- 1Department of Neuroscience, Central Clinical School, Monash University, Melbourne, VIC, Australia
- 2Department of Neurology, Alfred Health, Melbourne, VIC, Australia
- 3Australian Centre for the Prevention of Cervical Cancer (Formerly Victorian Cytology Service), Carlton South, VIC, Australia
- 4Centre for Epidemiology and Biostatistics, Melbourne School of Population and Global Health, University of Melbourne, Melbourne, VIC, Australia
- 5Department of Neurosciences, Eastern Health, Melbourne, VIC, Australia
There is a growing need to better understand the risk of malignancy in the multiple sclerosis (MS) population, particularly given the relatively recent and widespread introduction of immunomodulating disease modifying therapies (DMTs). Multiple sclerosis disproportionately affects women, and the risk of gynecological malignancies, specifically cervical pre-cancer and cancer, are of particular concern. The causal relationship between persistent human papillomavirus (HPV) infection and cervical cancer has been definitively established. To date, there is limited data on the effect of MS DMTs on the risk of persistent HPV infection and subsequent progression to cervical pre-cancer and cancer. This review evaluates the risk of cervical pre-cancer and cancer in women with MS, including the risk conferred by DMTs. We examine additional factors, specific to the MS population, that alter the risk of developing cervical cancer including participation in HPV vaccination and cervical screening programs.
1. Introduction
There is growing need to better understand the risk of cancer in the multiple sclerosis (MS) population, particularly with the recent and widespread introduction of highly effective immunomodulatory or immunosuppressive disease modifying therapies (DMTs) (1, 2). MS is a chronic inflammatory autoimmune disease of the central nervous system that is three times more prevalent in women and usually diagnosed between the ages of 20–40 years (3). Treatment with DMTs is usually commenced at diagnosis and continued life-long, leading to significant long-term exposure (4).
A recent scoping review highlighted gynecological cancer risk, including cervical cancer, as an important knowledge gap in the MS literature (1). The risk of cervical cancer may be altered in women with MS (wwMS). It remains unclear whether this is the result of the autoimmune condition or secondary to the DMTs used in the treatment of MS. Additionally, wwMS may be underrepresented in primary and secondary prevention programs (5–9), further contributing to the risk of cancer in this vulnerable patient population.
Almost all cervical cancer is due to an underlying persistent infection with oncogenic types of human papillomavirus (HPV) (10, 11), a very common double stranded DNA virus, that is transmitted via sexual contact (12–14). HPV is usually cleared by the immune system without symptoms within 1–2 years. Persistent infection with one of the 13 oncogenic HPV types (including the most oncogenic types, HPV 16 and HPV 18, which are associated with 70% of cervical cancers globally) increases the risk of developing cervical pre-cancerous abnormalities. Cervical pre-cancerous abnormalities are classified as cervical intraepithelial neoplasia (CIN) grade 2 or 3 (CIN3 is synonymous with carcinoma in situ), or adenocarcinoma in situ (AIS). These abnormalities also referred to as High-grade squamous intraepithelial lesions (HSIL). Pre-cancerous lesions are at risk of progression to cancer of the cervix (see Figure 1) (12, 13). Co-factors for the development of cervical cancer in the presence of persistent oncogenic HPV include smoking, high parity, oral contraceptive use, and immunocompromise. An intact immune system is necessary for adequate clearance of HPV. HPV clearance is impaired in patients who are severely immunocompromised, including patients with human immunodeficiency virus (HIV) or acquired immunodeficiency syndrome (AIDS), solid-organ transplant recipients and some autoimmune conditions (4, 15–17). The immunocompromised population are at risk of infection with multiple HPV types along with a greater diversity of HPV types (18–20).
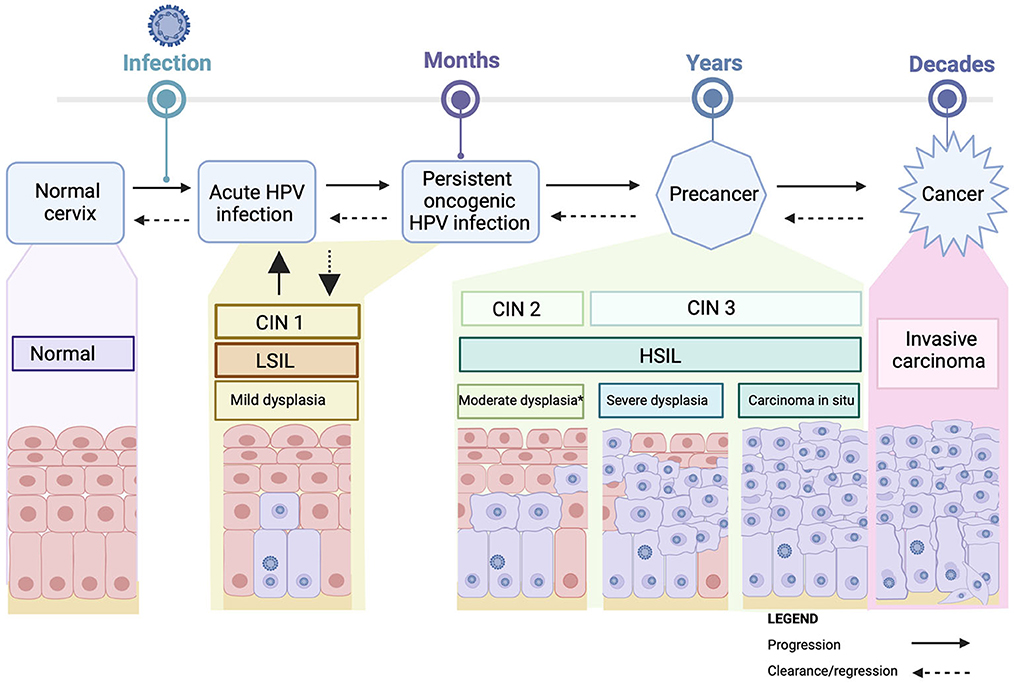
Figure 1. Cervical carcinogenesis. Acute Infection with HPV may cause mild cervical abnormalities [CIN I (mild dysplasia/LSIL)], which usually clear spontaneously. Persistent infection with oncogenic HPV can result in cervical pre-cancerous lesions [CIN 2 (moderate dysplasia), CIN3 (severe dysplasia or carcinoma in situ/HSIL)] which can progress over time to invasive cancer. Progression is not inevitable, with regression possible at any stage. *sometimes acute infections/infections with lower risk HPV types can produce an appearance that is identified as moderate disease (CIN2). CIN2 is a heterogeneous entity likely comprising “severe CIN1 cases” and “mild CIN2” cases rather than a single disease state with a uniform prognosis. HPV, human papillomavirus; CIN, cervical intraepithelial neoplasia; LSIL, low-grade squamous intraepithelial lesions; HSIL, high-grade squamous intraepithelial lesions. Created with BioRender.com.
Here we review the literature relating to the risk of cervical cancer in people with a cervix, hereby referred to as women with MS. We consider the role that immunotherapies play in altering this risk. Furthermore, we explore other factors, specific to the MS population, that may impede access to HPV vaccination and cervical screening programs and further increase patients' risk of developing cervical cancer.
2. Methodology
2.1. Literature search
We searched the PubMed database for peer-reviewed articles published in English from 1990 through October 2022 on multiple sclerosis and cervical cancer risk, using the following search strategy:
((((((“multiple sclerosis”[MeSH Terms] OR (“multiple”[All Fields] AND “sclerosis”[All Fields]) OR “multiple sclerosis”[All Fields]) AND (“uterine cervical neoplasms”[MeSH Terms] OR (“uterine”[All Fields] AND “cervical”[All Fields] AND “neoplasms”[All Fields]) OR “uterine cervical neoplasms”[All Fields] OR (“cervical”[All Fields] AND “cancer”[All Fields]) OR “cervical cancer”[All Fields])) AND (fft[Filter])) OR (((“neoplasms”[MeSH Major Topic] OR “uterine cervical neoplasms”[MeSH Major Topic]) AND “multiple sclerosis”[MeSH Major Topic]) AND ((fft[Filter]) OR ((viral)) AND (cancer)) AND (multiple sclerosis)) AND (1990:2022[pdat]))))
In addition, we hand-searched reference lists from the articles identified by the search and key review articles. We also reviewed the pivotal pharmaceutical trials for each DMT.
2.2. Selection criteria
We reviewed titles, abstracts to identify studies examining the risk of cervical abnormalities in wwMS, including the risk conferred by DMTs.
3. Risk of cervical cancer in wwMS
There is limited literature on the risk of cervical abnormalities and cancer in wwMS (see Table 1). The reported incidence of cervical cancer in wwMS ranges from 0.1–1.1 per 1,000 person years (21, 23, 24, 27, 28, 36). The heterogeneity in incidence estimates is likely due to the differences in the way the MS population was identified, study sample sizes, methods for reporting cancers, observation periods, and variation in DMT use across these studies (36, 37).
Two retrospective observational studies, one Finnish and the other Australian, evaluated the risk of cervical abnormalities in wwMS (4, 31). Women with MS were found not to be at increased risk of cervical cancer or high-grade histological and cytological abnormalities, respectively. However, it is important to consider important limitations of both studies which could impact their generalizability. Firstly, the cohorts were identified through hospital administration data (4, 31). Hospitalization is atypical for the MS population, and could represent patients with more severe disease, or significant medical comorbidities. Secondly, neither study examined the impact of DMT use, either due to lack of information, or insufficient power (4, 31).
The finding that cervical cancer risk in the MS population is not increased was supported by population-based registry studies. Again, the impact of DMTs, particularly high-efficacy therapies was not accounted for in many of these studies.
In contrast, a Norwegian nationwide cohort study found that, although the risk of female genital organ cancer was not different between wwMS and population-based controls between the years 1953–1995, the risk increased significantly from 1996–2017 (4, 21–35). They hypothesized that this may reflect the introduction of highly effective DMTs over this period (34). Again, the study did not directly explore this hypothesis.
Population-wide cohort studies have shown an increased risk of cervical abnormalities in females with autoimmune conditions including inflammatory bowel disease (IBD), systematic lupus erythematosus (SLE), and rheumatoid arthritis (RA), especially if treated with immunomodulatory therapy (4, 38). However, these conditions are not directly comparable with MS, as the diseases have different risk profiles for cervical cancer and most commonly are treated with different DMTs. While most studies in the MS population have found the incidence of cervical cancer to be equal to or less than the general population (4, 21–33, 35), there is growing concern that long-term exposure to DMTs may increase risk.
4. Risk of persistent HPV infection and cervical cancer attributable to disease modifying therapies in wwMS
The treatment of multiple sclerosis has been revolutionized over the past two decades with the introduction of highly effective DMTs (2). These therapies have transformed MS disease trajectories by significantly reducing disease activity, relapse rates and disability progression (37). Natalizumab was the first highly effective DMT to receive US Food and Drug Administration (FDA) approval in 2004, and since then there has been successive introductions of new agents, all of which target the immune system in varying ways.
While the benefit of these therapies should be emphasized, they are not without risk. High-efficacy DMTs have been found to increase the risk of opportunistic infections and, theoretically, can reduce immune surveillance and increase cancer risk (2, 39). There is potential for the impact of DMTs to be significant, given that these medications are often commenced at a young age and continued indefinitely (40).
4.1. DMTs and the risk of HPV infection
DMTs increase the risk of opportunistic infections within the MS population (39). However, there is currently insufficient data to draw conclusions on the risk of HPV infection (41).
HPV enters the basal keratinocytes of the cervical epithelium through micro-abrasions (42). As keratinocytes migrate to the upper epidermis there is increasing viral replication (14, 43). New infectious HPV is released from the surface of the epithelium. As HPV is essentially an intracellular pathogen, there is minimal HPV viraemia or lymphatic infection. This results in minimal exposure of HPV to the circulating immune system (14). However, in most cases, activation of the innate and adaptive immune systems does occur, leading to viral clearance in the majority of women (12–14, 44). DMTs alter the immune response via several mechanisms which may limit immune surveillance and clearance of the virus (Figure 2). During persistent infections oncogenic types may integrate into the host DNA, disrupting expression of the E6 and E7 viral oncogenes, which may inactivate critical cell cycle checkpoints and increase genetic instability in the host, which over time may lead to cervical cancer (45).
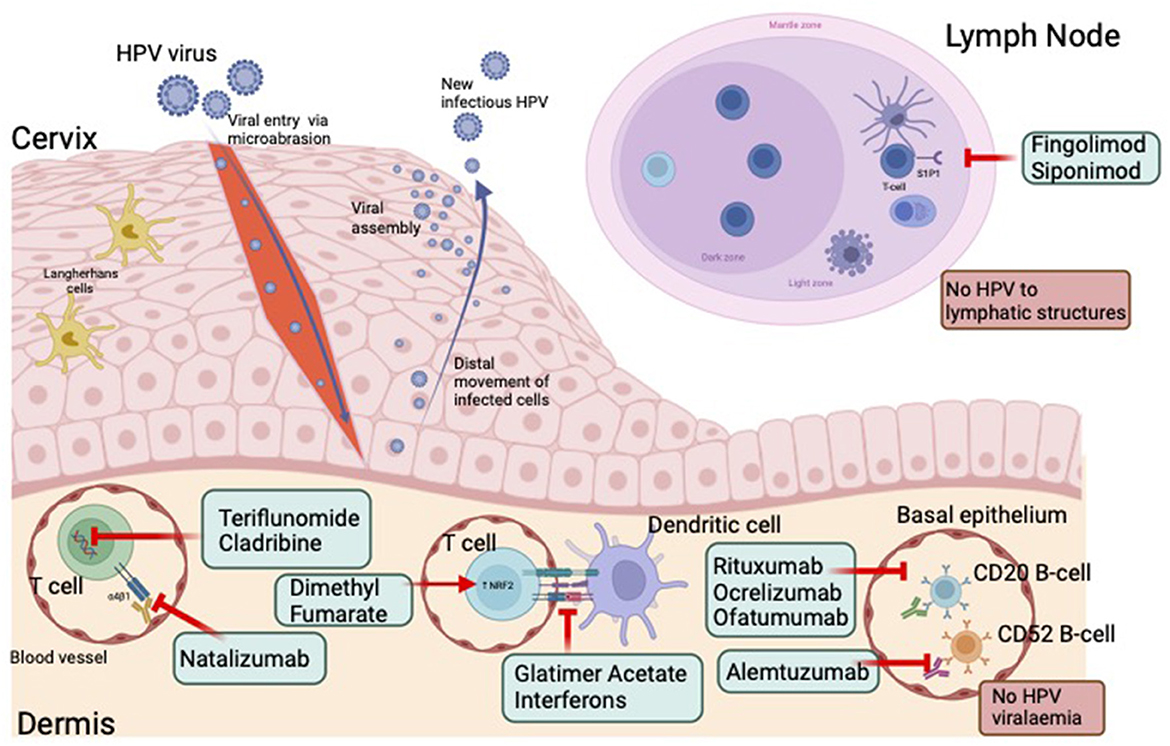
Figure 2. HPV infection, immune response and effect of disease modifying therapies. HPV enters the basal keratinocytes of the cervical epithelium through micro-abrasions. As keratinocytes migrate to the upper epidermis there is increasing viral replication. New infectious HPV is released from the surface of the epithelium. The absence of HPV viraemia or lymphatic infection, results in minimal exposure of HPV to the circulating immune system. DMTs alter the immune response via several mechanisms which may further limit immune surveillance and clearance of the virus. HPV, human papillomavirus. Created with BioRender.com.
Few studies have investigated the risk of DMTs on HPV infection. Fingolimod has been identified in a small number of case report series as being associated with a risk of HPV infection (39, 46–48). However, this finding has not been validated by higher quality evidence.
4.2. Disease modifying therapies and risk of cervical cancer
There is limited data on the effect of DMTs on cervical cancer risk in the MS population (see Supplementary Table 1). At present, studies are limited by sample size, duration of follow-up, difficulty capturing representative population-based samples and complete and accurate data. Additionally, there is likely underreporting of this outcome in part due to under-participation in screening programs in the MS population (6–8, 49). Importantly cervical cancer outcomes may not be captured in the pharmaceutical safety trials, as these trials are often conducted over a shorter duration (1–2 years), and oncogenesis secondary to HPV is known to occur over decades.
Studies to date have found conflicting results regarding the risk of overall cancer associated with DMTs. Most studies have reported no increased risk of cancer (22, 50–55), while others have found an increased cancer risk (22, 50–56). Individual classes of DMTs have also been associated with an increased (26, 57), or a reduced risk of cancer (58). The discrepancy is likely in part explained by study design, with many observational studies grouping therapies, for example as immunomodulating vs. immunosuppressing, in order to improve statistical power. However, many of these therapies have different mechanisms of action and thus different risk profiles. Across studies there is also a lack of uniformity as to which therapies are included. Several studies have included therapies such as azathioprine and cyclophosphamide in the analysis for immunosuppressive therapies (22, 26, 50, 51, 53, 55, 56). These therapies have been widely reported to increase the risk of cancer (59, 60), and are seldom used in the modern-day treatment of MS, thereby likely skewing results.
A further challenge is that patients with MS often switch DMTs throughout their life. This makes quantifying the risk attributable to individual DMTs difficult. Additionally, it poses challenges for calculating the risk for an individual who may have been exposed to several different therapies. The cumulative risk is likely the product of the combination of therapies used, along with the duration of exposure to each therapy (26, 50, 51).
Age at exposure to DMT also likely impacts risk. Similar to the general population, cancer risk in patients with MS increases with advancing age, likely in part due to weakening of the immune system (50, 61). Evidence suggests an additive effect from DMT exposure with several DMTs including cladribine, anti-CD20, alemtuzumab and sphingosine-1-phosphate modulators increasing the risk of malignancy with age (61). However, while aging increases cancer risk more broadly, cervical cancer incidence is highest in younger populations (25–50 years) (62). The impact of aging on cervical cancer risk in the MS population remains unclear but may have implications for long-term malignancy surveillance, and DMT counseling.
Despite the lack of definitive evidence to confirm the role of DMTs in the development of cancer, DMTs including cladribine, fingolimod, natalizumab, alemtuzumab, and ocrelizumab all carry a warning for potential cancer risk (34, 63). It is a requirement that all patients treated with these medications undergo cancer surveillance (40).
The cancer incidence among patients treated in the modern-DMT era was examined in a French study that identified 9,269 patients with MS who had been exposed to DMTs from two population-based disease registers and these were linked to patient records in the French National Cancer Register (26). DMTs were categorized into two groups: immunomodulatory drugs and immunosuppressive drugs. Interferons and glatiramer acetate were considered immunomodulatory therapies, whereas azathioprine, mitoxantrone, mycophenolate mofetil, natalizumab, methotrexate, fingolimod, cladribine and teriflunomide were classified as immunosuppressive therapies. For this analysis, all gynecological cancers, including ovarian, cervical, and uterine were grouped together. WwMS treated with DMTs had a non-significantly increased risk of gynecological cancers (SIR 1.2; CI 0.8–1.9). The risk of “all cancer” was increased if the patient had been exposed to more than three types of immunosuppressive drugs, or more than two types of immunomodulatory drugs. The risk of cancer was increased with increased duration of exposure to DMTs (P < 0.001). The mean duration of treatment with immunosuppressive drugs was 4.9 +/- 4.5 years for patients with MS and cancer and 3.6 +/- 4.5 years for patients with MS and no cancer. This observation period is arguably too short to see long-term implications from DMT exposure. Individual DMT analyses, identified only azathioprine and cyclophosphamide as increasing the risk of cancer (RR 1.9, CI 1.7–3.4, p = 0.4; RR = 1.9, CI 1.3–2.6, p = 0.5, respectively) (26).
5. Individual disease modifying therapies and risk of cervical abnormalities
5.1. Low efficacy DMTs
5.1.1. Interferons and glatiramer acetate
Glatiramer acetate and the interferon-beta preparations were the first available MS DMTs and were introduced in the 1990's. These therapies are now considered low-efficacy, relative to newer treatments. Interferons exert their effect on the immune system by shifting cytokine profiles toward an anti-inflammatory state and inhibition of leucocyte migration across the blood-brain barrier (64). The mechanism of action of glatiramer acetate is not fully understood, however, it is thought to inhibit T cell responses to several myelin antigens and cause a shift toward Th2 immunity (65, 66).
To date, these therapies have not been associated with an increased cervical cancer incidence in wwMS (67–73).
5.2. Moderate to high efficacy DMTs
5.2.1. Dimethyl fumarate
Dimethyl fumarate has been used in the treatment of MS since 2013 due to its proposed anti-inflammatory, immunomodulatory and oxidant properties. While the mechanism by which it exerts its effect is not fully understood, it is thought to suppress the transcription of nuclear factor-kappa B and activate the transcription of the nuclear (erythroid-derived2)-related factor (Nrf2) (74).
DEFINE, a phase three randomized control study found no increased risk of malignancy associated with the use of dimethyl fumarate, one case of cervical cancer was reported in the treatment arm, with no cases reported in the placebo arm (75). This finding was supported by a prospective observational study conducted in Spain over a 5 year period. Again they found no increased risk of malignancy, and only one case of cervical LSIL was reported (76).
5.2.2. Inhibition of lymphocyte migration: Natalizumab and sphingosine-1-phosphate receptor antagonists
5.2.2.1. Natalizumab
Natalizumab was one of the first high-efficacy DMTs and was approved by the FDA in 2004. It is a monoclonal antibody that inhibits alpha4beta1-integrin, thereby preventing T-cells from egressing from the circulation across the blood-brain barrier and into the central nervous system (77). Alpha4beta1 integrins are also located on the surface of the cervix (78) and it is postulated that alpha4beta1 inhibition prevents T-cells from being able to enter the genital mucosa resulting in impaired antimicrobial clearance (79, 80).
A possible association between natalizumab and cervical abnormalities has been described in case reports (79, 81, 82). One case series described four patients treated with natalizumab for 9–45 months who developed high-grade cervical pre-cancer. Three were diagnosed with CIN 2 and one was diagnosed with CIN 3. All four women were positive for HPV (81).
Larger studies have not supported a possible association between natalizumab and cervical cancer (83–86). A Swedish population-based registry study identified no significant risk of pre-cancer (CIN 3+) or cancer in patients treated with natalizumab compared with the general population. However, average duration of follow-up for women treated with natalizumab in this study was 3.94 years. This short duration of surveillance may not have been sufficient to capture cervical cancer outcomes (87).
AFFIRM, a stage 3 randomized, placebo-controlled trial evaluated the safety and efficacy of 627 MS patients treated with natalizumab over a 2-year period. This study identified one case of cervical carcinoma in situ in the natalizumab treatment arm (88).
5.2.2.2. Sphingosine 1-P receptor modulators: Fingolimod and siponimod
Fingolimod is a sphingosine-1-phosphate receptor modulator that blocks lymphocyte egress from lymph nodes, limiting the number of circulating T-cells in the peripheral circulation (89). Reductions in the number of circulating T-cells may have implications for immune surveillance, which is a possible mechanism for cancer development (2).
Small case series have found an increase in HPV associated lesions in patients treated with fingolimod (46, 47, 90). A case series of 16 MS patients without a previous history of HPV found that 11 women and 5 men developed HPV lesions following fingolimod initiation (46). The lesions were identified on average 4 years after commencement of fingolimod. Of the nine women who developed cervical abnormalities, five had LSIL and four had HSIL. Oncogenic HPV-16 was identified in three patients. An important limitation of this series was that most patients had been exposed to other immunomodulating therapies prior to the commencement of fingolimod (46).
A Swedish population registry-based cohort study found a borderline significant increased risk of invasive cancer in patients treated with fingolimod compared to the general population (HR 1.53, 95% CI 0.98–2.38) (87). Notably, however, they found no difference in the rates of high-grade cervical pre-cancer (CIN 3) in the fingolimod treated population compared with healthy controls. It is important to note that the average follow-up period was 3.96 years, which may not have been long enough to see an effect on cancers with a long oncogenic lag, such as HPV associated cervical cancer (87).
LONGTERMS, a phase IIIb open-label extension trial, of patients treated with fingolimod for up to 14 years identified seven cases of cervical pre-cancer (0.2%, IR 0.04) (91).
5.2.2.3. Siponimod
Similar to fingolimod, Siponimod is a sphingosine-1-phosphate receptor modulator, which prohibits the egress of immune cells from the lymph nodes into the peripheral circulation (92). It is also thought to exert anti-inflammatory and neuroprotective effects in secondary progressive MS patients (SPMS) (92). It has been FDA approved for both RRMS and SPMS (2).
The stage III randomized, placebo controlled EXPAND trial did not find a difference between the frequency of malignancies in the Siponimod cohort compared to the placebo cohort. There were 11 cases of basal cell carcinoma associated with treatment, however, this did not significantly differ from the placebo arm (93).
5.2.3. Inhibitors of DNA synthesis: Teriflunomide and cladribine
5.2.3.1. Teriflunomide
Teriflunomide inhibits the mitochondrial enzyme dihydroorotate dehydrogenase which is necessary for the synthesis of pyrimidines. This causes inhibition of DNA and RNA synthesis and affects actively dividing cells including immune T and B cells, resulting in less circulating lymphocytes (2). This may reduce immune surveillance, allowing tumorigenesis to go unchecked.
The frequency of malignancy, including cervical cancer, has not been found to be significantly increased in patients treated with teriflunomide (94–96). 9-year follow-up from the TEMSO trial, which evaluated safety and efficacy of teriflunomide, identified one case of cervical carcinoma in situ in the teriflunomide treatment arm (97).
5.2.3.2. Cladribine
Cladribine has been newly licensed for the treatment of RRMS and SPMS. It was approved by the FDA in 2019. Cladribine is a nucleoside analog that inhibits DNA synthesis and repair. This results in sustained reduction of circulating B and T lymphocytes (98).
The pharmaceutical safety trials for Cladribine identified one case of cervical carcinoma in situ (99, 100). Of note, HPV 16 was identified 3 years prior and the patient was prescribed the higher strength dose of 5.25 mg/kg (101). However, the combined safety data from three previously reported Phase III studies (CLARITY, CLARITY extension and ORACLE-MS), as well as the prospective observational PREMIERE registry for patients prescribed 3.5 mg/kg dose of cladribine, did not identify any cases of cervical pre-cancer or cancer in the cladribine cohort. Two cases of cervical carcinoma in situ were identified in the placebo group (102).
5.2.4. Monoclonal antibodies: Rituximab, ocrelizumab, ofatumumab, and alemtuzumab
5.2.4.1. Anti-CD20: Rituximab, ocrelizumab, and ofatumumab
Anti-CD20 monoclonal antibody therapies, including Rituximab, Ocrelizumab, and Ofatumumab, target CD 20 on B cells causing B cell depletion (103). B cells have an important role in the regulatory immunological response to cancer. Tumor metabolites can attract B cells to tumor sites, resulting in detection and lysis of proliferating tumor cells (104). In the absence of B cells, cancer growth may proceed unchecked (2).
Anti-CD20 therapies have not been associated with an increased incidence of invasive cancer (105–109). Class III evidence to support the safety profile of ocrelizumab was obtained by an analysis of pooled safety data from 11 clinical trials including controlled treatment, open-label extension periods of the phase II and III trials and the phase IIIb trials of ocrelizumab in patients with RRMS and SPMS. This data included 5680 patients with MS who received ocrelizumab in clinical trials. There were 18, 218 patient-years of exposure. The rate of malignancy was calculated to be 0.46 (0.37–0.57) per 100 patient-years, which is consistent with the ranges reported in epidemiologic data (110). One case of stage II cervical carcinoma was identified (110).
5.2.4.2. Alemtuzumab
Alemtuzumab is a humanized monoclonal anti-CD-52 antibody which targets B and T lymphocytes, resulting in peripheral lymphocyte depletion, along with a reduction of natural killer cells, dendritic cells, granulocytes and monocytes (111). Pharmaceutical safety trials have not found a statistically significant increased rate of malignancy compared to controls (108, 112–116). One case of cervical cancer has been reported in association with Alemtuzumab (117).
6. Other factors that may increase cervical cancer risk in wwMS
6.1. HPV vaccination
The development of HPV vaccinations and the introduction of population-wide vaccination programs have become the fundamental pillar of the WHO Global Strategy to accelerate the elimination of cervical cancer as a public health problem (118). There is emerging evidence for cancer prevention in higher Development Index countries with organized population-based vaccination programs (119–123). However, globally rates have yet to fall as the greatest disease burden remains in low-and middle-income countries with the highest populations, where vaccination programs are yet to be implemented (124–126).
HPV vaccines [including the bivalent (HPV 16, 18), quadrivalent (HPV 6, 11, 16, 18), and nonavalent (HPV 6, 11, 16, 18, 31, 33, 45, 52, 58)] are inactive and therefore safe and immunogenic for the immunocompromised population (43, 127–129). Immunocompromised women will likely benefit from vaccination, even if they have already been exposed to the virus, as vaccine induced antibodies can prevent any new infection, reinfection with previously cleared types or spread of infection throughout the genital tract in cases of reactivation of a previously controlled HPV infection. The vaccines will not, however, clear any existing HPV infection, and do not reduce the need for screening (130, 131). The relatively recent introduction of the HPV vaccination program and age criteria (in Australia, women aged younger than 27 in 2007), has meant that many women have not had access to vaccination and would likely benefit from a “catch-up” vaccine. This would preferably be administered prior to the introduction of immunomodulating therapy (46). However, in Australia this is not routinely funded, and is cost-prohibitive for many.
Current Australian guidelines recommend a two-dose schedule of the nonavalent vaccine for immunocompetent people aged 11–14 years (WHO recommends a global primary target age range for HPV vaccination of 9–14 years) (132). Three doses are advised for those who are severely immunocompromised at the time of vaccination and those aged 15 years or older at the time of the first dose (133). To our knowledge, there is no data specifically examining the MS populations response to HPV vaccination. On the basis of extrapolation from other immunocompromised patient populations, the current recommendation is that wwMS on DMTs should be treated as immunocompromised and offered a three-dose schedule (134).
6.2. Cervical screening programs
Cervical screening programs remain fundamental for cervical cancer prevention, regardless of HPV vaccination status (128, 135). Screening programs ensure early detection of pre-cancerous cervical abnormalities so that intervention can occur before progression to cervical cancer (42). The current recommendations for the Australian general population is for 5-yearly cervical screening tests (CST) for women aged 25–74 years. A CST comprises a HPV test followed by a liquid based cytology (LBC) test if oncogenic HPV is detected (133).
Many countries with organized population-based screening programs, including Australia, Canada, Sweden and North America, recommend more frequent screening for immunocompromised women compared to the general population (136–141). However, whether wwMS exposed to immunomodulating therapy are included in this recommendation is often unclear. The Cancer Council Australia's Cervical Cancer Screening guideline lists women treated with immunosuppression for autoimmune diseases as a “group that require special consideration.” They state that this group of women could be considered for screening on a 3-yearly interval, but there is no definitive recommendation for wwMS (142). No studies have specifically addressed this question.
WwMS may have reduced participation in preventative health assessments including cervical cancer screening (5–9). The risk of non-participation increases with increasing physical disability (5, 141, 143). Additionally, MS has the potential to impact cognition and mood which may negatively affect a patient's ability to access preventative health care (7).
Other barriers include physical and environmental barriers such as inaccessible medical offices, lack of transportation and difficulty with patient positioning and discomfort; time limitations as procedures such as CSTs may take longer in patients with physical limitations; and attitudinal barriers for both patients and clinicians. Physicians may hold misconceptions about the value of preventative health care due to an incorrect beliefs that physical disability precludes sexual activity, or that wwMS have a reduced life expectancy and therefore preventative screening is not required. WwMS bring their own attitudes about participation in cervical screening programs. Women may have had previous negative experiences with health care visits leading to reluctance to participate.
It is important that clinicians maintain awareness of current guidelines for immunocompromised patients and cervical screening and of new opportunities. For example, Australia's screening program now offers all people with a cervix the option of self-collection (a low-mid vaginal sample is collected by the patient or their clinician to screen for HPV, replacing the need for cervical specimen collection. This test is as accurate for the detection of CIN2+ as a clinician collected sample) (144).
It is likely that any reduced participation in screening will negatively impact on cervical cancer outcomes. A Canadian retrospective cohort study of 6,820 patients with MS found that, although the incidence of cancer was lower in the MS population, patients had a larger tumor size at diagnosis (27). They argued that increased tumor size may reflect a later cancer stage at diagnosis, possibly as a result of reduced engagement with preventative health care.
7. Conclusion
There is insufficient data regarding the risk of HPV infection and progression to cervical pre-cancer and cancer in wwMS treated with DMTs. This is particularly evident for women treated with high-efficacy DMTs. Many studies are underpowered to detect cervical pre-cancer and cancer, particularly due to insufficient follow up time to capture this serious outcome. This represents an important knowledge gap in the MS literature and understanding the risk is imperative for the health and safety of wwMS.
Establishing whether DMTs increase the risk of cervical abnormalities will allow individualized counseling for patients regarding their risk profile. It will also guide international primary and secondary prevention strategies including HPV vaccination and cervical screening programs.
More research is needed to identify and address the barriers to participation in vaccination and screening programs for wwMS. Patients and clinicians need to be aware that wwMS are vulnerable to poor participation in these programs so they can better utilize strategies to optimize engagement.
Addressing these factors will significantly impact the rates of cervical abnormalities in the MS population and will help to advance the target of eliminating cervical cancer as a public health problem globally.
Author contributions
FB: conceptualization, methodology, writing original draft, reviewing and editing, and visualization. JB and YF: writing—reviewing and editing. HB: writing—reviewing and editing and supervision. VJ and AV: conceptualization, writing—reviewing and editing, and supervision. All authors contributed to the article and approved the submitted version.
Conflict of interest
FB has received travel support from Biogen and a travel grant from the European Committee for Treatment and Research in Multiple Sclerosis. YF has received travel support from Biogen and receives grant support from MS Research Australia, National Health and Medical Research Council of Australia, Australia and New Zealand Association of Neurologists, and Avant Foundation. HB served on scientific advisory boards for Biogen, Novartis and Sanofi-Aventis and received conference travel support from Novartis, Biogen and Sanofi Aventis, and serves on steering committees for trials conducted by Biogen and Novartis received research support from Merck, Novartis, and Biogen. VJ receives research grant support from MS Research Australia and the National Health and Medical Research Council of Australia (NHMRC 1156519). AV has received travel support and served on advisory boards for Novartis, Biogen, Merck Serono, Roche and Teva, and receives grant support from the National Health and Medical Research Council of Australia.
The remaining author declares that the research was conducted in the absence of any commercial or financial relationships that could be construed as a potential conflict of interest.
Publisher's note
All claims expressed in this article are solely those of the authors and do not necessarily represent those of their affiliated organizations, or those of the publisher, the editors and the reviewers. Any product that may be evaluated in this article, or claim that may be made by its manufacturer, is not guaranteed or endorsed by the publisher.
Supplementary material
The Supplementary Material for this article can be found online at: https://www.frontiersin.org/articles/10.3389/fneur.2023.1119660/full#supplementary-material
References
1. Ross L, Ng HS, O'Mahony J, Amato MP, Cohen JA, Harnegie MP, et al. Women's health in multiple sclerosis: a scoping review. Front Neurol. (2022) 12:12417. doi: 10.3389/fneur.2021.812147
2. Melamed E, Lee MW. Multiple sclerosis and cancer: the ying-yang effect of disease modifying therapies. Front Immunol. (2020) 10:2954. doi: 10.3389/fimmu.2019.02954
3. Wallin MT, Culpepper WJ, Coffman P, Pulaski S, Maloni H, Mahan CM, et al. The Gulf War era multiple sclerosis cohort: age and incidence rates by race, sex and service. Brain. (2012) 135(Pt 6):1778–85. doi: 10.1093/brain/aws099
4. Foster E, Malloy MJ, Jokubaitis VG, Wrede CDH, Butzkueven H, Sasadeusz J, et al. Increased risk of cervical dysplasia in females with autoimmune conditions—Results from an Australia database linkage study. PLoS ONE. (2020) 15:e0234813. doi: 10.1371/journal.pone.0234813
5. Cheng E, Myers L, Wolf S, Shatin D, Cui XP, Ellison G, et al. Mobility impairments and use of preventive services in women with multiple sclerosis: observational study. BMJ. (2001) 323:968–9. doi: 10.1136/bmj.323.7319.968
6. Nosek MA, Howland CA. Breast and cervical cancer screening among women with physical disabilities. Arch Phys Med Rehabil. (1997) 78(12 Supplement 5):S39–44. doi: 10.1016/S0003-9993(97)90220-3
7. Dobos K, Healy B, Houtchens M. Access to preventive health care in severely disabled women with multiple sclerosis. Int J MS Care. (2015) 17:200–5. doi: 10.7224/1537-2073.2013-046
8. Marrie RA, Walld R, Bolton JM, Sareen J, Patten SB, Singer A, et al. Effect of comorbid mood and anxiety disorders on breast and cervical cancer screening in immune-mediated inflammatory disease. PLoS ONE. (2021) 16:e0249809. doi: 10.1371/journal.pone.0249809
9. Groome PA, Webber C, Maxwell CJ, McClintock C, Seitz D, Mahar A, et al. Multiple sclerosis and the cancer diagnosis: diagnostic route, cancer stage, and the diagnostic interval in breast and colorectal cancer. Neurology. (2022) 98:e1798–809. doi: 10.1212/WNL.0000000000200163
10. International Agency for Research on Cancer. IARC Monographs on the Evaluation of Carcinogenic Risks to Humans. A Review of Human Carcinogens Part B: Biological Agents. Vol. 100B. Lyon: IARC (2012).
11. International Agency for Research on Cancer. IARC Monographs on the Evaluation of Carcinogenic Risks to Humans: Human Papillomaviruses. Vol. 90. Lyon: IARC (2007). Available from: https://publications.iarc.fr/Book-And-Report-Series/Iarc-Monographs-On-The-Identification-Of-Carcinogenic-Hazards-To-Humans/Human-Papillomaviruses-2007 (cited October 3, 2022).
12. Tommasino M. The human papillomavirus family and its role in carcinogenesis. Semin Cancer Biol. (2014) 26:13–21. doi: 10.1016/j.semcancer.2013.11.002
13. Wang R, Pan W, Jin L, Huang W, Li Y, Wu D, et al. Human papillomavirus vaccine against cervical cancer: opportunity and challenge. Cancer Lett. (2020) 471:88–102. doi: 10.1016/j.canlet.2019.11.039
14. Stanley MA. Epithelial cell responses to infection with human papillomavirus. Clin Microbiol Rev. (2012) 25:215–22. doi: 10.1128/CMR.05028-11
15. Jamieson DJ, Duerr A, Burk R, Klein RS, Paramsothy P, Schuman P, et al. Characterization of genital human papillomavirus infection in women who have or who are at risk of having HIV infection. Am J Obstet Gynecol. (2002) 186:21–7. doi: 10.1067/mob.2002.119776
16. Kane S, Khatibi B, Reddy D. Higher incidence of abnormal pap smears in women with inflammatory bowel disease. Off J Am Coll Gastroenterol. (2008) 103:631–6. doi: 10.1111/j.1572-0241.2007.01582.x
17. Adami J, Gäbel H, Lindelöf B, Ekström K, Rydh B, Glimelius B, et al. Cancer risk following organ transplantation: a nationwide cohort study in Sweden. Br J Cancer. (2003) 89:1221–7. doi: 10.1038/sj.bjc.6601219
18. Duerr A, Kieke B, Warren D, Shah K, Burk R, Peipert JF, et al. Human papillomavirus–associated cervical cytologic abnormalities among women with or at risk of infection with human immunodeficiency virus. Am J Obstet Gynecol. (2001) 184:584–90. doi: 10.1067/mob.2001.111791
19. Shrestha S, Sudenga SL, Smith JS, Bachmann LH, Wilson CM, Kempf MC. The impact of highly active antiretroviral therapy on prevalence and incidence of cervical human papillomavirus infections in HIV-positive adolescents. BMC Infect Dis. (2010) 10:295. doi: 10.1186/1471-2334-10-295
20. Heard I, Tassie JM, Schmitz V, Mandelbrot L, Kazatchkine MD, Orth G. Increased risk of cervical disease among human immunodeficiency virus–infected women with severe immunosuppression and high human papillomavirus load. Obst Gynecol. (2000) 96:403–9. doi: 10.1097/00006250-200009000-00016
21. Møller H, Kneller RW, Boice JD, Olsen JH. Cancer incidence following hospitalization for multiple sclerosis in Denmark. Acta Neurol Scand. (1991) 84:214–20. doi: 10.1111/j.1600-0404.1991.tb04941.x
22. Moisset X, Perié M, Pereira B, Dumont E, Lebrun-Frenay C, Lesage FX, et al. Decreased prevalence of cancer in patients with multiple sclerosis: a case-control study. PLoS ONE. (2017) 12:e0188120. doi: 10.1371/journal.pone.0188120
23. Nielsen NM, Rostgaard K, Rasmussen S, Koch-Henriksen N, Storm HH, Melbye M, et al. Cancer risk among patients with multiple sclerosis: a population-based register study. Int J Cancer. (2006) 118:979–84. doi: 10.1002/ijc.21437
24. Bahmanyar S, Montgomery SM, Hillert J, Ekbom A, Olsson T. Cancer risk among patients with multiple sclerosis and their parents. Neurology. (2009) 72:1170–7. doi: 10.1212/01.wnl.0000345366.10455.62
25. Fois AF, Wotton CJ, Yeates D, Turner MR, Goldacre MJ. Cancer in patients with motor neuron disease, multiple sclerosis and Parkinson's disease: record linkage studies. J Neurol Neurosurg Psychiatry. (2010) 81:215. doi: 10.1136/jnnp.2009.175463
26. Lebrun C, Vermersch P, Brassat D, Defer G, Rumbach L, Clavelou P, et al. Cancer and multiple sclerosis in the era of disease-modifying treatments. J Neurol. (2011) 258:1304–11. doi: 10.1007/s00415-011-5929-9
27. Kingwell E, Bajdik C, Phillips N, Zhu F, Oger J, Hashimoto S, et al. Cancer risk in multiple sclerosis: findings from British Columbia, Canada. Brain. (2012) 135:2973–9. doi: 10.1093/brain/aws148
28. Hemminki K, Liu X, Ji J, Försti A, Sundquist J, Sundquist K. Effect of autoimmune diseases on risk and survival in female cancers. Gynecol Oncol. (2012) 127:180–5. doi: 10.1016/j.ygyno.2012.07.100
29. Dugué PA, Rebolj M, Hallas J, Garred P, Lynge E. Risk of cervical cancer in women with autoimmune diseases, in relation with their use of immunosuppressants and screening: population-based cohort study. Int J Cancer. (2015) 136:E711–719. doi: 10.1002/ijc.29209
30. Ajdacic-Gross V, Rodgers S, Aleksandrowicz A, Mutsch M, Steinemann N, von Wyl V, et al. Cancer co-occurrence patterns in Parkinson's disease and multiple sclerosis—Do they mirror immune system imbalances? Cancer Epidemiol. (2016) 44:167–73. doi: 10.1016/j.canep.2016.08.018
31. Hongell K, Kurki S, Sumelahti ML, Soilu-Hänninen M. Risk of cancer among Finnish multiple sclerosis patients. Mult Scler Relat Disord. (2019) 35:221–7. doi: 10.1016/j.msard.2019.08.005
32. Grytten N, Myhr KM, Celius EG, Benjaminsen E, Kampman M, Midgard R, et al. Risk of cancer among multiple sclerosis patients, siblings, and population controls: A prospective cohort study. Mult Scler. (2020) 26:1569–80. doi: 10.1177/1352458519877244
33. Johnson DK, Reynolds KM, Poole BD, Montierth MD, Todd VM, Barnado A, et al. Contribution of viral infection to risk for cancer in systemic lupus erythematosus and multiple sclerosis. PLoS One. (2021) 16:e0243150. doi: 10.1371/journal.pone.0243150
34. Grytten N, Myhr KM, Celius EG, Benjaminsen E, Kampman MT, Midgard R, et al. Incidence of cancer in multiple sclerosis before and after the treatment era– a registry- based cohort study. Mult Scler Relat Disord. (2021) 55:103209. doi: 10.1016/j.msard.2021.103209
35. Marrie RA, Maxwell C, Mahar A, Ekuma O, McClintock C, Seitz D, et al. Cancer incidence and mortality rates in multiple sclerosis: a matched cohort study. Neurology. (2021) 96:e501–12. doi: 10.1212/WNL.0000000000011219
36. Marrie RA, Reider N, Cohen J, Stuve O, Trojano M, Sorensen PS, et al. A systematic review of the incidence and prevalence of cancer in multiple sclerosis. Mult Scler. (2015) 21:294–304. doi: 10.1177/1352458514564489
37. Beiki O, Frumento P, Bottai M, Manouchehrinia A, Hillert J. Changes in the risk of reaching multiple sclerosis disability milestones in recent decades: a nationwide population-based cohort study in Sweden. JAMA Neurol. (2019) 76:665–71. doi: 10.1001/jamaneurol.2019.0330
38. Kim SC, Glynn RJ, Giovannucci E, Hernández-Díaz S, Liu J, Feldman S, et al. Risk of high-grade cervical dysplasia and cervical cancer in women with systemic inflammatory diseases: a population-based cohort study. Ann Rheum Dis. (2015) 74:1360–7. doi: 10.1136/annrheumdis-2013-204993
39. Papeix C, Donze C, Lebrun-Frénay C, Papeix C, Donzé C, Lebrun-Frénay C, et al. Infections and multiple sclerosis: recommendations from the French Multiple Sclerosis Society. Rev Neurol. (2021) 177:980–94. doi: 10.1016/j.neurol.2021.04.011
40. Rae-Grant A, Day GS, Marrie RA, Rabinstein A, Cree BAC, Gronseth GS, et al. Practice guideline recommendations summary: disease-modifying therapies for adults with multiple sclerosis: report of the guideline development, dissemination, and implementation subcommittee of the american academy of neurology. Neurology. (2018) 90:777–88. doi: 10.1212/WNL.0000000000005347
41. Moiola L, Barcella V, Benatti S, Capobianco M, Capra R, Cinque P, et al. The risk of infection in patients with multiple sclerosis treated with disease-modifying therapies: a Delphi consensus statement. Mult Scler. (2021) 27:331–46. doi: 10.1177/1352458520952311
42. Frazer IH. Prevention of cervical cancer through papillomavirus vaccination. Nat Rev Immunol. (2004) 4:46–54. doi: 10.1038/nri1260
43. Reusser NM, Downing C, Guidry J, Tyring SK, HPV. Carcinomas in immunocompromised patients. J Clin Med. (2015) 4:260–81. doi: 10.3390/jcm4020260
44. Moscicki AB, Shiboski S, Hills NK, Powell KJ, Jay N, Hanson EN, et al. Regression of low-grade squamous intra-epithelial lesions in young women. Lancet. (2004) 364:1678–83. doi: 10.1016/S0140-6736(04)17354-6
45. McBride AA, Warburton A. The role of integration in oncogenic progression of HPV-associated cancers. PLoS Pathog. (2017) 13:e1006211. doi: 10.1371/journal.ppat.1006211
46. Mhanna E, Nouchi A, Louapre C, De Paz R, Heinzlef O, Bodini B, et al. Human papillomavirus lesions in 16 MS patients treated with fingolimod: outcomes and vaccination. Mult Scler. (2021) 27:1794–8. doi: 10.1177/1352458521991433
47. Triplett J, Kermode AG, Corbett A, Reddel SW. Warts and all: Fingolimod and unusual HPV-associated lesions. Mult Scler. (2019) 25:1547–50. doi: 10.1177/1352458518807088
48. Macaron G, Ontaneda D. Clinical commentary on “Warts and all: Fingolimod and unusual HPV associated lesions”. Mult Scler. (2019) 25:1550–2. doi: 10.1177/1352458518813109
49. Huang KH, Tsai WC, Kung PT. The use of Pap smear and its influencing factors among women with disabilities in Taiwan. Res Dev Disabil. (2012) 33:307–14. doi: 10.1016/j.ridd.2011.09.016
50. D'Amico E, Chisari CG, Arena S, Zanghì A, Toscano S, Lo Fermo S, et al. Cancer risk and multiple sclerosis: evidence from a large Italian cohort. Front Neurol. (2019) 10:337. doi: 10.3389/fneur.2019.00337
51. Lebrun C, Debouverie M, Vermersch P, Clavelou P, Rumbach L, de Seze J, et al. Cancer risk and impact of disease-modifying treatments in patients with multiple sclerosis. Mult Scl Clin Lab Res. (2008) 14:399–405. doi: 10.1177/1352458507083625
52. Achiron A, Barak Y, Mitchell G, Mandel M, Pee D, Ayyagari R, et al. Cancer incidence in multiple sclerosis and effects of immunomodulatory treatments. Breast Cancer Res Treat. (2005) 89:265–70. doi: 10.1007/s10549-004-2229-4
53. Mariottini A, Forci B, Gualdani E, Romoli M, Repice AM, Barilaro A, et al. Incidence of malignant neoplasms and mortality in people affected by multiple sclerosis in the epoch of disease-modifying treatments: a population-based study on Tuscan residents. Mult Scler Relat Disord. (2022) 60:103679. doi: 10.1016/j.msard.2022.103679
54. Stamatellos VP, Siafis S, Papazisis G. Disease-modifying agents for multiple sclerosis and the risk for reporting cancer: a disproportionality analysis using the us food and drug administration adverse event reporting system database. Br J Clin Pharmacol. (2021) 87:4769–79. doi: 10.1111/bcp.14916
55. Gaindh D, Kavak KS, Teter B, Vaughn CB, Cookfair D, Hahn T, et al. Decreased risk of cancer in multiple sclerosis patients and analysis of the effect of disease modifying therapies on cancer risk. J Neurol Sci. (2016) 370:13–7. doi: 10.1016/j.jns.2016.09.005
56. Ragonese P, Aridon P, Vazzoler G, Mazzola MA, Lo Re V, Lo Re M, et al. Association between multiple sclerosis, cancer risk, and immunosuppressant treatment: a cohort study. BMC Neurol. (2017) 17:155. doi: 10.1186/s12883-017-0932-0
57. Dolladille C, Chrétien B, Peyro-Saint-Paul L, Alexandre J, Dejardin O, Fedrizzi S, et al. Association between disease-modifying therapies prescribed to persons with multiple sclerosis and cancer: a WHO pharmacovigilance database analysis. Neurotherapeutics. (2021) 18:1657–64. doi: 10.1007/s13311-021-01073-y
58. Gil-Bernal R, González-Caballero JL, Espinosa-Rosso R, Gómez-Gómez C. Potential risk of disease modifying therapies on neoplasm development and coadjutant factors in multiple sclerosis outpatients. Sci Rep. (2021) 11:12533. doi: 10.1038/s41598-021-91912-x
59. Travis LB, Curtis RE, Glimelius B, Holowaty EJ, Van Leeuwen FE, Lynch CF, et al. Bladder and kidney cancer following cyclophosphamide therapy for non-Hodgkin's lymphoma. J Natl Cancer Inst. (1995) 87:524–30. doi: 10.1093/jnci/87.7.524
60. Pasternak B, Svanström H, Schmiegelow K, Jess T, Hviid A. Use of azathioprine and the risk of cancer in inflammatory bowel disease. Am J Epidemiol. (2013) 177:1296–305. doi: 10.1093/aje/kws375
61. Graves JS, Krysko KM, Hua LH, Absinta M, Franklin RJM, Segal BM. Ageing and multiple sclerosis. Lancet Neurol. (2022) 3:S1474442222001843. doi: 10.1016/S1474-4422(22)00184-3
62. Cervical cancer incidence statistics. Cancer Research UK. (2015). Available online at: https://www.cancerresearchuk.org/health-professional/cancer-statistics/statistics-by-cancer-type/cervical-cancer/incidence (accessed Jan 17, 2023).
63. Lebrun C, Rocher F. Cancer risk in patients with multiple sclerosis: potential impact of disease-modifying drugs. CNS Drugs. (2018) 32:939–49. doi: 10.1007/s40263-018-0564-y
64. Kasper LH, Reder AT. Immunomodulatory activity of interferon-beta. Ann Clin Transl Neurol. (2014) 1:622–31. doi: 10.1002/acn3.84
65. Prod'homme T, Zamvil SS. The evolving mechanisms of action of glatiramer acetate. Cold Spring Harb Perspect Med. (2019) 9:a029249. doi: 10.1101/cshperspect.a029249
66. Schrempf W, Ziemssen T. Glatiramer acetate: mechanisms of action in multiple sclerosis. Autoimmun Rev. (2007) 6:469–75. doi: 10.1016/j.autrev.2007.02.003
67. Doosti R, Togha M, Moghadasi AN, Aghsaie A, Azimi AR, Khorramnia S, et al. Evaluation of the risk of cervical cancer in patients with Multiple Sclerosis treated with cytotoxic agents: a cohort study. Iran J Neurol. (2018) 17:64–70.
68. Bloomgren G, Sperling B, Cushing K, Wenten M. Assessment of malignancy risk in patients with multiple sclerosis treated with intramuscular interferon beta-1a: retrospective evaluation using a health insurance claims database and post-marketing surveillance data. Ther Clin Risk Manag. (2012) 8:313–21. doi: 10.2147/TCRM.S31347
69. Kappos L, Freedman MS, Polman CH, Edan G, Hartung HP, Miller DH, et al. Long-term effect of early treatment with interferon beta-1b after a first clinical event suggestive of multiple sclerosis: 5-year active treatment extension of the phase 3 BENEFIT trial. Lancet Neurol. (2009) 8:987–97. doi: 10.1016/S1474-4422(09)70237-6
70. O'Connor P, Filippi M, Arnason B, Comi G, Cook S, Goodin D, et al. 250 or 500 μg interferon beta-1b vs. 20 mg glatiramer acetate in relapsing-remitting multiple sclerosis: a prospective, randomised, multicentre study. Lancet Neurol. (2009) 8:889–97. doi: 10.1016/S1474-4422(09)70226-1
71. Reder AT, Ebers GC, Traboulsee A, Li D, Langdon D, Goodin DS, et al. Cross-sectional study assessing long-term safety of interferon-−1b for relapsing-remitting MS. Neurology. (2010) 74:1877–85. doi: 10.1212/WNL.0b013e3181e240d0
72. Wolinsky JS, Borresen TE, Dietrich DW, Wynn D, Sidi Y, Steinerman JR, et al. GLACIER: An open-label, randomized, multicenter study to assess the safety and tolerability of glatiramer acetate 40 mg three-times weekly versus 20 mg daily in patients with relapsing-remitting multiple sclerosis. Mult Scler Relat Disord. (2015) 4:370–6. doi: 10.1016/j.msard.2015.06.005
73. Kappos L, Polman CH, Freedman MS, Edan G, Hartung HP, Miller DH, et al. Treatment with interferon beta-1b delays conversion to clinically definite and McDonald MS in patients with clinically isolated syndromes. Neurology. (2006) 67:1242–9. doi: 10.1212/01.wnl.0000237641.33768.8d
74. Loewe R, Holnthoner W, Gröger M, Pillinger M, Gruber F, Mechtcheriakova D, et al. Dimethylfumarate inhibits TNF-induced nuclear entry of NF-kappa B/p65 in human endothelial cells. J Immunol. (2002) 168:4781–7. doi: 10.4049/jimmunol.168.9.4781
75. Gold R, Kappos L, Arnold DL, Bar-Or A, Giovannoni G, Selmaj K, et al. Placebo-controlled phase 3 study of oral BG-12 for relapsing multiple sclerosis. N Eng J Med. (2012) 367:1098–107. doi: 10.1056/NEJMoa1114287
76. Gómez-Moreno M, Sánchez-Seco VG, Moreno-García S, Cámara PS, Sabin-Muñoz J, Ayuso-Peralta L, et al. Cancer diagnosis in a Spanish cohort of multiple sclerosis patients under dimethylfumarate treatment. Mult Scler Relat Disord. (2021) 49:102747. doi: 10.1016/j.msard.2021.102747
77. Steinman L. Blocking adhesion molecules as therapy for multiple sclerosis: natalizumab. Nat Rev Drug Discov. (2005) 4:510–8. doi: 10.1038/nrd1752
78. Perciani CT, Jaoko W, Farah B, Ostrowski MA, Anzala O, MacDonald KS. αEβ7, α4β7 and α4β1 integrin contributions to T cell distribution in blood, cervix, and rectal tissues: potential implications for HIV transmission. PLoS ONE. (2018) 13:e0192482. doi: 10.1371/journal.pone.0192482
79. Durrieu G, Dardonville Q, Clanet M, Montastruc JL. Cervical dysplasia in a patient with multiple sclerosis treated with natalizumab. Fundam Clin Pharmacol. (2019) 33:125–6. doi: 10.1111/fcp.12394
80. Davila SJ, Olive AJ, Starnbach MN. Integrin α4β1 is necessary for CD4+ T cell-mediated protection against genital C. trachomatis infection. J Immunol. (2014) 192:4284–93. doi: 10.4049/jimmunol.1303238
81. Rolfes L, Lokhorst B, Samijn J, van Puijenbroek E. Cervical dysplasia associated with the use of natalizumab. Neth J Med. (2013) 71:494–5.
82. Wan KM, Oehler MK. Rapid progression of low-grade cervical dysplasia into invasive cancer during natalizumab treatment for relapsing remitting multiple sclerosis. Case Rep Oncol. (2019) 12:59–62. doi: 10.1159/000496198
83. Kappos L, Radue EW, O'Connor P, Polman C, Hohlfeld R, Calabresi P, et al. A placebo-controlled trial of oral fingolimod in relapsing multiple sclerosis. N Eng J Med. (2010) 362:387–401. doi: 10.1056/NEJMoa0909494
84. Cohen JA, Barkhof F, Comi G, Hartung HP, Khatri BO, Montalban X, et al. Oral fingolimod or intramuscular interferon for relapsing multiple sclerosis. N Engl J Med. (2010) 362:402–15. doi: 10.1056/NEJMoa0907839
85. Calabresi PA, Radue EW, Goodin D, Jeffery D, Rammohan KW, Reder AT, et al. Safety and efficacy of fingolimod in patients with relapsing-remitting multiple sclerosis (FREEDOMS II): a double-blind, randomised, placebo-controlled, phase 3 trial. Lancet Neurol. (2014) 13:545–56. doi: 10.1016/S1474-4422(14)70049-3
86. Lublin F, Miller DH, Freedman MS, Cree BAC, Wolinsky JS, Weiner H, et al. Oral fingolimod in primary progressive multiple sclerosis (INFORMS): a phase 3, randomised, double-blind, placebo-controlled trial. Lancet. (2016) 387:1075–84. doi: 10.1016/S0140-6736(15)01314-8
87. Alping P, Askling J, Burman J, Fink K, Fogdell-Hahn A, Gunnarsson M, et al. Cancer risk for fingolimod, natalizumab, and rituximab in multiple sclerosis patients. Ann Neurol. (2020) 87:688–99. doi: 10.1002/ana.25701
88. Polman CH, O'Connor PW, Havrdova E, Hutchinson M, Kappos L, Miller DH, et al. A randomized, placebo-controlled trial of natalizumab for relapsing multiple sclerosis. N Eng J Med. (2006) 354:899–910. doi: 10.1056/NEJMoa044397
89. Yanagawa M. Chiba. FTY720, a novel immunosuppressant, induces sequestration of circulating mature lymphocytes by acceleration of lymphocyte homing in rats, III Increase in frequency of CD62L-positive T cells in Peyer's patches by FTY720-induced lymphocyte homing. Immunology. (1998) 95:591–4. doi: 10.1046/j.1365-2567.1998.00639.x
90. Jaafar N, Zeineddine M, Massouh J, Yamout B. Skin warts during fingolimod treatment in patients with multiple sclerosis. Mult Scler Relat Disord. (2018) 26:263–4. doi: 10.1016/j.msard.2018.10.101
91. Cohen JA, Tenenbaum N, Bhatt A, Zhang Y, Kappos L. Extended treatment with fingolimod for relapsing multiple sclerosis: the 14-year LONGTERMS study results. Ther Adv Neurol Disord. (2019) 12:1756286419878324. doi: 10.1177/1756286419878324
92. Behrangi N. Link to external site this link will open in a new window, Fischbach F, Kipp M, Link to external site this link will open in a new window. Mechanism of siponimod: anti-inflammatory and neuroprotective mode of action. Cells. (2019) 8:24. doi: 10.3390/cells8010024
93. Kappos L, Bar-Or A, Cree BAC, Fox RJ, Giovannoni G, Gold R, et al. Siponimod versus placebo in secondary progressive multiple sclerosis (EXPAND): a double-blind, randomised, phase 3 study. Lancet. (2018) 391:1263–73. doi: 10.1016/S0140-6736(18)30475-6
94. Comi G, Freedman MS, Kappos L, Olsson TP, Miller AE, Wolinsky JS, et al. Pooled safety and tolerability data from four placebo-controlled teriflunomide studies and extensions. Mult Scler Relat Disord. (2016) 5:97–104. doi: 10.1016/j.msard.2015.11.006
95. Confavreux C, O'Connor P, Comi G, Freedman MS, Miller AE, Olsson TP, et al. Oral teriflunomide for patients with relapsing multiple sclerosis (TOWER): a randomised, double-blind, placebo-controlled, phase 3 trial. Lancet Neurol. (2014) 13:247–56. doi: 10.1016/S1474-4422(13)70308-9
96. Miller AE, Wolinsky JS, Kappos L, Comi G, Freedman MS, Olsson TP, et al. Oral teriflunomide for patients with a first clinical episode suggestive of multiple sclerosis (TOPIC): a randomised, double-blind, placebo-controlled, phase 3 trial. Lancet Neurol. (2014) 13:977–86. doi: 10.1016/S1474-4422(14)70191-7
97. O'Connor P, Comi G, Freedman MS, Miller AE, Kappos L, Bouchard JP, et al. Long-term safety and efficacy of teriflunomide: 9-year follow-up of the randomized TEMSO study. Neurology. (2016) 86:920–30. doi: 10.1212/WNL.0000000000002441
98. Leist TP, Weissert R. Cladribine: Mode of action and implications for treatment of multiple sclerosis. Clin Neuropharmacol. (2011) 34:28–35. doi: 10.1097/WNF.0b013e318204cd90
99. Leist TP, Comi G, Cree BAC, Coyle PK, Freedman MS, Hartung HP, et al. Effect of oral cladribine on time to conversion to clinically definite multiple sclerosis in patients with a first demyelinating event (ORACLE MS): a phase 3 randomised trial. Lancet Neurol. (2014) 13:257–67. doi: 10.1016/S1474-4422(14)70005-5
100. Giovannoni G, Soelberg Sorensen P, Cook S, Rammohan K, Rieckmann P, Comi G, et al. Safety and efficacy of cladribine tablets in patients with relapsing–remitting multiple sclerosis: results from the randomized extension trial of the CLARITY study. Mult Scler. (2018) 24:1594–604. doi: 10.1177/1352458517727603
101. Giovannoni G, Comi G, Cook S, Rammohan K, Rieckmann P, Sørensen PS, et al. A placebo-controlled trial of oral cladribine for relapsing multiple sclerosis. N Engl J Med. (2010) 362:416–26. doi: 10.1056/NEJMoa0902533
102. Leist T, Cook S, Comi G, Montalban X, Giovannoni G, Nolting A, et al. Long-term safety data from the cladribine tablets clinical development program in multiple sclerosis. Mult Scler Relat Disord. (2020) 46:102572. doi: 10.1016/j.msard.2020.102572
103. Clark EA, Ledbetter JA. How does B cell depletion therapy work, and how can it be improved? Ann Rheum Dis. (2005) 64:iv77–80. doi: 10.1136/ard.2005.042507
104. Schwartz M, Zhang Y, Rosenblatt JD, B. cell regulation of the anti-tumor response and role in carcinogenesis. J Immunother Cancer. (2016) 4:40. doi: 10.1186/s40425-016-0145-x
105. Montalban X, Hauser SL, Kappos L, Arnold DL, Bar-Or A, Comi G, et al. Ocrelizumab vs. placebo in primary progressive multiple sclerosis. N Eng J Med. (2017) 376:209–20. doi: 10.1056/NEJMoa1606468
106. Hauser SL, Bar-Or A, Comi G, Giovannoni G, Hartung HP, Hemmer B, et al. Ocrelizumab vs. interferon beta-1a in relapsing multiple sclerosis. N Engl J Med. (2017) 376:221–34. doi: 10.1056/NEJMoa1601277
107. Wolinsky JS, Arnold DL, Brochet B, Hartung HP, Montalban X, Naismith RT, et al. Long-term follow-up from the ORATORIO trial of ocrelizumab for primary progressive multiple sclerosis: a post-hoc analysis from the ongoing open-label extension of the randomised, placebo-controlled, phase 3 trial. Lancet Neurol. (2020) 19:998–1009. doi: 10.1016/S1474-4422(20)30342-2
108. Cohen JA, Coles AJ, Arnold DL, Confavreux C, Fox EJ, Hartung HP, et al. Alemtuzumab versus interferon beta 1a as first-line treatment for patients with relapsing-remitting multiple sclerosis: a randomised controlled phase 3 trial. Lancet. (2012) 380:1819–28. doi: 10.1016/S0140-6736(12)61769-3
109. Hauser SL, Bar-Or A, Cohen JA, Comi G, Correale J, Coyle PK, et al. Ofatumumab vs. teriflunomide in multiple sclerosis. N Engl J Med. (2020) 383:546–57. doi: 10.1056/NEJMoa1917246
110. Hauser SL, Kappos L, Montalban X, Craveiro L, Chognot C, Hughes R, et al. Safety of ocrelizumab in patients with relapsing and primary progressive multiple sclerosis. Neurology. (2021) 97:e1546–59. doi: 10.1212/WNL.0000000000012700
111. Rao SP, Sancho J, Campos-Rivera J, Boutin PM, Severy PB, Weeden T, et al. Human peripheral blood mononuclear cells exhibit heterogeneous CD52 expression levels and show differential sensitivity to alemtuzumab mediated cytolysis. PLoS ONE. (2012) 7:e39416. doi: 10.1371/journal.pone.0039416
112. Havrdova E, Horakova D, Kovarova I. Alemtuzumab in the treatment of multiple sclerosis: key clinical trial results and considerations for use. Ther Adv Neurol Disord. (2015) 8:31–45. doi: 10.1177/1756285614563522
113. Steingo B, Al Malik Y, Bass AD, Berkovich R, Carraro M, Fernández Ó, et al. Long-term efficacy and safety of alemtuzumab in patients with RRMS: 12-year follow-up of CAMMS223. J Neurol. (2020) 267:3343–53. doi: 10.1007/s00415-020-09983-1
114. Coles AJ, Twyman CL, Arnold DL, Cohen JA, Confavreux C, Fox EJ, et al. Alemtuzumab for patients with relapsing multiple sclerosis after disease-modifying therapy: a randomised controlled phase 3 trial. Lancet. (2012) 380:1829–39. doi: 10.1016/S0140-6736(12)61768-1
115. Guarnera C, Bramanti P, Mazzon E. Alemtuzumab: a review of efficacy and risks in the treatment of relapsing remitting multiple sclerosis. Ther Clin Risk Manag. (2017) 13:871–9. doi: 10.2147/TCRM.S134398
116. Coles AJ, Cohen JA, Fox EJ, Giovannoni G, Hartung HP, Havrdova E, et al. Alemtuzumab CARE-MS II 5-year follow-up: efficacy and safety findings. Neurology. (2017) 89:1117–26. doi: 10.1212/WNL.0000000000004354
117. Compston DAS, Selmaj KW, Lake SL, Moran S, et al. Alemtuzumab vs. interferon beta-1a in early multiple sclerosis. N Engl J Med. (2008) 359:1786–801. doi: 10.1056/NEJMoa0802670
118. Surveillace NC for IR. Impact Evaluation of Australian National Human Papillomavirus Vaccination Program. National Centre for Immunisation and Research Surveillance, Australia (2021).
119. Lei J, Ploner A, Elfström KM, Wang J, Roth A, Fang F, et al. HPV Vaccination and the risk of invasive cervical cancer. N Eng J Med. (2020) 383:1340–8. doi: 10.1056/NEJMoa1917338
120. Kjaer SK, Dehlendorff C, Belmonte F, Baandrup L. Real-world effectiveness of human papillomavirus vaccination against cervical cancer. J Natl Cancer Inst. (2021) 113:1329–35. doi: 10.1093/jnci/djab080
121. Falcaro M, Castañon Castañon A, Ndlela B, Checchi M, Soldan K, Lopez-Bernal J, et al. The effects of the national HPV vaccination programme in England, UK, on cervical cancer and grade 3 cervical intraepithelial neoplasia incidence: a register-based observational study. Lancet. (2021) 398:2084–92. doi: 10.1016/S0140-6736(21)02178-4
122. Lehtinen M, Lagheden C, Luostarinen T, Eriksson T, Apter D, Bly A, et al. Human papillomavirus vaccine efficacy against invasive, HPV-positive cancers: Population-based follow-up of a cluster-randomised trial. BMJ Open. (2021) 11:12. doi: 10.1136/bmjopen-2021-050669
123. Mix JM, Van Dyne EA, Saraiya M, Hallowell BD, Thomas CC. Assessing impact of HPV vaccination on cervical cancer incidence among women aged 15–29 years in the United States, 1999–2017: an ecologic study. Cancer Epidemiol Biomarkers Prev. (2021) 30:30–7. doi: 10.1158/1055-9965.EPI-20-0846
124. Global strategy to accelerate the elimination of cervical cancer as a public health problem. Available online at: https://www.who.int/publications-detail-redirect/9789240014107. (accessed October 5, 2022).
125. Bray F, Ferlay J, Soerjomataram I, Siegel RL, Torre LA, Jemal A. Global cancer statistics 2018: GLOBOCAN estimates of incidence and mortality worldwide for 36 cancers in 185 countries. CA Cancer J Clin. (2018) 68:394–424. doi: 10.3322/caac.21492
126. Ferlay J, Ervik M, Lam F, Colombet M, Mery L, Piñeros M, et al. Global Cancer Observatory: Cancer Today. Lyon: International Agency for Research on Cancer (2020). Available online at: https://gco.iarc.fr/today (accessed October 5, 2022).
127. Reyes S, Ramsay M, Ladhani S, Amirthalingam G, Singh N, Cores C, et al. Protecting people with multiple sclerosis through vaccination. Pract Neurol. (2020) 20:2527. doi: 10.1136/practneurol-2020-002527
128. Garland SM, Brotherton JML, Skinner SR, Pitts M, Saville M, Mola G, et al. Human papillomavirus and cervical cancer in Australasia and Oceania: risk-factors, epidemiology and prevention. Vaccine. (2008) 26 Suppl 12:M80–88. doi: 10.1016/j.vaccine.2008.05.041
129. WHO. Meeting of the Strategic Advisory Group of Experts on immunization, April 2014 – conclusions and recommendations. Wkly Epidemiol Rec. (2014) 89:221–36.
130. Clifford GM, Gonçalves MAG, Franceschi S. HPV and HIV Study group. Human papillomavirus types among women infected with HIV: a meta-analysis. AIDS. (2006) 20:2337–44. doi: 10.1097/01.aids.0000253361.63578.14
131. Garland SM, Brotherton JML, Moscicki AB, Kaufmann AM, Stanley M, Bhatla N, et al. HPV vaccination of immunocompromised hosts. Papillomavirus Res. (2017) 4:35–8. doi: 10.1016/j.pvr.2017.06.002
132. World Health Organization. Human papillomavirus vaccines: WHO position paper, May 2017–Recommendations. Vaccine. (2017) 35:5753–5. doi: 10.1016/j.vaccine.2017.05.069
133. Australian Institute of Health and Welfare. Cervical Screening in Australia 2012-2013. Cancer series no. 93. Cat. no. CAN 91. Canberra, ACT: AIHW (2015).
134. Nesbitt C, Rath L, Zhong M, Cheng AC, Butzkueven H, Wesselingh R, et al. Vaccinations in patients with multiple sclerosis: review and recommendations. Med J Aus. (2021) 214:350. doi: 10.5694/mja2.51012
135. Hall MT, Simms KT, Lew JB, Smith MA, Brotherton JM, Saville M, et al. The projected timeframe until cervical cancer elimination in Australia: a modelling study. Lancet Public Health. (2019 J) 4:e19–27. doi: 10.1016/S2468-2667(18)30183-X
136. Jeronimo J, Castle PE, Temin S, Denny L, Gupta V, Kim JJ, et al. Secondary prevention of cervical cancer: ASCO resource-stratified clinical practice guideline. J Glob Oncol. (2017) 3:635–57. doi: 10.1200/JGO.2016.006577
137. Arbyn M, Anttila A, Jordan J, Ronco G, Schenck U, Segnan N, et al. European guidelines for quality assurance in cervical cancer screening. Summ Doc Ann Oncol. (2010) 21:448–58. doi: 10.1093/annonc/mdp471
138. World Health Organization. WHO Guidelines for Screening and Treatment of Precancerous Lesions for Cervical Cancer Prevention. Geneva: World Health Organization (2013). Available online at: https://apps.who.int/iris/handle/10665/94830
139. Fontham ETH, Wolf AMD, Church TR, Etzioni R, Flowers CR, Herzig A, et al. Cervical cancer screening for individuals at average risk: 2020 guideline update from the American cancer society. CA Cancer J Clin. (2020) 70:321–46. doi: 10.3322/caac.21628
140. Regionala cancercentrum. Cervixcancer- prevention. Regionala Cancercentrum I Samverkan. (2021). Available online at: https://kunskapsbanken.cancercentrum.se/globalassets/vara-uppdrag/prevention-tidig-upptackt/gynekologisk-cellprovskontroll/vardprogram/nationellt-vardprogram-cervixcancerprevention.pdf (accessed November 15, 2022).
141. Smeltzer SC. Preventive health screening for breast and cervical cancer and osteoporosis in women with physical disabilities. Family Commun Health. (2006) 29(1S):35S−43S. doi: 10.1097/00003727-200601001-00007
142. Cancer Council Australia. National Cervical Screening Program: Guidelines for the Management of Screen-Detected Abnormalities, Screening in Specific Populations Investigation of Abnormal Vaginal Bleeding. (2021). Available online at: http://wiki.cancer.org.au/australiawiki/images/a/ad/National_Cervical_Screening_Program_guidelines_long-form_PDF.pdf
143. Becker H, Stuifbergen A, Tinkle M. Reproductive health care experiences of women with physical disabilities: a qualitative study. Arch Phys Med Rehabil. (1997) 78:S26–33. doi: 10.1016/S0003-9993(97)90218-5
Keywords: cervical cancer, disease modifying therapy (DMT), autoimmune disease (AID), multiple sclerosis (MS), human papilloma virus (HPV)
Citation: Bridge F, Brotherton JML, Foong Y, Butzkueven H, Jokubaitis VG and Van der Walt A (2023) Risk of cervical pre-cancer and cancer in women with multiple sclerosis exposed to high efficacy disease modifying therapies. Front. Neurol. 14:1119660. doi: 10.3389/fneur.2023.1119660
Received: 09 December 2022; Accepted: 24 January 2023;
Published: 10 February 2023.
Edited by:
Zsolt Illes, University of Southern Denmark, DenmarkCopyright © 2023 Bridge, Brotherton, Foong, Butzkueven, Jokubaitis and Van der Walt. This is an open-access article distributed under the terms of the Creative Commons Attribution License (CC BY). The use, distribution or reproduction in other forums is permitted, provided the original author(s) and the copyright owner(s) are credited and that the original publication in this journal is cited, in accordance with accepted academic practice. No use, distribution or reproduction is permitted which does not comply with these terms.
*Correspondence: Francesca Bridge, RnJhbmNlc2NhLmJyaWRnZUBtb25hc2guZWR1
†These authors have contributed equally to this work