- 1Neuroscience Research Group (NRG), Universal Scientific Education and Research Network (USERN), Tehran, Iran
- 2School of Medicine, Iran University of Medical Sciences, Tehran, Iran
- 3Department of Neuroscience and Padova Neuroscience Center (PNC), University of Padova, Padua, Italy
Background: The traditional approach to studying the neurobiological mechanisms of brain disorders and localizing brain function involves identifying brain abnormalities and comparing them to matched controls. This method has been instrumental in clinical neurology, providing insight into the functional roles of different brain regions. However, it becomes challenging when lesions in diverse regions produce similar symptoms. To address this, researchers have begun mapping brain lesions to functional or structural networks, a process known as lesion network mapping (LNM). This approach seeks to identify common brain circuits associated with lesions in various areas. In this review, we focus on recent studies that have utilized LNM to map neurological and psychiatric symptoms, shedding light on how this method enhances our understanding of brain network functions.
Methods: We conducted a systematic search of four databases: PubMed, Scopus, and Web of Science, using the term “Lesion network mapping.” Our focus was on observational studies that applied lesion network mapping in the context of neurological and psychiatric disorders.
Results: Following our screening process, we included 52 studies, comprising a total of 6,814 subjects, in our systematic review. These studies, which utilized functional connectivity, revealed several regions and network overlaps across various movement and psychiatric disorders. For instance, the cerebellum was found to be part of a common network for conditions such as essential tremor relief, parkinsonism, Holmes tremor, freezing of gait, cervical dystonia, infantile spasms, and tics. Additionally, the thalamus was identified as part of a common network for essential tremor relief, Holmes tremor, and executive function deficits. The dorsal attention network was significantly associated with fall risk in elderly individuals and parkinsonism.
Conclusion: LNM has proven to be a powerful tool in localizing a broad range of neuropsychiatric, behavioral, and movement disorders. It holds promise in identifying new treatment targets through symptom mapping. Nonetheless, the validity of these approaches should be confirmed by more comprehensive prospective studies.
Introduction
For many years, the method of understanding the function of a specific brain region was through the study of focal brain lesions that occurred as a result of strokes, tumors, and hemorrhages. If patients with similar symptoms had overlapping lesions in a specific brain region, we could pinpoint those neurological symptoms or behavioral deficits to that region (1, 2). Traditional lesion mapping has been the cornerstone of clinical neurology, providing valuable insights into the functional roles of different brain regions. However, most neurological and psychiatric symptoms cannot be traced back to a single region; instead, they involve a network of interconnected regions (3). Additionally, patients exhibiting similar symptoms may have lesions located in diverse areas, which poses a challenge to traditional lesion mapping in pinpointing those symptoms (4). Such symptom overlap could potentially be due to the disruption of an underlying, cohesive brain network (5, 6).
One strategy to address this challenge is through the implementation of lesion network mapping (LNM), a methodology that connects brain lesions to either functional or structural networks to identify common brain circuits tied to diverse lesion locations (4, 7).
This technique operates on the hypothesis that a lesion present at any location within a network mapped for a specific symptom has the potential to trigger that symptom (4, 8). The process of performing LNM overlap studies comprises four stages: Firstly, lesions are traced into a standardized brain atlas, establishing a foundation for connectivity analyses (9) (Figure 1). Secondly, an assessment is undertaken to determine the connectivity of each lesion location with other brain regions, using either structural or functional normative human connectome data. Thirdly, correlations between lesion locations and all other brain voxels are thresholded to delineate a map of interconnected regions. Finally, these maps from each patient are superimposed to pinpoint brain regions that are most frequently connected to lesion locations associated with the symptom in question (10). In addition, certain studies incorporate voxelwise statistical analyses, either by leveraging continuous outcomes or by contrasting patients with controls, with the aim of elucidating common networks associated with symptomatic lesions (11, 12). The availability of large-scale functional and anatomical normative maps, like those offered by the Human Connectome Project data, provide a robust foundation for correlating lesion locations with a shared network, thereby facilitating the study of neurological and psychiatric symptoms (13).
This methodology has been broadly utilized since its introduction in 2015 for the localization of an array of neuropsychiatric, behavioral, and movement disorders (8, 10, 14–18). Previous investigations have substantiated the validity of LNM; while several outcomes confirmed primary hypotheses concerning the neuroanatomical underpinnings of specific symptoms (19), some unexpected findings also emerged from LNM studies. For instance, a notable association between the putamen and hemichorea-hemiballism was identified, despite earlier evidence suggesting the involvement of the subthalamic nucleus in the genesis of hemichorea-hemiballism (20). As such, LNM may offer an innovative avenue to further investigate the functionality of brain networks.
The intent of this systematic review is to spotlight recent studies that have utilized LNM to map neurological and psychiatric symptoms, thereby providing insight into how this methodology enhances our comprehension of the function of distinct brain regions.
Methods and materials
This systematic review was conducted in adherence to the Preferred Reporting Items for Systematic Reviews and Meta-Analyses (PRISMA) guidelines (21).
Literature search and eligibility criteria
A literature search was performed on PubMed, Scopus, Embase, and Web of Science in June 2022 using the term “Lesion network mapping.” In addition, we manually reviewed the reference lists of pertinent review studies to identify relevant research. Inclusion criteria encompassed observational studies on LNM in neurological and psychiatric disorders. Exclusions were made for case reports, review articles, and non-English language studies.
Study selection
Two researchers (F.N, M.A) independently examined the titles and abstracts and eliminated irrelevant studies. Subsequently, the remaining articles’ full texts were scrutinized, and studies were selected based on our eligibility criteria.
Data extraction
Data was collated from the selected studies using a pre-designed data sheet. The collected information included: author, publication year, study design, data source, sample size, age, gender distribution, study duration, underlying disease, lesion cause, lesion location, lesion type, investigated symptom or disorders, number of subjects with investigated symptoms or disorders, analytical software used, normative data, and LNM findings.
Quality assessments
The quality of the included studies was evaluated using the Newcastle-Ottawa scale (NOS) for observational studies, which operates on a scoring range from 0 to 8 (22).
Results
Search results
Our literature search and additional records resulted in 69 studies after eliminating duplicates (Figure 2). Post title and abstract screening, 10 studies were excluded. Ultimately, a total of 52 studies involving 6,814 subjects were included in our systematic review following a full-text review (4–8, 10–12, 14–20, 23–57).
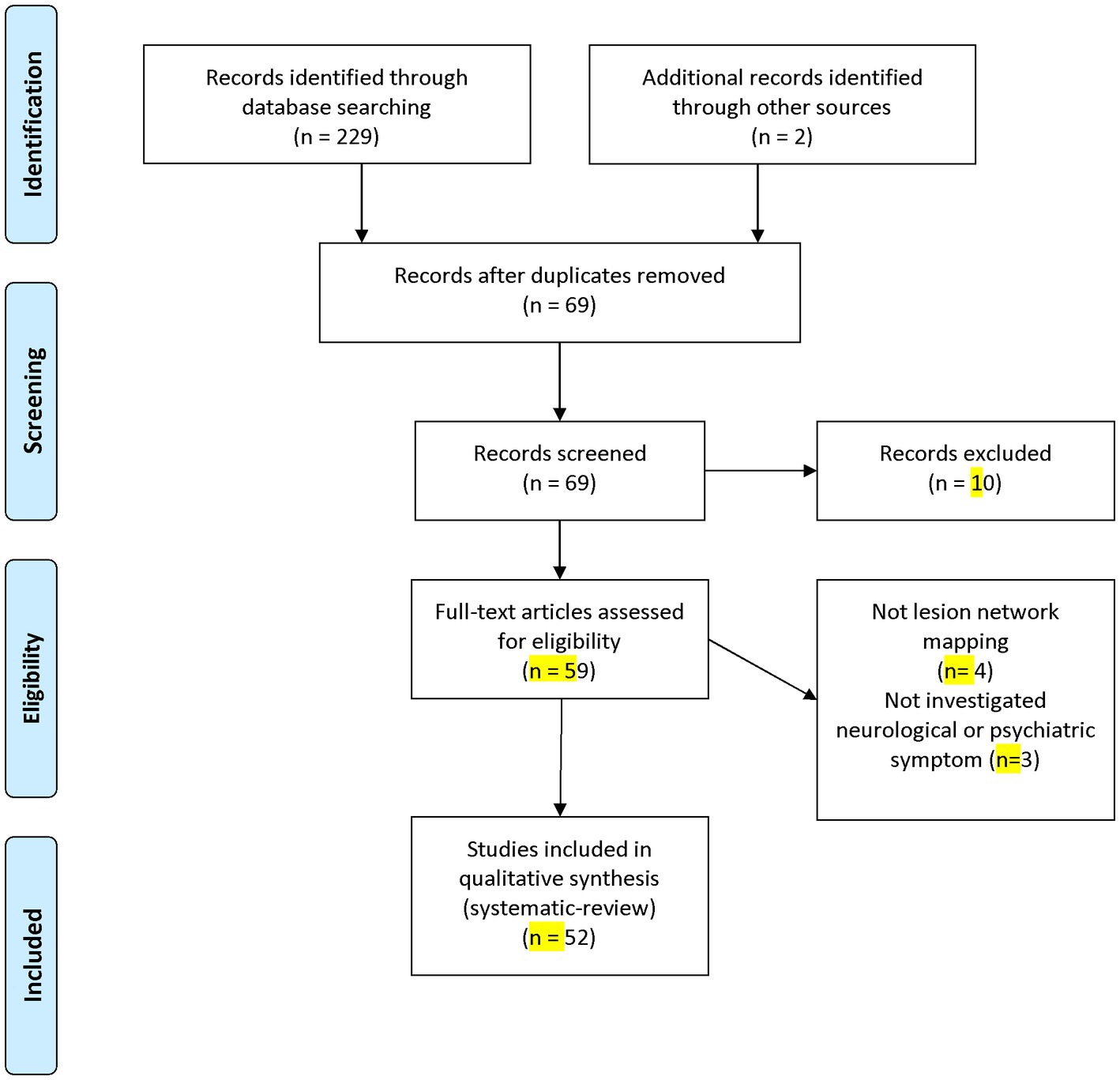
Figure 2. PRISMA flow diagram depicting the flow of information through the different phases of a systematic review.
Among the incorporated studies, 39 were cross-sectional, ten were case–control, two were cohort studies, and the remaining one was a longitudinal study. In terms of data sources, 30 studies utilized private data, 20 used published case reports, and two studies employed both private data and published case reports. Notably, 41 studies utilized FSL as their analytical software. A detailed overview of the characteristics of the included studies can be found in Table 1. Among the analyzed studies, functional LNM was performed in 38, structural LNM was conducted in 7, and both forms of LNM were employed in 7 studies. The quality assessment indicated that the mean NOS score of the included studies was 7.48.
Functional lesion network mapping
Non-motor symptoms were found to be associated with fronto-parieto-temporal networks (24), sensorimotor and ventral attention networks (29), and the thalamic mediodorsal nucleus (14). Executive function deficits demonstrated connectivity with the anterior cingulate cortex, dorsomedial prefrontal cortex, and frontoparietal network (16). Symptoms such as prosopagnosia, anosognosia for hemiplegia, and diminished mind-wandering revealed connections to the left frontal cortex, anterior cingulate cortex, right fusiform face area (10), right posterior hippocampus (33), and left inferior parietal lobule (35) respectively.
Symptoms such as loss of consciousness, mania, and delusional misidentifications were associated with connectivity to the dorsal brainstem (37), right orbitofrontal cortex, right inferior temporal gyrus, right frontal pole (27), and left retro splenial and right frontal cortex (7). Additionally, hallucinations were linked to the extrastriate visual cortex (4) and to the cerebellar vermis, inferior cerebellum, and right superior temporal sulcus (5). Lesions causing autoscopic phenomena showed functional connections to the bilateral temporoparietal junction (23).
Cortical vertigo showed associations with connectivity to the posterior insula (52). Lesions causing obsessive–compulsive disorder (OCD) and depression were linked to the dorsal anterior cingulate cortex and left dorsolateral prefrontal cortex (11, 30).
Findings indicate that behavioral deficits were better predicted by direct measures of functional MRI connectivity than indirect functional disconnection (38). Moreover, Darby et al. (15) identified that criminal behavior was associated with a shared network encompassing the inferior orbitofrontal cortex, anterior temporal lobes, and intraparietal sulcus (Table 2).
Movement disorders, such as essential tremor, Parkinsonism, freezing of gait, and Holmes tremor, were linked to networks involving the cerebellum and thalamus (32), midbrain, basal ganglia, cingulate cortex, and cerebellum (19), and red nucleus, thalamus, globus pallidus, and cerebellum (17), respectively.
Hemichorea hemiballism, cervical dystonia, and increased fall risk were associated with the posterolateral putamen (20), cerebellum and somatosensory cortex (26), and the dorsal attention network (28), respectively. Additionally, asymmetric step length after a unilateral stroke demonstrated functional connectivity to the dorsolateral prefrontal cortex (18).
Speech disorders such as semantic aphasia, foreign accent syndrome, and apraxia of speech were associated with distinctive patterns of structural and functional disconnection (58), and networks involving the bilateral lower and middle portions of the precentral gyrus and medial frontal cortex (31).
Studies using functional connectivity revealed several region and network overlaps across different movement or psychiatric disorders (Figure 3 and Table 3). The cerebellum appeared in a common network for conditions such as essential tremor relief (32), parkinsonism (19), Holmes tremor (17), freezing of gait (8), cervical dystonia (26), and tics (6). It also showed functional connections to tuberous sclerosis lesions in children with infantile spasms (59). The thalamus demonstrated involvement in a common network for essential tremor relief (32) and Holmes tremor (17), and showed significant functional connectivity with lesions associated with executive function deficits (16). The midbrain, basal ganglia, and cingulate cortex were connected to lesions causing parkinsonism (19) and were part of the common network implicated in tics (6).
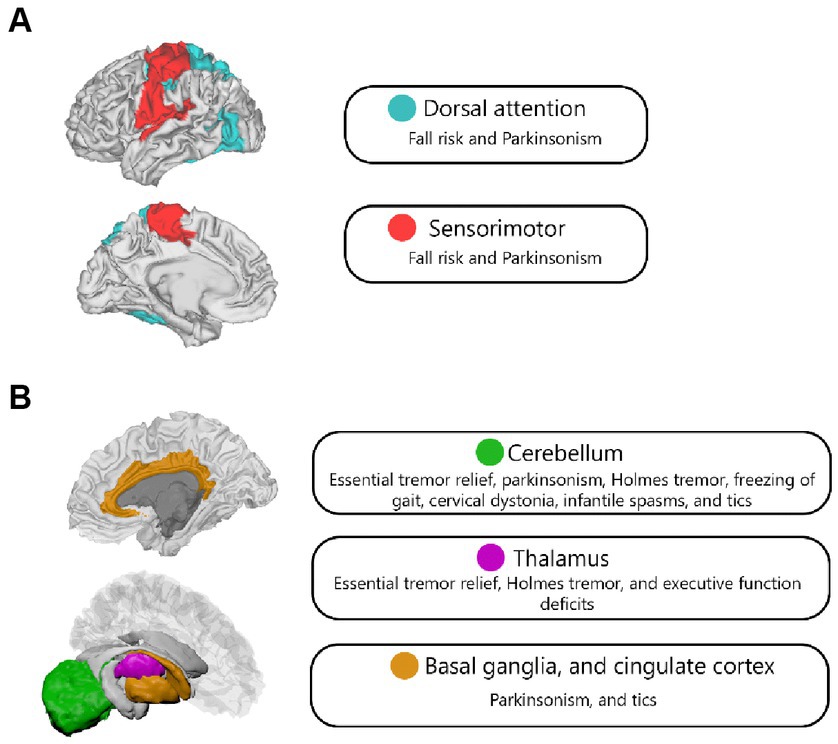
Figure 3. Network (A) and region (B) overlap across different psychiatric and neurological disorders in functional LNM studies.
The dorsal attention network was significantly correlated with the Physiological Profile Assessment (PPA) score, which measures fall risk in elderly individuals (28), and demonstrated functional connectivity related to focal brain lesions causing parkinsonism (19). It was also part of the frontoparietal network, which showed functional connectivity overlap in regions connected to epileptogenic mass lesions (34). Moreover, the sensorimotor network showed significant overlap in lesions causing cognitive impairment assessed by the MoCA score (29), and was connected to resection cavity maps in patients with body awareness disorders (42).
Structural lesion network mapping
The emerging evidence underscores the reciprocal nature of structural and functional Lesion Network Mapping (LNM) in elucidating the nexus between cerebral lesions and cognitive functionality (24, 29, 51). Structural mapping has pinpointed specific regions, such as the right insular and frontal operculum, superior temporal gyrus, and putamen, whose impairment tends to precipitate cognitive deficits (51). Concurrently, functional mapping has demystified the distinctive brain networks correlated with various cognitive faculties, exemplified by the fronto-parieto-temporal network (24).
The intricate neural networks engaged in spatial perception have been brought to light, with aberrations in the right ventrolateral prefrontal and right temporal clusters linked to spatial delusions (47). Importantly, the integral role of the left retro splenial and right frontal cortex in spatial information processing has been underscored, underlining their pivotal contribution to spatial cognition and awareness (48).
Furthermore, the studies spotlight the necessity of incorporating structural connectivity into the neurological evaluation of stroke sequelae for optimal therapeutic results (38). Structural LNM exhibited superior reliability in forecasting post-stroke behavioral repercussions compared to its functional counterpart. However, there were instances where direct measures of functional connectivity outperformed, underscoring the indispensable role of a holistic evaluation of both structural and functional connectivity to tailor personalized therapeutic interventions for stroke survivors.
The critical role of the frontal lobe in gait regulation was reaffirmed in the context of motor and speech disorders (18). The choice of LNM methodology appears to be contingent upon the specific functional impairment in question, with functional mapping outperforming in the domain of language deficits, while structural mapping took the lead for motor deficits (24). Both techniques unveiled significant pathways, indicating that diverse lesion types might disrupt distinct neural circuits, thus informing rehabilitative strategies post-stroke (45). For example, the left anterior thalamic radiation and bilateral superior longitudinal fasciculus were highlighted as significant pathways by both methodologies (45).
Lastly, the studies emphasized the significance of comprehending the intricate structural praxis network in limb apraxia patients (53). The indirect structural disconnection method discerned significant pathological alterations in the white matter within an extensively interconnected fronto-temporo-parietal network, incorporating both short and long-distance association fibers (53). This revelation suggests that the disparate topographical outcomes reported in earlier lesion mapping studies might not exclusively arise from methodological variations but could also be attributed to the inherent limitations of univariate topographical mapping techniques.
Discussion
This review consolidates and critically assesses existing knowledge on the application of Lesion Network Mapping (LNM) methods in the diagnosis and treatment of neurological and psychiatric disorders. Our findings underscore the complementary role of functional and structural LNM, emphasizing their potential utility in improving therapeutic outcomes in neurological afflictions. Our analysis draws attention to certain brain regions and networks integral to specific neurological domains. For instance, the fronto-parieto-temporal network emerged as pivotal to cognitive functioning, while the dorsal anterior cingulate cortex and the left dorsolateral prefrontal cortex demonstrated considerable relevance for obsessive–compulsive disorder. These insights indicate that LNM may aid in pinpointing viable targets for neuromodulation interventions across various neurological disorders. The examined studies also underlined the indispensable role of LNM in deciphering the intricacies of motor and speech disorders. These studies highlighted the use of LNM in identifying brain regions and neural pathways implicated in diverse movement disorders, such as Parkinsonism, Essential Tremor, and Holmes Tremor. Consequently, we can anticipate LNM’s instrumental role in informing neuromodulation strategies tailored to specific motor and speech disorders, such as cervical dystonia and hemichorea hemiballismus. Our results suggest that the choice of LNM method should align with the specific type of functional deficit under consideration. Since the advent of LNM studies in 2015, numerous investigations have successfully employed this technique to elucidate how alterations in functional and structural networks can account for symptoms post focal brain lesions.
Moreover, our analysis identified several overlaps between different regions and networks implicated in diverse psychiatric and neurological disorders. Both structural (51) and functional LNM (24, 29) pinpointed the fronto-parieto-temporal network as integral to cognitive function, thereby asserting a potent correlation between this network and cognitive impairment. Furthermore, studies revealed that damage to the right ventrolateral prefrontal and right temporal cluster (47), coupled with alterations in the left retro splenial and right frontal cortex (7), could precipitate spatial perception deficits and engender delusions of space. Intriguingly, both functional LNM (54) and structural disconnectome-based analyses (57) associated the left dorsolateral prefrontal cortex with depression. This concurrence insinuates a common neural foundation for depressive symptoms, stressing the necessity of considering both structural and functional connectivity when attempting to comprehend the neurological underpinnings of varying disorders and enhancing treatment outcomes. The complex neural networks implicated in these disorders can guide the formulation of tailored treatment strategies and facilitate a deeper understanding of their neurobiological origins. By delineating these overlaps and parallels, we underscore the interwoven nature of the brain and its instrumental role across an array of disorders.
Our study unearthed a significant overlap within the thalamus for essential tremor relief, Holmes tremor, and executive function deficits. This intriguing finding challenges the conventional practice of symptom localization that ascribes particular symptoms to specific, isolated brain regions. Instead, it accentuates the importance of investigating the complex interactions among various brain regions and acknowledges the distributed nature of neural processing (4, 24). This shared neural substrate within the thalamus proposes potential unified therapeutic targets for these conditions, underscoring the imperative for a more holistic approach when studying neurological and psychiatric disorders. By harmonizing the strengths of both conventional localization and network-based perspectives, researchers can attain a comprehensive understanding of the brain’s structure and function, ultimately leading to more effective treatment strategies for a multitude of disorders.
The neurobiological mechanisms underlying brain disorders and the localization of brain function are traditionally explored by identifying abnormalities within the brain and contrasting them with matched controls, as exemplified by the study of hemiparesis following stroke lesions. However, this process becomes complex when the lesion is located in an unanticipated region or when numerous heterogeneous lesions are observed in disparate locations. For instance, hemiparkinsonism patients with varied causal lesions outside the nigrostriatal tract were found to map onto a common network (19). Moreover, most neurological and psychiatric symptoms cannot be ascribed to a single region; instead, they involve a network of interconnected areas. As such, LNM has proven to be a significant advance over traditional lesion analysis, enabling the localization of symptoms across different lesion locations, a task that was previously unachievable (60).
Focal brain lesions have been observed to modify resting functional connectivity and reduce the variability of neural states, thereby limiting the brain’s ability to process information (59, 61, 62). Empirical evidence from previous studies has indicated that alterations in functional connectivity following focal brain lesions are not restricted to a singular network but engage numerous regions (63). Strokes tend to affect white matter and subcortical regions more often than the cortex. Given that white matter contains numerous fiber pathways, strokes within these regions can result in widespread alterations (59, 64).
The pioneering study employing LNM was conducted by Boes et al. (4), investigating peduncular hallucinosis subsequent to subcortical lesions. These lesions were hypothesized to disrupt the extrastriate visual cortex, despite their heterogeneous locations. The researchers found that 22 out of 23 lesions demonstrated a negative correlation with the extrastriate visual cortex. Moreover, a study by Kim et al. discovered that lesions causing hallucinations localized to a shared brain network, encompassing the cerebellar vermis, inferior cerebellum, and the right superior temporal sulcus (5). Following the promising results of this inaugural LNM study, the method was applied to elucidate long-standing neurological enigmas characterized by heterogeneous lesions dispersed across different regions (6, 18, 31).
Considering the comparable effects of deep brain stimulation (DBS) and therapeutic lesions, a promising avenue for LNM involves leveraging the identified regions associated with disorders or common networks linked to beneficial brain lesions as therapeutic targets for DBS (65, 66). A pertinent example is the observed clinical improvement in patients where DBS electrode connectivity was in the claustrum, which was also identified as a shared network for lesions causing parkinsonism via LNM (19). Further, the exploration of beneficial brain lesions that alleviate symptoms to pinpoint optimal DBS targets should extend to various neurological or psychiatric disorders. However, it’s important to note that beneficial brain lesions are exceedingly rare. A study by Joutsa et al. demonstrated that varied lesion locations resulting in essential tremor relief overlapped in a common network within the cerebellum and thalamus, known targets for deep brain stimulation in the management of tremors (32).
Furthermore, a recent study by the same group delved into brain lesions associated with addiction improvement, identifying the paracingulate gyrus and the left frontal operculum. The medial frontopolar cortex emerged as the best-matching connectivity profile for addiction remission (39). These discoveries could pave the way to optimal treatment targets for addiction disorders, lending support to ongoing neuromodulation trials (67). However, it is clear that additional research is required to understand how to extrapolate and interpret LNM findings to identify the most suitable therapeutic targets.
When lesion connections coincide within a single brain network, it’s reasonable to infer that the network has a causative role in symptom production. Nevertheless, the regions at the network’s core may not be crucial in symptom development (68). The correlation between symptoms and the central region of the network is gleaned from brain connectivity patterns. Consequently, regional associations gleaned from LNM must be appraised in comparison with functional neuroimaging results.
A prominent question within LNM pertains to the neurobiological mechanism at play when a network is disrupted by lesions. Although lesions induce dysfunction at their locations, the remote impact of lesions on functional loss in interconnected regions—dependent on the type of connection—remains a topic of debate (69). Dysfunctions in excitatory connections could lead to decreased activity, while disruption in inhibitory connections could result in increased activity (26). Furthermore, it remains uncertain whether the labeling of functional connectivity as positive or negative can indicate decreases or increases in activity, respectively.
LNM’s focus has largely been on the spatial aspect of symptoms induced by lesions, often neglecting the temporal component. Investigating the temporal aspect of symptoms is just as important, given that symptoms evolve over time due to recovery processes and dynamic changes post-injury (3).
Limitation and future direction
While the development of LNM has yielded intriguing results, complete elucidation of neurological enigmas via this method alone remains a considerable distance away. More robust studies are necessary, particularly those utilizing prospective data that assess pre-and post-lesion symptoms. Salvalaggio et al. (38) have previously suggested that direct and indirect measures of functional networks may not be as sensitive to behavioral deficits compared to using structural disconnections, possibly due to the coincidence of structural disconnections with structural damage following lesions. However, Cohen et al. contended that the poor outcome of LNM in predicting behavioral deficits in Salvalaggio et al.’s study could be attributed to methodological considerations (25). Ferguson et al. (40) presented contrasting results, affirming the value of LNM in localizing behavioral deficits. These discrepancies underscore the need for future studies to compare and enhance LNM methods. Furthermore, the efficacy of LNM in identifying therapeutic targets should be examined by strategically placing DBS electrodes in the proposed hub of a network (69).
Cotovio et al. (27) probed the effects of utilizing distinct connectomes on LNM outcomes for mania, concluding that the findings remained consistent and reliable, regardless of the specific connectome used for analysis. Future directions could involve refining normative connectome atlases using higher resolution imaging, integrating results from various connectomes obtained during different tasks, and employing age-and sex-matched datasets for each patient. As functional and structural normative data often involve young, healthy individuals, employing normative lifespan data could yield deeper insights into brain function and enable more accurate symptom mapping. While most studies use normative connectomes, the exploration of disease-specific connectomes may provide more precise results.
Moreover, Bonkhoff et al. (70) discovered that women tend to experience more severe strokes than men, and a recent large-scale study highlighted the effect of sex on neuroimaging metrics over time (71). Thus, incorporating sex-specific normative data in LNM could enhance the precision of this approach.
While we have primarily considered functional and structural networks as relatively stable entities in this review, research increasingly shows that these networks are dynamically changing over time. These temporal fluctuations in connectivity patterns are believed to be crucial for flexible cognitive function, and disturbances in these dynamics have been linked to various neurological and psychiatric conditions. Incorporating these dynamic changes into LNM analyses could therefore potentially increase the sensitivity of the method to detect functional abnormalities linked to behavioral deficits. This integration of dynamics would be a novel addition to the methodology and potentially offers a new avenue for understanding brain function and dysfunction.
Another important discussion point to add is the development and application of machine learning techniques in the context of LNM. Recent advancements in machine learning and artificial intelligence (AI) present exciting opportunities for further refining and enhancing the predictive power of LNM. Machine learning models could be trained to predict the likelihood of specific deficits or symptom severity based on the observed lesion distribution and connectivity disruptions. Such models could provide clinicians with additional tools for prognosis and treatment planning, complementing the traditional, more qualitative approaches.
Lastly, the inter-individual variability in brain connectivity and anatomy should not be overlooked. Even among healthy individuals, there can be substantial differences in the structure and functional connectivity of the brain. This variability might influence the impact of a lesion on cognitive and behavioral function and might partly explain the heterogeneity observed in clinical outcomes following brain damage. Future studies could consider incorporating measures of inter-individual variability into the LNM framework to provide more personalized predictions about treatment outcomes. This could be especially relevant when considering DBS targets, as the optimal target might differ slightly between individuals due to this variability.
These proposed additions aim to enrich the current findings and provide a more comprehensive view of the applications and potential advancements in lesion network mapping.
Methodological consideration
There are several important methodological considerations that should be addressed in LNM.
Normalization and standardization
While LNM has proven to be a powerful tool in understanding brain disorders, it’s important to note that the results are highly dependent on the normalization and standardization of brain images. Subtle variations in these processes can lead to significant differences in results. Moreover, the choice of template used for spatial normalization can significantly impact the localization of brain regions and networks. Future studies should aim to implement standardized preprocessing pipelines to ensure the reliability and reproducibility of results.
Limitations of normative connectomes
Most studies used normative connectomes in their LNM analyses. Although this approach provides a general framework for analyzing brain networks, it overlooks inter-individual variability. Individualized connectomes that account for each patient’s unique brain architecture may provide more precise mapping results and should be the focus of future research.
Thresholding and statistical analysis
The manner in which network connections are thresholded and analyzed can greatly influence the results of LNM. The application of overly stringent thresholds may fail to capture weaker, yet potentially significant, network connections, whereas lenient thresholds may lead to false positive findings. The choice of statistical tests and corrections for multiple comparisons also plays a crucial role in the interpretation of results.
Choice of parcellation scheme
The way in which the brain is divided into distinct regions, or parcels, can significantly impact the results of network analyses. Different parcellation schemes can yield differing, and sometimes conflicting, results. Hence, the choice of parcellation scheme should be carefully considered and justified in future LNM studies.
Multimodal integration
The integration of multiple imaging modalities (e.g., structural MRI, functional MRI, diffusion tensor imaging) can provide complementary insights into the brain’s structure–function relationships. Despite this, many studies still focus on a single imaging modality, potentially missing important aspects of network functionality and connectivity.
While LNM presents an exciting tool in neurology and neuroimaging, attention to these methodological considerations is critical for the advancement of the field and to ensure the reliability and validity of findings.
Conclusion
LNM offers solid findings in localizing a wide range of neuropsychiatric, behavioral, and movement disorders. Furthermore, LNM is anticipated to identify new treatment targets through symptom mapping. However, the veracity of these methodologies must be validated through more comprehensive prospective studies.
Data availability statement
The original contributions presented in the study are included in the article/supplementary material, further inquiries can be directed to the corresponding author.
Author contributions
FN and MA contributed to searching and writing and revising the manuscript. All authors contributed to the article and approved the submitted version.
Funding
MA is funded by the EU-project euSNN European School of Network Neuroscience (MSCA-ITN-ETN H2020-860563).
Conflict of interest
The authors declare that the research was conducted in the absence of any commercial or financial relationships that could be construed as a potential conflict of interest.
Publisher’s note
All claims expressed in this article are solely those of the authors and do not necessarily represent those of their affiliated organizations, or those of the publisher, the editors and the reviewers. Any product that may be evaluated in this article, or claim that may be made by its manufacturer, is not guaranteed or endorsed by the publisher.
References
1. Raymont, V, Salazar, AM, Krueger, F, and Grafman, J. "studying injured minds" - the Vietnam head injury study and 40 years of brain injury research. Front Neurol. (2011) 2:15. doi: 10.3389/fneur.2011.00015
2. Rorden, C, and Karnath, HO. Using human brain lesions to infer function: a relic from a past era in the fMRI age? Nat Rev Neurosci. (2004) 5:813–9. doi: 10.1038/nrn1521
3. Fornito, A, Zalesky, A, and Breakspear, M. The connectomics of brain disorders. Nat Rev Neurosci. (2015) 16:159–72. doi: 10.1038/nrn3901
4. Boes, AD, Prasad, S, Liu, H, Liu, Q, Pascual-Leone, A, Caviness, VS, et al. Network localization of neurological symptoms from focal brain lesions. Brain. (2015) 138:3061–75. doi: 10.1093/brain/awv228
5. Kim, NY, Hsu, J, Talmasov, D, Joutsa, J, Soussand, L, Wu, O, et al. Lesions causing hallucinations localize to one common brain network. Mol Psychiatry. (2021) 26:1299–309. doi: 10.1038/s41380-019-0565-3
6. Ganos, C, Al-Fatly, B, Fischer, JF, Baldermann, JC, Hennen, C, Visser-Vandewalle, V, et al. A neural network for tics: insights from causal brain lesions and deep brain stimulation. Brain. (2022) 145:4385–97. doi: 10.1093/brain/awac009
7. Darby, RR, Laganiere, S, Pascual-Leone, A, Prasad, S, and Fox, MD. Finding the imposter: brain connectivity of lesions causing delusional misidentifications. Brain. (2017) 140:497–507. doi: 10.1093/brain/aww288
8. Fasano, A, Laganiere, SE, Lam, S, and Fox, MD. Lesions causing freezing of gait localize to a cerebellar functional network. Ann Neurol. (2017) 81:129–41. doi: 10.1002/ana.24845
9. Joutsa, J, and Fox, MD. Chapter 15 - using brain lesions to inform connectomic DBS In: A Horn , editor. Connectomic Deep Brain Stimulation. Cambridge: Academic Press (2022). 325–37.
10. Cohen, AL, Soussand, L, Corrow, SL, Martinaud, O, Barton, JJS, and Fox, MD. Looking beyond the face area: lesion network mapping of prosopagnosia. Brain. (2019) 142:3975–90. doi: 10.1093/brain/awz332
11. Padmanabhan, JL, Cooke, D, Joutsa, J, Siddiqi, SH, Ferguson, M, Darby, RR, et al. A human depression circuit derived from focal brain lesions. Biol Psychiatry. (2019) 86:749–58. doi: 10.1016/j.biopsych.2019.07.023
12. Siddiqi, SH, Schaper, FLWVJ, Horn, A, Hsu, J, Padmanabhan, JL, Brodtmann, A, et al. Brain stimulation and brain lesions converge on common causal circuits in neuropsychiatric disease. Nature human. Behaviour. (2021) 5:1707–16. doi: 10.1038/s41562-021-01161-1
13. Glasser, MF, Smith, SM, Marcus, DS, Andersson, JLR, Auerbach, EJ, Behrens, TEJ, et al. The human connectome Project's neuroimaging approach. Nat Neurosci. (2016) 19:1175–87. doi: 10.1038/nn.4361
14. Albazron, FM, Bruss, J, Jones, RM, Yock, TI, Pulsifer, MB, Cohen, AL, et al. Pediatric postoperative cerebellar cognitive affective syndrome follows outflow pathway lesions. Neurology. (2019) 93:e1561–71. doi: 10.1212/WNL.0000000000008326
15. Darby, RR, Horn, A, Cushman, F, and Fox, MD. Lesion network localization of criminal behavior. Proc Natl Acad Sci U S A. (2018) 115:601–6. doi: 10.1073/pnas.1706587115
16. Hwang, K, Bruss, J, Tranel, D, and Boes, AD. Network localization of executive function deficits in patients with focal thalamic lesions. J Cogn Neurosci. (2020) 32:2303–19. doi: 10.1162/jocn_a_01628
17. Joutsa, J, Shih, LC, and Fox, MD. Mapping holmes tremor circuit using the human brain connectome. Ann Neurol. (2019) 86:812–20. doi: 10.1002/ana.25618
18. Kyeong, S, and Kim, DH. Lesion-based structural and functional networks in patients with step length asymmetry after stroke. NeuroRehabilitation. (2021) 48:133–8. doi: 10.3233/NRE-201555
19. Joutsa, J, Horn, A, Hsu, J, and Fox, MD. Localizing parkinsonism based on focal brain lesions. Brain. (2018) 141:2445–56. doi: 10.1093/brain/awy161
20. Laganiere, S, Boes, AD, and Fox, MD. Network localization of hemichorea-hemiballismus. Neurology. (2016) 86:2187–95. doi: 10.1212/WNL.0000000000002741
21. Moher, D, Liberati, A, Tetzlaff, J, and Altman, DG. Preferred reporting items for systematic reviews and meta-analyses: the PRISMA statement. BMJ. (2009) 339:b2535. doi: 10.1136/bmj.b2535
22. Lo, CK-L, Mertz, D, and Loeb, M. Newcastle-Ottawa scale: comparing reviewers’ to authors’ assessments. BMC Med Res Methodol. (2014) 14:45. doi: 10.1186/1471-2288-14-45
23. Blondiaux, E, Heydrich, L, and Blanke, O. Common and distinct brain networks of autoscopic phenomena. Neuroimage Clin. (2021) 30:102612. doi: 10.1016/j.nicl.2021.102612
24. Bowren, M, Bruss, J, Manzel, K, Edwards, D, Liu, C, Corbetta, M, et al. Post-stroke outcomes predicted from multivariate lesion-behaviour and lesion network mapping. Brain. (2022) 145:1338–53. doi: 10.1093/brain/awac010
25. Cohen, AL, Ferguson, MA, and Fox, MD. Lesion network mapping predicts post-stroke behavioural deficits and improves localization. Brain. (2021) 144:E35. doi: 10.1093/brain/awab002
26. Corp, DT, Joutsa, J, Darby, RR, Delnooz, CCS, Van De Warrenburg, BPC, Cooke, D, et al. Network localization of cervical dystonia based on causal brain lesions. Brain. (2019) 142:1660–74. doi: 10.1093/brain/awz112
27. Cotovio, G, Faro Viana, F, Fox, MD, and Oliveira-Maia, AJ. Lesion network mapping of mania using different normative connectomes. Brain Struct Funct. (2022) 227:3121–7. doi: 10.1007/s00429-022-02508-8
28. Crockett, RA, Hsu, CL, Dao, E, Tam, R, Alkeridy, W, Eng, JJ, et al. Mind the gaps: functional networks disrupted by white matter hyperintensities are associated with greater falls risk. Neurobiol Aging. (2022) 109:166–75. doi: 10.1016/j.neurobiolaging.2021.09.023
29. Crockett, RA, Hsu, CL, Dao, E, Tam, R, Eng, JJ, Handy, TC, et al. Painting by lesions: White matter hyperintensities disrupt functional networks and global cognition. NeuroImage. (2021) 236:118089. doi: 10.1016/j.neuroimage.2021.118089
30. Germann, J, Elias, GJB, Neudorfer, C, Boutet, A, Chow, CT, Wong, EHY, et al. Potential optimization of focused ultrasound capsulotomy for obsessive compulsive disorder. Brain. (2021) 144:3529–40. doi: 10.1093/brain/awab232
31. Higashiyama, Y, Hamada, T, Saito, A, Morihara, K, Okamoto, M, Kimura, K, et al. Neural mechanisms of foreign accent syndrome: lesion and network analysis. Neuroimage Clin. (2021) 31:102760. doi: 10.1016/j.nicl.2021.102760
32. Joutsa, J, Shih, LC, Horn, A, Reich, MM, Wu, O, Rost, NS, et al. Identifying therapeutic targets from spontaneous beneficial brain lesions. Ann Neurol. (2018) 84:153–7. doi: 10.1002/ana.25285
33. Klingbeil, J, Wawrzyniak, M, Stockert, A, Karnath, HO, and Saur, D. Hippocampal diaschisis contributes to anosognosia for hemiplegia: evidence from lesion network-symptom-mapping. NeuroImage. (2020) 208:116485. doi: 10.1016/j.neuroimage.2019.116485
34. Mansouri, AM, Germann, J, Boutet, A, Elias, GJB, Mithani, K, Chow, CT, et al. Identification of neural networks preferentially engaged by epileptogenic mass lesions through lesion network mapping analysis. Sci Rep. (2020) 10:10989. doi: 10.1038/s41598-020-67626-x
35. Philippi, CL, Bruss, J, Boes, AD, Albazron, FM, Deifelt Streese, C, Ciaramelli, E, et al. Lesion network mapping demonstrates that mind-wandering is associated with the default mode network. J Neurosci Res. (2021) 99:361–73. doi: 10.1002/jnr.24648
36. Pini, L, Salvalaggio, A, De Filippo De Grazia, M, Zorzi, M, Thiebaut de Schotten, M, and Corbetta, M. A novel stroke lesion network mapping approach: improved accuracy yet still low deficit prediction. Brain Commun. (2021) 3:fcab259. doi: 10.1093/braincomms/fcab259
37. Snider, SB, Hsu, J, Darby, RR, Cooke, D, Fischer, D, Cohen, AL, et al. Cortical lesions causing loss of consciousness are anticorrelated with the dorsal brainstem. Hum Brain Mapp. (2020) 41:1520–31. doi: 10.1002/hbm.24892
38. Salvalaggio, A, De Filippo De Grazia, M, Zorzi, M, Thiebaut de Schotten, M, and Corbetta, M. Post-stroke deficit prediction from lesion and indirect structural and functional disconnection. Brain. (2020) 143:2173–88. doi: 10.1093/brain/awaa156
39. Joutsa, J, Moussawi, K, Siddiqi, SH, Abdolahi, A, Drew, W, Cohen, AL, et al. Brain lesions disrupting addiction map to a common human brain circuit. Nat Med. (2022) 28:1249–55. doi: 10.1038/s41591-022-01834-y
40. Ferguson, MA, Lim, C, Cooke, D, Darby, RR, Wu, O, Rost, NS, et al. A human memory circuit derived from brain lesions causing amnesia. Nat Commun. (2019) 10:3497. doi: 10.1038/s41467-019-11353-z
41. Fischer, DB, Boes, AD, Demertzi, A, Evrard, HC, Laureys, S, Edlow, BL, et al. A human brain network derived from coma-causing brainstem lesions. Neurology. (2016) 87:2427–34. doi: 10.1212/WNL.0000000000003404
42. Herbet, G, Lemaitre, AL, Moritz-Gasser, S, Cochereau, J, and Duffau, H. The antero-dorsal precuneal cortex supports specific aspects of bodily awareness. Brain. (2019) 142:2207–14. doi: 10.1093/brain/awz179
43. Darby, RR, Joutsa, J, Burke, MJ, and Fox, MD. Lesion network localization of free will. Proc Natl Acad Sci. (2018) 115:10792–7. doi: 10.1073/pnas.1814117115
44. Ferguson, MA, Schaper, F, Cohen, A, Siddiqi, S, Merrill, SM, Nielsen, JA, et al. A neural circuit for spirituality and religiosity derived from patients with brain lesions. Biol Psychiatry. (2022) 91:380–8. doi: 10.1016/j.biopsych.2021.06.016
45. Jimenez-Marin, A, De Bruyn, N, Gooijers, J, Llera, A, Meyer, S, Alaerts, K, et al. Multimodal and multidomain lesion network mapping enhances prediction of sensorimotor behavior in stroke patients. Sci Rep. (2022) 12:22400. doi: 10.1038/s41598-022-26945-x
46. Kletenik, I, Ferguson, MA, Bateman, JR, Cohen, AL, Lin, C, Tetreault, A, et al. Network localization of unconscious visual perception in Blindsight. Ann Neurol. (2022) 91:217–24. doi: 10.1002/ana.26292
47. Alves, PN, Silva, DP, Fonseca, AC, and Martins, IP. Mapping delusions of space onto a structural disconnectome that decouples familiarity and place networks. Cortex. (2022) 146:250–60. doi: 10.1016/j.cortex.2021.11.008
48. Conrad, J, Boegle, R, Ruehl, RM, and Dieterich, M. Evaluating the rare cases of cortical vertigo using disconnectome mapping. Brain Struct Funct. (2022) 227:3063–73. doi: 10.1007/s00429-022-02530-w
49. Dulyan, L, Talozzi, L, Pacella, V, Corbetta, M, Forkel, SJ, and Thiebaut de Schotten, M. Longitudinal prediction of motor dysfunction after stroke: a disconnectome study. Brain Struct Funct. (2022) 227:3085–98. doi: 10.1007/s00429-022-02589-5
50. Jiang, J, Ferguson, MA, Grafman, J, Cohen, AL, and Fox, MD. A lesion-derived brain network for emotion regulation. Biol Psychiatry. (2023) S0006-3223:00081-1. doi: 10.1016/j.biopsych.2023.02.007
51. Kolskår, KK, Ulrichsen, KM, Richard, G, Dørum, ES, de Schotten, MT, Rokicki, J, et al. Structural disconnectome mapping of cognitive function in poststroke patients. Brain Behav. (2022) 12:e2707. doi: 10.1002/brb3.2707
52. Li, Y, Qi, L, Schaper, FLWVJ, Wu, D, Friedrich, M, Du, J, et al. A vertigo network derived from human brain lesions and brain stimulation. Brain Communications. (2023) 5:fcad071. doi: 10.1093/braincomms/fcad071
53. Rosenzopf, H, Wiesen, D, Basilakos, A, Yourganov, G, Bonilha, L, Rorden, C, et al. Mapping the human praxis network: an investigation of white matter disconnection in limb apraxia of gesture production. Brain Commun. (2022) 4:fcac004. doi: 10.1093/braincomms/fcac004
54. Siddiqi, SH, Kletenik, I, Anderson, MC, Cavallari, M, Chitnis, T, Glanz, BI, et al. Lesion network localization of depression in multiple sclerosis. J Neurol. (2023) 1:36–44. doi: 10.1038/s44220-022-00002-y
55. Sotelo, MR, Kalinosky, BT, Goodfriend, K, Hyngstrom, AS, and Schmit, BD. Indirect structural connectivity identifies changes in brain networks after stroke. Brain Connect. (2020) 10:399–410. doi: 10.1089/brain.2019.0725
56. Ulrichsen, KM, Kolskår, KK, Richard, G, Alnæs, D, Dørum, ES, Sanders, A-M, et al. Structural brain disconnectivity mapping of post-stroke fatigue. Neuroimage Clin. (2021) 30:102635. doi: 10.1016/j.nicl.2021.102635
57. Weaver, NA, Lim, JS, Schilderinck, J, Biessels, GJ, Kang, Y, Kim, BJ, et al. Strategic infarct locations for Poststroke depressive symptoms: a lesion-and disconnection-symptom mapping study. Biol Psychiatry Cogn Neurosci Neuroimaging. (2023) 8:387–96. doi: 10.1016/j.bpsc.2021.09.002
58. Souter, NE, Wang, X, Thompson, H, Krieger-Redwood, K, Halai, AD, Lambon Ralph, MA, et al. Mapping lesion, structural disconnection, and functional disconnection to symptoms in semantic aphasia. Brain Struct Funct. (2022) 227:3043–61. doi: 10.1007/s00429-022-02526-6
59. Corbetta, M, Siegel, JS, and Shulman, GL. On the low dimensionality of behavioral deficits and alterations of brain network connectivity after focal injury. Cortex. (2018) 107:229–37. doi: 10.1016/j.cortex.2017.12.017
60. Carrera, E, and Tononi, G. Diaschisis: past, present, future. Brain. (2014) 137:2408–22. doi: 10.1093/brain/awu101
61. Seyedmirzaei, H, Shafie, M, Kargar, A, Golbahari, A, Bijarchian, M, Ahmadi, S, et al. White matter tracts associated with alexithymia and emotion regulation: a diffusion MRI study. J Affect Disord. (2022) 314:271–80. doi: 10.1016/j.jad.2022.07.039
62. Seyedmirzaei, H, Nabizadeh, F, Aarabi, MH, and Pini, L. Neurite orientation dispersion and density imaging in multiple sclerosis: a systematic review. J Magn Reson Imaging. (2023). doi: 10.1002/jmri.28727
63. Baldassarre, A, Ramsey, L, Hacker, CL, Callejas, A, Astafiev, SV, Metcalf, NV, et al. Large-scale changes in network interactions as a physiological signature of spatial neglect. Brain. (2014) 137:3267–83. doi: 10.1093/brain/awu297
64. Corbetta, M, Ramsey, L, Callejas, A, Baldassarre, A, Hacker Carl, D, Siegel Joshua, S, et al. Common behavioral clusters and subcortical anatomy in stroke. Neuron. (2015) 85:927–41. doi: 10.1016/j.neuron.2015.02.027
65. Dougherty, DD . Deep brain stimulation: clinical applications. Psychiatr Clin North Am. (2018) 41:385–94. doi: 10.1016/j.psc.2018.04.004
66. Kapur, N . Paradoxical functional facilitation in brain-behaviour research: a critical review. Brain. (1996) 119:1775–90. doi: 10.1093/brain/119.5.1775
67. Hanlon, CA, Dowdle, LT, and Henderson, JS. Modulating neural circuits with transcranial magnetic stimulation: implications for addiction treatment development. Pharmacol Rev. (2018) 70:661–83. doi: 10.1124/pr.116.013649
68. Fox, MD . Mapping symptoms to brain networks with the human connectome. N Engl J Med. (2018) 379:2237–45. doi: 10.1056/NEJMra1706158
69. Joutsa, J, Corp, DT, and Fox, MD. Lesion network mapping for symptom localization: recent developments and future directions. Curr Opin Neurol. (2022) 35:453–9. doi: 10.1097/WCO.0000000000001085
70. Bonkhoff, AK, Schirmer, MD, Bretzner, M, Hong, S, Regenhardt, RW, Brudfors, M, et al. Outcome after acute ischemic stroke is linked to sex-specific lesion patterns. Nat Commun. (2021) 12:3289. doi: 10.1038/s41467-021-23492-3
Keywords: lesion network mapping, connectivity, lesions, stroke, network, localization
Citation: Nabizadeh F and Aarabi MH (2023) Functional and structural lesion network mapping in neurological and psychiatric disorders: a systematic review. Front. Neurol. 14:1100067. doi: 10.3389/fneur.2023.1100067
Edited by:
Alessandra Griffa, Université de Genève, SwitzerlandReviewed by:
Joshua C. Cheng, Beth Israel Deaconess Medical Center and Harvard Medical School, United StatesAlexandre Boutet, University of Toronto, Canada
Copyright © 2023 Nabizadeh and Aarabi. This is an open-access article distributed under the terms of the Creative Commons Attribution License (CC BY). The use, distribution or reproduction in other forums is permitted, provided the original author(s) and the copyright owner(s) are credited and that the original publication in this journal is cited, in accordance with accepted academic practice. No use, distribution or reproduction is permitted which does not comply with these terms.
*Correspondence: Mohammad Hadi Aarabi, bW9oYW1tYWRoYWRpYXJhYmlAZ21haWwuY29t