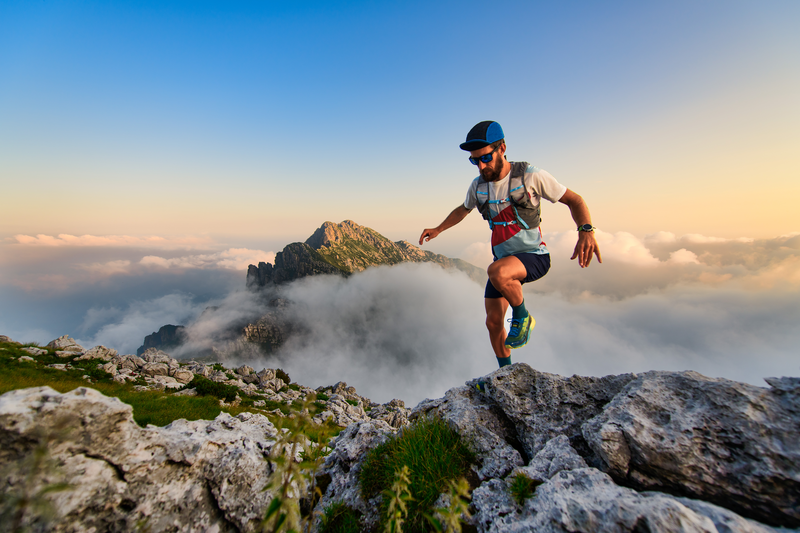
95% of researchers rate our articles as excellent or good
Learn more about the work of our research integrity team to safeguard the quality of each article we publish.
Find out more
STUDY PROTOCOL article
Front. Neurol. , 13 April 2023
Sec. Neurorehabilitation
Volume 14 - 2023 | https://doi.org/10.3389/fneur.2023.1116115
Background: Transcutaneous auricular vagus nerve stimulation (taVNS) has recently been explored for the treatment of Disorders of consciousness (DoC) caused by traumatic brain injury. The evidence of taVNS during the consciousness recovery has been recently reported. However, the mechanism of taVNS in the recovery of consciousness is not clear. This study attempts to investigate the effectiveness of taVNS in DoC by means of Coma Recovery Scale-Revised (CRS-R), Magnetic resonance imaging (MRI), Electrophysiology (EEG), and Single-molecular array (Simoa).
Methods/design: Nighty patients with DoC acquired brain injury are randomized into one of three groups receiving sham taVNS or active taVNS (just left and left or right), respectively. Each of the three groups will experience a 40 days cycle (every 10 days for a small period, baseline 2 weeks, intervention 2 weeks, 40 min per day, 5 days per week, then no intervention for 2 weeks, intervention 2 weeks, 40 min per day, and 5 days per week). Primary outcomes (CRS-R) will be recorded five times during every period. Secondary outcomes will be recorded at the first and at the last period [MRI, EEG, Phosphorylated tau (P-tau), and Neurofilament light chain (NFL)]. We will take notes the adverse events and untoward effects during all cycles.
Discussion: Transcutaneous auricular vagus nerve stimulation as a painless, non-invasive, easily applied, and effective therapy was applied for treatment of patients with depression and epilepsy several decades ago. Recent progress showed that taVNS has behavioral effects in the consciousness recovery. However, there is no clinical evidence to support the effects of taVNS on brain activity. Therefore, we will design a randomized controlled trial to evaluate the effectiveness and safety of taVNS therapy for DoC, and explore neural anatomy correlated to taVNS during the consciousness recovery. Finally, this protocol also tests some biomarkers along with the recovery of consciousness.
Clinical Trial Registration: Chinese Clinical Trial Registry, ChiCTR2100045161. Registered on 9 April 2021.
Acquired brain injury is often known as a “silent epidemic disease,” which is a general term for all brain injuries (1, 2). Acquired brain injury includes infection, hypoxia, and trauma, which can cause systemic or focal injury, and a series of pathophysiological changes (such as brain hematoma, brain contusion, and diffuse axonal injury). There are about 1.7 million Traumatic brain injury (TBI) events occur every year in the United States (3). But in China, there are no accurate epidemiological investigation data. The survival rate of Disorders of consciousness (DoC) patients after severe brain injury has been significantly increased due to advanced medical treatment and healthcare (4). However, it caused the heavy economic burden and great mental stress for family members. Currently, early assessment and health management of DoC are facing a great challenge because of its unclear pathophysiological nature. Understanding the biological basis of DoC would have important implication for the development of diagnostic and therapeutic strategies.
Dysfunction of the brain in certain areas, combined with the disruption of the neural connections between and within neural networks, can lead to DoC (4). DoC patients are in coma kept closed eyes, lack of arousal, and awareness (5), and do not respond to environment. Unresponsive wakefulness syndrome (UWS) of DoC (previously called Vegetative State, VS) is defined by some intermittent spontaneous arousal observed after tactile, auditory, or painful stimulation (1), but these DoC patients in no self-consciousness cannot consciously interact with their surrounding environment (6). Minimally consciousness state (MCS) patients have arousal and the ability to consciously interact with the environment, such as visual tracking, object localization; reaching, or instruction following (6). These changes are unstable but reproducible. MCS can be further divided into minus (MCS−) or plus (MCS+) (7). While consciousness is fluctuating in MCS, it is still possible to have the residual consciousness behavior can be monitored by careful assessment.
To date, there is no single effective way to predict, evaluate, and intervene in early severe brain injury (2), Therefore, exploring accurate assessment methods and effective intervention strategies for DoC are urgently required in this emerging fields. Transcutaneous auricular vagus nerve stimulation (taVNS) is a potential intervention in treatment of DoC. Certain visceral representative areas of the ear region produce brainstem-to-central neural modulation effects similar to invasive vagus and neural stimulation (8, 9). Because taVNS is non-invasive and has no need for surgical condition (8, 9), low cost and easy to perform for DoC patients in families or communities. It has been shown that taVNS can produce a beneficial effect in DoC (4), but the underlying mechanism is unclear.
The auricular branch of the vagus nerve is the source of sensory information to the ear. The external ear’s central area contains sensory fibers, which are situated close to the Cymba Concha section of the auricle. The auricular vagus nerve’s afferent neurons go to the inferior vagal ganglion, and then the impulse is sent to the solitary tract nucleus in the brainstem. The solitary tract is the primary receptor of sensory information from different branches of the vagus nerve, which then sends out various signals to other parts of the body, such as the locus coeruleus. This area of the reticular activating system is a major source of adrenaline-inducing projections that reach the cortex, subcortex, and brainstem. It appears that the activation of the locus coeruleus may be the cause of many of the observed therapeutic outcomes from VNS and taVNS (10). The firing of neurons in the locus coeruleus causes a massive release of norepinephrine in the thalamus and hippocampus, which is an essential part of the noradrenergic pathway and is crucial for alertness, arousal, and the fight-or-flight reaction (11). The vagus nerve in humans stimulates metabolic processes in the forebrain, thalamus, and reticular formation, and it also regulates activity in the brainstem, as well as the nucleus of the solitary tract (NTS), dorsal raphe nuclei, amygdala, and hippocampus (11).
Based on our current understanding of taVNS related to the awareness-related neural pathways, vagal cortical pathway model has been proposed (4). The vagal cortical pathway model through six distinct strategies to influence brain activity and consciousness: boosting the excitability of the ascending reticular activating system, activating the thalamus, reconstructing the cortical striatal-thalamic-cortical network, establishing negative connections between external and default mode networks by stimulating salience networks, stimulating the norepinephrine pathway to raise external network activity and connectivity, and raising excitability within the default mode network through the serotonin pathway (4). This model was constructed to investigate the mechanism of action that taVNS exerts neural modulatory effects as therapeutic intervention in the management of DoC. Therefore, the vagal cortical pathway model should be demonstrated in prospective randomized controlled clinical trials. This current study, with its randomly chosen participants, seeks to (1) gauge the effectiveness of taVNS in DoC; (2) confirm or refute vagal cortical pathway model (10, 12, 13); and (3) identify biomarkers for diagnosis and targets for therapeutic intervention (2).
Ninety patients suffering from DoC will be chosen for this prospective, randomized trial which involves double-blinded assessments. The participants will be randomly divided into the intervention and control groups with an even distribution. The control group will be administered ear lobe therapy, in contrast to the intervention group which will receive the active taVNS. We will stimulate left cymba conchae for one group, the second group subjects will be stimulated left cymba 20 min and right cymba 20 min once a day. Because we have not seen the side effects from the clinical practice, and the right vagus nerves modulate the left hemisphere. This study will last 40 days and a half-year observation period will follow. CRS-R behavioral improvement will be monitored five times every 10 days. At the beginning and the end of the fourth period, the following tests will be carried out: EEG, MRI, and measurement of certain biomarkers related to brain injuries. All side effects during each treatment will be documented. This study will be implemented in Shanghai Yongci Hospital, and rehabilitation therapists are held accountable for following the standard operating procedure and evaluating the trial’s progress at all clinical sites. This trial is illustrated in Figure 1.
Participants will be recruited from the neurologic care unit. The study will be advertised at the bedside between April 2021 and December 2022. Researchers will select potential candidates by reviewing their medical records in the Computerized Patient Record System (CPRS) and communicate the details of this trial to their families. Following the agreement of the participants’ guardians, a neurologist will review them based on the eligibility criteria. Those who meet the criteria have been invited to join the experiment and must go through a neurological examination before being evaluated for rehabilitation. All study participants must give permission by their family members.
Patients who meet the following criteria will be deemed eligible for the trial: (1) age > 18 years, onset >28 days; (2) patients were assessed by using CRS-R (five times) as DoC (UWS, MCS−, and MCS+); (3) patients had not received central stimulants drugs within 48 h before enrollment; (4) no neuromuscular blockers were applied within 24 h prior to enrollment; and (5) no facial or ear pain, no recent ear-related injuries, no metallic prosthetics, and no pregnancy. Patients who meet the following criteria will be excluded from the trial: (1) personal or familial history of seizures and history of cardiovascular disease, with developmental neurological or psychiatric illness history; (2) untreated brain edema; (3) metal implants in the body; (4) unstable vital signs; and (5) contraindications: such as claustrophobia.
The main outcome index of this study is the CRS-R scale score, which is set as a paired experiment count, the sample estimation formula is as follow:
According to the results of previous studies (4), the estimated difference standard deviation is 15, and the difference is 10, set two-sided = 0.05, and the grasp degree is 90%. According to the sample size calculation formula, 24 subjects were needed. Considering the loss of visits and refusal, at least 30 subjects were needed. Therefore, 30 VS/UWS and MCS patients will be randomly selected for each sample group, with a total sample size of 90.
The study will utilize block randomization. The participants will be classified into either the “MCS group” or the “UWS/VS group.” All subjects will be randomly divided into the intervention group and control group in a 1:1:1 ratio. To ensure impartiality, a random number will be used to produce a random allocation order, this task will be handled by a person who is not involved in the trial. The order of random allocation will remain undisclosed to those assessing the outcomes and the statistician. After evaluating the baseline information of the suitable participants, their healthcare personals and therapists (including acupuncturists and cognitive therapists) will not know patients random number assigned.
Ninety patients will undergo two sessions 10 days apart, with CRS-R and EEG performed before and after VNS. The active session will stimulate the left or right cymba conchae and inner tragus (TENS-200A, SN number: E200A16A000144, the B mode type; stimulation parameters: output pulse width degree of 200us, 20 Hz output 7 s, 4 Hz output, and 3 s alternate cycle output). The taVNS stimulator will be monitored according to patients’ response. The sham stimulation will be applied over the earlobe. We expect behavioral and EEG metrics to be improved in a majority of patients (See Figure 2).
The clinical trial concluded 3 months ago and since then, behavioral scale data have been gathered at regular intervals of 3, 6, and 12 months. For eligible subjects, the MRI/EEG will be performed, and blood samples will be collected for target protein determination.
The trial has been designed to be double-blind; thus, an external party that is unaffiliated with the experiment will monitor and oversee the execution of the blinding protocol, with the random numbers placed in a sealed envelope. Evaluators are not involved in the process of recruiting and managing patients, while other investigators, operators, and statisticians act on their own. Prior to the conclusion of the statistical analysis, unblinding is done to counteract the analyst’s potential subjectivity in data analysis; meantime, the analyst knew that the patients have been split into three groups, but was unaware of which one is the intervention group. Following the statistical study, the second step of unblinding is executed to identify which group is the treatment group.
The Ethics Committee of Hangzhou Normal University [NO: (20190083)] and Shanghai Yongci Hospital [No: (YCYY-20211021-003) have both given the green light for the study protocol and consent forms, respectively]. We will ensure that we have the approval of family members of subjects before proceeding.
This study will collect descriptive data including gender, age, onset, weight, etiology etc. before randomization. The CRS-R was used to evaluate DoC’s consciousness level, and this will be applied initially to check if the three groups are the same prior to the intervention.
The primary outcome for this study, coma recovery as measured by the Coma Recovery Scale-Revised (CRS-R), will be assessed at five time points for each period, ranging from the first to the fourth. This data will be used to determine the efficacy of the intervention being studied. The secondary outcomes of this study will be measured at both the baseline and the end of the fourth week of the study. Specifically, EEG, MRI, P-tau, and NFL will be used to measure these outcomes. These measurements will provide valuable insight into the effects of the study and help to further understand the results. Any detrimental outcomes or side effects will be noted during the course of the treatment.
The CRS-R is used to measure the progress of individuals suffering from DoC (UWS,MCS−, and MCS+) (7). The CRS-R is made up of 23 components organized into six sections that evaluate auditory, visual, motor, oromotor, communication, and arousal functions. Scores that are higher indicate conscious-related behavior, while lower scores signify reflexive activity. The evaluation of performance is dependent on the occurrence or lack of the specified action in response to standard prompts (14).
The benchmark for assessing levels of consciousness is the CRS-R (15, 16), whereas EEG is a crucial diagnostic and prognostic tool for patients with DoC (17). Recent advancements in the field have enabled researchers to develop feature-based measures like spectral power analysis, functional connectivity, and complexity measures, for the purpose of diagnosing and predicting outcomes (18–20). Despite this, their use to assess the efficacy of treatments has not been implemented (More EEG details are shown in Supplementary material). In this study, we would analyze spectral power analysis mostly to identify an improvement on EEG (such as alpha power) (17).
In this study, rest Fmri research must always include a brainstem assessment in order to verify the Vagal Cortical Pathways model’s pathways. This can be confidently relied upon as a guarantee of quality in taVNS studies, as it clearly demonstrates the stimulation of the vagus nerve (4). Furthermore, subcortical regions like the thalamus and striatum should be taken into account. Brain connectivity can be estimated by utilizing the thalamus, frontal lateral cortex, anterior cingulate cortex, insula, and posterior cingulate cortex as seed regions in Fmri studies. Moreover, measuring brain activity during tasks should be the preferred approach (21, 22). In severe TBI, an increase in fractional anisotropy (FA) on the initial postinjury diffusion tensor imaging (DTI) was associated with a favorable outcome, and these results suggest that it is secondary to axonal regrowth during later recovery. A variety of other cognitive and functional outcomes in mild to severe TBI have been correlated to DTI (23). Finally, DTI-FA should be analyzed in our study (More MRI details are shown in Supplementary material).
The peripheral blood will be taken regularly according to the experimental protocol, imaging samples will be selected, and the target protein determination will be performed using Simoa HD-1 (Single-Molecule Array Analyzer), known as the digital ELISA with more than 1,000 times higher sensitivity than conventional ELISA (24).
The enrolled subjects will be collected peripheral venous blood before and after the taVNS intervention. Samples with obvious imaging effects will be selected, and the target protein (p-tau, NFL) will be determined using Simoa HD-1 Analyzer to explore whether the target protein levels changed before and after taVNS intervention and whether this change is correlated with consciousness (25).
Main statistical analyses will be performed at the individual and group levels (pre vs. post, treatment vs. sham) (26). To examine the differences between the groups, we shall employ the t-test or Mann–Whitney test for continuous variables and the Pearson Chi-squared or Fisher’s exact test for discrete values. If a statistically significant result appears, the inequality factors will be considered as potential confounding factors in the final analysis of effectiveness. To account for any potential external influences, linear models or linear regression will be implemented on continuous dependent variables and logistic regression models on categorical dependent variables. The Chi-square test or Fisher’s exact test will be employed to document and assess unfavorable events (26). We will test outcomes on behavior (diagnosis, CRS-R score) and brain data (MRI/EEG). The proportion of responders and the effect size of treatment (r, Cohen’s d) will be calculated. We will use t-tests, Chi-square tests, ANOVA (for normally distributed data), and Wilcoxon test (for non-ordinal data), and mixed-effect regression models, depending on the tested outcomes. EEG and MRI analysis will be evaluated using SPM12 software1 and EEGLAB2 implemented in MATLAB 9.5 (MathWorks, Natick, MA, United States), respectively. All other statistical analyses will be conducted in SPSS software (version 25). All data will be adjusted to account for multiple comparisons and considered statistically significant if the value of p is lower than 0.05.
To date, no intervention strategy could provide evidence-based grade I evidence for patients with DoC patients (27). In 2018, we reported the effectiveness of peripheral sensory stimulation in patients with consciousness disorders (28). Vagus nerve stimulation is an important part of modern acupuncture and sensory stimulation. In our study, we observed behavioral improvement and brain activity in patients receiving sensory stimulation intervention (28). New investigations have demonstrated that stimulating the vagus nerve has an effect on DoC (11, 29–31). Despite this, the manner in which vagus nerve stimulation influences consciousness remains unclear.
Evidence has shown that there are three important structural bases for consciousness: the brainstem ascending reticular activation system (ARAS) (32), the central thalamus (33), and the posterior cingulate cortex (4). In addition, some brain networks are equally important for the recovery of consciousness, and understanding these brain neural correlates is critical for understanding the mechanism of action of taVNS. These neural networks include the default mode (DMN) (34), external parietal (ExN) (35), and salience network (SN) (36, 37). To better understand the mechanism of taVNS, the connection between these neural networks and brain structure must be clarified. Based on the central thalamus (lamina nucleus and related para-nucleus) and its main connection to the striatum and frontal cortex, the Mesocircuit model has been suggested as a potential explanation for the return of consciousness following severe head trauma (38, 39). Under this hypothesis, striatal dysfunction suppresses the central thalamic excitability state, which, in turn, results in a disruption of its excitatory cortical projection, and ultimately led to the DoC (4). The model states that the striatum suppresses the pallidus, which, in turn, suppresses the thalamus and pedunculopontine nucleus, and the striatum is sensitive to hypoxia, resulting in inhibition of the thalamus (4). It further disrupted the loop and exacerbated the inhibitory state (4). PET glucose metabolism showed significantly higher levels of the DoC medial pallidum compared to healthy subjects and lower levels in the central thalamus (4). Additionally, DoC patients showed a marked difference in the fractional anisotropy between the striatum and the pallidum in the left hemisphere compared to healthy controls (4). Moreover, the central loop model incorporates possible mechanisms of various intervention strategies that can explain the potential neuron conduction pathways to prevent DoC (8, 24, 40–45).
The goal of this protocol is to examine the consequences and operational aspects of taVNS in individuals suffering from DoC. The double-blind test design was implemented to corroborate the test outcomes. Additionally, an array of evaluation indexes including the CRS-R, EEG, MRI, and chemicals were employed to accurately and objectively gauge any alterations in the patient’s consciousness level (46). Ultimately, new medical data on the application of taVNS in DoC patients will be available.
The trial is anticipated to conclude by December 2023. Enrollment of participants commenced in April 2021.This protocol has a version number of ChiCTR2100045161.
The studies involving human participants were reviewed and approved by Hangzhou Normal University. Written informed consent to participate in this study was provided by the participants’ legal guardian/next of kin. Written informed consent was obtained from the individual(s), and minor(s)’ legal guardian/next of kin, for the publication of any potentially identifiable images or data included in this article.
LC conceived and designed the study. LC and LS wrote the protocol and manuscript. FZ, LX, XL, and AW helped with the experiments. Y-SC and HD advised the experiments and revised the manuscript. All authors contributed to the article and approved the submitted version.
This work was supported by the Department of Education of Zhejiang Province (Grant no. Y202147477), the Chen Xiaoying Education Fund of Hangzhou Normal University Qianjiang College (Grant no. 4065J2051911001) and National Natural Science Foundation of China (Grant no. 81920108023).
The authors declare that the research was conducted in the absence of any commercial or financial relationships that could be construed as a potential conflict of interest.
All claims expressed in this article are solely those of the authors and do not necessarily represent those of their affiliated organizations, or those of the publisher, the editors and the reviewers. Any product that may be evaluated in this article, or claim that may be made by its manufacturer, is not guaranteed or endorsed by the publisher.
The Supplementary material for this article can be found online at: https://www.frontiersin.org/articles/10.3389/fneur.2023.1116115/full#supplementary-material
1. Peeters, W, van den Brande, R, Polinder, S, Brazinova, A, Steyerberg, EW, Lingsma, HF, et al. Epidemiology of traumatic brain injury in Europe. Acta Neurochir. (2015) 157:1683–96. doi: 10.1007/s00701-015-2512-7
2. Wang, KK, Yang, Z, Zhu, T, Shi, Y, Rubenstein, R, Tyndall, JA, et al. An update on diagnostic and prognostic biomarkers for traumatic brain injury. Expert Rev Mol Diagn. (2018) 18:165–80. doi: 10.1080/14737159.2018.1428089
3. Flanagan, G, Velez, T, Gu, W, and Singman, E. The relationship between severe visual acuity loss, traumatic brain injuries, and ocular injuries in American service membersfrom 2001 to 2015. Mil Med. (2020) 185:e1576–83. doi: 10.1093/milmed/usaa154
4. Briand, M-M, Gosseries, O, Staumont, B, Laureys, S, and Thibaut, A. Transcutaneous auricular vagal nerve stimulation and disorders of consciousness: a hypothesis for mechanisms of action. Front Neurol. (2020) 11:933. doi: 10.3389/fneur.2020.00933
5. Sakusic, A, and Rabinstein, AA. Acute coma. Neurol Clin. (2021) 39:257–72. doi: 10.1016/j.ncl.2021.01.001
6. Laureys, S, Celesia, GG, Cohadon, F, Lavrijsen, J, León-Carrión, J, Sannita, WG, et al. Unresponsive wakefulness syndrome: a new name for the vegetative state or apallic syndrome. BMC Med. (2010) 8:68. doi: 10.1186/1741-7015-8-68
7. Bruno, MA, Vanhaudenhuyse, A, Thibaut, A, Moonen, G, and Laureys, S. From unresponsive wakefulness to minimally conscious PLUS and functional locked-in syndromes: recent advances in our understanding of disorders of consciousness. J Neurol. (2011) 258:1373–84. doi: 10.1007/s00415-011-6114-x
8. Badran, BW, Dowdle, LT, Mithoefer, OJ, LaBate, NT, Coatsworth, J, Brown, JC, et al. Neurophysiologic effects of transcutaneous auricular vagus nerve stimulation (taVNS) via electrical stimulation of the tragus: a concurrent taVNS/FmrI study and review. Brain Stimul. (2018) 11:492–500. doi: 10.1016/j.brs.2017.12.009
9. Rong, PJ, Fang, JL, Wang, LP, Meng, H, Liu, J, Ma, YG, et al. Transcutaneous vagus nerve stimulation for the treatment of depression: a study protocol for a double blinded randomized clinical trial. BMC Complement Altern Med. (2012) 12:255. doi: 10.1186/1472-6882-12-255
10. Butt, MF, Albusoda, A, Farmer, AD, and Aziz, Q. The anatomical basis for transcutaneous auricular vagus nerve stimulation. J Anat. (2020) 236:588–611. doi: 10.1111/joa.13122
11. Corazzol, M, Lio, G, Lefevre, A, Deiana, G, Tell, L, André-Obadia, N, et al. Restoring consciousness with vagus nerve stimulation. Curr Biol. (2017) 27:R994–6. doi: 10.1016/j.cub.2017.07.060
12. Rangon, CM. Reconsidering sham in transcutaneous vagus nerve stimulation studies. Clin Neurophysiol. (2018) 129:2501–2. doi: 10.1016/j.clinph.2018.08.027
13. Conio, B, Martino, M, Magioncalda, P, Escelsior, A, Inglese, M, Amore, M, et al. Opposite effects of dopamine and serotonin on resting-state networks: review and implications for psychiatric disorders. Mol Psychiatry. (2020) 25:82–93. doi: 10.1038/s41380-019-0406-4
14. Giacino, JT, Kalmar, K, and Whyte, J. The JFK coma recovery scale-revised: measurement characteristics and diagnostic utility. Arch Phys Med Rehabil. (2004) 85:2020–9. doi: 10.1016/j.apmr.2004.02.033
15. Di, H, He, M, Zhang, Y, Cheng, L, Wang, F, Nie, Y, et al. Chinese translation of the coma recovery scale-revised. Brain Inj. (2017) 31:363–5. doi: 10.1080/02699052.2016.1255780
16. Seel, RT, Sherer, M, Whyte, J, Katz, DI, Giacino, JT, Rosenbaum, AM, et al. Assessment scales for disorders of consciousness: evidence-based recommendations for clinical practice and research. Arch Phys Med Rehabil. (2010) 91:1795–813. doi: 10.1016/j.apmr.2010.07.218
17. Ballanti, S, Campagnini, S, Liuzzi, P, Hakiki, B, Scarpino, M, Macchi, C, et al. EEG-based methods for recovery prognosis of patients with disorders of consciousness: a systematic review. Clin Neurophysiol. (2022) 144:98–114. doi: 10.1016/j.clinph.2022.09.017
18. Piarulli, A, Bergamasco, M, Thibaut, A, Cologan, V, Gosseries, O, and Laureys, S. EEG ultradian rhythmicity differences in disorders of consciousness during wakefulness. J Neurol. (2016) 263:1746–60. doi: 10.1007/s00415-016-8196-y
19. Chennu, S, Annen, J, Wannez, S, Thibaut, A, Chatelle, C, Cassol, H, et al. Brain networks predict metabolism, diagnosis and prognosis at the bedside in disorders of consciousness. Brain J Neurol. (2017) 140:2120–32. doi: 10.1093/brain/awx163
20. Engemann, DA, Raimondo, F, King, JR, Rohaut, B, Louppe, G, Faugeras, F, et al. Robust EEG-based cross-site and cross-protocol classification of states of consciousness. Brain J Neurol. (2018) 141:3179–92. doi: 10.1093/brain/awy251
21. Stender, J, Gosseries, O, Bruno, MA, Charland-Verville, V, Vanhaudenhuyse, A, Demertzi, A, et al. Diagnostic precision of PET imaging and functional MRI in disorders of consciousness: a clinical validation study. Lancet. (2014) 384:514–22. doi: 10.1016/s0140-6736(14)60042-8
22. Gosseries, O, Di, H, Laureys, S, and Boly, M. Measuring consciousness in severely damaged brains. Annu Rev Neurosci. (2014) 37:457–78. doi: 10.1146/annurev-neuro-062012-170339
23. Smith, LGF, Milliron, E, Ho, ML, Hu, HH, Rusin, J, Leonard, J, et al. Advanced neuroimaging in traumatic brain injury: an overview. Neurosurg Focus. (2019) 47:E17. doi: 10.3171/2019.9.FOCUS19652
24. Shahim, P, Tegner, Y, Wilson, DH, Randall, J, Skillbäck, T, Pazooki, D, et al. Blood biomarkers for brain injury in concussed professional ice hockey players. JAMA Neurol. (2014) 71:684–92. doi: 10.1001/jamaneurol.2014.367
25. Rubenstein, R, Chang, B, Yue, JK, Chiu, A, Winkler, EA, Puccio, AM, et al. Comparing plasma Phospho tau, Total tau, and Phospho tau-Total tau ratio as acute and chronic traumatic brain injury biomarkers. JAMA Neurol. (2017) 74:1063–72. doi: 10.1001/jamaneurol.2017.0655
26. Li, S, Dong, X, and Sun, W. Effects of transcranial direct current stimulation on patients with disorders of consciousness after traumatic brain injury: study protocol for a randomized, double-blind controlled trial. Trials. (2019) 20:596. doi: 10.1186/s13063-019-3680-1
27. Thibaut, A, Schiff, N, Giacino, J, Laureys, S, and Gosseries, O. Therapeutic interventions in patients with prolonged disorders of consciousness. Lancet Neurol. (2019) 18:600–14. doi: 10.1016/s1474-4422(19)30031-6
28. Cheng, L, Cortese, D, Monti, MM, Wang, F, Riganello, F, Arcuri, F, et al. Do sensory stimulation programs have an impact on consciousness recovery? Front Neurol. (2018) 9:826. doi: 10.3389/fneur.2018.00826
29. Yu, YT, Yang, Y, Wang, LB, Fang, JL, Chen, YY, He, JH, et al. Transcutaneous auricular vagus nerve stimulation in disorders of consciousness monitored by FmrI: the first case report. Brain Stimul. (2017) 10:328–30. doi: 10.1016/j.brs.2016.12.004
30. Noé, E, Ferri, J, Colomer, C, Moliner, B, O'Valle, M, Ugart, P, et al. Feasibility, safety and efficacy of transauricular vagus nerve stimulation in a cohort of patients with disorders of consciousness. Brain Stimul. (2020) 13:427–9. doi: 10.1016/j.brs.2019.12.005
31. Hakon, J, Moghiseh, M, and Poulsen, I. Transcutaneous vagusnerve stimulation in patients with severe traumatic brain injury: a feasibility trial. Neuromodulation. (2020) 23:859–64. doi: 10.1111/ner.13148
32. Maldonato, M. The ascending reticular activating system In: S Bassis, A Esposito, and FC Morabito, editors. Recent Advances of Neural Network Models and Applications. Cham: Springer International Publishing (2014). 333–44.
34. Davey, CG, Pujol, J, and Harrison, BJ. Mapping the self in the brain's default mode network. NeuroImage. (2016) 132:390–7. doi: 10.1016/j.neuroimage.2016.02.022
35. Thibaut, A, Bruno, MA, Chatelle, C, Gosseries, O, Vanhaudenhuyse, A, Demertzi, A, et al. Metabolic activity in external and internal awareness networks in severely brain-damaged patients. J Rehabil Med. (2012) 44:487–94. doi: 10.2340/16501977-0940
36. Bonnelle, V, Ham, TE, Leech, R, Kinnunen, KM, Mehta, MA, Greenwood, RJ, et al. Salience network integrity predicts default mode network function after traumatic brain injury. Proc Natl Acad Sci U S A. (2012) 109:4690–5. doi: 10.1073/pnas.1113455109
37. Qin, P, Wu, X, Huang, Z, Duncan, NW, Tang, W, Wolff, A, et al. How are different neural networks related to consciousness? Ann Neurol. (2015) 78:594–605. doi: 10.1002/ana.24479
38. van den Heuvel, MP, Mandl, RC, Kahn, RS, and Hulshoff Pol, HE. Functionally linked resting-state networks reflect the underlying structural connectivity architecture of the human brain. Hum Brain Mapp. (2009) 30:3127–41. doi: 10.1002/hbm.20737
39. van den Heuvel, MP, and Hulshoff Pol, HE. Exploring the brain network: a review on resting-state FmrI functional connectivity. Eur Neuropsychopharmacol. (2010) 20:519–34. doi: 10.1016/j.euroneuro.2010.03.008
40. Frangos, E, Ellrich, J, and Komisaruk, BR. Non-invasive access to the vagus nerve central projections via electrical stimulation of the external ear: FmrI evidence in humans. Brain Stimul. (2015) 8:624–36. doi: 10.1016/j.brs.2014.11.018
41. Yakunina, N, Kim, SS, and Nam, EC. Optimization of transcutaneous vagus nerve stimulation using functional MRI. Neuromodul J Int Neuromod Soc. (2017) 20:290–300. doi: 10.1111/ner.12541
42. Sclocco, R, Garcia, RG, Kettner, NW, Isenburg, K, Fisher, HP, Hubbard, CS, et al. The influence of respiration on brainstem and cardiovagal response to auricular vagus nerve stimulation: a multimodal ultrahigh-field (7T) FmrI study. Brain Stimul. (2019) 12:911–21. doi: 10.1016/j.brs.2019.02.003
43. Sclocco, R, Garcia, RG, Kettner, NW, Fisher, HP, Isenburg, K, Makarovsky, M, et al. Stimulus frequency modulates brainstem response to respiratory-gated transcutaneous auricular vagus nerve stimulation. Brain Stimul. (2020) 13:970–8. doi: 10.1016/j.brs.2020.03.011
44. Osińska, A, Rynkiewicz, A, Binder, M, Komendziński, T, Borowicz, A, and Leszczyński, A. Non-invasive Vagus nerve stimulation in treatment of disorders of consciousness—longitudinal case study. Front Neurosci. (2022) 16:834507. doi: 10.3389/fnins.2022.834507
45. Zerbi, V, Floriou-Servou, A, Markicevic, M, Vermeiren, Y, Sturman, O, Privitera, M, et al. Rapid reconfiguration of the functional connectome after chemogenetic locus coeruleus activation. Neuron. (2019) 103:702–718.e5. doi: 10.1016/j.neuron.2019.05.034
Keywords: transcutaneous auricular vagus nerve stimulation, disorders of consciousness, acquired brain injury, randomized controlled trial, vagal cortical pathways model, multi-modal assessment
Citation: Cheng L, Sun L, Xu L, Zhao F, Liu X, Wang A, Di H and Cong Y-S and (2023) Randomized trial of transcutaneous auricular vagus nerve stimulation on patients with disorders of consciousness: A study protocol. Front. Neurol. 14:1116115. doi: 10.3389/fneur.2023.1116115
Received: 14 December 2022; Accepted: 24 March 2023;
Published: 13 April 2023.
Edited by:
Bahia Hakiki, IRCCS Don Carlo Gnocchi Firenze, ItalyReviewed by:
Eric Azabou, Assistance Publique Hopitaux De Paris, FranceCopyright © 2023 Cheng, Sun, Xu, Zhao, Liu, Wang, Di and Cong. This is an open-access article distributed under the terms of the Creative Commons Attribution License (CC BY). The use, distribution or reproduction in other forums is permitted, provided the original author(s) and the copyright owner(s) are credited and that the original publication in this journal is cited, in accordance with accepted academic practice. No use, distribution or reproduction is permitted which does not comply with these terms.
*Correspondence: Yu-Sheng Cong, eXNjb25nQGh6bnUuZWR1LmNu; Haibo Di, aGFpYm9kaUBoem51LmVkdS5jbg==
†These authors have contributed equally to this work
Disclaimer: All claims expressed in this article are solely those of the authors and do not necessarily represent those of their affiliated organizations, or those of the publisher, the editors and the reviewers. Any product that may be evaluated in this article or claim that may be made by its manufacturer is not guaranteed or endorsed by the publisher.
Research integrity at Frontiers
Learn more about the work of our research integrity team to safeguard the quality of each article we publish.