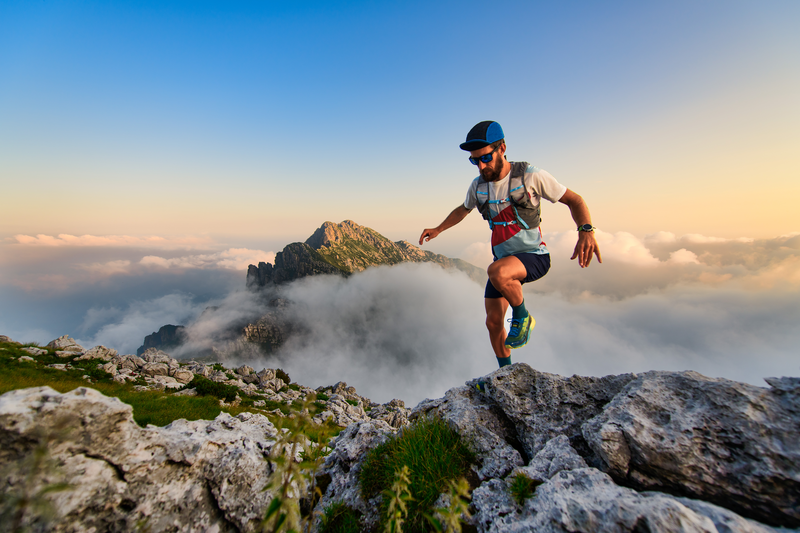
94% of researchers rate our articles as excellent or good
Learn more about the work of our research integrity team to safeguard the quality of each article we publish.
Find out more
ORIGINAL RESEARCH article
Front. Neurol. , 09 March 2023
Sec. Epilepsy
Volume 14 - 2023 | https://doi.org/10.3389/fneur.2023.1106511
This article is part of the Research Topic Neuromodulation for Pharmacoresistant Epilepsy: from Bench to Bed View all 11 articles
Objective: Evaluation of the antiseizure efficacy, side effects and neuropsychological effects of Deep brain stimulation (DBS) of the anterior nucleus of the thalamus (ANT). ANT-DBS is a treatment option for patients with difficult-to-treat epilepsy. Though several works outline the cognitive and/or mood effects of ANT-DBS for the treatment of epilepsy, data on the intersection between antiseizure efficacy, cognitive and undesired effects are scarce.
Methods: We retrospectively analyzed the data of our cohort of 13 patients. Post-implantation seizure frequencies were measured at 6 months, 12 months and last follow-up, as well as averaged throughout follow-up. These values were then compared with mean seizure frequencies in the 6 months before implantation. To address acute cognitive effects of DBS a baseline assessment was performed after implantation and before stimulation, and a follow-up assessment was conducted under DBS. The long-term effects of DBS on cognition were assessed by comparing the preoperative neuropsychological profile with a long-term follow-up under DBS.
Results: In the entire cohort, 54.5% of patients were responders, with an average seizure reduction of 73.6%. One of these patients achieved temporary seizure freedom and near-total seizure reduction during the entire follow-up. Seizure reduction of <50% was achieved in 3 patients. Non-responders suffered an average seizure increase of 27.3%. Eight of twenty-two active electrodes (36,4%) were off-target. Two of our patients had both electrodes implanted off-target. When removing these two patients from the analysis and averaging seizure frequency during the entire follow-up period, four patients (44.4%) were responders and three experienced a seizure reduction of <50%. Intolerable side effects arose in 5 patients, mostly psychiatric. Regarding acute cognitive effects of DBS, only one patient showed a significant decline in executive functions. Long-term neuropsychological effects included significant intraindividual changes in verbal learning and memory. Figural memory, attention and executive functions, confrontative naming and mental rotation were mostly unchanged, and improved in few cases.
Significance: In our cohort, more than half of patients were responders. Psychiatric side effects seem to have been more prevalent compared to other published cohorts. This may be partially explained by a relatively high occurrence of off-target electrodes.
- Some multifocal and genetic epilepsies may respond well to ANT-DBS.
- Long-term neuropsychological outcomes are mixed.
- The most common side effects in our cohort were psychiatric.
Deep brain stimulation (DBS) of the anterior nucleus of the thalamus (ANT) is a treatment option for patients with difficult-to-treat epilepsy. ANT-DBS became an established therapy after the first (and only) prospective randomized controlled trial, the Stimulation of the Anterior Nucleus of the Thalamus for Epilepsy (SANTE) trial (1), showed promising results in its 3, 5 and 10-year follow-up studies (2, 3), with a 43% responder rate (≥50% reduction in seizure frequency) at 1 year (n = 99) and 74% at seven years (n = 50).
The antiseizure effects of ANT-DBS are thought to be based on the inhibition of seizure propagation through the thalamus (4) and modulation of the Circuit of Papez (5). Furthermore, increasing responder rates over the years have been attributed to long-term neuromodulation effects in neural networks.
Though several works (6, 7) outline the cognitive and/or mood effects of ANT-DBS for the treatment of epilepsy, data on the intersection between antiseizure efficacy, cognitive and undesired effects are scarce. We aimed to systematically evaluate the antiseizure efficacy, side effects and neuropsychological effects of ANT-DBS in epilepsy patients treated at our center.
We retrospectively analyzed the data of our cohort of 13 patients, stereotactically and transventricularly implanted between 2012 and 2014, who underwent DBS for refractory epilepsy (Medtronic Activa PC Models 37601, 3787). Data on seizure reduction and side effects were complete in 11 of 13 patients. We defined refractory epilepsy according to the 2017 ILAE guidelines as non-responding to ≥2 anti-seizure medications (ASMs). Stimulation was usually initiated 3–5 weeks after implantation. We initially used the parameters described in the aforementioned landmark SANTE (1) study (Impulse width 90 μsec, Frequency 145 Hz, stimulation voltage 5.0 V, cycle: stimulation for 60 s every 5 min). Monopolar stimulation was used in all patients except the one patient with active VNS. When seizure frequency increased or did not decrease, changes in stimulation parameters were preferred to changes in ASM in order to better isolate the therapeutic effects of DBS. The preferred order of these changes was firstly changes in voltage (increase by 0.5–1 V), secondly changes in cycle speed (e.g. stimulation for 60 s every 3 min), and thirdly change into bipolar stimulation. These changes were carried out similarly in case of side effects, beginning with voltage decrease in 0.5 V steps. Nevertheless, ASM changes happened when deemed clinically necessary.
Post-implantation seizure reductions were expressed as percentages and interquartile ranges (IQR) and measured at 6 months, 12 months and last follow-up, as well as averaged throughout follow-up. These values were then compared with mean seizure frequencies in the 6 months before implantation. Seizure frequencies were assessed using seizure diaries. Seizure semiology was classified according to 2017 ILAE guidelines, based on our video-EEG (vEEG) recordings and descriptions by patients or witnesses. We analyzed the cohort of 11 patients in its entirety, and calculated the average seizure reduction during follow-up including only the patients who had at least one electrode on-target (n = 9).
In the current study we analyzed acute as well as long-term effects of DBS on cognition. To address acute cognitive effects a baseline assessment was performed after implantation and before initiating stimulation, and a follow-up assessment was conducted under DBS. The cognitive screening focused on attention and executive functions [EpiTrack® (8)] and on verbal learning and episodic memory [short version of the Verbaler Lern- und Merkfähigkeitstest, VLMT (9)]. To analyze the long-term effects of DBS on cognition we compared the preoperative neuropsychological profile with a long-term follow-up under DBS. The neuropsychological assessment included tests on attention and executive functions [EpiTrack® (8)], episodic long-term memory, i.e. verbal and figural learning and memory [VLMT (10) and a revised version of the Diagnosticum für Cerebralschädigung, DCS-R (11)], confrontative naming [Boston Naming Test, BNT (12)], and mental rotation [Leistungsprüfsystem, LPS subtest 7 (13)]. A mild impairment was defined as a performance lower than 1 standard deviation below the mean of the normative sample, a severe impairment as a performance lower than 2 standard deviations below the mean of the normative sample. Given the small sample size, we analyzed the frequencies of statistically significant intraindividual changes under DBS, employing reliable change indices (RCIs).
Follow-up duration during stimulation ranged from 9 to 111 months (average 51.5 months), and was ongoing until deactivation in all cases where deactivation occurred.
Age at implantation ranged from 22 to 50 (mean 35.5) years. Age at epilepsy onset was mostly in childhood and ranged from 4 to 24 (mean 14.5) years. Our cohort was 63.6% assigned female at birth (Table 1).
Among the 9 patients who experienced seizure reduction in the overall follow-up period, the etiology was most commonly unclear (5 patients, 55.5%), followed by structural origin. Five (55.5%) had multifocal epilepsy. Of the 2 patients with seizure increase, one had epilepsy of unknown etiology, the other structural epilepsy due to posttraumatic lesions. The patient who achieved seizure freedom had genetic generalized epilepsy. One of the responders with epilepsy of unknown origin, who underwent explantation due to polydipsia and the emergence of functional non-epileptic seizures, later underwent genetic testing that revealed a Dyamin-1 mutation suggesting a generalized encephalopathic epilepsy. Follow-up duration during stimulation ranged from 9 to 111 months (average 51.5 months), and is either ongoing, or continued until deactivation/explantation in all cases. Mean duration of stimulation (excluding the 3 patients with ongoing stimulation) was 52.6 months (range 10–97 months). When excluding the patient who underwent explantation before the 12 month follow-up mark due to intolerable side effects, all 7 patients underwent stimulation for at least 2 years and up to 6 years.
Patients were taking an average of 3.6 ASM at the time of implantation (range 2–5). Two patients underwent no changes in ASM during stimulation. Five patients, all of them DBS responders before the ASM change, underwent a substitution of one ASM due to side effects (exchange of one ASM for another), three patients were subject to more than one change in ASM (exchange, increase and/or reduction): two of these patients experienced a seizure increase during stimulation and underwent substitution and increase of one ASM, and one was a DBS responder (71% seizure decrease on average during entire follow-up) and underwent an exchange of one ASM due to side effects and a reduction of one ASM. One patient, who achieved temporary seizure freedom, was able to decrease the number of ASM. In two patients Perampanel was added, and in one Valproat was added, which may have influenced their psychiatric side effects, whether positively in the case of Valproate, or negatively in the case of Perampanel.
Presurgical evaluation including vEEG and/or stereo-EEG and 1.5 (due to VNS) or 3T MRI had taken place in all patients. Five of the patients in our cohort had undergone vagal nerve stimulation (VNS). Three of these were explanted at the time of initiating DBS, 1 remained implanted with an inactive VNS system, and 1 patient underwent simultaneous VNS (with constant stimulation parameters) and DBS stimulation. This patient suffered no side effects, and experienced a seizure reduction of 48.2% (average entire follow-up). One patient had undergone resective epilepsy surgery (lesionectomy of a left temporal cortical cavernoma) 12 years prior to implantation in another hospital. One patient had previously had a callosotomy 8 years prior.
Five patients remained implanted at the end of follow-up, and stimulation was ongoing in 3 patients (Figure 1). Causes for explantation or deactivation were: increased seizure frequency in 2 cases, side effects in 5 cases, and subjective insufficient seizure reduction in the remaining case.
Data on seizure control were complete in all 11 patients. When averaging seizure frequency during the entire follow-up period, six patients (54.5%) were responders (achieved seizure reduction of ≥50%) (average 73.6% reduction, range 50–94.9%, interquartile range (IQR) 49.75). One of these patients achieved temporary seizure freedom and near-total seizure reduction during the entire follow-up. Seizure reduction of <50% was achieved in 3 patients (average seizure reduction of 42.7%). Among these nine patients with seizure reduction, the average seizure decrease during entire follow-up was 58.7% (range 36.5–100%, IQR 44.85). The 2 remaining patients had a seizure frequency increase ranging from 21.3 to 33.3% (average 27.3%). During follow-up, all patients underwent neuropsychological testing and were explicitly asked about side effects, including mood disorders.
At 6 months, 10 of the 11 patients (90.9%) reported seizure frequency reduction (7%-99% reduction in seizure frequency, average 53.8%, IQR 60.8). Of these, 4 patients (36.4%) reported a seizure frequency reduction of <50% (7%-43%, IQR 21.95). One patient had a 33.3% seizure increase. The remaining 6 patients (54.5%) were responders. At this point in time, 2 patients presented with side effects, both of psychiatric nature (one patient presented with new-onset daily functional non-epileptic seizures, one patient showed an exacerbation of previously existing depression).
At 12 months, the device had been deactivated in 1 patient, who previously had experienced a seizure reduction of >80%, due to side effects (intolerable paresthesias along subcutaneous cable trajectory, exacerbation of pre-existing depression). Eight of the 10 patients (80%) reported seizure frequency reduction (range 28.6–100%, average 78.8%, IQR 12.9), seven of them of >50%, with one patient reporting seizure freedom (61.9–100%, average 76.9%, IQR 11.8). The two remaining patients, one of whom had previously reported an increase in seizure frequency, had dramatic seizure increases of more than double the preoperative seizure frequency (145 and 233% increase), leading to changes in anti-seizure medication. At this point in follow-up, 5 patients reported side effects (emergence of delusions and episodic agitation, functional non-epileptic seizures, burning dysesthesia along the cable trajectory, functional dysarthria and dysphagia, functional polydipsia and emergence of functional non-epileptic seizures). All side effects were reported by patients with seizure reduction during stimulation, except in the case of emergence of delusions and episodic agitation in a patient with seizure increase.
At last point of follow-up (beyond 12 months) of the ten patients who remained implanted and undergoing stimulation, seven patients (70%) reported a decrease in seizure frequency (43.3–100%, IQR 35.3), six of them of >50% (58.3–99.8%, IQR 33.1). The three remaining patients, including the 2 patients who had suffered a significant seizure increase at 12 months, had returned to their preoperative seizure frequency. All of the previously reported side effects persisted, and ultimately led to explantation or deactivation. No patients reported suicidal ideation at any time point.
Two of our patients had both electrodes implanted off-target. They had a seizure reduction of 71% and 50% during the entire follow-up. Both showed side effects (functional non-epileptic seizures and functional dysarthria and dysphagia) that ultimately led to deactivation and/or explantation. When removing these two patients from the analysis and averaging seizure frequency during the entire follow-up period, four patients (44.4%) were responders (average reduction 86.7%, range 65.6–94.9, IQR 18.97) and three experienced a seizure reduction of < 50% (average reduction 42.6%, range 36.5–48.15, IQR 11.65). This cohort included the two patients with seizure increase as well as the patient that achieved temporary seizure freedom and near-total seizure reduction during the entire follow-up.
At their simplest, the outcomes in terms of side effects and seizure frequency in our cohort can be summarized as follows:
- one patient suffered a seizure increase and no side effects (etiology unknown)
- one patient suffered a seizure increase coupled with intolerable psychiatric side effects (structural posttraumatic etiology)
- four patients experienced a reduction in seizure frequency and no side effects (2 structural etiology, 1 genetic generalized epilepsy)
- one reported seizure reduction and tolerable side effects (etiology unknown)
- and the remaining four patients experienced seizure reduction coupled with intolerable side effects (etiologies unknown in 3 patients, structural 1, Dynamin-1 mutation 1).
We created a model of electrode placement using the Lead-DBS toolbox for MatLab (14) (Figures 2A–C) with the DISTAL atlas for 3D visualization (15, 16). Eight of twenty-two active electrodes (36.4%) were outside of the ANT, both electrodes in two patients and one electrode in four patients. Only two of these patients had intolerable side effects (Figures 2A–C, black-ringed dots).
Figure 2. (A–C) Active electrode placements in the ANT (translucent blue). Blue dot—seizure reduction <50%; Green dot—seizure reduction ≥50%; Pink dot—seizure increase; Black ring around dot—intolerable side effects. Seizure outcomes displayed are averages over follow-up.
Both patients with seizure increase had one active electrode outside of the ANT (the right electrode in both cases). The 4 remaining patients had seizure decreases of 43.3–86.3%. Two of these four patients had both electrodes off-target.
Data on cognitive effects was complete in 8 of the 13 patients.
At baseline, i.e., after implantation and before stimulation, 4 of the 8 patients showed impairment in attention and executive functions (1 mild, 3 severe; no floor effects). Under DBS, the one patient with mild impairment significantly deteriorated to a severely impaired level, the other 7 were unchanged according to RCIs. Regarding episodic memory, in 6 of the 8 patients a deficit was registered at baseline (3 mild, 3 severe; no floor effects). Although we did not observe any statistically significant intraindividual memory changes under DBS, there were some categorical changes, i.e., under DBS all patients showed an impairment (4 mild, 4 severe).
To address the long-term effects of DBS on cognition we compared the preoperative neuropsychological profile with a long-term follow-up under DBS. The median interval between DBS implantation and follow-up assessment was 54.5 weeks. The preoperative cognitive profile of the 8 patients indicated deficits in attention and executive functions in 6 patients (2 mild, 4 severe), in verbal memory in 7 patients (2 mild, 5 severe), in figural memory in 6 patients (6 severe), in confrontative naming in 8 patients (1 mild, 7 severe), and in mental rotation in 3 patients (3 mild). Data did not indicate relevant floor effects that may have masked subsequent (significant) deteriorations. At the long-term follow-up, we observed significant intraindividual changes in verbal learning and memory in 5 of the 8 patients (3 deteriorated, 2 improved). In detail, 1 patient significantly declined in verbal learning and memory performance, 1 patient in verbal learning and recognition performance, and 1 in absolute delayed free recall. Regarding figural memory, none of the patients declined, 1 patient improved. The same is valid for attention and executive functions (1 improvement), confrontative naming (1 improvement, 2 missing), and mental rotation (1 improvement, 1 missing).
In our cohort, 81.8% of patients treated with ANT-DBS for refractory epilepsy experienced seizure reduction, with an average seizure reduction during entire follow-up of 58.7% (36.5–94.9%). Two patients (18.2%) suffered an average seizure increase of 27.3% during entire follow-up, with a period of significant seizure increase at 12 months. Intolerable side effects arose in 5 patients, mostly psychiatric in nature, and most commonly the emergence of functional neurological disorders.
In our cohort, several patients with multifocal epilepsies benefit from ANT-DBS, in accordance with other centers' experiences (17). Patients with genetic generalized epilepsies may also benefit from DBS. Psychiatric side effects were more common in our cohort than in other published cohorts, and were occasionally severe enough to entail explantation. This may be explained by the fact that more than a third of our implanted electrodes were off-target, compared to approximately 10% in SANTE (1, 2). It is also relevant that, at the time of implantation, usage of electrode model Medtronic 3387 was widespread. In the years of its use, the 3387 electrode had 1.5 mm spacing, with fewer contacts in ANT, compared to the current electrode with 0.5 mm spacing. Additionally, it was not known at that time that it was optimal to target the region of termination of the mammillothalamic tract. Both patients with seizure increase had one active electrode outside of the ANT (the right electrode in both cases). Interestingly, the 4 remaining patients (two of whom had both electrodes off-target) had seizure decreases of 43.3% to 86.3%. This may be due to a variety of reasons: firstly, stimulation in the ANT or in close proximity may be similarly effective. Secondly, the modeling of electrode placement using software cannot be expected to be 100% accurate. Thirdly, interindividual anatomical variation of the exact placement of the ANT may pose a challenge for neurosurgical targeting (18). Furthermore, published data support the hypothesis that proximity to the ANT alone does not correlate with seizure reduction in ANT-DBS, whereas proximity to the mammillothalamic junction does (19). The electrode placement of patients with intolerable side effects seemed to form a cluster in the anterolateral segment of the ANT (Figures 2A–C, black-ringed dots). Interestingly, patients with ≥50% seizure reduction similarly seemed to cluster in a narrow band of the mid- to anterior segment of the ANT (Figures 2A–C, green dots).
Wound-related side effects including paresthesias occurred in our cohort and seem to be among the most common undesirable outcomes of DBS, as described in the SANTE studies (1, 2). It is now known that they usually result from use of the stimulator case as the anode, and that if turning down the current does not relieve the paresthesias, then switching to bipolar stimulation with the stimulator and extension leads taken out of the circuit usually does (1, 2).
Regarding the acute cognitive effects of DBS, only 1 of the assessed patients showed a statistically significant deterioration in executive functions. Although there were no significant changes in verbal memory, 2 patients showed a de novo deficit after a non-significant decline. Long-term neuropsychological effects included significant intraindividual deteriorations as well as improvements in verbal learning and memory. Figural memory, attention and executive functions, confrontative naming and mental rotation were mostly unchanged, and improved in few cases. Though these findings are meaningful, pinpointing their exact cause is challenging: several factors may be at play, such as stimulation programming, stimulation site, and the effect of seizure reduction on cognition, among others.
Due to lack of high-level evidence, there are currently no available standardized treatment guidelines for ANT-DBS with detailed evidence-based stimulation settings. Nevertheless, recently a European expert-panel consensus paper and an international consensus paper (20, 21) issued a series of recommendations and causes for concern, as well as experience-based opinions on the implementation of ANT-DBS. The majority of the panel agreed on broad aspects of stimulation settings (initial monopolar stimulation, most parameters according to the SANTE study). Currently, two main aspects seem decisive, but uncertain, in the effectiveness of ANT-DBS in published works (5, 17, 22–25): patient recruitment (more specifically etiology of epilepsy), and optimal stimulation settings. One of the largest single-center cohorts of patients treated with ANT-DBS (22) followed a systematic approach beginning with voltages under 5V and with minimal medication changes, and reported a responder rate of 73.9%. Lower voltages are coupled with decreased risk of side effects and longer battery life, though patients with higher impedances may need higher amplitudes. When deciding whether to apply monopolar or bipolar stimulation, it is important to consider that monopolar settings result in a wider range of stimulated tissue. When this is coupled with higher voltage, adverse reactions may arise.
Five patients in our cohort had undergone VNS. One patient received concomitant stimulation from the VNS and ANT-DBS. Though initially, it was common practice to require deactivation and/or removal of the VNS system before proceeding with ANT-DBS, recent data shows that here were no complications related to concomitant VNS and ANT-DBS, and removal of VNS does not appear to be necessary (26). Since ANT-DBS and VNS affect seizure control through different mechanisms, concomitant implantation may even be beneficial in certain patients.
Our study is limited by the small sample size and the heterogeneity in patient characteristics. This rendered subgroup analyses uninformative. Though we have strived to offer a more complete picture of life after implantation of DBS by including neuropsychological and side effect outcomes, a more nuanced approach including sleep disruption, subjective impact on quality of life, etc. is needed. Furthermore, all epilepsy studies based on patient-reported seizure frequencies probably suffer from seizure under-reporting (27), and ours is no exception.
We confirm that we have read the Journal's position on issues involved in ethical publication and affirm that this report is consistent with those guidelines.
The raw data supporting the conclusions of this article will be made available by the authors, without undue reservation.
Ethical review and approval was not required for the study on human participants in accordance with the local legislation and institutional requirements. Written informed consent for participation was not required for this study in accordance with the national legislation and the institutional requirements.
KO: data curation (lead), data analysis (lead), statistical analysis (lead), MATLAB graphics (lead), writing—original draft preparation (lead), and writing—review and editing (equal). J-AW: neuropsychological data curation (lead), neuropsychological data analysis (lead), writing—original draft preparation (equal), and writing—review and editing (equal). RW: data curation (supporting) and writing—review and editing (equal). CH: supervision (equal), neuropsychological data analysis (supporting), writing—original draft preparation (supporting), and writing—review and editing (supporting). RS: supervision (equal), writing—original draft preparation (supporting), and writing—review and editing (equal). All authors contributed to the article and approved the submitted version.
We thank the reviewers for their valuable comments.
KO has received speaker fees from Eisai, unrelated to this work. RS has received fees as speaker or for serving on the advisory board from Angelini, Arvelle, Bial, Desitin, Eisai, Janssen-Cilag GmbH, LivaNova, Novartis, Precisis GmbH, UCB Pharma, UnEEG, and Zogenix. These activities were not related to the content of this manuscript.
The remaining authors declare that the research was conducted in the absence of any commercial or financial relationships that could be construed as a potential conflict of interest.
All claims expressed in this article are solely those of the authors and do not necessarily represent those of their affiliated organizations, or those of the publisher, the editors and the reviewers. Any product that may be evaluated in this article, or claim that may be made by its manufacturer, is not guaranteed or endorsed by the publisher.
1. Fisher R, Salanova V, Witt T, Worth R, Henry T, Gross R, et al. Electrical stimulation of the anterior nucleus of thalamus for treatment of refractory epilepsy. Epilepsia. (2010) 51:899–908. doi: 10.1111/j.1528-1167.2010.02536.x
2. Salanova V, Witt T, Worth R, Henry TR, Gross RE, Nazzaro JM, et al. Long-term efficacy and safety of thalamic stimulation for drug-resistant partial epilepsy. Neurology. (2015) 84:1017–25. doi: 10.1212/WNL.0000000000001334
3. Salanova V, Sperling MR, Gross RE, Irwin CP, Vollhaber JA, Giftakis JE, et al. The SANTÉ study at 10 years of follow-up: Effectiveness, safety, and sudden unexpected death in epilepsy. Epilepsia. (2021) 62:1306–17. doi: 10.1111/epi.16895
4. Hartl E, Feddersen B, Bötzel K, Mehrkens JH, Noachtar S. Seizure control and active termination by anterior thalamic deep brain stimulation. Brain Stimul. (2017) 10:168–70. doi: 10.1016/j.brs.2016.10.003
5. Hodaie M, Wennberg RA, Dostrovsky JO, Lozano AM. Chronic anterior thalamus stimulation for intractable epilepsy. Epilepsia. (2002) 43:603–8. doi: 10.1046/j.1528-1157.2002.26001.x
6. Tröster AI, Meador KJ, Irwin CP, Fisher RS. SANTE Study Group. Memory and mood outcomes after anterior thalamic stimulation for refractory partial epilepsy. Seizure. (2017) 45:133–41. doi: 10.1016/j.seizure.2016.12.014
7. Oh YS, Kim HJ, Lee KJ, Kim YI, Lim SC, Shon YM. Cognitive improvement after long-term electrical stimulation of bilateral anterior thalamic nucleus in refractory epilepsy patients. Seizure. (2012) 21:183–7. doi: 10.1016/j.seizure.2011.12.003
8. Helmstaedter C. EpiTrack® – Veränderungssensitives kognitives Screening zur Beurteilung der Aufmerksamkeit und der Exekutivfunktionen für die Qualitäts- und Ergebniskontrolle der Behandlung von Patienten mit Epilepsie. 3rd ed. Frankfurt: Eisai GmbH (2019).
9. Witt J-A, Elger CE, Helmstaedter C. Short-term and longer-term effects of brivaracetam on cognition and behavior in a naturalistic clinical setting – Preliminary data. Seizure. (2018) 62:49–54. doi: 10.1016/j.seizure.2018.09.016
10. Helmstaedter C, Lendt MLS VLMT Verbaler Lern- und Merkfähigkeitstest. Göttingen: Beltz Test GmbH (2001).
11. Helmstaedter C, Pohl C, Hufnagel A, Elger CE. Visual learning deficits in nonresected patients with right temporal lobe epilepsy. Cortex. (1991) 27:547–55. doi: 10.1016/S0010-9452(13)80004-4
14. Horn A, Kühn AA. Lead-DBS: a toolbox for deep brain stimulation electrode localizations and visualizations. Neuroimage. (2015) 107:127–35. doi: 10.1016/j.neuroimage.2014.12.002
15. Ewert S, Plettig P, Li N, Chakravarty MM, Collins DL, Herrington TM, et al. Toward defining deep brain stimulation targets in MNI space: a subcortical atlas based on multimodal MRI, histology and structural connectivity. Neuroimage. (2017). doi: 10.1101/062851
16. Chakravarty MM, Bertrand G, Hodge CP, Sadikot AF, Collins DL. The creation of a brain atlas for image guided neurosurgery using serial histological data. NeuroImage. (2006) 30:359-76. doi: 10.1016/j.neuroimage.2005.09.041
17. Herrera ML, Suller-Marti A, Parrent A, MacDougall K, Burneo JG. Stimulation of the anterior nucleus of the thalamus for epilepsy: a Canadian experience. Can J Neurol Sci. (2021) 48:469–78. doi: 10.1017/cjn.2020.230
18. Lehtimäki K, Möttönen T, Järventausta K, Katisko J, Tähtinen T, Haapasalo J, et al. Outcome based definition of the anterior thalamic deep brain stimulation target in refractory epilepsy. Brain Stimul. (2016) 9:268–75. doi: 10.1016/j.brs.2015.09.014
19. Freund BE, Greco E, Okromelidze L, Mendez J, Tatum WO, Grewal SS, et al. Clinical outcome of imaging-based programming for anterior thalamic nucleus deep brain stimulation. J Neurosurg. (2022) 1–8. doi: 10.3171/2022.7.JNS221116. [Epub ahead of print].
20. Kaufmann E, Bartolomei F, Boon P, Chabardes S, Colon AJ, Eross L, et al. European Expert Opinion on ANT-DBS therapy for patients with drug-resistant epilepsy (a Delphi consensus). Seizure. (2020) 81:201–9. doi: 10.1016/j.seizure.2020.08.015
21. Fasano A, Eliashiv D, Herman ST, Lundstrom BN, Polnerow D, Henderson JM, Fisher RS. Experience and consensus on stimulation of the anterior nucleus of thalamus for epilepsy. Epilepsia. (2021) 62:2883–98 doi: 10.1111/epi.17094
22. Kaufmann E, Bötzel K, Vollmar C, Mehrkens JH, Noachtar S. What have we learned from 8 years of deep brain stimulation of the anterior thalamic nucleus? Experiences and insights of a single center. J Neurosurg. (2020) 135:1–10. doi: 10.3171/2020.6.JNS20695
23. Krishna V, King NKK, Sammartino F, Strauss I, Andrade DM, Wennberg RA, et al. Anterior nucleus deep brain stimulation for refractory epilepsy: insights into patterns of seizure control and efficacious target. Neurosurgery. (2016) 78:802–11. doi: 10.1227/NEU.0000000000001197
24. Osorio I, Overman J, Giftakis J, Wilkinson SB. High frequency thalamic stimulation for inoperable mesial temporal epilepsy. Epilepsia. (2007) 48:1561–71. doi: 10.1111/j.1528-1167.2007.01044.x
25. Kuncel AM, Grill WM. Selection of stimulus parameters for deep brain stimulation. Clin Neurophysiol. (2004) 115:2431–41. doi: 10.1016/j.clinph.2004.05.031
26. Parisi V, Lundstrom BN, Kerezoudis P. Alcala Zermeno JL, Worrell GA, Van Gompel JJ. Anterior nucleus of the thalamus deep brain stimulation with concomitant vagus nerve stimulation for drug-resistant epilepsy. Neurosurgery. (2021) 89:686–94. doi: 10.1093/neuros/nyab253
Keywords: deep brain stimulation, refractory epilepsy, cognition, side effects, neuropsychological assessment
Citation: Olaciregui Dague K, Witt J-A, von Wrede R, Helmstaedter C and Surges R (2023) DBS of the ANT for refractory epilepsy: A single center experience of seizure reduction, side effects and neuropsychological outcomes. Front. Neurol. 14:1106511. doi: 10.3389/fneur.2023.1106511
Received: 23 November 2022; Accepted: 21 February 2023;
Published: 09 March 2023.
Edited by:
Xiangping Chu, University of Missouri–Kansas City, United StatesReviewed by:
Anteneh Feyissa, Mayo Clinic Florida, United StatesCopyright © 2023 Olaciregui Dague, Witt, von Wrede, Helmstaedter and Surges. This is an open-access article distributed under the terms of the Creative Commons Attribution License (CC BY). The use, distribution or reproduction in other forums is permitted, provided the original author(s) and the copyright owner(s) are credited and that the original publication in this journal is cited, in accordance with accepted academic practice. No use, distribution or reproduction is permitted which does not comply with these terms.
*Correspondence: Karmele Olaciregui Dague, a2FybWVsZS5vbGFjaXJlZ3VpX2RhZ3VlQHVrYm9ubi5kZQ==
Disclaimer: All claims expressed in this article are solely those of the authors and do not necessarily represent those of their affiliated organizations, or those of the publisher, the editors and the reviewers. Any product that may be evaluated in this article or claim that may be made by its manufacturer is not guaranteed or endorsed by the publisher.
Research integrity at Frontiers
Learn more about the work of our research integrity team to safeguard the quality of each article we publish.