- 1Moterum Technologies, Inc., Salt Lake City, UT, United States
- 2Department of Mechanical Engineering, University of South Florida, Tampa, FL, United States
Background: Gait impairments after stroke are associated with numerous physical and psychological consequences. Treatment with the iStride® gait device has been shown to facilitate improvements to gait function, including gait speed, for chronic stroke survivors with hemiparesis. This study examines the long-term gait speed changes up to 12 months after treatment with the gait device.
Methods: Eighteen individuals at least one-year post-stroke completed a target of 12, 30-minute treatment sessions with the gait device in their home environment. Gait speed was measured at baseline and five follow-up sessions after the treatment period: one week, one month, three months, six months, and 12 months. Gait speed changes were analyzed using repeated-measures ANOVA from baseline to each follow-up time frame. Additional analysis included comparison to the minimal clinically important difference (MCID), evaluation of gait speed classification changes, and review of subjective questionnaires.
Results: Participants retained an average gait speed improvement >0.21 m/s compared to baseline at all post-treatment time frames. Additionally, 94% of participants improved their gait speed beyond the MCID during one or more post-treatment measurements, and 88% subjectively reported a gait speed improvement.
Conclusion: Treatment with the gait device may result in meaningful, long-term gait speed improvement for chronic stroke survivors with hemiparetic gait impairments.
Clinical trial registration: https://clinicaltrials.gov/ct2/show/NCT03649217, identifier NCT03649217.
1 Introduction
Over seven million stroke survivors currently live in the United States (1), and early projections indicate this figure may rise to over 10 million in the next decade (1–3). Improvements in medical interventions have reduced mortality (4, 5), however the disability after stroke is often long-term and remains an economic burden globally (1) and a personal affliction for well over 60% of stroke survivors (6–8) and their caregivers (9). Impairments after stroke can be widespread with effects to multiple physiologic systems. However, when questioned on rehabilitation goals, a majority of stroke survivors cite improving gait as a top priority (10, 11). Gait dysfunction is experienced by more than 80% of stroke survivors (12), and approximately 30–40% of stroke survivors have limited to no walking ability, even after completion of traditional rehabilitation (13, 14).
The motivation to improve gait is multi-faceted. Impaired gait is inefficient, with a metabolic cost 40–50% higher than neurologically intact individuals (15), which can lead to difficulties performing daily activities (16). Impaired gait contributes to abnormal joint loading, which can lead to musculoskeletal complications and pain (17, 18). Additionally, impaired gait is associated with an increased risk of falls (12, 19) which compromises safety and contributes to the seven-fold higher risk of fractures seen in individuals with a history of stroke (20). Adding to the potential physical consequences of falls, fear of falling can further limit community participation, which contributes to mental health issues related to isolation, among other things (21). The need to enhance gait training outcomes post-stroke is critical; however, access to effective, long-term treatment can be insufficient, especially for chronic stroke survivors with limited access to clinical environments and/or resources.
The assessment of gait function provides unique insight not only into the disability status and rehabilitation needs of stroke survivors, but their overall health as well. The most studied and utilized measurement of gait function is the measurement of self-paced gait speed (7, 22). Despite its simplicity, the utility of gait speed measurement is heavily emphasized in medical literature with its clinical value stated to rival routinely measured vital signs such as blood pressure and pulse (23). While commonly associated with quality of life, general health status, and functional abilities, gait speed measurement has additionally been praised for its predictive value with factors such as mortality (24), falls (25), and community participation (26, 27). Its versatility enables utilization within multiple settings, including home environments (28), earning gait speed the highest level of outcome measure recommendation by an expert chronic stroke panel (StrokEDGE) (29) and designation as a core outcome measure for chronic stroke within clinical practice guidelines published by the Journal of Neurologic Physical Therapy (30).
The iStride® gait device (31) (Moterum Technologies, Inc.) is a wearable gait treatment device designed for individuals with hemiparetic gait impairments caused by stroke (32, 33). The device, worn on the foot of the non-paretic limb during overground ambulation, features four kinetic wheels (34) which alter interlimb coordination.
While ambulating with the gait device, the wheel motion causes a posterior translation of the non-paretic limb during mid-stance. This motion lengthens the steps on the paretic side – a mechanism which can reduce asymmetry for some individuals through error augmentation, as seen with split-belt treadmill training (35, 36). Additionally, the device creates a subtle destabilization of the non-paretic limb, which encourages greater usage of the paretic limb during the gait cycle and prompts the user to adapt to such instability throughout the treatment session and with repeated sessions. Encouraging usage of the paretic limb, a central principle of constraint-induced movement therapy for the lower extremity (37), is a key reason the device is donned to the foot of the non-paretic limb. Before-and-after studies (38, 39) conducted with 27 ambulatory post-stroke participants with heterogenous gait patterns have revealed post-treatment benefits to symmetry, functional mobility and balance, and gait speed after four weeks of treatment. We suspect that the device’s therapeutic effects combine uniquely to benefit each user individually. The gait device and its associated motion are shown in Figure 1. Further details of the device’s development, design, and mechanism can be reviewed in previously published manuscripts (32, 33, 36, 38).
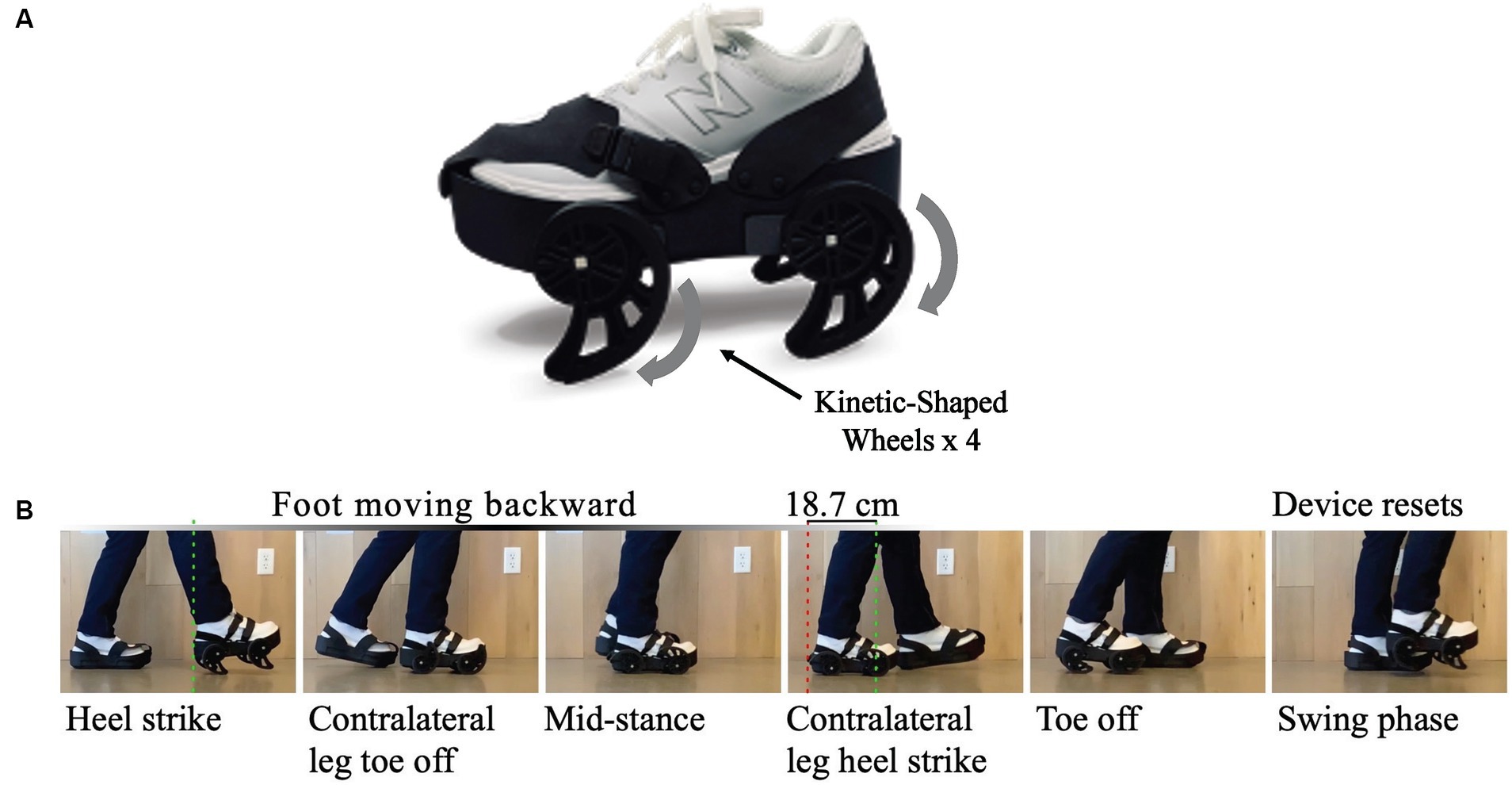
Figure 1. (A) The iStride® gait device. (B) Gait device motion: As the user takes a step, the device pushes the nonparetic foot backward during stance. This exaggeration of the step length asymmetry for some users yields a more symmetric gait pattern once the device is removed and the user returns to overground walking without the device. In addition, the device encourages usage of the paretic leg by slightly destabilizing the nonparetic leg. A similar height but stationary platform is worn on the foot of the paretic limb for symmetry.
The gait device was designed to be lightweight and portable, therefore, offering the potential for gait treatment to occur outside of clinical environments, a likely benefit for individuals with mobility restriction and difficulty accessing clinical settings. To investigate the feasibility and efficacy of treatment with the gait device in natural settings, a recent study explored treatment with the gait device in participants’ homes (39). Outcome measures centering on the functional aspects of gait that could be routinely measured in home settings were selected, including gait speed. Results after 12 treatment sessions revealed clinically relevant improvements, beyond the minimal detectable change or minimal clinically important difference, for both gait speed and functional balance, indicating an immediate post-treatment benefit for the home-use translation of this device (39). Retention of these gait improvements, however, remains undetermined, making the relevant, long-term clinical value unknown.
The objective of this study was to explore the long-term effects of treatment with the gait device in the home environment for individuals with gait impairments from chronic stroke. Specifically, this study investigates retention of the post-treatment therapeutic effect observed for gait speed by evaluating several follow-up time frames after the treatment period. Our hypothesis is that the gait speed improvement attained after gait device treatment will be sustained through all measured time frames.
2 Materials and methods
2.1 Selection criteria
Inclusion and exclusion criteria were identified as follows. Inclusion criteria: (1) age 21–80, (2) one or more cerebral strokes (all on the same side), (3) stroke occurred at least six months previously, (4) gait asymmetry (assessed by visual observation) but can walk independently with or without a cane, (5) no evidence of severe cognitive impairment that would interfere with understanding instructions, (6) not currently receiving physical therapy, (7) no evidence of one-sided neglect affecting ambulation, (8) adequate walking space within the home, and (9) weight less than 250 pounds. Exclusion Criteria: (1) uncontrolled seizures, (2) pregnancy, (3) metal implants (stents), (4) chronic obstructive pulmonary disease, (5) uncontrolled high blood pressure, (6) myocardial infarction within the last 180 days, (7) head injury within the last 180 days, or (8) a history of a neurologic disorder other than stroke. Additionally, during the period of treatment with the gait device, participants were excluded if the supervising physical therapist observed concerns regarding the participant’s ability to complete the treatment safely. Recruitment occurred during the months of July 2018 through September 2018. Treatment occurred between July 2018 and December 2018. All study-related follow-up was completed in December 2019.
Eighteen individuals with chronic stroke participated in this study, which features the long-term gait speed follow-up results from our home-based study with the gait device (39). This prior study reported results from a sample size of 21 participants. We derived the sample size for this study using power analyses from two previous studies using the gait device (32, 38). In the first study (32), the t-test was powered between pre-treatment and post-treatment data in healthy individuals and calculated an effect size of 0.68 for step length difference, resulting in an estimated minimal sample size of 18 participants. We initially included a higher number of participants since we expected more variation when testing on individuals with stroke. The second study (38), based on a pilot in-clinic study using the device with individuals with stroke, calculated an effect size of 0.71 for gait speed. A power analysis based on gait speed showed that statistical findings from 21 participants would obtain a power of 0.85. This power analysis does exclude one participant who started at a very fast walking speed of 1.14 m/s (and ended with a speed of 1.45 m/s), which is uncommonly fast for an individual with stroke; all of our participants in this study started with a gait speed less than 1.0 m/s. Note that these studies used step length asymmetry as a primary measure (which is not a variable in this study). Between the one-week and 12-month follow-up sessions, three out of the 21 participants did not complete all follow-up sessions. Since we are reporting repeated-measures statistical tests in this study, only the results of the 18 participants who completed all outcome assessments at all time periods will be included.
2.2 Experimental setup
The study followed a single group, before-after design with multiple follow-ups. Eligibility verification included an initial phone screen followed by a home visit to confirm compliance with eligibility criteria and to assess the home environment for suitability of device treatment. After consenting to participate, the participants’ gait parameters were measured at baseline (approximately one week before starting treatment), followed by four weeks of treatment with the gait device. After treatment was complete, gait speed was measured at five follow-up time frames: one week, one month, three months, six months, and 12 months post-treatment. At the final follow-up session, participants were provided a questionnaire regarding their clinical trial experience and observed gait changes after treatment with the gait device. All study aspects were performed within the participants’ home environments and were overseen by licensed, non-employee physical therapists hired as contractors for clinical trial data collection. This study was approved by the Western Institutional Review Board (IRB) and was confirmed to meet ethical standards for research with human participants. The study was registered with the identifier NCT03649217. Each participant signed a consent form that was approved by the Western Institutional Review Board prior to their study inclusion.
2.3 Treatment sessions
The participants were treated using the gait device in their home environment three times per week for four weeks (for a target of 12 treatment sessions). During each treatment session, the participant wore the device on their non-paretic foot. An approximate height-matched platform was worn on the paretic foot. The participants ambulated over ground on the gait device in their home environment for a maximum of 30 minutes during each treatment session. Rest breaks were provided at five-minute intervals (or more frequently if requested by the participants). Ambulation on the device was supervised by licensed physical therapists who provided the level of mobility assistance needed for participant safety and comfort while ambulating on the device. No other treatment or physical therapy services were provided to the participants during the treatment period.
2.4 Gait assessments
Gait speed assessment occurred at baseline (approximately one week before treatment) and at five follow-up time frames: one week, one month, three months, six months, and 12 months after the four-week treatment period. Gait speed was measured using the 10-Meter Walk Test (10MWT) at their comfortable walking speed. The protocol specified a 12-meter course using a measured distance of 10 meters and an untimed acceleration/deceleration distance of 1 meter. Three trials were conducted and averaged to determine the participant’s gait speed. For each participant, the most ideal home location (both for treatment with the gait device and for gait speed assessment) was identified by the therapist. This determination was based on identifying the longest straight path for walking in the home that was without thresholds, obstacles, or other walking surface changes. Testing setups were kept consistent within each participant’s environment and across all time frames to allow for within-subject comparisons. Figure 2 shows the study procedures and timeline.
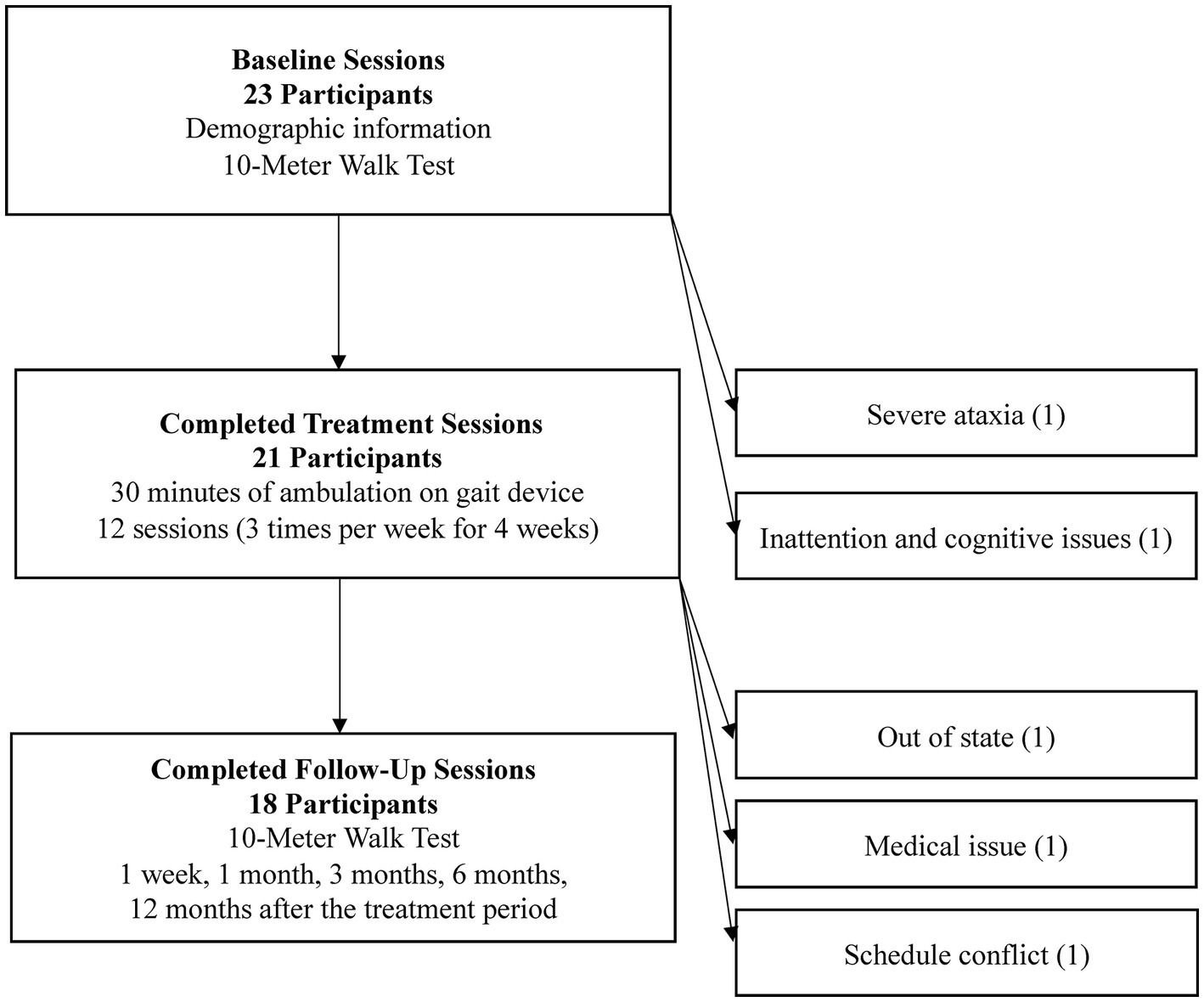
Figure 2. Study participants at each stage. Key study activities are listed within each phase, and reasons for non-participation are available to the right of each phase heading.
2.5 Data analysis
Normality of data was checked using the Shapiro–Wilk test. Sphericity was evaluated using Mauchly’s test for sphericity, and corrections using Greenhouse–Geisser estimates were applied if sphericity had been violated. A one-way repeated measures ANOVA test was conducted with gait speed as the dependent variable and time frame as the independent variable (baseline and five follow-up time frames). When statistical significance was found (using an alpha of 0.05), Tukey’s honestly significant difference post-hoc test was performed with Bonferroni corrections. Statistical analyses were performed using SPSS Version 26 software (IBM Corporation, Armonk, NY).
To monitor for the meaningfulness of gait speed changes, we compared each individual’s gait speed change as well as the study group average to the minimal clinically important difference (MCID) for gait speed improvement (40). The MCID refers to the smallest amount of change in an outcome that might be considered “important” to the patient or clinician. Additionally, the speed of an individual’s gait also corresponds with their ability to participate within the community. A study using walking data and activity monitors by Fulk reported that a comfortable gait speed of 0.49 m/s discriminated between household and community ambulators and a speed of 0.93 m/s discriminated between limited and unlimited community ambulators (27). To monitor for changes in expected community participation ability, each participant’s walking speed was compared to these gait speed classifications, as well as a “normal walking speed” classification (>1.2 m/s) as characterized by Fritz and Lusardi (23), during each study time frame. Finally, participant responses to a questionnaire regarding clinical trial experience and subjective gait observations after treatment were manually tabulated for the percentage of positive or negative responses to each questionnaire item.
3 Results
3.1 Participants
Twenty-three participants were initially included for study participation. Figure 2 shows the study activities and the total number of participants after each study phase. Key study activities are listed within each phase, and reasons for non-participation are available to the right of each phase heading. The discussed results and analysis center on the 18 study participants that completed all assessments through the twelve-month follow-up.
The demographic characteristics of the 18 study participants are shown in Table 1.
3.2 Statistical findings
Data for each time period followed a normal distribution (p > 0.2 on the Shapiro–Wilk test for all periods). Sphericity was violated (p < 0.05 on Mauchly’s Test of Sphericity), so Greenhouse–Geisser corrections were applied. Repeated-measures ANOVA revealed statistical significance for gait speed (measured using the 10MWT at comfortable gait speed) after treatment with the gait device {F(2.871, 48.815) = 9.195, p < 0.001}. Post hoc analysis revealed statistically significant differences from baseline to all follow-up time frames except three month (p < 0.0033, the Bonferroni corrected alpha based on 15 observations). Comfortable walking speed increased 0.27 m/s (p < 0.001) from baseline to one week post-treatment, 0.28 m/s (p = 0.002) from baseline to one month post-treatment, 0.25 m/s (p = 0.006) from baseline to three months post-treatment, 0.24 m/s (p = 0.001) from baseline to six months post-treatment, and 0.21 m/s (p = 0.001) from baseline to 12 months post-treatment. No statistically significant changes occurred between any of the post-treatment follow-up periods (p > 0.999). Due to near significance of the three-month follow-up, we also calculated the Cohen’s d effect sizes which were all 1.0 or greater, indicating a strong effect. Figure 3 shows the mean gait speed, associated p-values, and effect sizes at each of the six time frames. Additionally, while the focus of this paper is retention of gait speed, other measures of performance which have been reported (41, 42) showed similar patterns of improvement and retention. Supplementary Figure S1 shows outcome boxplots and association statistics.
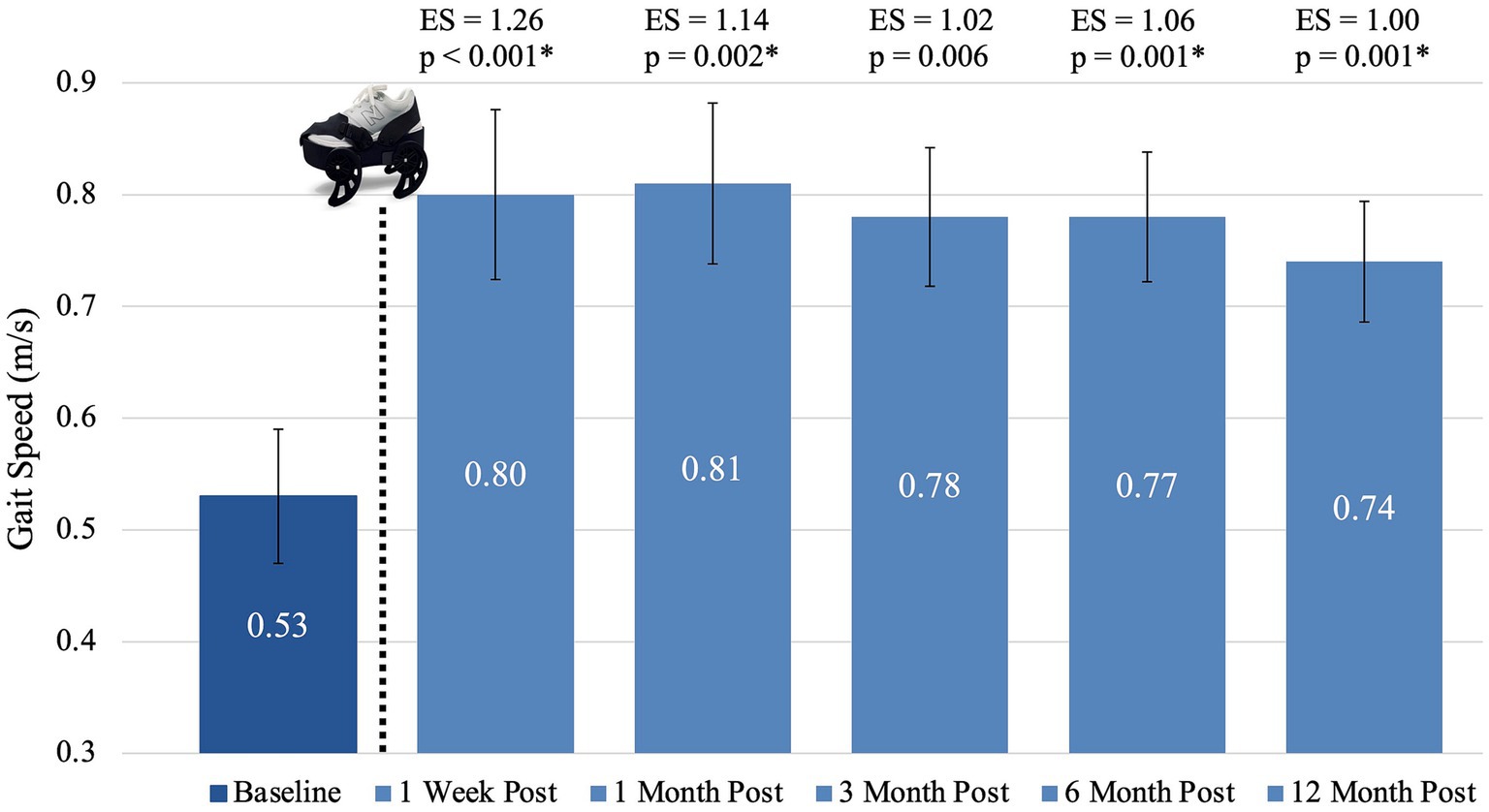
Figure 3. Mean gait speed at each time frame. The dotted line and gait device image represent the sequence within the study activities that the device treatment occurred. An asterisk (*) indicates statistical significance (p < 0.0033) compared to baseline. Bars represent standard error. ES, effect size.
3.3 Minutes of treatment
The 18 study participants completed an average of 11.7 treatment sessions (range 9–12 sessions) and 290 minutes on the device (range 165–360 minutes) out of a maximum 360 minutes of device treatment. Documented primary reasons for reduced completion included scheduling conflicts and fatigue. A strong, statistically significant relationship was found between treatment duration (measured in minutes of treatment on the gait device) and gait speed at 12 months post-treatment; r (16)=0.60, p = 0.009.
3.4 Minimal clinically important difference
Gait speed changes of the individual participants, mean improvements by time period, and percentages of participants exceeding the gait speed MCID (40) threshold of 0.16 m/s at each time frame are shown in Table 2. Numbers in bold indicate an improvement beyond the MCID value. Participants whose 10MWT included a “turn” due to spatial constraints are identified with an asterisk (*), and participants that received any additional therapy services throughout the entire clinical trial period are identified with a “TX.”
3.5 Gait speed classification
Using the Fulk gait speed classifications, at baseline, nine of the participants were classified as household ambulators, eight as limited community ambulators, and one as an unlimited community ambulator (27). After the device treatment, only between one and three participants remained household ambulators throughout the remainder of the study period. The remaining participants in this initial category improved one or two gait speed classifications. Twelve months post-treatment, two participants remained household ambulators, 12 were classified as limited community ambulators, and four improved to unlimited community ambulators. Figure 4 shows the number of participants in each gait speed classification during all study time frames (27). Additionally, in Figure 4 we highlight that one or two participants achieved a gait speed categorized as “normal speed” (at or above 1.2 m/s) during four of the five assessments after treatment with the gait device (23).
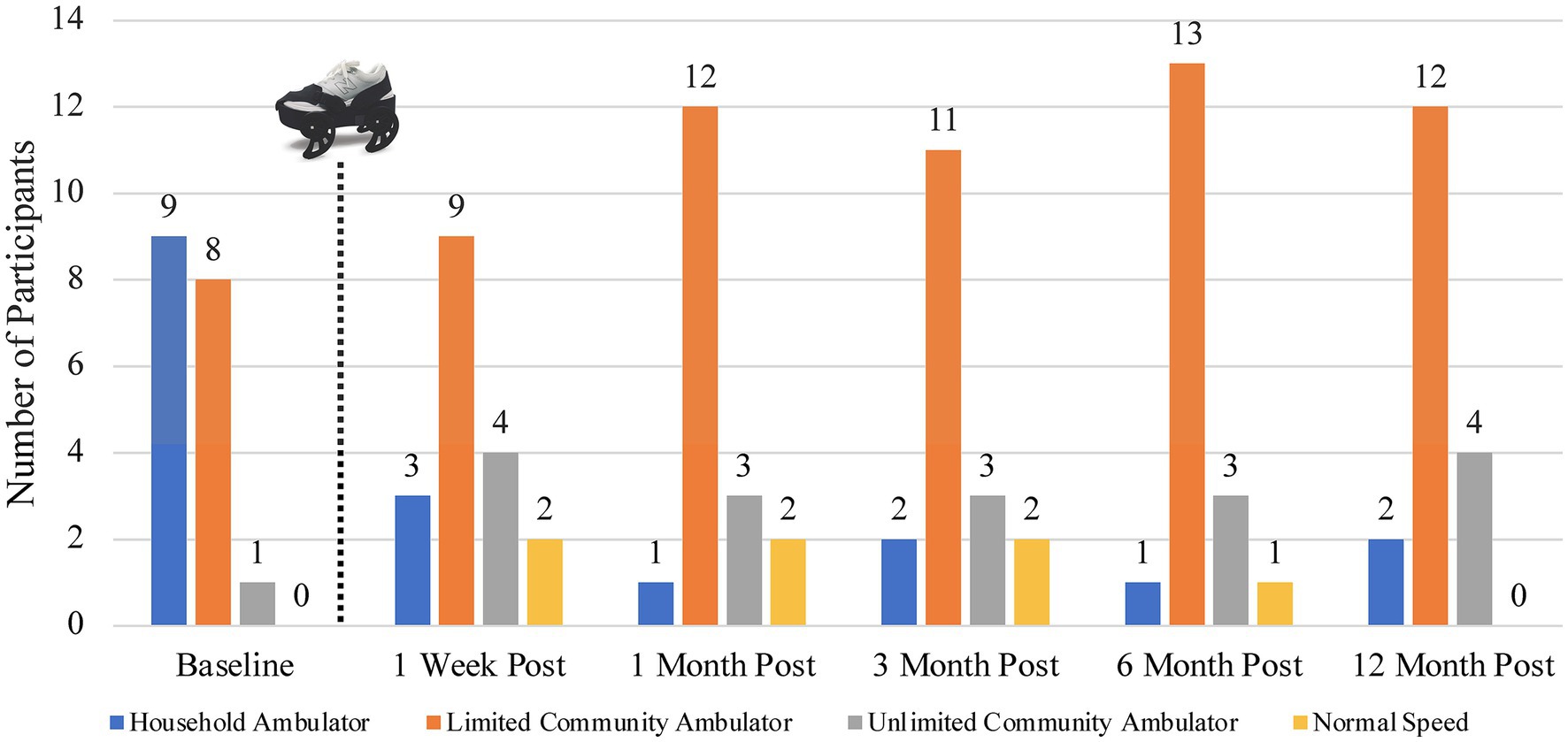
Figure 4. Number of participants in each gait speed category at each study time period utilizing the classifications proposed by Fulk (27). The dotted line and gait device image represent the sequence within the study activities that the device treatment occurred.
3.6 Questionnaire responses
The questionnaire consisted of multiple choice questions regarding observations of potential gait speed changes, functional independence changes, and clinical trial experience. (Note: questionnaire responses are only available for 17 of the 18 participants). Fifteen of 17 participants (88%) reported noticing an improvement in their walking speed, 12 of 17 participants (71%) reported improved functional independence, and 17 of 17 (100%) reported a positive clinical trial experience with the gait device. Questions and response percentages are shown in Table 3.
4 Discussion
The objective of this study was to investigate the long-term gait speed changes after treatment with the iStride® gait device in the home environment for individuals with gait impairments from chronic stroke. A review of the participants’ gait speed changes over the study period demonstrates an average gait speed improvement >0.21 m/s across the measured time frames and a gait speed improvement greater than the MCID (compared to baseline) one-year after treatment for over 60% of participants. These results are supported by the vast majority of participants (88%) who reported a subjective gait speed improvement as noted by questionnaire results at study completion.
The combined mechanisms utilized in the gait device provide a novel approach to gait treatment in the population of chronic stroke. While multiple gait treatment techniques and technologies cite the ability to improve the gait speed of stroke survivors, comparison to studies of various overground gait intervention approaches indicates an improvement of this magnitude (which is also retained and fosters an expected improvement in community participation ability) is notable (43). Specifically, a 2009 analysis of seven studies and nearly 400 participants found an average gait speed improvement of 0.07 m/s after traditional, overground gait training (14). DePaul et al. (44) used a motor-learning-science-based overground walking program, which resulted in an average 0.14 m/s gait speed improvement. Park et al. (45) compared the effects of gait training overground versus treadmills and found the largest gait speed improvement, from any of the training conditions, to be 0.1 m/s. The reported gait speed changes from the present study are approximately two to three times greater than those reported by these gait-focused studies and additionally highlight retention, a critical factor not demonstrated in the prior mentioned studies. Further support of the treatment effect is demonstrated by a strong, statistically significant relationship between treatment duration and gait speed 12 months post-treatment.
The gait speed results of this study also exceed those seen in our laboratory-based pilot study (38), suggesting a benefit for the adapted, home-based treatment protocol described in this study. Many studies have emphasized the impact of treatment “context” on motor adaptation and motor learning after stroke. For example, a contextual mismatch of cues is thought to account for the decreased locomotor transfer to overground walking following split-belt treadmill training (46), and Torres-Oviedo and Bastian (47) found that removing vision to eliminate the visual-proprioceptive contextual mismatch specific to treadmills improved the transfer of treadmill adaptation to natural walking. The greater improvements achieved in the present study compared to the pilot, laboratory-based study yield consideration that contextual differences between laboratory and home treatment environments may also influence outcomes after treatment with the gait device. Similarly, potential benefits of adapting and de-adapting (48) the modified gait pattern with natural, overground ambulation in the home environment following each treatment session is a mechanism that could be further explored as possibly contributing to our study’s findings. Moreover, while not yet entirely understood, the value of rehabilitation at home after stroke is further emphasized by the improved outcomes seen with home-centered approaches such as early supported discharge (49) or the effectiveness of the active “control” home environment condition in the LEAPS study (50, 51). Compared to a laboratory environment, performing this gait treatment in the context of where the individual resides (and performs the majority of their gait activities) may have enhanced the transfer and adoption of a modified gait pattern and facilitated the retained gait speed effects seen in the present study.
Continuing, or at least maintaining the functional improvements achieved during rehabilitation is a primary goal of post-stroke management. However, the factors influencing the retention of functional improvements after stroke are not fully understood. In upper extremity literature, studies have shown that gains in arm function after stroke can be maintained or even improved if use is sufficient (52). While seemingly less studied in the context of gait, multiple published studies suggest that critical gait speed thresholds must be reached to achieve increased levels of community participation (26, 27). The results of our study show that a majority (12/18, 67%) of participants achieved a higher gait speed classification immediately following treatment, and 10/17 (59%) maintained a greater gait speed classification at their 12-month follow-up session compared to baseline (27). Given the long-term, sustained gait speed improvement, these results suggest that an improved walking ability was achieved following treatment that could be maintained through increased participation in regular, daily activities. For example, Partcipant A was interviewed following his treatment with the gait device and described the ability to become substantially more active after treatment with the gait device, including resuming recreational activities. We suspect increased activity levels, such as these, may reinforce and sustain the immediate post-treatment gait speed improvements.
Interestingly, the group of participants that maintained a greater gait speed classification at their 12-month follow-up session includes five of the six participants that were two-years or less post-stroke and the most chronic participant who was 25+ years post-stroke at baseline. These results highlight the value of treatment in the immediate two-year post-stroke time frame and further emphasize that meaningful improvement is achievable even many years post-stroke. Moreover, reviewing the participants’ gait speeds over the 12-month period appears to reveal several unique patterns of gait speed change and retention. Some participants improved their gait speed post-treatment and maintained this improvement through the 12-month follow-up, such as Participants A and C, for example. Others demonstrated initial improvement post-treatment but returned to their original gait speed (approximately) by the 12-month follow-up (such as Participant H), and yet others continued to improve their gait speed over the 12-month period, despite no further treatment (such as Participant I). Future studies would be useful to differentiate these individual trends, as well as the specific characteristics that may have influenced treatment responsiveness and retention.
4.1 Limitations
There are several limitations to our study. When possible, consistent physical therapists were used with each of the participants in this study. This consistency, while minimizing interrater reliability issues, does not permit blinding of the therapists. Repeated outcomes testing could also introduce a practice effect, and retrospective recall could limit the accuracy of questionnaire results. Additionally, a lack of a control group limits direct comparison to standard treatments and does not explore the effect of the device in comparison to the concomitant walking activity. However, while the lack of a control group is a limitation, the effect of simply walking on the outcome of gait speed has been thoroughly investigated in the literature (with varying durations, contexts, and intensities explored) (45, 53–55). The outcomes of these studies demonstrate a substantially lesser gait speed effect than noted in the present study. Moreover, it is unlikely that the gait speed results could be attributed to spontaneous improvement given the chronicity of our study population.
As noted in our inclusion criteria, the clinical trial participants did not receive any additional physical therapy treatment from the clinical trial physical therapists or external physical therapists during the treatment period through the one-week follow-up. However, given the extended duration of the study, we did not preclude participants from obtaining services after this time. Six of 18 participants did resume some form of additional physical therapy treatment between the one-week follow-up and 12-month follow-up, as annotated in Table 2. Of these six participants, three received six or fewer total additional therapy sessions. Of the remaining three participants, two received services focusing on upper extremity function (with some full-body therapy included). It is important to note that two-thirds of our participants (12 out of 18 participants) did not receive any additional physical therapy treatment throughout the study duration.
We also encountered challenges related to gait speed assessment in the home environment. While the home environments of the majority of participants (12 of 18) were able to accommodate the spatial requirements for a 10MWT, six of the 18 participants required a “turn” to achieve the full 10 meters of walking due to spatial constraints within the home (and no suitable outdoor alternative). Of these six participants, two performed a turn after six meters, two turned after seven meters, and two turned after nine meters. Challenges as these are commonly encountered during testing in home environments (28), but are likely outweighed by the benefits of capturing outcomes in natural conditions. These participants have been identified with an asterisk in Table 2. Importantly, the overall trends seen in our results with all participants do not change if we remove the participants that had some additional physical therapy (between the one-week and 12-month follow-ups) or those that had a “turn” during their gait evaluation or both of these groups (see Supplementary Tables S1–S3).
5 Conclusion
The present study supports the usage of a four-week gait device treatment protocol for chronic stroke survivors in their home environment. Results indicate that the described treatment has the potential to result in long-term, meaningful gait speed improvement for individuals with hemiparetic gait impairments. This treatment appears impactful in its ability to facilitate clinically significant gait speed changes, which have been correlated in the literature with decreased disability and improved quality of life, in a relatively short time frame and from the home environment. Additionally, our findings provide additional support to the notion that novel treatments can enhance recovery, even many years post-stroke. These promising results warrant further study to elucidate the full impact of this home-use gait treatment device.
Data availability statement
The original contributions presented in the study are included in the article/Supplementary material, further inquiries can be directed to the corresponding author.
Ethics statement
The studies involving humans were approved by Western Institutional Review Board (now WCG). The studies were conducted in accordance with the local legislation and institutional requirements. The participants provided their written informed consent to participate in this study.
Author contributions
LR, DH, and KR designed the study. LR screened the participants and obtained informed consent. LR oversaw the study protocol. BD, DH, KR, NT, and SB analyzed the data. BD wrote the manuscript with critical feedback from all authors. All authors contributed to the article and approved the submitted version.
Funding
Funding for this study was provided by Moterum Technologies, Inc., Salt Lake City, USA.
Acknowledgments
The authors would like to thank the study participants and physical therapists for their time and efforts associated with participation in this study.
Conflict of interest
KR has a patent (US 9,295,302) related to this work. A management plan has been implemented and followed to reduce any effects of these conflicts of interest. The gait device is related to a commercial product by Moterum Technologies, Inc. BD, LR, DH, and SB receive a salary from Moterum Technologies. BD, LR, NT, DH, KR, and SB have stock options in Moterum Technologies, Inc.
Publisher’s note
All claims expressed in this article are solely those of the authors and do not necessarily represent those of their affiliated organizations, or those of the publisher, the editors and the reviewers. Any product that may be evaluated in this article, or claim that may be made by its manufacturer, is not guaranteed or endorsed by the publisher.
Supplementary material
The Supplementary material for this article can be found online at: https://www.frontiersin.org/articles/10.3389/fneur.2023.1089083/full#supplementary-material
References
1. Virani, SS, Alonso, A, Benjamin, EJ, Bittencourt, MS, Callaway, CW, Carson, AP, et al. Heart disease and stroke statistics-2020 update: a report from the American Heart Association. Circulation. (2020) 141:e139–596. doi: 10.1161/CIR.0000000000000757
2. Heidenreich, PA, Trogdon, JG, Khavjou, OA, Butler, J, Dracup, K, Ezekowitz, MD, et al. Forecasting the future of cardiovascular disease in the United States: a policy statement from the American Heart Association. Circulation. (2011) 123:933–44. doi: 10.1161/CIR.0b013e31820a55f5
3. Billinger, SA, Arena, R, Bernhardt, J, Eng, JJ, Franklin, BA, Johnson, CM, et al. Physical activity and exercise recommendations for stroke survivors: a statement for healthcare professionals from the American Heart Association/American Stroke Association. Stroke. (2014) 45:2532–53. doi: 10.1161/STR.0000000000000022
4. Center for Disease Control and Prevention. Vital signs: recent trends in stroke death rates – United States, 2000–2015. Available at: https://www.cdc.gov/mmwr/volumes/66/wr/mm6635e1.htm (Accessed May 7, 2020).
5. Lackland, DT, Roccella, EJ, Deutsch, AF, Fornage, M, George, MG, Howard, G, et al. Factors influencing the decline in stroke mortality: a statement from the American Heart Association/American Stroke Association. Stroke. (2014) 45:315–53. doi: 10.1161/01.str.0000437068.30550.cf
6. Prencipe, M, Ferretti, C, Casini, AR, Santini, M, Giubilei, F, and Culasso, F. Stroke, disability, and dementia. Stroke. (1997) 28:531–6. doi: 10.1161/01.STR.28.3.531
7. Eng, JJ, and Tang, PF. Gait training strategies to optimize walking ability in people with stroke: a synthesis of the evidence. Expert Rev Neurother. (2007) 7:1417–36. doi: 10.1586/14737175.7.10.1417
8. Mayo, NE, Wood-Dauphinee, S, Cote, R, Durcan, L, and Carlton, J. Activity, participation, and quality of life 6 months Poststroke. Arch Phys Med Rehabil. (2002) 83:1035–42. doi: 10.1053/apmr.2002.33984
9. Opara, JA, and Jaracz, K. Quality of life of post-stroke patients and their caregivers. J Med Life. (2010) 3:216–20.
10. Harris, JE, and Eng, JJ. Goal priorities identified through client-centred measurement in individuals with chronic stroke. Physiother Can. (2004) 56:171–6. doi: 10.2310/6640.2004.00017
11. Bohannon, RW, Andrews, AW, and Smith, MB. Rehabilitation goals of patients with hemiplegia. Int J Rehabil Res. (1988) 11:181–4. doi: 10.1097/00004356-198806000-00012
12. Li, S, Francisco, GE, and Zhou, P. Post-stroke hemiplegic gait: new perspective and insights. Front Physiol. (2018) 9:1021. doi: 10.3389/fphys.2018.01021
13. Jorgensen, HS, Nakayama, H, Raaschou, HO, and Olsen, TS. Recovery of walking function in stroke patients: the Copenhagen stroke study. Arch Phys Med Rehabil. (1995) 76:27–32. doi: 10.1016/s0003-9993(95)80038-7
14. States, RA, Pappas, E, and Salem, Y. Overground physical therapy gait training for chronic stroke patients with mobility deficits. Cochrane Database Syst Rev. (2009) 2009:CD006075. doi: 10.1002/14651858.CD006075.pub2
15. Reisman, DS, Binder-MacLeod, S, and Farquhar, WB. Changes in metabolic cost of transport following locomotor training Poststroke. Top Stroke Rehabil. (2013) 20:161–70. doi: 10.1310/tsr2002-161
16. Olney, SJ, Monga, TN, and Costigan, PA. Mechanical energy of walking of stroke patients. Arch Phys Med Rehabil. (1986) 67:92–8. doi: 10.1016/0003-9993(86)90109-7
17. Tunç, H, Oken, O, Kara, M, Tiftik, T, Doğu, B, Unlü, Z, et al. Ultrasonographic measurement of the femoral cartilage thickness in Hemiparetic patients after stroke. Int J Rehabil Res. (2012) 35:203–7. doi: 10.1097/MRR.0b013e3283532736
18. Aderibigbe, A, Famurewa, O, Komolafe, M, Omisore, A, and Adetiloye, V. Sonographic soft tissue arthritic changes associated with post-stroke hemiplegic knee pain: utility of musculoskeletal ultrasound in a resource-limited setting. Pol J Radiol. (2020) 85:45–52. doi: 10.5114/pjr.2020.93149
19. Batchelor, FA, Mackintosh, SF, Said, CM, and Hill, KD. Falls after Stroke. Int J Stroke. (2012) 7:482–90. doi: 10.1111/j.1747-4949.2012.00796.x
20. Kanis, J, Oden, A, and Johnell, O. Acute and long-term increase in fracture risk after hospitalization for stroke. Stroke. (2001) 32:702–6. doi: 10.1161/01.str.32.3.702
21. Liu, T-W, Ng Gabriel, YF, Chung Raymond, CK, and Ng Shamay, SM. Decreasing fear of falling in chronic stroke survivors through cognitive behavior therapy and task-oriented training. Stroke. (2019) 50:148–54. doi: 10.1161/STROKEAHA.118.022406
22. Wonsetler, EC, and Bowden, MG. A systematic review of mechanisms of gait speed change post-stroke. Part 2: exercise capacity, muscle activation, kinetics, and kinematics. Top Stroke Rehabil. (2017) 24:394–403. doi: 10.1080/10749357.2017.1282413
23. Fritz, S, and Lusardi, M. White paper: "walking speed: the sixth vital sign". J Geriatr Phys Ther. (2009) 32:46–9.
24. Studenski, S, Perera, S, Patel, K, Rosano, C, Faulkner, K, Inzitari, M, et al. Gait speed and survival in older adults. JAMA. (2011) 305:50–8. doi: 10.1001/jama.2010.1923
25. Middleton, A, Fritz, SL, and Lusardi, M. Walking speed: the functional vital sign. J Aging Phys Act. (2015) 23:314–22. doi: 10.1123/japa.2013-0236
26. Perry, J, Garrett, M, Gronley, JK, and Mulroy, SJ. Classification of walking handicap in the stroke population. Stroke. (1995) 26:982–9. doi: 10.1161/01.STR.26.6.982
27. Fulk, GD, He, Y, Boyne, P, and Dunning, K. Predicting home and community walking activity Poststroke. Stroke. (2017) 48:406–11. doi: 10.1161/STROKEAHA.116.015309
28. Bohannon, RW. Measurement of gait speed of older adults is feasible and informative in a home-care setting. J Geriatr Phys Ther. (2009) 32:22–3. doi: 10.1519/00139143-200932010-00005
29. Sullivan, JE, Crowner, BE, Kluding, PM, Nichols, D, Rose, DK, Yoshida, R, et al. Outcome measures for individuals with stroke: process and recommendations from the American Physical Therapy Association neurology section task force. Phys Ther. (2013) 93:1383–96. doi: 10.2522/ptj.20120492
30. Moore, JL, Potter, K, Blankshain, K, Kaplan, SL, Oʼdwyer, LC, and Sullivan, JE. A Core set of outcome measures for adults with neurologic conditions undergoing rehabilitation: a clinical practice guideline. J Neurol Phys Ther. (2018) 42:174–220. doi: 10.1097/NPT.0000000000000229
31. Reed, KB, and Handzic, Ismet, inventor; University of South Florida, assignee. Gait altering shoes. USA patent 9,295,302 (2016).
32. Handzic, I, Barno, EM, Vasudevan, EV, and Reed, KB. Design and pilot study of a gait enhancing mobile shoe. Paladyn. (2011) 2:193–201. doi: 10.2478/s13230-012-0010-7
33. Handzic, I, Vasudevan, E, and Reed, KB. Developing a gait enhancing mobile shoe to alter over-ground walking coordination. IEEE Int Conf Robot Autom. (2012) 2012:4124–9. doi: 10.1109/ICRA.2012.6225346
34. Handzzic, I, and Reed, KB. Kinetic shapes: analysis, verification, and applications. J Mech Des N Y. (2014) 136:0610051–8. doi: 10.1115/1.4027168
35. Dzewaltowski, AC, Hedrick, EA, Leutzinger, TJ, Remski, LE, and Rosen, AB. The effect of Split-Belt treadmill interventions on step length asymmetry in individuals Poststroke: a systematic review with Meta-analysis. Neurorehabil Neural Repair. (2021) 35:563–75. doi: 10.1177/15459683211011226
36. Handzic, I, and Reed, KB. Comparison of the passive dynamics of walking on ground, tied-belt and split-belt treadmills, and via the gait enhancing mobile shoe (gems). IEEE Int Conf Rehabil Robot. (2013) 2013:6650509. doi: 10.1109/ICORR.2013.6650509
37. dos Anjos, S, Morris, D, and Taub, E. Constraint-induced movement therapy for lower extremity function: describing the Le-Cimt protocol. Phys Ther. (2020) 100:698–707. doi: 10.1093/ptj/pzz191
38. Kim, SH, Huizenga, DE, Handzic, I, Ditwiler, RE, Lazinski, M, Ramakrishnan, T, et al. Relearning functional and symmetric walking after stroke using a wearable device: a feasibility study. J Neuroeng Rehabil. (2019) 16:106. doi: 10.1186/s12984-019-0569-x
39. Huizenga, D, Rashford, L, Darcy, B, Lundin, E, Medas, R, Shultz, ST, et al. Wearable gait device for stroke gait rehabilitation at home. Top Stroke Rehabil. (2020) 28:443–55. doi: 10.1080/10749357.2020.1834272
40. Tilson, JK, Sullivan, KJ, Cen, SY, Rose, DK, Koradia, CH, Azen, SP, et al. Meaningful gait speed improvement during the first 60 days Poststroke: minimal clinically important difference. Phys Ther. (2010) 90:196–208. doi: 10.2522/ptj.20090079
41. Darcy, B, Rashford, L, Shultz, ST, DuBose, E, and Reed, KBR. Home use gait device for treatment of post-stroke hemiparetic gait impairments – 12-month follow-up results [abstract]. PMR. 2020; 12:(suppl 1). Available at: https://pmrjabstracts.org/abstract/home-use-gait-device-for-treatment-of-post-stroke-hemiparetic-gait-impairments-12-month-follow-up-results/. (Accessed December 13, 2023).
42. Huizenga, D, Rashford, L, Darcy, B, Lundin, E, Medas, R, Shultz, S, et al. Home use gait device for stroke hemiparesis: long-term follow-up results. Arch Phys Med Rehabil. (2020) 101:e68. doi: 10.1016/j.apmr.2020.09.206
43. Dickstein, R. Rehabilitation of gait speed after stroke: a critical review of intervention approaches. Neurorehabil Neural Repair. (2008) 22:649–60. doi: 10.1177/15459683080220060201
44. DePaul, VG, Wishart, LR, Richardson, J, Thabane, L, Ma, J, and Lee, TD. Varied Overground walking training versus body-weight-supported treadmill training in adults within 1 year of stroke: a randomized controlled trial. Neurorehabil Neural Repair. (2015) 29:329–40. doi: 10.1177/1545968314546135
45. Park, I-m, Lee, Y-s, Moon, B-m, and Sim, S-m. A comparison of the effects of overground gait training and treadmill gait training according to stroke patients’ gait velocity. J Phys Ther Sci. (2013) 25:379–82. doi: 10.1589/jpts.25.379
46. Reisman, DS, Wityk, R, Silver, K, and Bastian, AJ. Split-belt treadmill adaptation transfers to overground walking in persons poststroke. Neurorehabil Neural Repair. (2009) 23:735–44. doi: 10.1177/1545968309332880
47. Torres-Oviedo, G, and Bastian, AJ. Seeing is believing: effects of visual contextual cues on learning and transfer of locomotor adaptation. J Neurosci. (2010) 30:17015–22. doi: 10.1523/JNEUROSCI.4205-10.2010
48. Park, SH, Yan, S, Dee, W, Reed, R, Roth, EJ, Rymer, WZ, et al. Repeated adaptation and De-adaptation to the pelvis resistance force facilitate retention of motor learning in stroke survivors. J Neurophysiol. (2022) 127:1642–54. doi: 10.1152/jn.00046.2022
49. Langhorne, P, Taylor, G, Murray, G, Dennis, M, Anderson, C, Bautz-Holter, E, et al. Early supported discharge services for stroke patients: a meta-analysis of individual patients' data. Lancet. (2005) 365:501–6. doi: 10.1016/s0140-6736(05)17868-4
50. Duncan, PW, Sullivan, KJ, Behrman, AL, Azen, SP, Wu, SS, Nadeau, SE, et al. Body-weight-supported treadmill rehabilitation after stroke. N Engl J Med. (2011) 364:2026–36. doi: 10.1056/NEJMoa1010790
51. Mayo, NE. Stroke rehabilitation at home. Stroke. (2016) 47:1685–91. doi: 10.1161/STROKEAHA.116.011309
52. Wang, C, Winstein, C, D'Argenio, DZ, and Schweighofer, N. The efficiency, efficacy, and retention of task practice in chronic stroke. Neurorehabil Neural Repair. (2020) 34:881–90. doi: 10.1177/1545968320948609
53. Langhammer, B, and Stanghelle, JK. Exercise on a treadmill or walking outdoors? A randomized controlled trial comparing effectiveness of two walking exercise programmes late after stroke. Clin Rehabil. (2009) 24:46–54. doi: 10.1177/0269215509343328
54. Ada, L, Dean, CM, and Lindley, R. Randomized trial of treadmill training to improve walking in community-dwelling people after stroke: the ambulate trial. Int J Stroke. (2013) 8:436–44. doi: 10.1111/j.1747-4949.2012.00934.x
Keywords: gait device, gait speed, iStride, stroke rehabilitation, walking speed, retention
Citation: Darcy B, Rashford L, Tsai NT, Huizenga D, Reed KB and Bamberg SJM (2024) One-year retention of gait speed improvement in stroke survivors after treatment with a wearable home-use gait device. Front. Neurol. 14:1089083. doi: 10.3389/fneur.2023.1089083
Edited by:
Giorgio Sandrini, Fondazione Cirna Onlus, ItalyReviewed by:
Dorian Kay Rose, University of Florida, United StatesMukul Mukherjee, University of Nebraska Omaha, United States
Copyright © 2024 Darcy, Rashford, Tsai, Huizenga, Reed and Bamberg. This is an open-access article distributed under the terms of the Creative Commons Attribution License (CC BY). The use, distribution or reproduction in other forums is permitted, provided the original author(s) and the copyright owner(s) are credited and that the original publication in this journal is cited, in accordance with accepted academic practice. No use, distribution or reproduction is permitted which does not comply with these terms.
*Correspondence: Brianne Darcy, brie@moterum.com