- 1Department of Neurology, Clinical Medical School of Jiujiang University, Jiujiang, Jiangxi, China
- 2Jiujiang Clinical Precision Medicine Research Center, Jiujiang, Jiangxi, China
- 3Medical College of Jiujiang University, Jiujiang, Jiangxi, China
- 4Emergency Department, Affiliated Hospital of Jiujiang University, Jiujiang, Jiangxi, China
Non-traumatic intraparenchymal brain hemorrhage is referred to as intracerebral hemorrhage (ICH). Although ICH is associated with a high rate of disability and case fatality, active intervention can significantly lower the rate of severe disability. Studies have shown that the speed of hematoma clearance after ICH determines the patient's prognosis. Following ICH, depending on the hematoma volume and mass effect, either surgical- or medication-only conservative treatment is chosen. The goal of promoting endogenous hematoma absorption is more relevant because surgery is only appropriate for a small percentage of patients, and open surgery can cause additional trauma to patients. The primary method of removing hematoma after ICH in the future will involve understanding how to produce and manage macrophage/microglial endogenous phagocytic hematomas. Therefore, it is necessary to elucidate the regulatory mechanisms and key targets for clinical purposes.
1. Introduction
Intracerebral hemorrhage (ICH) refers to non-traumatic intraparenchymal brain hemorrhage, which is generally caused by long-term hypertension and arteriosclerosis. A sudden rise in blood pressure can result in vascular rupture and bleeding, and the blood can enter the brain parenchyma, where it solidifies and causes a mass effect. Subsequently, cells with phagocytic function engulf these blood clots. Blood clots are chemical accumulations of red blood cells (RBCs) and their lysis products such as hemoglobin (Hb), heme, iron, and globin. The pathological mechanisms of brain injury caused by these clots in the brain parenchyma include the inhibition of cell metabolism, inflammatory response, iron overload, oxidative stress, and tissue edema. Currently, hematoma-scavenging craniectomy or minimally invasive hematoma removal is the primary treatment approach for rapid hematoma clearance. However, the surgical approach has not yet been able to dramatically improve the long-term neurological prognoses in such cases. There are strict surgical guidelines, for example, hematoma-scavenging craniectomy requires craniotomy and can result in additional secondary injury. It is frequently used for patients with significant cerebral bleeding. Although minimally invasive hematoma removal can prevent craniotomy, it may result in incomplete removal of the hematoma, particularly when there is active bleeding. Accelerating the absorption of endogenous hematomas is another crucial strategy to inhibit nerve damage secondary to hematomas and for their complete removal. It has been discovered that peroxisome proliferator-activated receptor (PPAR) and nuclear factor erythroid 2-related factor 2 (Nrf2) signaling pathways can control the expression of scavenger receptor genes and activate microglia to promote endogenous hematoma absorption (1, 2). Promoting the expression of CD36 and CD163 on microglia/macrophages can enhance the phagocytosis of Hb, whereas blocking CD47 on red blood cells can promote the phagocytosis of RBCs. In addition, endogenous hematoma absorption can be facilitated by several mechanisms that increase the phagocytic capability of macrophages and microglia. Based on the current status of ICH research and treatment, this review intends to identify specific targets, explain the relevant mechanisms of endogenous hematoma absorption, and propose novel ideas for the treatment of endogenous hematoma resorption.
2. Cells involved in phagocytosis after ICH
In a mouse model of ICH with autologous blood injection, macrophages and dendritic cells were found to be congregated within hours of ICH (3). There is a slight increase in the percentage of dendritic cells in the hematoma compared to that in the peripheral blood of patients with ICH (4). Among the various types of phagocytic cells, macrophages/microglia remain primarily researched for phagocytosis of hematoma components following ICH (5, 6). Essentially, there are two types of macrophages. Tissue-resident macrophages are highly specialized cells that perform specific functions in the tissues where they settle, such as microglia in the central nervous system, perivascular macrophages, osteoclasts in bones, and intestinal macrophages in the gastrointestinal tract. The other type, the monocyte-derived macrophages (MDMs), can infiltrate tissues because of inflammation and chemokines. However, tissue-settled macrophages and MDMs have also been studied as a class. In 2018, Chang proposed that MDMs are essential for hematoma clearance and functional recovery after ICH (7). Subsequently, in 2021, marked differences were observed in the transcriptional states of MDMs and tissue-resident macrophages (microglia) in ICH, suggesting that the two have different functions. MDMs have a better phagocytic ability, as evidenced by the fact that they phagocytize RBCs around the hematoma in the majority of cases (8). The discovery of unique markers of tissue-resident macrophages and MDMs will aid in future research to determine their roles separately. To clarify whether endogenous hematoma absorption after ICH is required to target tissue-resident macrophages and MDMs, additional experimental data are necessary.
3. Scavenger receptors associated with endogenous hematoma phagocytosis
Scavenger receptors (SRs) are a class of architecturally varied proteins capable of recognizing a multitude of ligands on the cell surface, including pathogens and endogenous and modified host-derived molecules. In addition to serving as phagocytes and innate immune recognition receptors, SRs play a significant role in several physiological and pathological processes as inflammatory signal regulators. At least six distinct molecular types have been reported in the literature, among which CD36, CD163, CD91, SR-A (also known as CD204), and Lox-1 are connected to hematoma absorption after ICH.
3.1. CD36
CD36 belongs to the class B scavenger receptor family and is widely expressed in a variety of cells, such as microvascular endothelium cells, adipocytes, skeletal muscle cells, dendritic cells, smooth muscle cells, and hematopoietic cells. CD36 features as an SR involved in cell adhesion, antigen presentation, and identification and internalization of apoptotic cells (9). The majority of CD36 is expressed on microglia and mostly on Iba1+ cells with microglial morphology around the core area of the hematoma, as observed in a rat collagenase-induced ICH model (6). However, the expression of CD36 does not have an impact on hemoglobin levels within 24 h of ICH (6), and the volume of hematoma that is absorbed in the middle and late stages is much lower in CD36 gene-deficient rats than in normal rats, indicating that CD36 expression can influence the absorption rate of hematoma in these stages (6, 10). Mouse RBCs purified by density gradient centrifugation were diluted to 108 cells/ml and added to cultured microglia at a ratio of 10:1 to establish an in vitro ICH model. Deletion of the CD36 gene led to a reduction in microglial phagocytosis of RBC (10), indicating that CD36 on microglia plays a role in promoting phagocytosis of RBC and hematoma absorption. However, it is still uncertain how CD36 expression in macrophages/microglia can influence erythrocyte phagocytosis. Notably, studies have shown that increasing CD36 expression can influence microglia to induce the M2 phenotype surrounding hematoma, which enhances their phagocytosis and anti-inflammatory effects (11). In addition, microglia are the only cells specifically identified in ICH for the CD36-mediated clearance of hematoma components. Therefore, we believe that upregulating CD36 after ICH can increase endogenous hematoma absorption; however, the precise mechanism and cell types involved remain to be investigated.
3.2. CD163
The hemoglobin SR CD163 is expressed on cells of the monocyte–macrophage lineage and participates in the uptake of hemoglobin–haptoglobin (Hb–Hp) complexes and promotes free Hb uptake (12, 13). Hb produced by erythrolysis in hematomas attaches to Hp once it is free, and the Hp–Hb complex can then be endocytosed by CD163-mediated phagocytes (14). In addition, increased Hb levels have been found to upregulate neuronal CD163 expression (15), although a significant association between neuronal CD163 and endogenous hematoma absorption was not confirmed in the current investigation. Leclerc et al. discovered that although there is a significant correlation between phagocyte CD163 and Hb clearance, hematoma volume in mice with CD163 gene deletion 3 days after ICH was 43.4±5.0% less than that in the wild-type mice. However, the mortality of ICH mice with CD163 −/− in 4–10 days was 66.7%, which was significantly higher than that of WT mice (33.3%), and the residual hematoma after 10 days was also higher than that of WT mice. Subsequent research has shown that CD163 is a scavenger receptor for Hp-Hb complexes and clears uncomplexed Hb under severe hemolytic conditions associated with Hp depletion. The authors believe that it plays a more important role in secondary brain injury after ICH (16). In previous studies, the time windows for CD163 to function were disregarded after ICH. Future studies can evaluate these time windows to efficiently connect the various hematoma absorption mechanisms. Future studies should focus on CD163, which has clinically meaningful therapeutic potential in post-ICH phagocytes.
3.3. Other related SRs
To absorb heme after erythrocytolysis, cells that express low-density lipoprotein receptor-related protein-1 (LRP1, also known as CD91) engulf the heme–hemopexin (heme–Hx) complex (17, 18). In addition, Bruton's tyrosine kinase–calreticulin–LRP1–Hx (BTK–CRT–LRP1–Hx) pathway regulated by the Toll-like receptor 7 (TLR7) agonist imiquimod simultaneously increases heme-Hx clearance (19). Toll-like receptor 9 (TLR9) promotes the clearance of hematoma and iron by activating macrophages/microglia after ICH, and the Nrf2/CD204/HO-1 pathway is involved in TLR9-induced macrophage/microglial phagocytosis (20). However, more studies are required to demonstrate that the CD91–heme–Hx pathway facilitates the absorption of endogenous hematomas because investigations on the aforementioned pathways have not been conducted at sufficient depth. The role of CD 204 and other SR after ICH must also be explored, as well as their potential involvement in the phagocytosis of hematoma components by macrophages/microglia.
4. Substances and methods for regulating phagocyte function
4.1. CD47
CD47 is a transmembrane protein that is a ligand for signal-regulating protein α (SIRPα) expressed in phagocytes, including macrophages and dendritic cells. When SIRP is activated, a signal transduction cascade that inhibits phagocytosis is activated (21). RBCs express the “don't eat me” signal through CD47, and this signal can interact with the macrophage inhibitory receptor SIRP to prevent phagocytosis (22). CD47 inhibiting antibodies can facilitate hematoma removal and alleviate brain damage in mice (23). Another study on ICH elderly rat model showed similar results. CD47 blocking antibody can promote hematoma clearance, reduce secondary injuries, and increase the number of macrophages/microglia in a hematoma (5). Furthermore, it has been proposed that the depletion of M2 microglia with clodronate liposomes can aggravate brain damage caused by ICH; thus, it was discovered that RBC CD47 expression inhibits microglial polarization to the M2 phenotype and phagocytic RBC, thereby inhibiting hematoma clearance, as these changes enhance brain damage after ICH (24). The inhibition of CD47 expression on RBC can improve hematoma absorption by phagocytes. Targeting the inhibition of CD47 expression on RBC is necessary because it can stop phagocytes from devouring other intraparenchymal cells that are not part of a hematoma.
4.2. Phosphatidylserine
Phosphatidylserine is a ubiquitous phospholipid located at the entrance of the plasma membrane. During apoptosis, phosphatidylserine is exposed to the outer surface of the plasma membrane, which is called phosphatidylserine eversion. It serves as a signal for the phagocyte to “eat me” and promotes phagocytosis (25). Macrophages phagocytose RBC, which are largely dependent on phosphatidylserine, and this has been observed in both patients with ICH and mouse ICH models. In addition, engulfing RBCs with phosphatidylserine eversion regulates the MDMs phenotype in humans and mice, as well as hematoma absorption and patient rehabilitation (7). In fact, many phosphatidylserine receptors are also categorized as SR, such as CD91, T-cell immunoglobulin and mucin receptor 4 (TIM 4), stabilin-1, and others (26). Furthermore, phosphatidylserine has been proposed to identify and attach to the extracellular domain of CD36, recognizing and phagocytizing senescent cells through phosphatidylserine-CD36 interaction (27). As a result, we believe that it will be particularly interesting to investigate the relationship between erythrocyte phosphatidylserine eversion and SR in ICH.
4.3. Heme oxygenase 1
Heme oxygenase 1 (HO-1) can catalyze heme to produce carbon monoxide, iron, and biliverdin. Zhang et al. investigated the function of HO-1 in 12-month-old mice (28) and found that intraperitoneal injection of the HO-1 inducer cobalt protoporphyrin IX in a collagenase-induced mouse ICH model can promote hematoma clearance, while the HO-1 inhibitor zinc protoporphyrin IX inhibits hematoma clearance in the later stages of ICH (7–28 days). In contrast, cobalt protoporphyrin IX-induced HO-1 expression can exacerbate secondary brain injury and neurological defects in the early stages of ICH (1–3 days). Consistent with the results of our research group, we believe that the neuroprotective effect of HO-1 begins early (12 h to 7 days), as observed in a rat autologous blood ICH model. In addition, HO-1 controls the Nrf2-ARE pathway in the ICH model by preventing Nrf2 from accessing the nucleus and stimulating the production of NF-κB and TNF-α, and the early neuroprotection of HO-1 is related to the nuclear translocation of Nrf2 and NF-κB (29). The ICH modeling techniques and the selection of HO-1 inducers could be responsible for the variations in HO-1 expression levels and physiological consequences in different phases in the ICH model. Notably, Nrf2-ARE transcription can enhance CD36 expression to encourage macrophage/microglial hematoma phagocytosis (2). Although there is no logical connection between this and the findings of our research team, the significance between them necessitates further consideration. Similarly, the PPAR-γ pathway can enhance the expression of microglia CD163 and HO-1 as well as promote hematoma absorption (30); therefore, targeting HO-1 to promote hematoma absorption after ICH requires a combination of other molecules and multiple signaling pathways. In addition, further investigation is required into the therapeutic window of targeted HO-1, ideally in conjunction with its clinical application.
4.4. Complement component C1q
Complement mediates the phagocytosis of apoptotic cells and cell debris (31). C1q is the serum complement component, which initiates the conventional complement activation pathway and is mainly involved in immunological and inflammatory responses. Some researchers have examined plasma C1q levels in patients with ICH and discovered that these levels were significantly higher than those in healthy individuals. Moreover, poor prognosis at 3 months can be independently predicted by plasma C1q, indicating that C1q may be a potential prognostic biomarker for ICH (32). Further research is needed to determine whether slow hematoma absorption or associated inflammatory responses are responsible for poor prognosis. Interestingly, there may be an interaction between C1q and CD91 (33) because CD91 functions as a heme receptor in the phagocytosis of heme, a component of hematoma after ICH (17). It cannot be denied that complement receptors play a role in the phagocytosis of RBCs by macrophages, which simultaneously release proinflammatory factors (34). In addition, these receptors are easily associated with secondary brain injuries. Further research is required to determine specific steps required to balance the complement-mediating phagocytosis and inflammation.
4.5. Hydrogen sulfide
Hydrogen sulfide (H2S) is a novel gas-signaling molecule. The primary enzyme in the brain that produces H2S is cystathionine β-synthase (CBS), and a decrease in CBS during ICH causes the downregulation of endogenous H2S synthesis (35). H2S has been studied for its ability to reduce inflammation after ICH (36) and provide neuroprotective benefits (35). In a recent study, H2S was found to be an endogenous regulator that mediates the sustained phagocytosis of microglia after ICH. Sulfide-quinone oxidoreductase (SQR) can oxidize CBS-derived endogenous H2S, which results in the reverse electron transport of mitochondrial complex I, leading to the production of superoxide, which conversely activates uncoupling protein 2 (UCP2) to promote microglial phagocytosis of RBC. In summary, the microglial CBS–H2S–complex I axis is essential for sustained phagocytosis following ICH (37). This study implies that starting from a redox approach, we can conduct research by concentrating on H2S to encourage hematoma absorption, offering a novel approach for future studies.
4.6. Remote ischemic conditioning
Remote ischemic conditioning (RIC) is a physical therapy method in which the limb is pressurized using a compression cuff. Usually, RIC is performed at 200 mmHg for four cycles of 5 min each, with a reperfusion break of 5 min (38–40). Research has shown that AMPK, which acts as a switch to control cellular metabolism, is essential for RIC to promote hematoma absorption (40). In an isolated perfused rat heart model, the number of delayed remote ischemic preconditioning stimuli was positively correlated with HO-1, and HO-1 was involved in cardioprotection (41). As mentioned earlier, HO-1 levels are closely associated with hematoma clearance (28–30). Although the pathological mechanisms of ICH and cardiovascular disease are different, the relationship between RIC and HO-1 indicates interesting possibilities for future research. In the latest clinical trial study, the safety of RIC in clinical patients with ICH was shown by comparing drug therapy alone and drug plus RIC for 7 consecutive days in patients with ICH. The hematoma-scavenging rate of the drug plus RIC was significantly higher than that of drug treatment alone. While this cannot directly explain the effect of RIC on absolute hematoma scavenging in patients with ICH, the higher scavenging rate of combination therapy is sufficient to show that RIC can promote hematoma clearance (42). Although it has been demonstrated that four cycles of RIC at 200 mmHg are clinically safe and successful in eliminating hematoma, it is still important to determine whether this is the optimal pressure.
4.7. Cerebral white matter fiber
Other studies have identified strategies to influence endogenous hematoma absorption after ICH. White matter fibers, for instance, are present in the core of the hematoma after ICH, and those that survive enhance the quantity of microglia/macrophages that remain there, which facilitates RBC phagocytosis and increases hematoma absorption (43). White matter fibers in the hematoma area either aid microglia/macrophages in entering the hematoma area by reducing phagocyte death or by acting as scaffolding to allow phagocytes to penetrate the hematoma area. Currently, studies on the white matter after ICH have mainly focused on white matter injury (44–47). However, the connection between white matter and endogenous hematoma absorption after ICH has not been well researched. Notably, the white matter of rodents accounts for 10–20% of brain volume, while in humans it accounts for 50% of brain volume (48), indicating that white matter plays a major role in our brain and has great research prospects in endogenous hematoma absorption after ICH. These contents are shown in Figure 1.
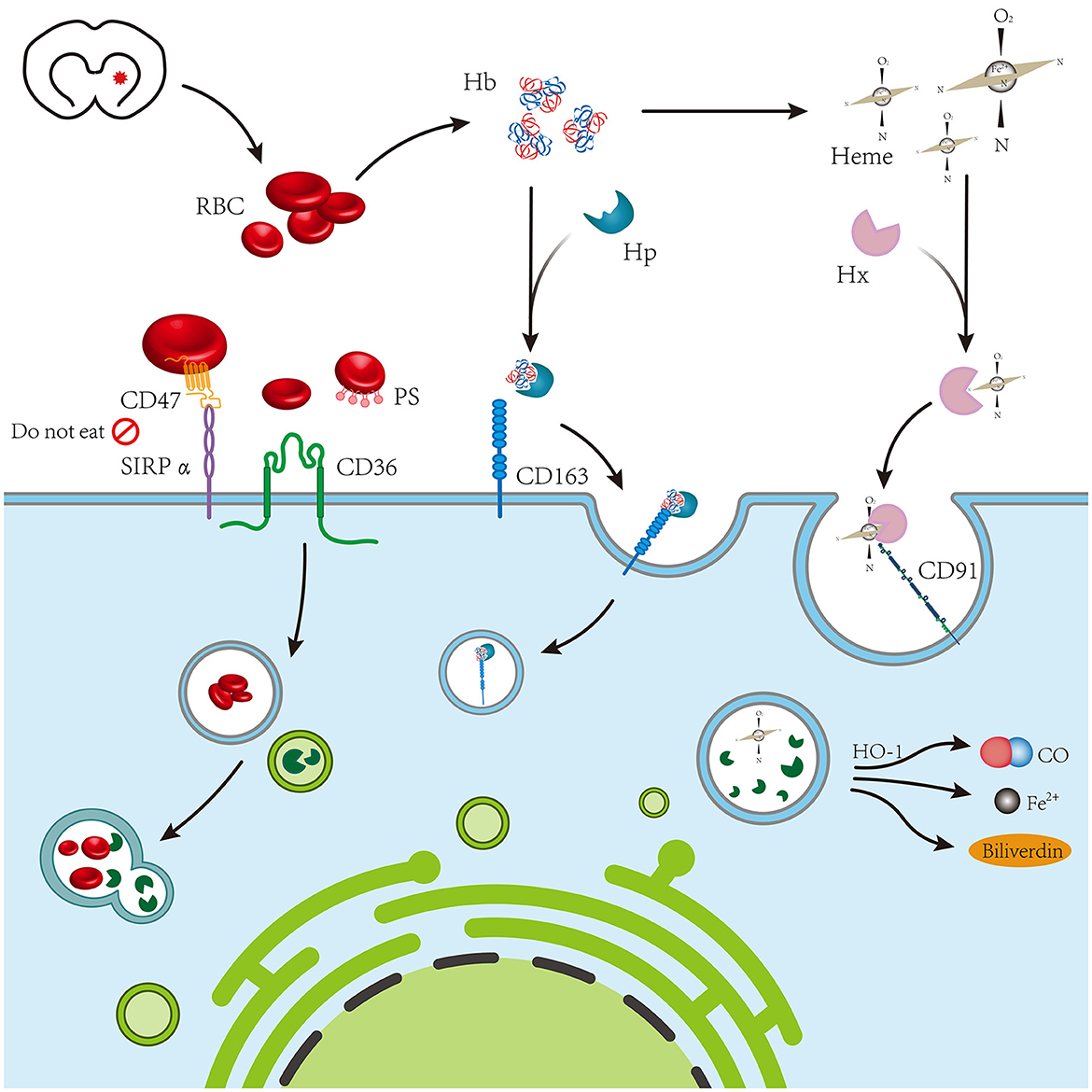
Figure 1. The blood chemical components of coagulation following intracerebral hemorrhage mainly include red blood cells, hemoglobin, and heme, which are mediated by CD36, CD163, and CD91 expressed on phagocytes, respectively.
5. Signal regulation of endogenous hematoma absorption
5.1. PPAR-γ signaling pathway
Peroxisome proliferator-activated receptors (PPARs) are ligand-activated receptors of the nuclear hormone receptor family. There are three subtypes of ligand-induced nuclear receptors that control intracellular metabolism: PPAR-α, PPAR-β/δ, and PPAR-γ. PPAR-γ has received considerable attention in ICH research. In in vivo and in vitro ICH models, fractalkine located in neuronal cells can interact with the unique fractalkine receptor CX3CR1 and promote hematoma absorption through the PPAR-γ/CD163/HO-1 signaling pathway. Meanwhile, a study on 30 patients with ICH reported that patients with reduced hematoma had higher serum fractalkine and modified Rankin scores (mRS scores) than patients with enlarged hematoma (30). In the autologous blood injection ICH model, the PPAR-γ agonist ISO-alpha-acids (IAAs) can activate PPAR-γ and increase the expression of CD36 around the hematoma, causing microglia to polarize to the M2 phenotype, increasing endogenous hematoma absorption and decreasing inflammation around the hematoma (49). The expression of CD36 and CD163 scavenger receptors on microglia/macrophages can be simultaneously increased through the PPAR-γ signaling pathway, effectively promoting endogenous hematoma absorption. Consequently, PPAR agonists are a highly promising class of medications that merit in-depth examination.
5.2. Nrf2 signaling pathway
The Nrf2 signaling pathway effectively reduces leukocyte infiltration and ROS production and is essential for preventing secondary brain damage in animal models of ICH (50). In addition, Nrf2 regulates cellular phagocytosis and promotes hematoma clearance by upregulating the expression of CD36 on the surface of microglia/macrophages through ARE transcription (2). Recombinant C-C chemokine ligand17 (CCL17) promotes hematoma clearance and improves nerve injury by activating the C-C chemokine receptor 4 (CCR4)/extracellular regulated protein kinase (ERK)/Nrf2 signaling pathway to increase CD163 expression (51). Numerous mechanisms may be involved in the mutual regulation of Nrf2 and PPAR-γ gene transcription (52). In addition, research has demonstrated that the synergistic effect of PPAR-γ and the Nrf2 pathway prevents ferroptosis-induced neuronal damage in a rat ICH model (53). The effects of the interaction between PPAR-γ and Nrf2 on endogenous hematoma absorption after ICH cannot be clearly explained by the available experimental data. However, this still provides a new direction for future studies that aim to establish a perfect system for endogenous hematoma absorption after ICH by determining the relationship between various signaling pathways and their interactions.
5.3. Signal transducer and activator of transcription 6 signaling pathway
The signal transducer and activator of transcription 6 (STAT6) is an important signaling pathway in macrophage function and is necessary for macrophages to evolve into M2 macrophages through an alternative pathway. Important cytokines for the polarization of macrophages to M2 include interleukin 4 (IL-4) and interleukin 13 (IL-13), which function by causing STAT6 phosphorylation and stimulating the transcription of STAT6 response genes (54). A small number of studies have demonstrated that IL-4 treatment after ICH promotes hematoma absorption, relieves neuroinflammation, and enhances neural functional recovery through the STAT6 signaling pathway (55, 56). According to one study, IL-4 can induce the polarization of M2 macrophage and microglia via the Janus kinase 1 (JAK1)/STAT6 pathway (55). Another study revealed that the STAT6 downstream signaling molecule STAT2 mediates the IL-4-provided function of hematoma absorption after ICH, and since STAT6 and ST2 are both necessary, the IL-4/STAT6/ST2 signaling pathway plays a crucial role in hematoma absorption after ICH (56). Nonetheless, the question remains as to whether the two signaling pathways mentioned above, IL-4/JAK1/STAT6 and IL-4/STAT6/ST2, are identical or whether they interact. However, we do not believe these two pathways are independent. In terms of effects, they both support that IL-4 promotes hematoma resolution by targeting microglia. Of course, this is only a hypothesis and needs to be verified by further experiments. The limited available data indicates that IL-4, as an anti-inflammatory factor, may induce the differentiation of anti-inflammatory microglia (also known as M2 microglia) through the STAT6 signaling pathway and mediate the phagocytosis of hematoma components. There is another signaling pathway that targets microglia-mediated hematoma phagocytosis after PPAR-γ and Nrf2, which will provide a novel idea for evaluating endogenous hematoma absorption after ICH. These contents are shown in Figure 2.
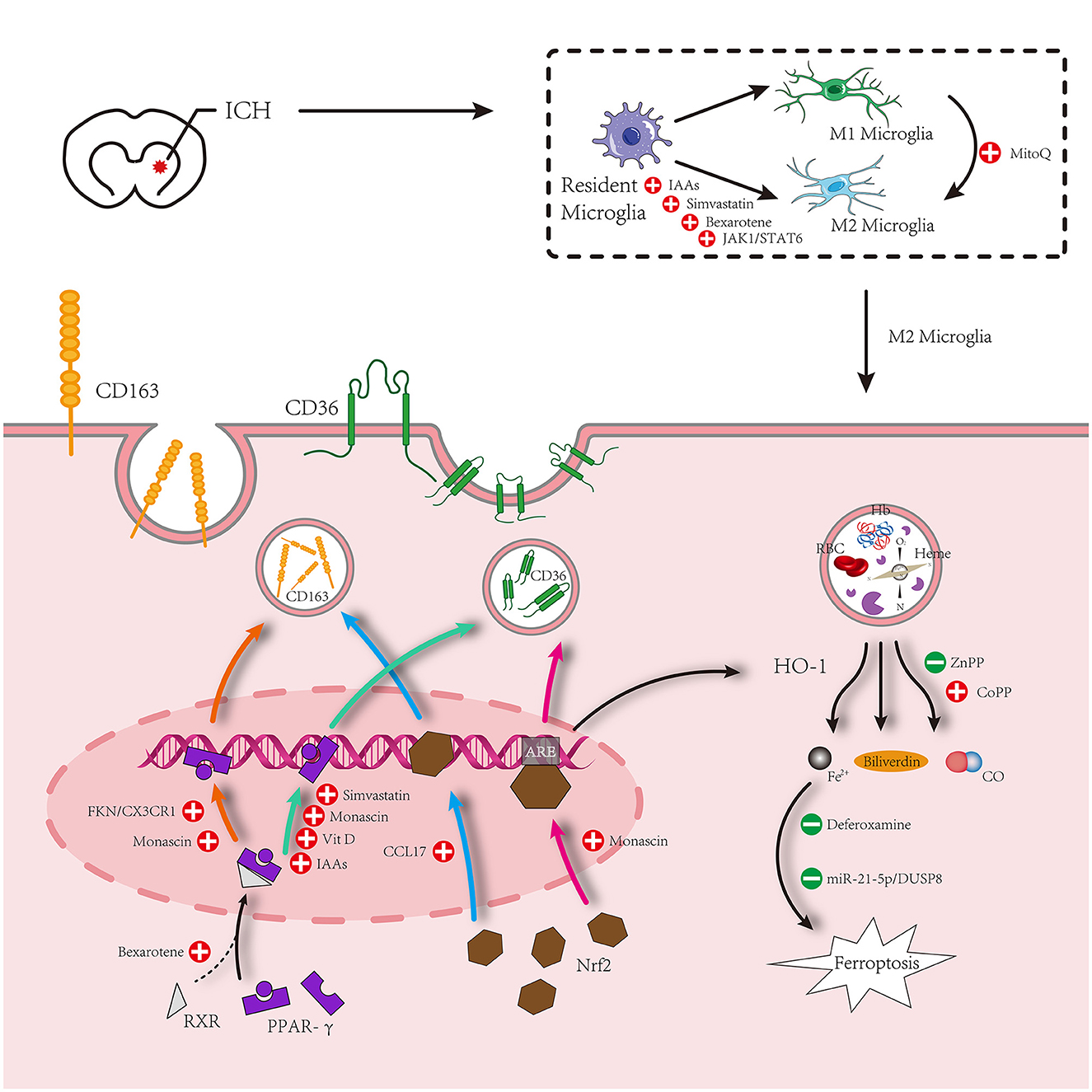
Figure 2. Microglia surrounding hematomas after intracerebral hemorrhage are polarized to an M1 or M2 phenotype. M1 phenotype promotes inflammation. M2 inhibits inflammation and promotes phagocytosis. The hematoma component is engulfed by M2 microglia, which mainly increases the expression of proteins related to phagocytosis under the action of PPAR-γ and Nrf2 signaling pathways and relieves the cytotoxicity of metabolites after phagocytosis of hematoma components. Simultaneously, M1 is inhibited by some other drugs to promote the polarization of M2 microglia.
5.4. Regulation of microRNAs
MicroRNAs (miRNAs) are a class of small non-coding RNAs that usually target the inhibition of mRNAs to regulate the transcription and protein expression of associated genes. Through the collection of clinical data, some researchers discovered that miR-21-5p was downregulated in peripheral blood and hematoma samples of patients with ICH, with miRNA-21-5p in hematoma samples being more obviously downregulated (57), although the cause remained unknown. Using bioinformatics techniques, we found that dual-specificity phosphatase 8 (DUSP8) is a direct target of miR-21-5p. A study on the collagenase-induced rat ICH model showed inhibition of DUSP8-induced miR-21-5p activation, and involvement of the phospho-extracellular regulated protein kinase (p-ERK)/HO-1 pathway secondary brain injury. In addition, injection of a miR-21-5p antagonist can significantly inhibit ferrugination in tissues and promote hematoma absorption (58). Although some researchers have investigated how miRNAs regulate the polarization of M1 and M2 microglia after ICH (59, 60), there are no studies linking this to endogenous hematoma absorption. With the gradual development of miRNA research and the maturity of related technologies in recent years, the regulation of miRNAs to promote endogenous hematoma absorption requires more attention. Related research has accelerated new miRNA-based therapeutic strategies to promote endogenous hematoma absorption following ICH.
6. Drugs for promoting hematoma absorption after intracerebral hemorrhage
6.1. Simvastatin
Statins are a class of lipid-lowering medications. Based on recent research involving both in vivo and in vitro phagocytic models, simvastatin can upregulate CD36 and increase the polarization of M2 microglia through the PPAR-γ pathway, thereby promoting hematoma absorption after ICH (11). Simvastatin shows neuroprotective effects in ICH, and simvastatin–ezetimibe combination therapy after ICH can repair impaired nerve function and reduce inflammation (61). Simvastatin reduces the infiltration of post-ICH neutrophils into the brain parenchyma by regulating peripheral blood neutrophil apoptosis to relieve neuroinflammation (62). These may be potentially linked to endogenous hematoma absorption after ICH. The impact of simvastatin on endogenous hematoma absorption has not been studied in any relevant clinical trial, and some pertinent animal studies are required to confirm that simvastatin has the potential to be used clinically to increase endogenous hematoma absorption.
6.2. Deferoxamine (DFX)
Following ICH, RBCs are lysed to release Hb, which decomposes into heme under a series of pathological actions, and heme is further decomposed into iron. Deferoxamine (DFX), an iron chelator, can effectively bind iron and reduce neuroinflammation caused by iron overload (63). According to Hu et al., DFX treatment after ICH enhances heme clearance and reduces heme levels in and around hematomas through the heme–Hx–CD91 pathway (18), which is likely to be a critical step in endogenous hematoma absorption after ICH. However, studies have also demonstrated that DFX reduces erythrolysis and iron overload by inhibiting membrane–attack complexes. In addition, DFX reduces the loss of CD47 in RBC and the invasion of microglia/macrophages after ICH, which weakens RBC phagocytosis (64). Furthermore, Liu et al. revealed that DFX exerts neuroprotective effects by reducing erythrolysis and chelating iron, and inhibits Hb-induced neuronal CD163 upregulation, which may be related to the inhibition of neuronal death (15). Early clinical trials have shown that DFX mesylate therapy after ICH can inhibit encephaloedema but delay hematoma absorption (65); therefore, we are more inclined to believe that DFX has neuroprotective effects while the promoting effect on hematoma component absorption remains debatable. With the clarification of the ferroptosis mechanism and in-depth study of DFX in neuroprotection, we believe that the link between the neuroprotective effect of DFX after brain injury and the inhibition of endogenous hematoma absorption is worth exploring and has important clinical value. Nonetheless, further animal experiments are needed to provide a basis for clinical trials to verify whether DFX can be used as a drug for clinical endogenous hematoma absorption regulation.
6.3. Monascin
Monascin is a yellow natural pigment formed by the cultivation and fermentation of Monascus ruber in cereals under certain conditions, which can reduce blood lipid and blood pressure and has anti-inflammatory and antioxidant effects (66). Monascin has been shown to play a role in endogenous hematoma absorption as a natural dual agonist of Nrf2 and PPAR-γ. A high dose of monascin can promote the reduction of hematoma volume 1–7 days after ICH (67). Long-term investigations showed that Nrf2 and PPAR-γ are crucial for increasing hematoma absorption, which significantly reduces iron overload and brain atrophy after ICH (68). It has also been pointed out that the PPAR-γ agonist monascin increases the levels of haptoglobin (Hp) and CD163 on the surface of phagocytes in plasma, accelerating hematoma absorption through the Hp–Hb–CD163 pathway (1). These animal experiments have found that monascin can promote endogenous hematoma absorption to improve ICH prognosis. However, corresponding clinical data have not been collected to demonstrate the safety of monascin in the treatment of ICH. Phase I clinical studies should be conducted as the next step to verify the security and effectiveness of monascin treatment.
6.4. Other potential drugs
Other potential drugs that promote endogenous hematoma absorption after ICH have also been extensively studied, including wogonin (69), vitamin D (70), and ISO-α-acids (IAAs) (49), which can upregulate surface CD36 of phagocytes via the PPAR-γ pathway, promote the polarization of M2 type microglia, and enhance the phagocytosis of M/MΦ to promote endogenous hematoma absorption after ICH. Bexarotene has the ability to pharmacologically activate retinoid X receptor-α (RXRα) and induce nuclear translocation of RXRα and PPAR-γ, which controls the M2 phenotype of microglia, reduces neuroinflammation, and increases hematoma absorption (71). According to a recent study by Chen et al., the mitochondrial reactive oxygen species (ROS)/NLRP-3 pathway, which is also strongly linked to hematoma absorption, may promote the transition of microglia from the M1 to the M2 phenotype under the influence of MitoQ treatment (72). However, the clinical applications of these potential drugs require further research.
7. Prospect
Over the past years, there have been several novel research directions on endogenous hematoma absorption after ICH, such as H2S-mediated sustained microglial phagocytosis of hematoma, regulation of downstream signaling by miRNAs, the role of cerebral white matter fibers, and even physical therapeutic treatment methods such as remote ischemic conditioning. In conclusion, the core of hematoma absorption after ICH is the ability of phagocytes to engulf RBC. Most existing research is aimed at promoting hematoma absorption by enhancing the M2 shift of microglia and increasing the expression of their surface SRs. However, compared with other factors, PPAR-γ and Nrf2 signaling pathways that regulate leukocyte differentiation antigens on the surface of microglia to promote hematoma absorption have attracted more attention and in-depth research. Some agonists of the PPAR-γ and Nrf2 pathways can be used as clinical drugs for patients with ICH. Therefore, we believe that the regulation of microglia/macrophages will be the “final answer” to endogenous hematoma absorption after ICH. Identifying regulatory mechanisms and significant targets will be a direction for future research.
Author contributions
ZC and CD contributed to the conception and design of the study. ZC and XY provided administrative support. PF and MW provided the study materials. PF and MW collected and assembled the data. PF, MW, and WZ performed data analysis and interpretation. MZ and ZC revised the final version. All authors contributed to the writing of the manuscript and approved the submitted version.
Funding
This study was partially supported by the National Natural Science Foundation of China (81960221 and 82260249 to XY), the National Science & Technology Fundamental Resource Investigation Program of China (2018FY100903 to XY), the Jiangxi Provincial Health Commission Science and Technology Plan Project (202311506 to ZC), the Jiangxi Provincial Administration of Traditional Chinese Medicine Science and Technology Plan Project (2022A322 to ZC), and the Science and Technology Project Founded by the Education Department of Jiangxi Province (GJJ21181 to CD).
Acknowledgments
We sincerely thank the staff of the Jiujiang Precision Clinical Medicine Research Center and its students Qinghua Huang, Mayue Yu, Jiaxing Lin, and Baoqiang Weng.
Conflict of interest
The authors declare that the research was conducted in the absence of any commercial or financial relationships that could be construed as a potential conflict of interest.
Publisher's note
All claims expressed in this article are solely those of the authors and do not necessarily represent those of their affiliated organizations, or those of the publisher, the editors and the reviewers. Any product that may be evaluated in this article, or claim that may be made by its manufacturer, is not guaranteed or endorsed by the publisher.
Abbreviations
ICH, intracerebral hemorrhage; Hb, hemoglobin; PPAR-γ, peroxisome proliferator-activated receptor-γ; Nrf2, nuclear factor erythroid 2-related factor 2; MDMs, monocyte-derived macrophages; RBCs, red blood cells; SRs, scavenger receptors; Hb–Hp, hemoglobin–haptoglobin; SIRPα, signal-regulating protein α; HO-1, heme oxygenase 1; H2S, hydrogen sulfide; UCP2, uncoupling protein 2; RIC, remote ischemic conditioning; STAT6, signal transducer and activator of transcription 6; IL-4, interleukin 4; DUSP8, dual-specificity phosphatase 8; DFX, deferoxamine.
References
1. Wang G, Li T, Duan SN, Dong L, Sun XG, Xue F. PPAR-gamma promotes hematoma clearance through haptoglobin-hemoglobin-CD163 in a rat model of intracerebral hemorrhage. Behav Neurol. (2018) 2018:7646104. doi: 10.1155/2018/7646104
2. Zhao X, Sun G, Ting SM, Song S, Zhang J, Edwards NJ, et al. Cleaning up after ICH: the role of Nrf2 in modulating microglia function and hematoma clearance. J Neurochem. (2015) 133:144–52. doi: 10.1111/jnc.12974
3. Hammond MD, Ai Y, Sansing LH. Gr1+ macrophages and dendritic cells dominate the inflammatory infiltrate 12 hours after experimental intracerebral hemorrhage. Transl Stroke Res. (2012) 3:s125–31. doi: 10.1007/s12975-012-0174-9
4. Jiang C, Wang Y, Hu Q, Shou J, Zhu L, Tian N, et al. Immune changes in peripheral blood and hematoma of patients with intracerebral hemorrhage. FASEB J. (2020) 34:2774–91. doi: 10.1096/fj.201902478R
5. Tao C, Keep RF, Xi G, Hua Y. CD47 blocking antibody accelerates hematoma clearance after intracerebral hemorrhage in aged rats. Transl Stroke Res. (2020) 11:541–51. doi: 10.1007/s12975-019-00745-4
6. Li Q, Lan X, Han X, Durham F, Wan J, Weiland A, et al. Microglia-derived interleukin-10 accelerates post-intracerebral hemorrhage hematoma clearance by regulating CD36. Brain Behav Immun. (2021) 94:437–57. doi: 10.1016/j.bbi.2021.02.001
7. Chang CF, Goods BA, Askenase MH, Hammond MD, Renfroe SC, Steinschneider AF, et al. Erythrocyte efferocytosis modulates macrophages towards recovery after intracerebral hemorrhage. J Clin Invest. (2018) 128:607–24. doi: 10.1172/JCI95612
8. Chang CF, Goods BA, Askenase MH, Beatty HE, Osherov A, DeLong JH, et al. Divergent functions of tissue-resident and blood-derived macrophages in the hemorrhagic brain. Stroke. (2021) 52:1798–808. doi: 10.1161/STROKEAHA.120.032196
9. Febbraio M, Hajjar DP, Silverstein RL. CD36: a class B scavenger receptor involved in angiogenesis, atherosclerosis, inflammation, and lipid metabolism. J Clin Invest. (2001) 108:785–91. doi: 10.1172/JCI14006
10. Fang H, Chen J, Lin S, Wang P, Wang Y, Xiong X, et al. CD36-mediated hematoma absorption following intracerebral hemorrhage: negative regulation by TLR4 signaling. J Immunol. (2014) 192:5984–92. doi: 10.4049/jimmunol.1400054
11. Wang Y, Chen Q, Tan Q, Feng Z, He Z, Tang J, et al. Simvastatin accelerates hematoma resolution after intracerebral hemorrhage in a PPARgamma-dependent manner. Neuropharmacology. (2018) 128:244–54. doi: 10.1016/j.neuropharm.2017.10.021
12. Thomsen JH, Etzerodt A, Svendsen P, Moestrup SK. The haptoglobin-CD163-heme oxygenase-1 pathway for hemoglobin scavenging. Oxid Med Cell Longev. (2013) 2013:523652. doi: 10.1155/2013/523652
13. Etzerodt A, Moestrup SK. CD163 and inflammation: biological, diagnostic, and therapeutic aspects. Antioxid Redox Signal. (2013) 18:2352–63. doi: 10.1089/ars.2012.4834
14. Wang G, Wang L, Sun XG, Tang J. Haematoma scavenging in intracerebral haemorrhage: from mechanisms to the clinic. J Cell Mol Med. (2018) 22:768–77. doi: 10.1111/jcmm.13441
15. Liu R, Cao S, Hua Y, Keep RF, Huang Y, Xi G. CD163 expression in neurons after experimental intracerebral hemorrhage. Stroke. (2017) 48:1369–75. doi: 10.1161/STROKEAHA.117.016850
16. Leclerc JL, Lampert AS, Loyola Amador C, Schlakman B, Vasilopoulos T, Svendsen P, et al. The absence of the CD163 receptor has distinct temporal influences on intracerebral hemorrhage outcomes. J Cereb Blood Flow Metab. (2018) 38:262–73. doi: 10.1177/0271678X17701459
17. Wang G, Manaenko A, Shao A, Ou Y, Yang P, Budbazar E, et al. Low-density lipoprotein receptor-related protein-1 facilitates heme scavenging after intracerebral hemorrhage in mice. J Cereb Blood Flow Metab. (2017) 37:1299–310. doi: 10.1177/0271678X16654494
18. Hu S, Hua Y, Keep RF, Feng H, Xi G. Deferoxamine therapy reduces brain hemin accumulation after intracerebral hemorrhage in piglets. Exp Neurol. (2019) 318:244–50. doi: 10.1016/j.expneurol.2019.05.003
19. Wang G, Guo Z, Tong L, Xue F, Krafft PR, Budbazar E, et al. TLR7. (toll-like receptor 7) facilitates heme scavenging through the BTK. (Bruton tyrosine kinase)-CRT. (calreticulin)-LRP1. (low-density lipoprotein receptor-related Protein-1)-Hx. (hemopexin) pathway in murine intracerebral hemorrhage. Stroke. (2018) 49:3020–9. doi: 10.1161/STROKEAHA.118.022155
20. Wei J, Dai S, Pu C, Luo P, Yang Y, Jiang X, et al. Protective role of TLR9-induced macrophage/microglia phagocytosis after experimental intracerebral hemorrhage in mice. CNS Neurosci Ther. (2022) 28:1800–13. doi: 10.1111/cns.13919
21. Oldenborg PA, Gresham HD, Lindberg FP. CD47-signal regulatory protein alpha. (SIRPalpha) regulates Fcgamma and complement receptor-mediated phagocytosis. J Exp Med. (2001) 193:855–62. doi: 10.1084/jem.193.7.855
22. Burger P, Hilarius-Stokman P, de Korte D, van den Berg TK, van Bruggen R. CD47 functions as a molecular switch for erythrocyte phagocytosis. Blood. (2012) 119:5512–21. doi: 10.1182/blood-2011-10-386805
23. Jing C, Bian L, Wang M, Keep RF, Xi G, Hua Y. Enhancement of hematoma clearance with CD47 blocking antibody in experimental intracerebral hemorrhage. Stroke. (2019) 50:1539–47. doi: 10.1161/STROKEAHA.118.024578
24. Ni W, Mao S, Xi G, Keep RF, Hua Y. Role of erythrocyte CD47 in intracerebral hematoma clearance. Stroke. (2016) 47:505–11. doi: 10.1161/STROKEAHA.115.010920
25. Leventis PA, Grinstein S. The distribution and function of phosphatidylserine in cellular membranes. Annu Rev Biophys. (2010) 39:407–27. doi: 10.1146/annurev.biophys.093008.131234
26. Penberthy KK, Ravichandran KS. Apoptotic cell recognition receptors and scavenger receptors. Immunol Rev. (2016) 269:44–59. doi: 10.1111/imr.12376
27. Banesh S, Ramakrishnan V, Trivedi V. Mapping of phosphatidylserine recognition region on CD36 ectodomain. Arch Biochem Biophys. (2018) 660:1–10. doi: 10.1016/j.abb.2018.10.005
28. Zhang Z, Song Y, Zhang Z, Li D, Zhu H, Liang R, et al. Distinct role of heme oxygenase-1 in early- and late-stage intracerebral hemorrhage in 12-month-old mice. J Cereb Blood Flow Metab. (2017) 37:25–38. doi: 10.1177/0271678X16655814
29. Yin XP, Wu D, Zhou J, Chen ZY, Bao B, Xie L. Heme oxygenase 1 plays role of neuron-protection by regulating Nrf2-ARE signaling post intracerebral hemorrhage. Int J Clin Exp Pathol. (2015) 8:10156–63.
30. You M, Long C, Wan Y, Guo H, Shen J, Li M, et al. Neuron derived fractalkine promotes microglia to absorb hematoma via CD163/HO-1 after intracerebral hemorrhage. Cell Mol Life Sci. (2022) 79:224. doi: 10.1007/s00018-022-04212-6
31. Melhorn MI, Brodsky AS, Estanislau J, Khoory JA, Illigens B, Hamachi I, et al. CR1-mediated ATP release by human red blood cells promotes CR1 clustering and modulates the immune transfer process. J Biol Chem. (2013) 288:31139–53. doi: 10.1074/jbc.M113.486035
32. Wang Z, Wu X, Yan T, Liu M, Yu W, Du Q, et al. Elevated plasma complement C1q levels contribute to a poor prognosis after acute primary intracerebral hemorrhage: a prospective cohort study. Front Immunol. (2022) 13:920754. doi: 10.3389/fimmu.2022.920754
33. Duus K, Hansen EW, Tacnet P, Frachet P, Arlaud GJ, Thielens NM, et al. Direct interaction between CD91 and C1q. FEBS J. (2010) 277:3526–37. doi: 10.1111/j.1742-4658.2010.07762.x
34. de Back DZ, Kostova EB, van Kraaij M, van den Berg TK, van Bruggen R. Of macrophages and red blood cells; a complex love story. Front Physiol. (2014) 5:9. doi: 10.3389/fphys.2014.00009
35. Shan H, Qiu J, Chang P, Chu Y, Gao C, Wang H, et al. Exogenous hydrogen sulfide offers neuroprotection on intracerebral hemorrhage injury through modulating endogenous H2S Metabolism in Mice. Front Cell Neurosci. (2019) 13:349. doi: 10.3389/fncel.2019.00349 H2S
36. Zhao H, Pan P, Yang Y, Ge H, Chen W, Qu J, et al. Endogenous hydrogen sulphide attenuates NLRP3 inflammasome-mediated neuroinflammation by suppressing the P2X7 receptor after intracerebral haemorrhage in rats. J Neuroinflammation. (2017) 14:163. doi: 10.1186/s12974-017-0940-4
37. Yan X, He M, Huang H, Wang Q, Hu Y, Wang X, et al. Endogenous H2S targets mitochondria to promote continual phagocytosis of erythrocytes by microglia after intracerebral hemorrhage. Redox Biol. (2022) 56:102442. doi: 10.1016/j.redox.2022.102442
38. Jarrahi A, Shah M, Ahluwalia M, Khodadadi H, Vaibhav K, Bruno A, et al. Pilot study of remote ischemic conditioning in acute spontaneous intracerebral hemorrhage. Front Neurosci. (2022) 16:791035. doi: 10.3389/fnins.2022.791035
39. Zhao W, Jiang F, Li S, Wu C, Gu F, Zhang Q, et al. Remote ischemic conditioning for intracerebral hemorrhage. (RICH-1): rationale and study protocol for a pilot open-label randomized controlled trial. Front Neurol. (2020) 11:313. doi: 10.3389/fneur.2020.00313
40. Vaibhav K, Braun M, Khan MB, Fatima S, Saad N, Shankar A, et al. Remote ischemic post-conditioning promotes hematoma resolution via AMPK-dependent immune regulation. J Exp Med. (2018) 215:2636–54. doi: 10.1084/jem.20171905
41. Zhou C, Li L, Li H, Gong J, Fang N. Delayed remote preconditioning induces cardioprotection: role of heme oxygenase-1. J Surg Res. (2014) 191:51–7. doi: 10.1016/j.jss.2014.03.054
42. Zhao W, Jiang F, Li S, Liu G, Wu C, Wang Y, et al. Safety and efficacy of remote ischemic conditioning for the treatment of intracerebral hemorrhage: a proof-of-concept randomized controlled trial. Int J Stroke. (2022) 17:425–33. doi: 10.1177/17474930211006580
43. Chen J, Koduri S, Dai S, Toyota Y, Hua Y, Chaudhary N, et al. Intra-hematomal white matter tracts act as a scaffold for macrophage infiltration after intracerebral hemorrhage. Transl Stroke Res. (2021) 12:858–65. doi: 10.1007/s12975-020-00870-5
44. Zheng J, Lu J, Mei S, Wu H, Sun Z, Fang Y, et al. Ceria nanoparticles ameliorate white matter injury after intracerebral hemorrhage: microglia-astrocyte involvement in remyelination. J Neuroinflammation. (2021) 18:43. doi: 10.1186/s12974-021-02101-6
45. Yang H, Ni W, Wei P, Li S, Gao X, Su J, et al. HDAC inhibition reduces white matter injury after intracerebral hemorrhage. J Cereb Blood Flow Metab. (2021) 41:958–74. doi: 10.1177/0271678X20942613
46. Fu X, Zhou G, Wu X, Xu C, Zhou H, Zhuang J, et al. Inhibition of P2X4R attenuates white matter injury in mice after intracerebral hemorrhage by regulating microglial phenotypes. J Neuroinflammation. (2021) 18:184. doi: 10.1186/s12974-021-02239-3
47. Li M, Xia M, Chen W, Wang J, Yin Y, Guo C, et al. Lithium treatment mitigates white matter injury after intracerebral hemorrhage through brain-derived neurotrophic factor signaling in mice. Transl Res. (2020) 217:61–74. doi: 10.1016/j.trsl.2019.12.006
48. Jiang YB, Wei KY, Zhang XY, Feng H, Hu R. White matter repair and treatment strategy after intracerebral hemorrhage. CNS Neurosci Ther. (2019) 25:1113–25. doi: 10.1111/cns.13226
49. Zhao JL, Chen YJ, Yu J, Du ZY, Yuan Q, Sun YR, et al. Iso-alpha-acids improve the hematoma resolution and prevent peri-hematoma inflammations by transforming microglia via PPARgamma-CD36 axis in ICH rats. Int Immunopharmacol. (2020) 83:106396. doi: 10.1016/j.intimp.2020.106396
50. Wang J, Fields J, Zhao C, Langer J, Thimmulappa RK, Kensler TW, et al. Role of Nrf2 in protection against intracerebral hemorrhage injury in mice. Free Radic Biol Med. (2007) 43:408–14. doi: 10.1016/j.freeradbiomed.2007.04.020
51. Deng S, Sherchan P, Jin P, Huang L, Travis Z, Zhang JH, et al. Recombinant CCL17 enhances hematoma resolution and activation of CCR4/ERK/Nrf2/CD163 signaling pathway after intracerebral hemorrhage in mice. Neurotherapeutics. (2020) 17:1940–53. doi: 10.1007/s13311-020-00908-4
52. Zhao XR, Gonzales N, Aronowski J. Pleiotropic role of PPARgamma in intracerebral hemorrhage: an intricate system involving Nrf2, RXR, and NF-kappaB. CNS Neurosci Ther. (2015) 21:357–66. doi: 10.1111/cns.12350
53. Duan C, Jiao D, Wang H, Wu Q, Men W, Yan H, et al. Activation of the PPARgamma prevents ferroptosis-induced neuronal loss in response to intracerebral hemorrhage through synergistic actions with the Nrf2. Front Pharmacol. (2022) 13:869300. doi: 10.3389/fphar.2022.869300
54. Waqas SFH, Ampem G, Röszer T. Analysis of IL-4/STAT6 signaling in macrophages. Methods Mol Biol. (2019) 1966:211–24. doi: 10.1007/978-1-4939-9195-2_17
55. He Y, Gao Y, Zhang Q, Zhou G, Cao F, Yao S. IL-4 switches microglia/macrophage M1/M2 polarization and alleviates neurological damage by modulating the JAK1/STAT6 pathway following ICH. Neuroscience. (2020) 437:161–71. doi: 10.1016/j.neuroscience.2020.03.008
56. Xu J, Chen Z, Yu F, Liu H, Ma C, Xie D, et al. IL-4/STAT6 signaling facilitates innate hematoma resolution and neurological recovery after hemorrhagic stroke in mice. Proc Natl Acad Sci U S A. (2020) 117:32679–90. doi: 10.1073/pnas.2018497117
57. Wang J, Zhu Y, Jin F, Tang L, He Z, He Z. Differential expression of circulating microRNAs in blood and haematoma samples from patients with intracerebral haemorrhage. J Int Med Res. (2016) 44:419–32. doi: 10.1177/0300060516630852
58. Ouyang Y, Li D, Wang H, Wan Z, Luo Q, Zhong Y, et al. MiR-21-5p/dual-specificity phosphatase 8 signalling mediates the anti-inflammatory effect of haem oxygenase-1 in aged intracerebral haemorrhage rats. Aging Cell. (2019) 18:e13022. doi: 10.1111/acel.13022
59. Shao G, Zhou C, Ma K, Zhao W, Xiong Q, Yang L, et al. MiRNA-494 enhances M1 macrophage polarization via Nrdp1 in ICH mice model. J Inflamm. (2020) 17:17. doi: 10.1186/s12950-020-00247-3
60. Pei H, Peng Q, Guo S, Gu Y, Sun T, Xu D, et al. MiR-367 alleviates inflammatory injury of microglia by promoting M2 polarization via targeting CEBPA. In Vitro Cell Dev Biol Anim. (2020) 56:878–87. doi: 10.1007/s11626-020-00519-5
61. Wang KW, Liang CL, Yeh LR, Liu KY, Chen CC, Chen JS, et al. Simvastatin-ezetimibe enhances growth factor expression and attenuates neuron loss in the hippocampus in a model of intracerebral hemorrhage. Fundam Clin Pharmacol. (2021) 35:634–44. doi: 10.1111/fcp.12635
62. Zhang J, Shi X, Hao N, Chen Z, Wei L, Tan L, et al. Simvastatin reduces neutrophils infiltration into brain parenchyma after intracerebral hemorrhage via regulating peripheral neutrophils apoptosis. Front Neurosci. (2018) 12:977. doi: 10.3389/fnins.2018.00977
63. Li Z, Liu Y, Wei R, Khan S, Zhang R, Zhang Y, et al. Iron neurotoxicity and protection by deferoxamine in intracerebral hemorrhage. Front Mol Neurosci. (2022) 15:927334. doi: 10.3389/fnmol.2022.927334
64. Cao S, Zheng M, Hua Y, Chen G, Keep RF, Xi G. Hematoma changes during clot resolution after experimental intracerebral hemorrhage. Stroke. (2016) 47:1626–31. doi: 10.1161/STROKEAHA.116.013146
65. Yu Y, Zhao W, Zhu C, Kong Z, Xu Y, Liu G, et al. The clinical effect of deferoxamine mesylate on edema after intracerebral hemorrhage. PLoS ONE. (2015) 10:e0122371. doi: 10.1371/journal.pone.0122371
66. Adin SN, Gupta I, Panda BP, Mujeeb M. Monascin and ankaflavin-Biosynthesis from Monascus purpureus, production methods, pharmacological properties: a review. Biotechnol Appl Biochem. (2022). doi: 10.1002/bab.2336
67. Wang J, Wang G, Yi J, Xu Y, Duan S, Li T, et al. The effect of monascin on hematoma clearance and edema after intracerebral hemorrhage in rats. Brain Res Bull. (2017) 134:24–9. doi: 10.1016/j.brainresbull.2017.06.018
68. Fu P, Liu J, Bai Q, Sun X, Yao Z, Liu L, et al. Long-term outcomes of monascin – a novel dual peroxisome proliferator-activated receptor gamma/nuclear factor-erythroid 2 related factor-2 agonist in experimental intracerebral hemorrhage. Ther Adv Neurol Disord. (2020) 13:1756286420921083. doi: 10.1177/1756286420921083
69. Zhuang J, Peng Y, Gu C, Chen H, Lin Z, Zhou H, et al. Wogonin accelerates hematoma clearance and improves neurological outcome via the PPAR-gamma pathway after intracerebral hemorrhage. Transl Stroke Res. (2021) 12:660–75. doi: 10.1007/s12975-020-00842-9
70. Liu J, Li N, Zhu Z, Kiang KM, Ng ACK, Dong CM, et al. Vitamin D enhances hematoma clearance and neurologic recovery in intracerebral hemorrhage. Stroke. (2022) 53:2058–68. doi: 10.1161/STROKEAHA.121.037769
71. Xu C, Chen H, Zhou S, Sun C, Xia X, Peng Y, et al. Pharmacological activation of RXR-alpha promotes hematoma absorption via a PPAR-gamma-dependent pathway after intracerebral hemorrhage. Neurosci Bull. (2021) 37:1412–26. doi: 10.1007/s12264-021-00735-3
Keywords: phagocytosis, microglia, scavenger receptor, intracerebral hemorrhage, haematoma absorption
Citation: Fu P, Zhang M, Wu M, Zhou W, Yin X, Chen Z and Dan C (2023) Research progress of endogenous hematoma absorption after intracerebral hemorrhage. Front. Neurol. 14:1115726. doi: 10.3389/fneur.2023.1115726
Received: 04 December 2022; Accepted: 16 February 2023;
Published: 10 March 2023.
Edited by:
Jun Yao, Northwestern University, United StatesReviewed by:
Wenyan Li, Army Medical University, ChinaHongkai Wang, Northwestern University, United States
Copyright © 2023 Fu, Zhang, Wu, Zhou, Yin, Chen and Dan. This is an open-access article distributed under the terms of the Creative Commons Attribution License (CC BY). The use, distribution or reproduction in other forums is permitted, provided the original author(s) and the copyright owner(s) are credited and that the original publication in this journal is cited, in accordance with accepted academic practice. No use, distribution or reproduction is permitted which does not comply with these terms.
*Correspondence: Zhiying Chen, Y2hlbnpoaXlpbmcmI3gwMDA0MDtjY211LmVkdS5jbg==; Chuanjun Dan, ZGFuY2h1YW5qdW4yMDA4JiN4MDAwNDA7MTYzLmNvbQ==