doi: 10.1001/jama.2020.26858
51. Wang L, Hu W, Wang J, Qian W, Xiao H. Low serum uric acid levels in patients with multiple sclerosis and neuromyelitis optica: an updated meta-analysis. Mult Scler Relat Disord. (2016) 9:17–22. doi: 10.1016/j.msard.2016.05.008
52. Drulović J, Dujmović I, Stojsavljević N, Mesaros S, Andjelković S, Miljković D, et al. Uric acid levels in sera from patients with multiple sclerosis. J Neurol. (2001) 248:121–6. doi: 10.1007/s004150170246
53. Simental-Mendía E, Simental-Mendía LE, Guerrero-Romero F. Serum uric acid concentrations are directly associated with the presence of benign multiple sclerosis. Neurol Sci. (2017) 38:1665–9. doi: 10.1007/s10072-017-3043-1
54. Moccia M, Lanzillo R, Palladino R, Russo C, Carotenuto A, Massarelli M, et al. Uric acid: a potential biomarker of multiple sclerosis and of its disability. Clin Chem Lab Med. (2015) 53:753–9. doi: 10.1515/cclm-2014-0744
55. Moccia M, Lanzillo R, Costabile T, Russo C, Carotenuto A, Sasso G, et al. (2015). Uric acid in relapsing-remitting multiple sclerosis: a 2-year longitudinal study. J Neurol. 262:961–7. doi: 10.1007/s00415-015-7666-y
56. Niu PP, Song B, Wang X, Xu YM. Serum uric acid level and multiple sclerosis: a mendelian randomization study. Front Genet. (2020) 11:254. doi: 10.3389/fgene.2020.00254
57. Junqueira SC, Coelho ID, Lieberknecht V, Cunha MP, Calixto JB, Rodrigues ALS, et al. Inosine, an endogenous purine nucleoside, suppresses immune responses and protects mice from experimental autoimmune encephalomyelitis: a role for A2A adenosine receptor. Mol Neurobiol. (2017) 54:3271–85. doi: 10.1007/s12035-016-9893-3
58. Spitsin S, Hooper DC, Leist T, Streletz LJ, Mikheeva T, Koprowskil H. Inactivation of peroxynitrite in multiple sclerosis patients after oral administration of inosine may suggest possible approaches to therapy of the disease. Mult Scler. (2001) 7:313–9. doi: 10.1177/135245850100700507
59. Toncev G. Therapeutic value of serum uric acid levels increasing in the treatment of multiple sclerosis therapeutic value of serum uric acid levels increasing in the treatment of multiple sclerosis. Vojnosanit Pregl. (2006) 63:879–82. doi: 10.2298/VSP0610879T
60. Markowitz CE. The treatment of multiple sclerosis with inosine. J Altern Complement Med. (2009) 15:619–25. doi: 10.1089/acm.2009.0104
61. Gonsette RE, Sindic C, D'hooghe MB, De Deyn PP, Medaer R, Michotte A, et al. Boosting endogenous neuroprotection in multiple sclerosis: the ASsociation of Inosine and Interferon beta in relapsing- remitting Multiple Sclerosis (ASIIMS) trial. Mult Scler. (2010) 16:455–62. doi: 10.1177/1352458509360547
62. García DM, Midaglia L, Vilela JM, Sánchez MM, González FJL, Gómez MA, et al. Associated Inosine to interferon: results of a clinical trial in multiple sclerosis. Acta Neurol Scand. (2015) 131:405–10. doi: 10.1111/ane.12333
63. Basile MS, Bramanti P, Mazzon E. Inosine in neurodegenerative diseases: from the bench to the bedside. Molecules. (2022) 27:4644. doi: 10.3390/molecules27144644
64. Wingerchuk DM, Banwell B, Bennett JL, et al. International panel for NMO diagnosis. international consensus diagnostic criteria for neuromyelitis optica spectrum disorders. Neurology. (2015) 85:177–89. doi: 10.1212/WNL.0000000000001729
65. Shu Y, Li H, Zhang L, Wang Y, Long Y, Li R, et al. Elevated cerebrospinal fluid uric acid during relapse of neuromyelitis optica spectrum disorders. Brain Behav. (2016) 7:e00584. doi: 10.1002/brb3.584
66. Min JH, Waters P, Vincent A, Lee SY, Shin H. Reduced serum uric acid levels in neuromyelitis optica: serum uric acid levels are reduced during relapses in NMO. Acta Neurol Scand. (2012) 126:287–91. doi: 10.1111/j.1600-0404.2012.01643.x
67. Xie H, Shao Y, Du J, Song Y, Li Y, Duan R, et al. Comparative analysis of clinical and imaging data between patients with myelin oligodendrocyte glycoprotein antibody disease and patients with aquaporin 4 antibody-positive neuromyelitis optica spectrum disorder. J Neurol. (2022) 269:1641–50. doi: 10.1007/s00415-021-10749-6
68. Liu C, Xu Y, Cui L, Wang J, Peng B, Zhong L, et al. Serum uric acid levels and their correlation with clinical and cerebrospinal fluid parameters in patients with neuromyelitis optica. J Clin Neurosci. (2013) 20:278–80. doi: 10.1016/j.jocn.2012.02.042
69. Hayashi N, Atsuta N, Yokoi D, Nakamura R, Nakatochi M, Katsuno M, et al. Prognosis of amyotrophic lateral sclerosis patients undergoing tracheostomy invasive ventilation therapy in Japan. J Neurol Neurosurg Psychiatry. (2020) 91:285–90. doi: 10.1136/jnnp-2019-322213
70. Gerou M, Hall B, Woof R, Allsop J, Kolb SJ, Meyer K, et al. Amyotrophic lateral sclerosis alters the metabolic aging profile in patient derived fibroblasts. Neurobiol Aging. (2021) 105:64–77. doi: 10.1016/j.neurobiolaging.2021.04.013
71. Kwon HS, Park Y, Kim JH, Kim SH, Jun JB, Park S, et al. Prevalence of motor neuron diseases in gout patients: a nationwide population-based cohort study. Neurol Sci. (2022) 22:6451. doi: 10.1007/s10072-022-06451-8
72. Tang J, Yang Y, Gong Z, Li Z, Huang L, Ding F, et al. Plasma uric acid helps predict cognitive impairment in patients with amyotrophic lateral sclerosis. Front Neurol. (2021) 12:789840. doi: 10.3389/fneur.2021.789840
73. Xu LQ, Hu W, Guo QF, Xu GR, Wang N, Zhang QJ. Serum uric acid levels predict mortality risk in male amyotrophic lateral sclerosis patients. Front Neurol. (2021) 12:602663. doi: 10.3389/fneur.2021.602663
74. Han HJ, Shin HY, Choi YC, Kim SM, Kim SW. Serum uric acid level predicts the progression of amyotrophic lateral sclerosis following treatment with edaravone. Redox Rep. (2022) 27:79–84. doi: 10.1080/13510002.2022.2051964
75. Nicholson K, Chan J, Macklin EA, Levine-Weinberg M, Breen C, Bakshi R, et al. Pilot trial of inosine to elevate urate levels in amyotrophic lateral sclerosis. Ann Clin Transl Neurol. (2018) 5:1522–33. doi: 10.1002/acn3.671
76. Euser SM, Hofman A, Westendorp RG, Breteler MM. Serum uric acid and cognitive function and dementia. Brain. (2009) 132:377–82. doi: 10.1093/brain/awn316
77. Lu N, Dubreuil M, Zhang Y, Neogi T, Rai SK, Ascherio A, et al. Gout and the risk of Alzheimer's disease: a population-based, BMI-matched cohort study. Ann Rheum Dis. (2016) 75:547–51. doi: 10.1136/annrheumdis-2014-206917
78. Du N, Xu D, Hou X, Song X, Liu C, Chen Y, et al. Inverse association between serum uric acid levels and Alzheimer's Disease Risk. Mol Neurobiol. (2016) 53:2594–9. doi: 10.1007/s12035-015-9271-6
79. Cascalheira JF, João SS, Pinhanços SS, Castro R, Palmeira M, Almeida S, et al. Serum homocysteine: interplay with other circulating and genetic factors in association to Alzheimer's type dementia. Clin Biochem. (2009) 42:783–90. doi: 10.1016/j.clinbiochem.2009.02.006
80. Khan AA, Quinn TJ, Hewitt J, Fan Y, Dawson J. Serum uric acid level and association with cognitive impairment and dementia: systematic review and meta-analysis. Age (Dordr). (2016) 38:16. doi: 10.1007/s11357-016-9871-8
81. Latourte A, Soumaré A, Bardin T, Perez-Ruiz F, Debette S, Richette P. Uric acid and incident dementia over 12 years of follow-up: a population-based cohort study. Ann Rheum Dis. (2018) 77:328–35. doi: 10.1136/annrheumdis-2016-210767
82. Xue L, Liu Y, Xue H, Xue J, Sun K, Wu L, et al. Low uric acid is a risk factor in mild cognitive impairment. Neuropsychiatr Dis Treat. (2017) 13:2363–7. doi: 10.2147/NDT.S145812
Keywords: hyperuricemia, uric acid, neurological disease, stroke, neurodegenerative disease
Citation: Otani N, Hoshiyama E, Ouchi M, Takekawa H and Suzuki K (2023) Uric acid and neurological disease: a narrative review. Front. Neurol. 14:1164756. doi: 10.3389/fneur.2023.1164756
Received: 16 February 2023; Accepted: 05 May 2023;
Published: 01 June 2023.
Edited by:
Santiago Perez-Lloret, National Scientific and Technical Research Council (CONICET), ArgentinaReviewed by:
Wooyoung Jang, Gangneung Asan Hospital, Republic of KoreaWei Yue, Tianjin Huanhu Hospital, China
Copyright © 2023 Otani, Hoshiyama, Ouchi, Takekawa and Suzuki. This is an open-access article distributed under the terms of the Creative Commons Attribution License (CC BY). The use, distribution or reproduction in other forums is permitted, provided the original author(s) and the copyright owner(s) are credited and that the original publication in this journal is cited, in accordance with accepted academic practice. No use, distribution or reproduction is permitted which does not comply with these terms.
*Correspondence: Naoyuki Otani, bmFveXVraSYjeDAwMDQwO2Rva2t5b21lZC5hYy5qcA==
†These authors have contributed equally to this work