- 1Department of Veterans Affairs Multiple Sclerosis Center of Excellence (VA MSCoE), Baltimore VA Medical Center, Baltimore, MD, United States
- 2Department of Neurology, University of Maryland Medical Center, Baltimore, MD, United States
- 3Health Sciences and Human Services Library, University of Maryland, Baltimore, MD, United States
Background: Neuromyelitis optica spectrum disorder (NMOSD) is a rare chronic neuroinflammatory autoimmune condition. Since the onset of the COVID-19 pandemic, there have been reports of NMOSD clinical manifestations following both SARS-CoV-2 infections and COVID-19 vaccinations.
Objective: This study aims to systematically review the published literature of NMOSD clinical manifestations associated with SARS-CoV-2 infections and COVID-19 vaccinations.
Methods: A Boolean search of the medical literature was conducted between December 1, 2019 to September 1, 2022, utilizing Medline, Cochrane Library, Embase, Trip Database, Clinicaltrials.gov, Scopus, and Web of Science databases. Articles were collated and managed on Covidence® software. The authors independently appraised the articles for meeting study criteria and followed PRISMA guidelines. The literature search included all case reports and case series that met study criteria and involved NMOSD following either the SARS-CoV-2 infection or the COVID-19 vaccination.
Results: A total of 702 articles were imported for screening. After removing 352 duplicates and 313 articles based on exclusion criteria, 34 articles were analyzed. A total of 41 cases were selected, including 15 patients that developed new onset NMOSD following a SARS-CoV-2 infection, 21 patients that developed de novo NMOSD following COVID-19 vaccination, 3 patients with known NMOSD that experienced a relapse following vaccination, and 2 patients with presumed Multiple Sclerosis (MS) that was unmasked as NMOSD post-vaccination. There was a female preponderance of 76% among all NMOSD cases. The median time interval between the initial SARS-CoV-2 infection symptoms and NMOSD symptom onset was 14 days (range 3–120 days) and the median interval between COVID-19 vaccination and onset of NMO symptoms was 10 days (range 1 to 97 days). Transverse myelitis was the most common neurological manifestation in all patient groups (27/41). Management encompassed acute treatments such as high dose intravenous methylprednisolone, plasmapheresis, and intravenous immunoglobulin (IVIG) and maintenance immunotherapies. The majority of patients experienced a favorable outcome with complete or partial recovery, but 3 patients died.
Conclusion: This systematic review suggests that there is an association between NMOSD and SARS-CoV-2 infections and COVID-19 vaccinations. This association requires further study using quantitative epidemiological assessments in a large population to better quantify the risk.
Introduction
Novel coronavirus disease (COVID-19), a respiratory disease caused by severe acute respiratory syndrome coronavirus 2 (SARS-CoV-2), was first detected in Wuhan, China in December 2019, and by March 2021 the World Health Organization (WHO) declared a worldwide pandemic (1). As of November 2, 2022, globally there have been over 628 million confirmed cases of COVID-19, including over 6.57 million deaths, and over 12.85 billion doses of the vaccine have been administered (2). Despite the rapid development and distribution of vaccinations, COVID-19 remains a prevalent and serious public health condition today.
SARS-CoV-2 has the ability to dysregulate the host immune system, producing various autoantibodies (3–5). This can induce a cascade of immune-mediated central nervous system (CNS) damage from either direct inoculation of the CNS or a systemic autoimmune response toward the virus (3–6). It has been shown that SARS-CoV2 can traverse the blood brain barrier and provoke CNS demyelination (3). Given this background, it is not surprising that a variety of case reports have linked the SARS-CoV-2 infection with an array of CNS autoimmune demyelinating disorders such as transverse myelitis (TM), acute demyelinating encephalomyelitis (ADEM), multiple sclerosis (MS), and neuromyelitis optica spectrum disorder (NMOSD) (6–8).
NMOSD is a chronic, relapsing, autoantibody-mediated astrocytopathy channelopathy that presents as severe CNS demyelination attacks commonly involving TM, optic neuritis (ON), area postrema syndrome (APS), and acute brainstem syndrome (BS) (9). The underlying pathogenic mechanism that leads to NMOSD is unclear, but mounting evidence suggests that there is an intricate interplay between environmental factors, such as vaccines and viral infection, and genetic susceptibility that leads to CNS inflammation (10–12).
As COVID-19 is likely to remain a prevalent infectious disease, it is essential that we elucidate the association between this SARS-CoV-2 infections and neuroinflammatory conditions such as NMOSD. Through this systematic review, we will assess the association between SARS-CoV-2 infections and the para and post-infectious manifestations of NMOSD. We will also investigate the potential association between COVID-19 vaccination and the development of de novo or relapsing NMOSD.
Methods
Design
Literature was retrieved from the following databases on September 13, 2022: Medline (Ovid), Cochrane Library (WileyOnline), Embase (Elsevier), Trip Database Pro, Clinicaltrials.gov, and Scopus (Elsevier). This systematic review was carried out in accordance with Preferred Reporting Items for Systematic Reviews and Meta-analysis (PRISMA) guidelines. We aimed to identify relevant articles reporting on NMOSD manifestations following a SARS-CoV-2 infection or a de novo or relapsing forms of NMOSD presenting in association with any type of approved COVID-19 vaccine.
Search strategy
The search strategy combined keywords and controlled vocabulary related to NMOSD and COVID-19 and was tailored to the specifications of each database (see Supplementary appendix 1). A detailed search strategy can be found in Supplementary material. A manual search of bibliographies of relevant studies was also conducted. All citations for this review were required to be indexed in the peer-reviewed literature. Results were carefully verified to avoid duplicates or overlapping publications.
Inclusion criteria
We identified and triaged manuscripts and included all peer-reviewed, full-text, English language manuscripts that reported cases of NMOSD that met the 2015 International Panel for NMOSD Diagnosis (IPND) criteria in association with SARS-CoV-2 infection or a COVID-19 vaccination (13).
Exclusion criteria
The review was restricted to studies published in English. Poster and symposium abstracts, non-peer reviewed publications, and clinical trials were excluded from this report. We also excluded review papers, editorial, hypothesis reports, and commentaries, unless there was a report of a case of NMOSD following a SARS-CoV-2 infection or COVID-19 vaccine. Studies were also excluded if they contained insufficient clinical data, if the data was repeated from an article that had already been included, or if they addressed peripheral nervous system (PNS) demyelinating diseases or CNS demyelinating disorders other than NMOSD such as myelin oligodendrocyte glycoprotein antibody disease (MOGAD), TM, ON, MS, and acute disseminated encephalomyelitis (ADEM). Cases involving other types of coronaviruses (e.g., SARS-CoV/MERS-CoV) infections were also excluded.
Data extraction
Titles and abstracts of all identified studies were independently screened for relevance by two reviewers, MW and TH, to ensure they met criteria for inclusion. Following a full-text screening of eligible articles, articles meeting criteria were retrieved, summarized, and managed on Covidence® software. Discordant abstract or article decisions and screening queries were resolved by consensus. The same reviewers then extracted data on the following parameters: article title, authors, publication year, country, age/gender of the patients, aquaporin-4 (AQP4) antibody status, SARS-CoV-2 infection presentation, NMOSD clinical presentation, COVID-19 vaccine related information, interval prior to onset of neurological symptoms, MRI findings, cerebrospinal fluid (CSF) analysis, SARS-COV-2 laboratory findings, treatment, and clinical outcome.
Statistical analysis
Quantitative data were described using range (minimum and maximum), mean and median, while qualitative data were described in percentages and numbers. Covidence software was used for evaluating and adjudicating articles for the systematic review and Microsoft Excel was used for statistical assessments.
Results
As seen in the PRISMA flowchart (Figure 1), our systematic search identified 702 potentially relevant articles through various databases. A total of 354 duplicate articles were discarded. The remaining 348 articles were screened by title and abstract, and 249 non peer reviewed or nonrelevant articles were removed. Thereafter, a total of 34 studies were deemed eligible by the authors after applying the inclusion/exclusion criteria to the full text documents, of which there were 24 single-case reports, 9 case-series, and 1 prospective cohort study. These 34 reports described 41 unique patients which were divided into three categories: NMOSD onset following a SARS-CoV-2 infection, NMOSD onset following COVID-19 vaccination, and relapses consistent with NMOSD following COVID-19 vaccination. The clinical characteristics for each of these categories are presented in Tables 1–4 which summarizing the demographic and clinical characteristics of patients with SARS-CoV-2 post-infection and COVID-19 post-vaccination NMOSD manifestations.
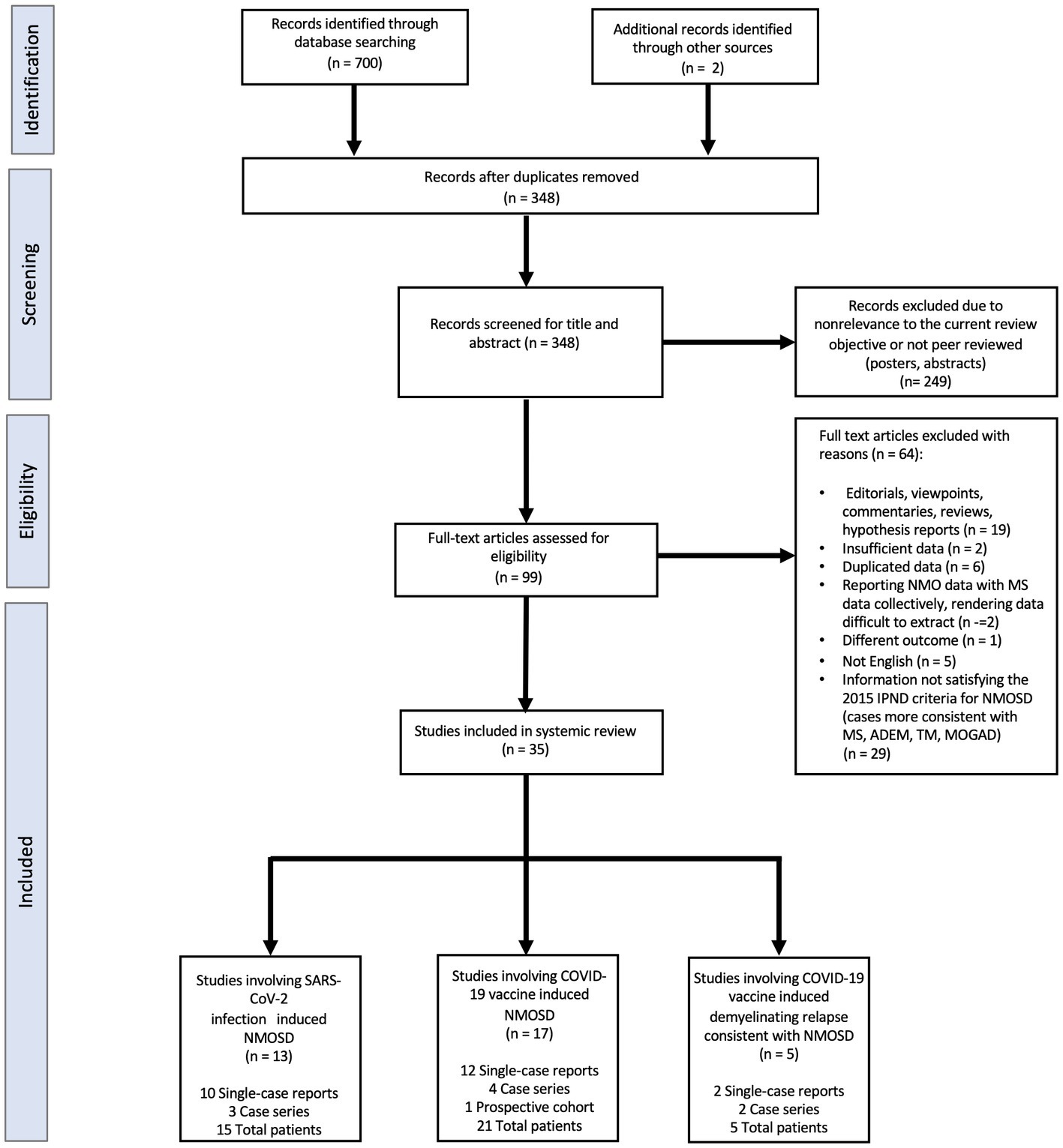
Figure 1. Flow chart of literature inclusion in accordance with PRISMA guidelines. ADEM, Acute Demyelinating Encephalomyelitis; IPND, International Panel for NMO Diagnosis; MOGAD, Myelin oligodendrocyte glycoprotein antibody-associated disease; MS, Multiple Sclerosis; NMOSD, Neuromyelitis Optica Spectrum Disorder; SARS-CoV-2, Severe Acute Respiratory Syndrome Coronavirus-2; TM, Transverse Myelitis.
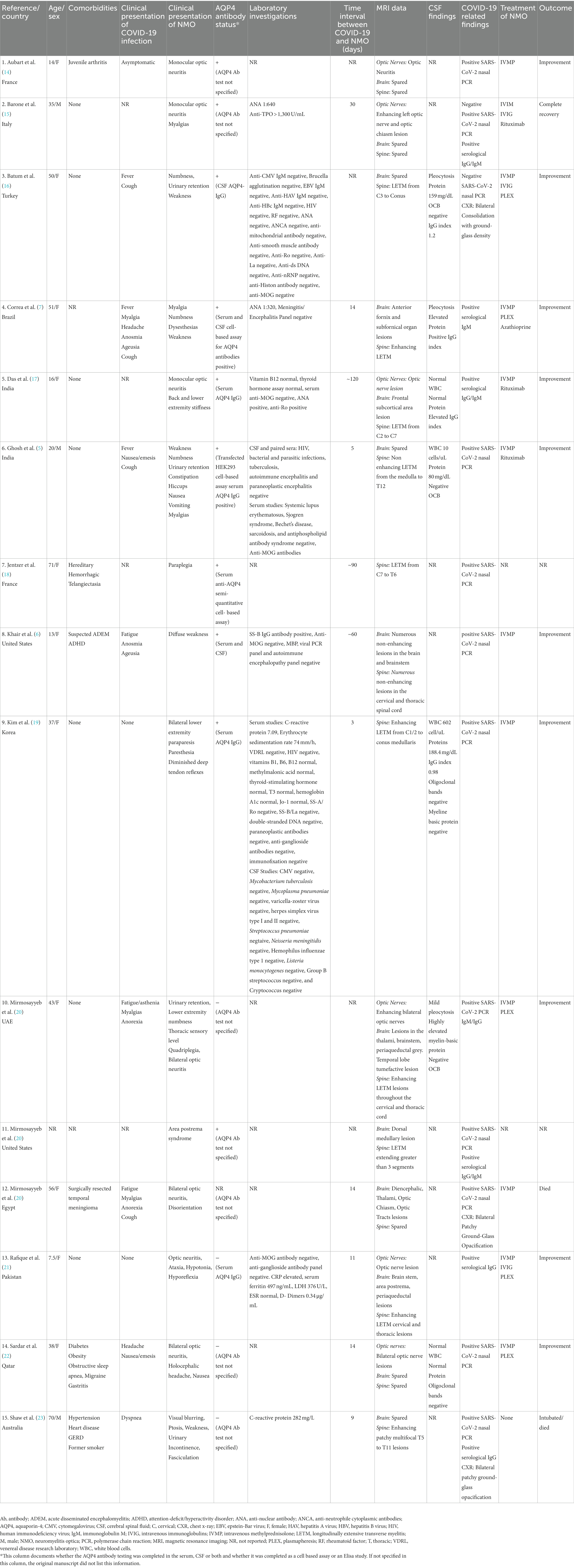
Table 1. Characteristics of cases presenting with Neuromyelitis Optica in relation to a SARS-CoV-2 infection.
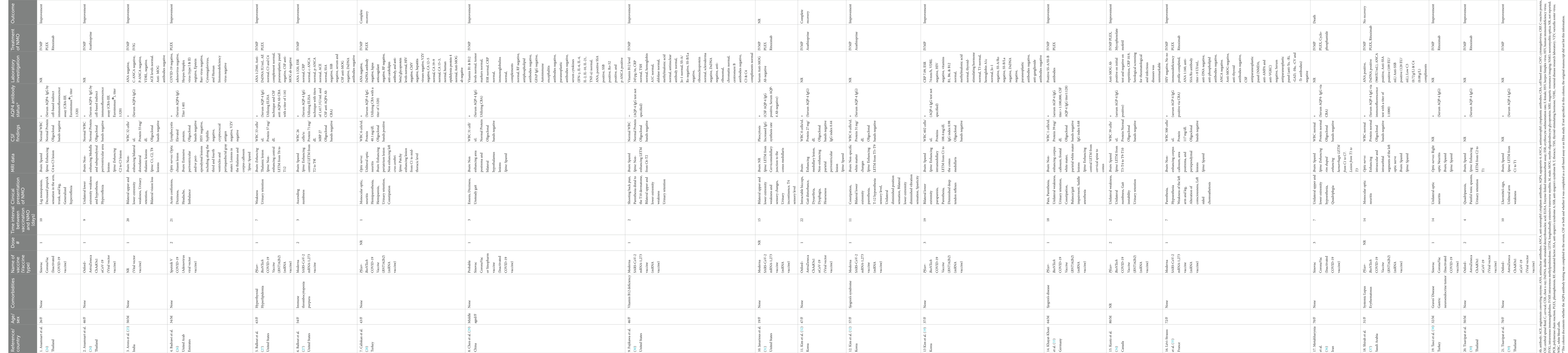
Table 2. Characteristics of cases presenting with de novo neuromyelitis optica in relation to COVID-19 vaccination.
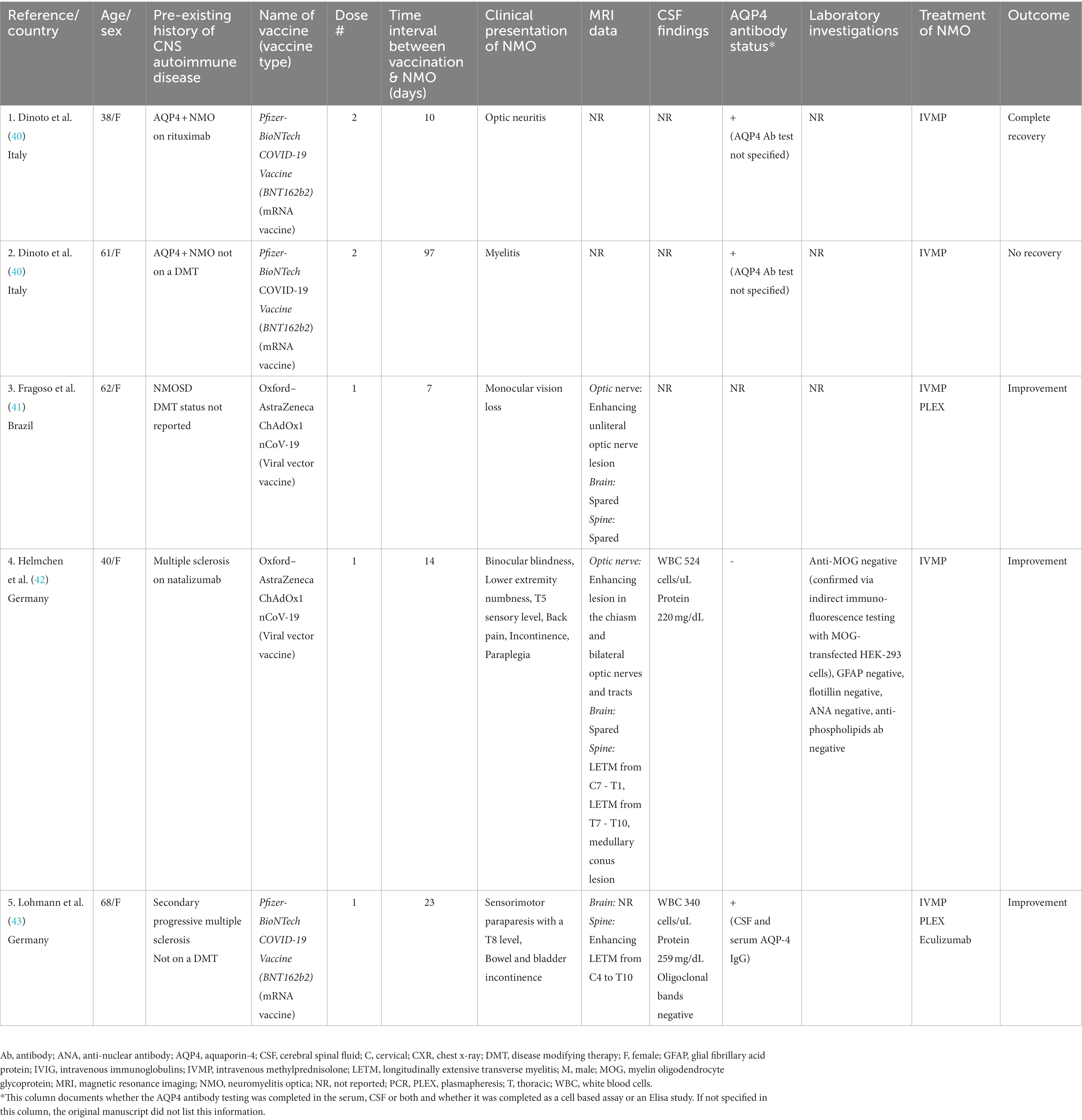
Table 3. Characteristics of cases presenting with central nervous system relapses consistent with neuromyelitis optica in relation to COVID-19 vaccination.
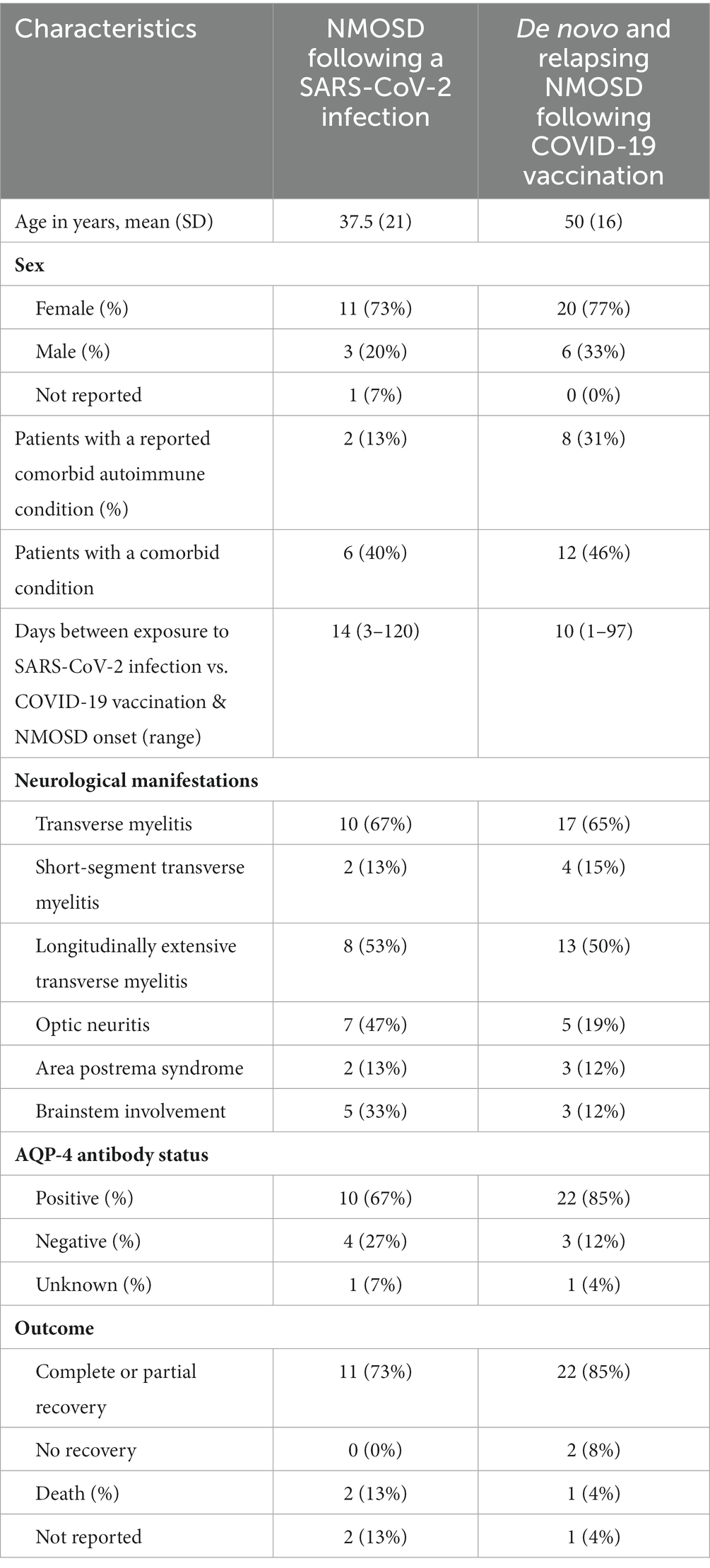
Table 4. Comparison of demographic and disease characteristics of patients with SARS-CoV-2 post-infection and COVID-19 post-vaccination NMOSD.
New onset NMOSD following SARS-CoV-2 infection
Of the 15 patients that developed NMOSD following SARS-CoV-2 infection, 11 were female (73%), 3 were male (20%), and one was not identified (7%). The reported cases came from 12 countries; 2 cases each from France, India, and the United States of America (USA), and 1 case each from Italy, Korea, Pakistan, Qatar, Turkey, the United Arab Emirates, Australia, Brazil, and Egypt. Given a total of 626,337,158 world-wide COVID-19 cases as of October 31, 2022 (2), the global incidence based on reported cases of NMOSD following a SARS-CoV-2 infection is 0.02 per million.
The median age of the patients was 37.5 years (range 7.5–71 years). The latency period from the onset of COVID-19 symptoms to the first neurological manifestations followed a dual distribution: (i) Short latency: 3 to 14 days in 8/15 patients (53%) and (ii) Long latency (60 to 120 days) in 3/15 patients (20%). The median time interval between the initial SARS-CoV-2 infection symptoms and NMOSD symptom onset was 14 days (range 3–120 days).
Interestingly, 2/15 (13%) of patients had a history of a previously diagnosed immune-mediated condition; one patient had juvenile arthritis, and the other patient had a past episode of suspected ADEM. Comorbidities were present in 6/15 patients (40%) and are summarized with other clinical characteristics in Table 1.
In terms of the clinical presentation, TM was the most common neurological phenotype occurring in 10/15 (67%) patients. Two of the 10 had short-segment TM (STM) spanning over less than 3 vertebral segments and 8 were longitudinally extensive TM (LETM) spanning 3 or more vertebral segments. The second most common presentation was ON, found in 7 (47%) patients. APS, defined as intractable nausea, vomiting, or hiccups persisting for at least 48 h, was found in 2 (13%) patients. Brainstem involvement was found in 5 (33%). Ten patients (67%) tested positive for AQP4 antibody, while 4 (27%) were AQP4 antibody negative (one case not reported). CSF analysis in this group demonstrated pleocytosis in 5/15 (33%) patients while 2/15 (13%) had normal white blood cell (WBC) counts. CSF findings were not reported for 8/15 (53%) patients. High protein levels were reported in 2/15 (13%) patients.
Of the 13 cases that reported on acute treatment, all but one patient (92%) was initially treated with intravenous methylprednisolone. In additional to methylprednisolone, 5/13 (39%) were treated with plasmapheresis and 3/13 (23%) were treated with intravenous immunoglobulin (IVIG). Maintenance immunotherapy was provided to only four patients, including rituximab (n = 3) and azathioprine (n = 1). The treatment outcomes were reported for 13 of the 15 patients. Of these patients, 11/13 (84%) experienced complete or partial recovery following treatment, while 2/13 (15%) patients died. One death was caused by multiorgan failure and sepsis secondary to the SARS-CoV-2 infection. The second patient died from respiratory insufficiency, lymphopenia, and fever following cyclophosphamide treatment.
New onset and relapsing NMOSD following COVID-19 vaccination
Tables 2, 3 describe the clinical presentation, laboratory and imaging findings, and treatment outcomes of both de novo and relapsing NMOSD cases following the COVID-19 vaccine.
After receiving a COVID-19 vaccination, 26 patients developed a new demyelinating event related to NMOSD. A total of 21 of the 26 (81%) cases experienced an initial relapse of NMOSD following the COVID-19 vaccination, while 5 of the 26 (19%) cases had a recurrent exacerbation attributed to NMOSD following vaccination. Of the 5 relapsing cases, 3 of the patients had a known diagnosis of NMOSD, while two patients had been initially diagnosed with MS, which was unmasked as NMOSD post vaccination. Of note, one of the patients with known NMOSD had been stable and relapse free for 8 years, prior to their vaccine inducing a new relapse.
Based on data from the WHO, a total of 12,830,378,906 vaccine doses have been administered globally as of October 31, 2022 (2), the global incidence of an NMOSD demyelinating events among reported cases in the literature following vaccination is 0.002 per million.
Of the 26 cases developing NMOSD manifestations following a COVID-19 vaccination, 9 cases (35%) occurred after receiving the Pfizer-BioNTech BNT162b2 mRNA vaccine, 6 (23%) following the Oxford–AstraZeneca ChAdOx1 nCoV-19 viral vector vaccine, 5 (19%) following the Moderna mRNA-1273 vaccine, 4 (15%) after the Sinovac or Sinopharm inactivated COVID-19 vaccine, and 1 (4%) following the Sputnik V adenovirus viral vector vaccine. The specific vaccine involved in one case was unspecified, but it was a viral vector vaccine. In sum, 54% (14/26) of cases involved an mRNA vaccine, 31% (8/26) of cases involved a viral vector vaccine, and 15% (4/26) of cases involved an inactivated COVID-19 vaccine.
In terms of demographics, there was a female preponderance with a 3.3:1 ratio of female to male cases. The median age was 50 years with an age range of 19 to 80. The reported cases came from 13 countries with 4 cases each from Thailand and the USA, 3 cases each from Germany and Korea, 2 cases each from Italy and Turkey, and 1 case each from Brazil, Canada, China, France, India, Iran, Saudi Arabia, and the United Arab Emirates.
The median duration between vaccination and onset of NMOSD related clinical symptoms was 10 days (range 1–97 days). Figure 2, displays time intervals between vaccination and neurological symptom onset for each COVID-19 vaccine. Breaking down symptom onset with dose of the vaccine, 15/26 (58%) patients, experienced the onset of neurological symptoms following the first dose of the vaccine. A total of 6/26 (23%) patients had the onset of neurological symptoms following the second dose of the vaccine, and in 2/26 (8%) patients, the onset of neurological symptoms followed the third dose of the vaccine. One case did not specify, which dose induced the neurological symptoms.
Interestingly, 8/26 (31%) patients had a history of a previously diagnosed immune-mediated condition. In addition, 4/26 (15%) patients reported a family history of an immune-mediated condition including MS, AQP4-IgG positive NMOSD, myasthenia gravis, and systemic lupus erythematous.
Turning to the clinical presentation, TM was the most common phenotype, occurring in 17 (65%) patients. Four of those were STM spanning over less than 3 vertebral segments and 13 were LETM spanning 3 or more vertebral segments. The second most common presentation was ON, found in 5 (19%) patients. APS was found in 3 (12%) patients and brainstem involvement was found in 3 (12%) patients.
Of the 25 patients with a reported AQP4 antibody status, 22/25 (88%) patients tested positive for AQP4 antibody, while 3 (12%) were AQP4 antibody negative. Of the 20 patients with reported CSF results 11/20 (55%) had pleocytosis, 9/20 (45%) had elevated CSF protein levels. Only one patient’s CSF findings were positive for oligoclonal bands (OCB) out of the 16 cases that explicitly documented OCB status.
In terms of therapy, all but one patient (96%) was initially treated with intravenous methylprednisolone. Subsequently, 12/26 (46%) patients were treated with pulses of plasmapheresis, and 1/26 (4%) patients was treated with IVIG. Maintenance immunotherapy was documented in 12/26 (46%) patients, including rituximab (n = 6), azathioprine (n = 3), cyclophosphamide (n = 1), eculizumab (n = 1), and mycophenolate mofetil (n = 1). The treatment outcomes were reported for 25/26 patients. Of these patients, 22/25 (88%) experienced complete or partial recovery following treatment, 2/25 (8%) patients did not improve with treatment, and 1/25 (4%) patients died. The cause of death was not discussed in the case series.
Comparison of demographic and clinical characteristics of patients with SARS-CoV-2 post-infection and COVID-19 post-vaccination NMOSD
Table 4 compares the demographic and clinical characteristics of NMOSD following SAR-CoV-2 infection and COVID-19 vaccination. The COVID-19 vaccine exposure group and the SARS-CoV-2 viral infection group had similar sex ratios with a female preponderance, but the vaccine group’s age was on average over a decade older than the SARS-CoV-2 infected group. Both groups had a similar percentage of comorbidities, but the COVID-19 vaccine group (31% vs. 13%) was more likely to present with a comorbid autoimmune condition. Both groups had a similar rate of transverse myelitis, but the SARS-CoV-2 infected group were more likely to present with optic neuritis and brain stem involvement. The COVID-19 vaccinated group was also more likely to present with positive AQP4 antibodies than the SARS-CoV-2 infected group (85% vs. 65%). Both groups demonstrated a similar mortality rate.
Discussion
As the COVID-19 pandemic has continued to persist, a mounting number of neurological manifestations and complications related to this disease have been described. Para and post infectious and post vaccination autoimmune CNS demyelination is a rare, but well documented phenomena. A small but accumulating base of literature suggests an association between the SARS-CoV-2 infection, the COVID-19 vaccine, and NMOSD. This systemic review contributes to this growing literature, including 41 worldwide cases of NMOSD temporally associated with the SARS-CoV-2 infection or COVID-19 vaccination. The analysis revealed that the NMOSD cases met standardized criteria, neurological symptoms developed within 2 weeks in most cases, the majority responded to standard immune therapies and overall neurological morbidity was moderate with 7% mortality.
The theory that a viral infection can trigger NMOSD pathogenesis is supported by several case series and case reports demonstrating an association between NMOSD and various viral infections including epstein barr virus, influenza virus, human immunodeficiency viruses (HIV), and varicella zoster virus (9, 44–47). SARS-CoV-2 infection has joined these other viral agents as a potential risk factor for PNS and CNS demyelinating disease (48, 49). In fact, TM, acute necrotizing encephalopathy, acute inflammatory demyelinating polyneuropathy (AIDP), and ADEM events have been associated with SARS-CoV-2 para and post infections, demonstrating that this emergent viral disease is associated with other CNS demyelinating disorders (50–53). Additionally, case reports have demonstrated an association between COVID-19 vaccinations and the onset of ADEM, TM, and MS following the COVID-19 vaccination (33, 54, 55).
The pathological mechanism explaining how the COVID-19 vaccine or the SARS-COV-2 infection induce NMOSD is not fully understood, but it is hypothesized that there is an interplay between viral and vaccine-related features and individual susceptibility factors (56). SARS-CoV-2 is thought to infect its host via the angiotensin-converting enzyme-2 (ACE-2) receptors on the cell surface of type II alveolar epithelial cells in the lung (57, 58). ACE-2 receptors are also expressed on the glial cells and the neurons (59). Therefore, in addition to infecting the respiratory system, SARS-CoV-2 can impact the central and peripheral nervous system. Once the host is exposed to either the COVID-19 vaccine or SARS-CoV-2 infection, NMOSD development may be mediated by either neurotropism or via aberrant immune mediated injury (5). Once SARS-CoV-2 has accessed the nervous system, several proposed pathological mechanisms have been suggested including bystander activation, spreading of the epitope, molecular/immunological mimicry involving cross-reactive autoantibodies targeting SARS-CoV-2 antigens, and amplified blood–brain barrier (BBB) permeability allowing antibody (i.e., AQP-4 peptides) entry into the CNS (5, 10). Evidence indicates that SARS-CoV-2 crosses the blood brain barrier (BBB) along with other cytokines including IL-1β, IL-2, IL-4, IL-6, IL-8, IL-10, TNF-α, and IFN-γ. This impacts macrophages, microglia, and astrocytes, which mediate a cytokine storm leading to the death of neurons and oligodendrocytes. This produces a cytokine storm and a proinflammatory state. Of these cytokines, IL-6 has particular significance as it has been implicated in playing a critical role in regulating the immune response in MS by promoting pathogenic T helper (Th) 17 cells generation (60). Disruption of Th 17 and regulatory T cell responses caused by SARs-CoV-2 exposure can induce inflammation and mitochondrial dysfunction that amplifies the inflammatory process, resulting in immune-metabolic constraints on neural energy metabolism (61). Additional mechanisms include activation of toll-like receptors (TLRs), antibody production against myelin via molecular mimicry, and the affinity for angiotensin-converting enzyme 2 (ACE2) receptors, which can induce myelin destruction (62). Furthermore, neuro-invasion by SAR-CoV-2 or its antigens may cause leakage of CNS antigens such as AQP-4 peptides into the systemic circulation, triggering the bystander immune cascade (5).
Several case reports have indicated that cytotoxic lesion of the corpus callosum (CLOCCs) are also associated with COVID-19 vaccinations (63–65). CLOCCs is caused by an influx of water into the cells due to cytokine induced glutamate release from astrocytes (63). The proposed underlying pathogenic mechanism between COVID-19 vaccine triggered NMOSD and CLOCCS is similar. For example, the CSF of CLOCCS patients is notable for elevated IL-6 and IL-10 and these cytokines are also implicated in NMOSD induction. Similarly, Toll-like receptors, which are activated by mRNA vaccines, have been implicated in both processes and both disorders respond to intravenous IV IgG and corticosteroids. Cytokine storm pathology is a central mechanism of both vaccine induced NMOSD and CLOCCs (65).
In terms of SARS-CoV-2 variants in our case series, it is difficult to assess which types were most often implicated. None of the individual case reports discussed which variant was responsible for the reported COVID-19 case associated with NMOSD onset. Except in one case, the original case reports and case series, did not document the date of infection, rendering it difficult to assess which variant was the dominant strain at the time. Furthermore, the publications that reviewed cases of SARS-Cov-2 associated NMOSD were published in 10 different countries across a 3 three-year time span. Using either the date of publication or the date the paper was received to determine the latest possible date that each case of SARS-CoV-2 infection, we found 8 cases reported in 2020, 3 cases in 2021, and 4 case in 2022. Given the diversity of locations and the range of dates of publication and the failure of these publications to document the date of infection, it is not possible to provide reliable data on which variants were represented in this case series. That said, most cases would have contracted the earlier pre-Omicron variants of SARS-CoV-2 (66).
The latency period between vaccine or infection exposure and NMOSD clinical onset ranged from 1 to 120 days but the majority of patients developed neurological symptoms within 1–2 weeks following exposure to the virus or the vaccine. In order for a disorder to be considered vaccine induced, the WHO suggests that there should be a clear temporal relationship between exposure and disease onset. The latency period between the exposure and the adverse event, however, was not defined by the WHO (67). Other studies that attempted to demonstrate a causal link between vaccination and disease onset included various latency time ranges from 8 weeks to 5 months (68, 69). For example, Karussis et al. (68) completed a PubMed search from 1979 to 2013 reviewing 71 documented cases of post-vaccination CNS demyelination secondary to various vaccines including influenza, HPV, and hepatitis A or B vaccines. In their review, symptoms typically manifested within 2 weeks (mean: 14.2 days), however, they also included delayed presentations from 4 weeks to 5 months post-vaccination (68). One study assessing the association between hepatitis B vaccination and the development of MS between 1991 and 1997 utilized an 8-week latency period between vaccination exposure and disease onset (69). Given the rarity of NMOSD, in our study, we included a delayed latency period of up to 120 days to ensure completeness of the data. However, the majority of the cases presented with a latency period of less than 30 days. The mean latency period between SARS-CoV-2 infection and NMOSD development was 34 days [Standard deviation (SD) 39 days]. Of the 11 cases that reported the latency period, only 3 were over 30 days. Of the 21 patients that developed de novo NMOSD following the COVID-19 vaccine, all patients had a latency period of less than 30 days (mean: 10 days). Of the patients that developed a relapsing CNS demyelination consistent with NMO following exposure to the COVID-19 vaccine, only one of the 5 cases presented with a latency period of more than 30 days (mean: 30 days). This short-term association, however, should be considered with reservations as there are no controls or quantitative risk outcomes (e.g., odds ratios).
The cases presenting with a long latency distribution, in which NMOSD occurred more than 28 days after the exposure, may represent coincidental NMOSD manifestations. In the long latency cases, the vaccine or infectious exposure and NMOSD disease onset may be causally related rather than causative. These cases of prolonged latency may represent sporadic NMOSD that may have occurred regardless of the exposure, especially as both the SARS-CoV-2 infections and COVID-19 vaccinations were wide spread over a brief interval and a large portion of the population encountered at least one of these exposures. The cases with a short latency distribution of less than 28 days are less likely to be coincidental, although causation cannot be proven. Both short and long latency periods were included, however, for completeness as this is a hypothesis generating study. We advise a case-controlled study for a more rigorous investigation.
The current data, spanning from December 2019 to the present provides too brief of an overview to give insight into the long-term risks of para-post infectious and post vaccine associated NMOSD. The data suggests, however, that if SARS-CoV-2 or COVID-19 vaccine exposed patients meet the diagnostic criteria for NMOSD, they should be managed like any other NMOSD patient to optimize the clinical outcome.
Females comprised the majority (76%) of cases in this series. This female preponderance corresponds with data in the literature that indicates a 2-fold higher incidence among females with NMOSD compared with males (70). The female preponderance found in our series may be secondary to a heightened immune response against self and foreign antigens in females compared to males.
With 24% of cases having a prior immune-mediated condition, de novo and relapsing NMOSD manifestations may be more prevalent among those with a pre-existing autoimmune disease. The results of this review suggest that in some susceptible individuals, exposure to the SARS-CoV-2 infection or COVID-19 vaccine may introduce a short-term risk of CNS demyelination.
Although this review indicates that there is a plausible association between the COVID-19 vaccination and NMOSD, the number of cases appears to be rare, and vaccination is still strongly encouraged. Currently, epidemiological and clinical data suggests that the benefits of vaccination conferred to both the individual and the public supersedes the possible risk of NMOSD associated with vaccination (34, 71). Furthermore, given the large number of patients that have received the COVID-19 vaccination, only a few reports have documented NMOSD manifestations following the vaccine, indicating that this is an uncommon occurrence.
This is a comprehensive systemic review of NMOSD cases associated with SARS-CoV-2 infections and the COVID-19 vaccine, including 34 published reports and 41 individual cases. The majority of cases reported in the existing literature were presented as case reports, and the few case series available were often more broadly focused on a variety of CNS demyelinating disorders rather than exclusively discussing NMOSD.
Given the established temporal relationship between SARS-CoV-2 infections and COVID-19 vaccination and the onset of NMOSD, our systemic review adds the current literature that underscores a potential link between viral infections and vaccinations and the development of de novo and relapsing NMOSD. This review suggests a probable association between post-infectious or vaccine triggered autoimmune mediated CNS demyelinating astrocytopathy. Our findings also suggest that vaccine and viral triggered CNS autoimmune demyelination may be more common among individuals with a pre-existing autoimmune disorder or a family history of autoimmune disease. However, the heterogeneity of the clinical data prevents a metanalysis from being performed. Although a causative relationship cannot be established on a temporal association alone, raising awareness of this potential correlation may influence the diagnosis and management of future patients presenting with demyelinating sequalae in the setting of infectious or vaccine mediated triggers. The lack of a control group prevented our ability to generate standard risk outcomes and future studies involving a control group are merited. This paper provides evidence for hypothesis generation that can be further tested with a case-control study allowing for a more detailed characterization of demographic, clinical characteristic, and genetic data to prove causality.
Strengths of this review include the comprehensive search of the literature, the detailed adjudication of cases and the comparison of COVID-19 vaccine and SARS-CoV-2 infection. Limitations included the small number of cases with retrospective observations. Several cases included incomplete workups and there was heterogeneity of clinical data available, impairing the ability to complete a meta-analysis.
Conclusion
This systematic review comprehensively demonstrates a temporal association between de novo and relapsing forms of NMOSD and SARS-CoV-2 infections and COVID-19 vaccinations. Association, however, does not away imply causation. We would also emphasize that the protective benefits that the COVID-19 vaccine conveys to both the individual and society as a whole far outweigh any hypothetical risk that would be implied from this review. Our report suggests, a link between the COVID-19 virus or vaccine exposure and the pathological cascade that may induce clinical NMOSD symptoms. Furthermore, given the brief duration of the study, the potential long-term effects of exposure are unknown. This systematic review does suggest that NMO manifestations following a COVID-19 viral or vaccine exposure may be more common than currently recognized, particularly among high-risk demographic groups. This association requires further study using quantitative epidemiological assessments in representative populations to better quantify the risk of developing clinical symptoms of NMOSD.
Author contributions
MW and TH contributed to conceptualization, study design, literature search, obtaining data, data management and analyses, data verification, drafting the manuscript and figures, manuscript revisions, statistical analysis, administrative oversight, study supervision, and validation. All authors contributed to the article and approved the submitted version.
Acknowledgments
The authors are grateful the University of Maryland Health Sciences and Human Services Library staff for their assistance on our review and development of the Covidence database.
Conflict of interest
The authors declare that the research was conducted in the absence of any commercial or financial relationships that could be construed as a potential conflict of interest.
Publisher’s note
All claims expressed in this article are solely those of the authors and do not necessarily represent those of their affiliated organizations, or those of the publisher, the editors and the reviewers. Any product that may be evaluated in this article, or claim that may be made by its manufacturer, is not guaranteed or endorsed by the publisher.
Supplementary material
The Supplementary material for this article can be found online at: https://www.frontiersin.org/articles/10.3389/fneur.2023.1099758/full#supplementary-material
References
1. Ismail, II, and Salama, S. Association of CNS demyelination and COVID-19 infection: an updated systematic review. J Neurol. (2021) 1–36.
2. World Health Organization. (2022). WHO COVID-19 dashboard. Available at: https://covid19.who.int (Accessed November 2, 2022).
3. Desforges, M, Le Coupanec, A, Dubeau, P, Bourgouin, A, Lajoie, L, Dubé, M, et al. Human coronaviruses and other respiratory viruses: underestimated opportunistic pathogens of the central nervous system? Viruses. (2019) 12:14. doi: 10.3390/v12010014
4. Kim, JE, Heo, JH, Kim, HO, Song, SH, Park, SS, Park, TH, et al. Neurological complications during treatment of Middle East respiratory syndrome. J Clin Neurol (Seoul, Korea). (2017) 13:227–33. doi: 10.3988/jcn.2017.13.3.227
5. Ghosh, R, De, K, Roy, D, Mandal, A, Biswas, S, Biswas, S, et al. A case of area postrema variant of neuromyelitis optica spectrum disorder following SARS-CoV-2 infection. J Neuroimmunol. (2021) 350:577439. doi: 10.1016/j.jneuroim.2020.577439
6. Khair, AM, Nikam, R, Husain, S, Ortiz, M, and Kaur, G. Para and post-COVID-19 CNS acute demyelinating disorders in children: A case series on expanding the Spectrum of clinical and radiological characteristics. Cureus. (2022) 14:e23405. doi: 10.7759/cureus.23405
7. Corrêa, DG, de Souza Lima, FC, da Cruz Bezerra, D, Coutinho, AC, and Hygino da Cruz, LC. COVID-19 associated with encephalomyeloradiculitis and positive anti-aquaporin-4 antibodies: cause or coincidence? Mult Scler J. (2021) 27:973–6. doi: 10.1177/1352458520949988
8. Yavari, F, Raji, S, Moradi, F, and Saeidi, M. Demyelinating changes alike to multiple sclerosis: a case report of rare manifestations of COVID-19. Case Rep Neurol Med. (2020) 2020:1–4. doi: 10.1155/2020/6682251
9. Lana-Peixoto, MA, and Talim, N. Neuromyelitis optica spectrum disorder and anti-MOG syndromes. Biomedicine. (2019) 7:42. doi: 10.3390/biomedicines7020042
10. Koga, M, Takahashi, T, Kawai, M, Fujihara, K, and Kanda, T. A serological analysis of viral and bacterial infections associated with neuromyelitis optica. J Neurol Sci. (2011) 300:19–22. doi: 10.1016/j.jns.2010.10.013
11. Zhong, X, Zhou, Y, Lu, T, Wang, Z, Fang, L, Peng, L, et al. Infections in neuromyelitis optica spectrum disorder. J Clin Neurosci. (2018) 47:14–9. doi: 10.1016/j.jocn.2017.10.005
12. Langer-Gould, A, Qian, L, Tartof, SY, Brara, SM, Jacobsen, SJ, Beaber, BE, et al. Vaccines and the risk of multiple sclerosis and other central nervous system demyelinating diseases. JAMA Neurol. (2014) 71:1506–13. doi: 10.1001/jamaneurol.2014.2633
13. Wingerchuk, DM, Banwell, B, Bennett, JL, Cabre, P, Carroll, W, Chitnis, T, et al. International consensus diagnostic criteria for neuromyelitis optica spectrum disorders. Neurology. (2015) 85:177–89. doi: 10.1212/WNL.0000000000001729
14. Aubart, M, Roux, CJ, Durrleman, C, Gins, C, Hully, M, Kossorotoff, M, et al. Neuroinflammatory disease following severe acute respiratory syndrome coronavirus 2 infection in children. J Pediatr. (2022) 247:22–28.e2. doi: 10.1016/j.jpeds.2022.05.018
15. Barone, S, Rapisarda, L, Manzo, L, Mechelli, A, Pascarella, A, Bruno, P, et al. A case of neuromyelitis optica spectrum disorder (NMOSD) and acute myositis following SARS-CoV-2 infection. J Neurol Sci. (2021) 429:119862. doi: 10.1016/j.jns.2021.119862
16. Batum, M, Kisabay Ak, A, and Mavioğlu, H. Covid-19 infection-induced neuromyelitis optica: a case report. Int J Neurosci. (2022) 132:999–1004. doi: 10.1080/00207454.2020.1860036
17. Das, D, Bhattacharjee, H, Rehman, O, Deori, N, Magdalene, D, Bharali, G, et al. Neuromyelitis optica spectrum disorder post-COVID-19 infection: A rare case report from Northeast India. Indian J Ophthalmol. (2022) 70:1833–6. doi: 10.4103/ijo.IJO_61_22
18. Jentzer, A, Carra-Dallière, C, Lozano, C, Riviere, S, Darmon, O, Ayrignac, X, et al. Neuromyelitis optica spectrum disorder following COVID-19 infection with increase in pre-existing anti-aquaporin-4 antibodies. J Neurol. (2022) 269:2850–3. doi: 10.1007/s00415-022-10972-9
19. Kim, Y, Heo, D, Choi, M, and Lee, JM. A case presenting with Neuromyelitis Optica Spectrum disorder and infectious Polyradiculitis following BNT162b2 vaccination and COVID-19. Vaccine. (2022) 10:1028. doi: 10.3390/vaccines10071028
20. Mirmosayyeb, O, Ghaffary, EM, Bagherieh, S, Barzegar, M, Dehghan, MS, and Shaygannejad, V. Post COVID-19 infection neuromyelitis optica spectrum disorder (NMOSD): A case report-based systematic review. Mult Scler Relat Disord. (2022) 60:103697. doi: 10.1016/j.msard.2022.103697
21. Rafique, S, Wasim, A, Sultan, T, and Ahmad, A. Post-COVID neuromyelitis optica spectrum disorder. J Coll Physicians Surg Pak. (2021) 31:138–40. doi: 10.29271/jcpsp.2021.Supp2.S138
22. Sardar, S, Safan, A, Okar, L, Sadik, N, and Adeli, G. The diagnostic dilemma of bilateral optic neuritis and idiopathic intracranial hypertension coexistence in a patient with recent COVID-19 infection. Clin Case Rep. (2021) 9:e04347. doi: 10.1002/ccr3.4347
23. Shaw, VC, Chander, G, and Puttanna, A. Neuromyelitis optica spectrum disorder secondary to COVID-19. Br J Hosp Med. (2020) 81:1–3. doi: 10.12968/hmed.2020.0401
24. Anamnart, C, Tisavipat, N, Owattanapanich, W, Apiwattanakul, M, Savangned, P, Prayoonwiwat, N, et al. Newly diagnosed neuromyelitis optica spectrum disorders following vaccination: case report and systematic review. Mult Scler Relat Disord. (2022) 58:103414. doi: 10.1016/j.msard.2021.103414
25. Arora, S, Sehgal, V, and Bansal, P. Neuromyelitis Optica like presentation following COVID vaccination. Eur J Mol Clin Med. (2021) 8:909–16.
26. Badrawi, N, Kumar, N, and Albastaki, U. Post COVID-19 vaccination neuromyelitis optica spectrum disorder: case report & MRI findings. Radiol Case Rep. (2021) 16:3864–7. doi: 10.1016/j.radcr.2021.09.033
27. Ballout, AA, Babaie, A, Kolesnik, M, Li, JY, Hameed, N, Waldman, G, et al. A single-health system case series of new-onset CNS inflammatory disorders temporally associated with mRNA-based SARS-CoV-2 vaccines. Front Neurol. (2022) 13:264. doi: 10.3389/fneur.2022.796882
28. Caliskan, I, Bulus, E, Afsar, N, and Altintas, A. A case with new-onset neuromyelitis optica spectrum disorder following COVID-19 mRNA BNT162b2 vaccination. Neurologist. (2022) 27:147–50. doi: 10.1097/NRL.0000000000000420
29. Chen, S, Fan, XR, He, S, Zhang, JW, and Li, SJ. Watch out for neuromyelitis optica spectrum disorder after inactivated virus vaccination for COVID-19. Neurol Sci. (2021) 42:3537–9. doi: 10.1007/s10072-021-05427-4
30. Fujikawa, P, Shah, FA, Braford, M, Patel, K, and Madey, J. Neuromyelitis optica in a healthy female after severe acute respiratory syndrome coronavirus 2 mRNA-1273 vaccine. Cureus. (2021) 13:e17961. doi: 10.7759/cureus.17961
31. Janarious, A., and Ullah, S., (2022). Severe presentation of Neuromyelitis Optica with positive antibody following COVID-19 vaccination (P 7–1.006). Neurology, [online] 98 (18 Supplement). Available at: https://n.neurology.org/content/98/18_Supplement/1666
32. Kim, KH, Kim, SH, Park, NY, Hyun, JW, and Kim, HJ. Onset of various CNS inflammatory demyelination diseases following COVID-19 vaccinations. Mult Scler Relat Disord. (2022) 68:104141. doi: 10.1016/j.msard.2022.104141
33. Khayat-Khoei, M, Bhattacharyya, S, Katz, J, Harrison, D, Tauhid, S, Bruso, P, et al. COVID-19 mRNA vaccination leading to CNS inflammation: a case series. J Neurol. (2022) 269:1093–106. doi: 10.1007/s00415-021-10780-7
34. Kuntz, S., Saab, G., and Schneider, R., (2022). Antibody-positive Neuromyelitis Optica Spectrum disorder after second COVID-19 vaccination: a case report. SN Comprehensive Clinical Medicine, 4:130.
35. Lévi-Strauss, J, Provost, C, Wane, N, Jacquemont, T, and Mélé, N. NMOSD typical brain lesions after COVID-19 mRNA vaccination. J Neurol. (2022) 269:5213–5. doi: 10.1007/s00415-022-11229-1
36. Motahharynia, A, Naghavi, S, Shaygannejad, V, and Adibi, I. Fulminant neuromyelitis optica spectrum disorder (NMOSD) following COVID-19 vaccination: A need for reconsideration? Mult Scler Relat Disord. (2022) 66:104035. doi: 10.1016/j.msard.2022.104035
37. Shirah, B, Mulla, I, and Aladdin, Y. Optic neuritis following the BNT162b2 mRNA COVID-19 vaccine in a patient with systemic lupus erythematosus uncovering the diagnosis of Neuromyelitis Optica Spectrum disorders. Ocul Immunol Inflamm. (2022) 18:1–3. doi: 10.1080/09273948.2022.2089901
38. Tascı, YY, Nalcacoglu, P, Gumusyayla, S, Vural, G, Toklu, Y, and Yesılırmak, N. Aquaporin-4 protein antibody-associated optic neuritis related to neuroendocrine tumor after receiving an inactive COVID-19 vaccine. Indian J Ophthalmol. (2022) 70:1828–31. doi: 10.4103/ijo.IJO_2494_21
39. Tisavipat, N, Anamnart, C, Owattanapanich, W, Apiwattanakul, M, Savangned, P, Prayoonwiwat, N, et al. Author's response to the commentary: Neuromyelitis optica complicating COVID vaccinations and additional case reports. Mult Scler Relat Disord. (2022) 66:104055. doi: 10.1016/j.msard.2022.104055
40. Dinoto, A, Sechi, E, Ferrari, S, Gajofatto, A, Orlandi, R, Solla, P, et al. Risk of disease relapse following COVID-19 vaccination in patients with AQP4-IgG-positive NMOSD and MOGAD. Mult Scler Relat Disord. (2022) 58:103424. doi: 10.1016/j.msard.2021.103424
41. Fragoso, YD, Gomes, S, Gonçalves, MVM, Junior, EM, de Oliveira, BES, Rocha, CF, et al. New relapse of multiple sclerosis and neuromyelitis optica as a potential adverse event of Astra Zeneca AZD1222 vaccination for COVID-19. Mult Scler Relat Disord. (2022) 57:103321. doi: 10.1016/j.msard.2021.103321
42. Helmchen, C, Buttler, GM, Markewitz, R, Hummel, K, Wiendl, H, and Boppel, T. Acute bilateral optic/chiasm neuritis with longitudinal extensive transverse myelitis in longstanding stable multiple sclerosis following vector-based vaccination against the SARS-CoV-2. J Neurol. (2022) 269:49–54. doi: 10.1007/s00415-021-10647-x
43. Lohmann, L, Glaser, F, Möddel, G, Lünemann, JD, Wiendl, H, and Klotz, L. Severe disease exacerbation after mRNA COVID-19 vaccination unmasks suspected multiple sclerosis as neuromyelitis optica spectrum disorder: a case report. BMC Neurol. (2022) 22:1–4. doi: 10.1186/s12883-022-02698-y
44. Machado, C, Amorim, J, Rocha, J, Pereira, JM, Lourenço, E, and Pinho, J. Neuromyelitis optica spectrum disorder and varicella-zoster infection. J Neurol Sci. (2015) 358:520–1. doi: 10.1016/j.jns.2015.09.374
45. Mathew, T, Avati, A, D’Souza, D, Therambil, M, Baptist, AA, Shaji, A, et al. HIV infection associated neuromyelitis optica spectrum disorder: clinical features, imaging findings, management and outcomes. Mult Scler Relat Disord. (2019) 27:289–93. doi: 10.1016/j.msard.2018.11.014
46. Sellner, J, Hemmer, B, and Mühlau, M. The clinical spectrum and immunobiology of parainfectious neuromyelitis optica (Devic) syndromes. J Autoimmun. (2010) 34:371–9. doi: 10.1016/j.jaut.2009.09.013
47. Ghosh, R., De, K., Roy, D., Mandal, A., Biswas, S., Biswas, S., et al., (2007). A case of area postrema variant of neuromyelitis optica spectrum disorder following SARS-CoV-2 infection. J. Neuroimmunol. (2021) 350:577439. doi: 10.1016/j.jneuroim.2020.577439
48. Schirinzi, T, Landi, D, and Liguori, C. COVID-19: dealing with a potential risk factor for chronic neurological disorders. J Neurol. (2021) 268:1171–8. doi: 10.1007/s00415-020-10131-y
49. Shabani, Z. Demyelination as a result of an immune response in patients with COVID-19. Acta Neurol Belg. (2021) 121:859–66. doi: 10.1007/s13760-021-01691-5
50. Nolen, LT, Mukerji, SS, and Mejia, NI. Post-acute neurological consequences of COVID-19: an unequal burden. Nat Med. (2022) 28:20–3. doi: 10.1038/s41591-021-01647-5
51. Al-Ramadan, A, Rabab’h, O, Shah, J, and Gharaibeh, A. Acute and post-acute neurological complications of COVID-19. Neurol Int. (2021) 13:102–19. doi: 10.3390/neurolint13010010
52. Moreno-Escobar, MC, Kataria, S, Khan, E, Subedi, R, Tandon, M, Peshwe, K, et al. Acute transverse myelitis with Dysautonomia following SARS-CoV-2 infection: A case report and review of literature. J Neuroimmunol. (2021) 353:577523. doi: 10.1016/j.jneuroim.2021.577523
53. Lahiri, D, and Ardila, A. COVID-19 pandemic: a neurological perspective. Cureus. (2020) 12:e7889. doi: 10.7759/cureus.7889
54. Román, GC, Gracia, F, Torres, A, Palacios, A, Gracia, K, and Harris, D. Acute transverse myelitis (ATM): clinical review of 43 patients with COVID-19-associated ATM and 3 post-vaccination ATM serious adverse events with the ChAdOx1 nCoV-19 vaccine (AZD1222). Front Immunol. (2021) 12:879. doi: 10.3389/fimmu.2021.653786
55. Havla, J, Schultz, Y, Zimmermann, H, Hohlfeld, R, Danek, A, and Kümpfel, T. First manifestation of multiple sclerosis after immunization with the Pfizer-BioNTech COVID-19 vaccine. J Neurol. (2022) 269:55–8. doi: 10.1007/s00415-021-10648-w
56. Ismail, II, and Salama, S. A systematic review of cases of CNS demyelination following COVID-19 vaccination. J Neuroimmunol. (2022) 362:577765. doi: 10.1016/j.jneuroim.2021.577765
57. Wan, Y, Shang, J, Graham, R, Baric, RS, and Li, F. Receptor recognition by the novel coronavirus from Wuhan: an analysis based on decade-long structural studies of SARS coronavirus. J Virol. (2020) 94:e00127–25. doi: 10.1128/JVI.00127-20
58. Hirano, T, and Murakami, M. COVID-19: a new virus, but a familiar receptor and cytokine release syndrome. Immunity. (2020) 52:731–3. doi: 10.1016/j.immuni.2020.04.003
59. Reyfman, PA, Walter, JM, Joshi, N, Anekalla, KR, McQuattie-Pimentel, AC, Chiu, S, et al. Single-cell transcriptomic analysis of human lung provides insights into the pathobiology of pulmonary fibrosis. Am J Respir Crit Care Med. (2019) 199:1517–36. doi: 10.1164/rccm.201712-2410OC
60. Ismail, II, and Salama, S. Association of CNS demyelination and COVID-19 infection: an updated systematic review. J Neurol. (2022) 269:541–76. doi: 10.1007/s00415-021-10752-x
61. Kappelmann, N, Dantzer, R, and Khandaker, GM. Interleukin-6 as potential mediator of long-term neuropsychiatric symptoms of COVID-19. Psychoneuroendocrinology. (2021) 131:105295. doi: 10.1016/j.psyneuen.2021.105295
62. Feizi, P, Sharma, K, Pasham, SR, Nirwan, L, Joseph, J, Jaiswal, S, et al. Central nervous system (CNS) inflammatory demyelinating diseases (IDDs) associated with COVID-19: A case series and review. J Neuroimmunol. (2022) 371:577939. doi: 10.1016/j.jneuroim.2022.577939
63. Youn, T, and Yang, H. Cytotoxic lesion of the corpus callosum (CLOCCs) after SARS-CoV-2 mRNA vaccination. J Korean Med Sci. (2021) 36:e228. doi: 10.3346/jkms.2021.36.e228
64. Poussaint, TY, LaRovere, KL, Newburger, JW, Chou, J, Nigrovic, LE, Novak, T, et al. Multisystem inflammatory-like syndrome in a child following COVID-19 mRNA vaccination. Vaccine. (2021) 10:43. doi: 10.3390/vaccines10010043
65. Ohara, H, Shimizu, H, Kasamatsu, T, Kajita, A, Uno, K, Lai, KW, et al. Cytotoxic lesions of the corpus callosum after COVID-19 vaccination. Neuroradiology. (2022) 64:2085–9. doi: 10.1007/s00234-022-03010-y
66. Young, M, Crook, H, Scott, J, and Edison, P. Covid-19: virology, variants, and vaccines. BMJ Med. (2022) 1:e000040. doi: 10.1136/bmjmed-2021-000040
67. World Health Organization (2018). Causality assessment of an adverse event following immunization (AEFI): user manual for the revised WHO classification. (2nd ed.). Geneva: World Health Organization; Licence: CC BY-NC-SA 3.0 IGO. Available at: https://apps.who.int/iris/bitstream/handle/10665/259959/9789241513654-eng.pdf?sequence=1&isAllowed=y
68. Karussis, D, and Petrou, P. The spectrum of post-vaccination inflammatory CNS demyelinating syndromes. Autoimmun Rev. (2014) 13:215–24. doi: 10.1016/j.autrev.2013.10.003
69. Wraith, DC, Goldman, M, and Lambert, PH. Vaccination and autoimmune disease: what is the evidence? Lancet. (2003) 362:1659–66. doi: 10.1016/S0140-6736(03)14802-7
70. Papp, V, Magyari, M, Aktas, O, Berger, T, Broadley, SA, Cabre, P, et al. Worldwide incidence and prevalence of neuromyelitis optica: a systematic review. Neurology. (2021) 96:59–77. doi: 10.1212/WNL.0000000000011153
Keywords: neuromyelitis optica, COVID-19, severe acute respiratory syndrome coronavirus 2, COVID-19 vaccine, outcomes
Citation: Harel T, Gorman EF and Wallin MT (2023) New onset or relapsing neuromyelitis optica temporally associated with SARS-CoV-2 infection and COVID-19 vaccination: a systematic review. Front. Neurol. 14:1099758. doi: 10.3389/fneur.2023.1099758
Edited by:
Tatjana Pekmezovic, University of Belgrade, SerbiaReviewed by:
Vincent Van Pesch, Université Catholique de Louvain, BelgiumAbhay Ranjan, Indira Gandhi Institute of Medical Sciences, India
Mattia Fonderico, University of Florence, Italy
Karlo Toljan, Cleveland Clinic, United States
Copyright © 2023 Harel, Gorman and Wallin. This is an open-access article distributed under the terms of the Creative Commons Attribution License (CC BY). The use, distribution or reproduction in other forums is permitted, provided the original author(s) and the copyright owner(s) are credited and that the original publication in this journal is cited, in accordance with accepted academic practice. No use, distribution or reproduction is permitted which does not comply with these terms.
*Correspondence: Tamar Harel, dGFtYXIuaGFyZWxAdmEuZ292; dGhhcmVsQHNvbS51bWFyeWxhbmQuZWR1