- 1Department of Neurology, Royal Brisbane and Women's Hospital, Herston, QLD, Australia
- 2Kids Research, Kids Neuroscience Centre, The Children's Hospital at Westmead, Sydney, NSW, Australia
- 3Discipline of Child and Adolescent Health, Faculty of Medicine and Health, The University of Sydney, Sydney, NSW, Australia
- 4School of Biotechnology and Biomolecular Sciences, University of New South Wales, Sydney, NSW, Australia
- 5Institute for Molecular Biosciences, The University of Queensland, St Lucia, QLD, Australia
- 6Department of Pathology, Royal Brisbane and Women's Hospital, Herston, QLD, Australia
- 7School of Biomedical Sciences, The University of Queensland, St Lucia, QLD, Australia
- 8The Children's Medical Research Institute, Sydney, NSW, Australia
- 9Centre for Clinical Research, The University of Queensland, Herston, QLD, Australia
Recessive pathogenic variants in the laminin subunit alpha 2 (LAMA2) gene cause a spectrum of disease ranging from severe congenital muscular dystrophy to later-onset limb girdle muscular dystrophy (LGMDR23). The phenotype of LGMDR23 is characterized by slowly progressive proximal limb weakness, contractures, raised creatine kinase, and sometimes distinctive cerebral white matter changes and/or epilepsy. We present two siblings, born to consanguineous parents, who developed adult-onset LGMDR23 associated with typical cerebral white matter changes and who both later developed dementia. The male proband also had epilepsy and upper motor neuron signs when he presented at age 72. Merosin immunohistochemistry and Western blot on muscle biopsies taken from both subjects was normal. Whole exome sequencing revealed a previously unreported homozygous missense variant in LAMA2 [Chr6(GRCh38):g.129297734G>A; NM_000426.3:c.2906G>A; p.(Cys969Tyr)] in the proband. The same homozygous LAMA2 variant was confirmed by Sanger sequencing in the proband's affected sister. These findings expand the genotypic and phenotypic spectrum of LGMDR23.
Introduction
Laminin subunit alpha 2 (LAMA2) encodes the α2 subunit of merosin (laminin-2), a glycoprotein critical for linking the sarcolemma to the extracellular matrix (1). Recessive pathogenic LAMA2 variants cause a wide spectrum of dystrophic muscle disease ranging from severe congenital muscular dystrophy to later-onset limb girdle muscular dystrophy (LGMDR23), which presents after 12 months of age (2). LGDMR23 is a rare form of LGMD, accounting for only about 2% of all patients in Western European LGMD cohorts (3, 4). Most cases of LGMDR23 are caused by missense variants that result in partial merosin deficiency (2).
LGMDR23 typically presents in childhood with slowly progressive proximal limb weakness, contractures and raised creatine kinase (CK) (2). Motor milestones can be delayed, though nearly all patients achieve independent ambulation, which is typically maintained throughout life (2). Central nervous system (CNS) complications feature prominently in LGMDR23, with distinctive cerebral white matter signal changes reported in almost all cases and epilepsy in some (2).
Here we describe a proband aged 72 years who presented in his early thirties with LGMD and progressed to develop severe dementia, which began manifesting in his mid-sixties, and diffuse upper motor neuron (UMN) signs without a clear alternative explanation for these features. His sister also had a history of LGMD followed by the development of dementia in her late fifties. Whole exome sequencing (WES) in the proband identified a previously unreported homozygous missense variant in LAMA2 [Chr6(GRCh38):g. 129297734G>A; NM_000426.3:c.2906G>A; p.(Cys969Tyr)]. The same homozygous LAMA2 variant was confirmed by Sanger sequencing in the proband's affected sister. Although cerebral white matter changes are common, UMN findings and severe cognitive impairment have not previously been reported in association with LGMDR23.
Methods
Ethics
Ethical approval for use of muscle tissue and DNA from probands and controls were obtained from the Human Research Ethics Committees of the Children's Hospital at Westmead, Australia (2019/ETH11736) and all study participants (or their guardians) provided informed, written consent.
Genetic testing
Whole exome sequencing was performed for the proband as part of a previous study, where the proband remained undiagnosed (5). The WES data was reanalysed in the present study. Variant filtering and analysis was performed in seqr (6) using a minor allele frequency cut-off of 0.001 from the gnomAD database (7) and genes associated with neuromuscular disorders in publicly available gene lists (8) (Supplementary Table 1). Sanger sequencing was performed to confirm the LAMA2 variant in the proband and his affected sister, using the primers; LAMA2ex21F: 5'-agttcctctgttcccacctg-3' and LAMA2ex21R: 5'-cgttgtatcaatctgtgcttcc-3.'The chromosomal microarray (CMA) was performed using the Illumina Whole-Genome Infinium CytoSNP 850K Array v1.1. Results were analyzed with BlueFuse Multiversion 4.4, using genome reference sequence GRCh37/hg19.
Muscle immunohistochemistry and western blot
Cryopreserved muscle tissue was sectioned at 8 μm thickness and collected in pre-cooled microcentrifuge tubes for western blot or on microscopic slides (SuperFrost®Plus, Menzel, Germany) for immunostaining.
Immunostaining was performed on non-fixed tissue as described previously (9) using α-merosin (laminin α2) antibodies (MAb1922, Chemicon, 1:20000; NCL-Merosin, Novocastra, 1:200) and α-spectrin antibody (NCL-SPEC1, Novocastra, 1:300). Slides were imaged on a Leica DMi8 microscope and figures prepared using Adobe Illustrator.
Protein sample preparation and western blot was performed as previously described (10). Protein samples were separated on a 4–12% 1 mm NuPAGE™ Bis-Tris gel (Life Technologies) using MOPS running buffer at 150 V and transferred onto a PVDF membrane (Merck Millipore Immobilon™-P, 0.45 μm) for 1 h at 400 mA in Tris-Glycine buffer containing 0.075% sodium dodecyl sulfate and 15% methanol. Total protein was detected using Revert™ 700 total protein stain (LI-COR Biosciences) according to manufacturer's instructions.
Membranes were cut at the 115kD marker band and probed with an α-merosin antibody (bottom half, MAB1922, Chemicon, 1:500) and α-dystrophin antibody (top half, NCL-DYS3, Novocastra, 1:100) overnight at 4°C. Antibody binding was detected using an α-mouse HRP secondary antibody (Merck, 1:1000 for DYS3 detection and 1:2000 for merosin detection) for 2 h at room-temperature. Blots were imaged using the Odyssey CLx imaging system (LI-COR Biosciences) and analyzed using ImageJ. Merosin protein levels were normalized to actin (from total protein stain) and dystrophin. Dystrophin protein levels were also normalized to total protein. The average of 2–3 experiments for each sample was plotted on the graph.
Case report
The male proband (IV-1) was the first child of consanguineous first cousin parents (III-1 and III-2) (Figure 1). He had three sisters (IV-2, IV-3 and IV-4). He had a history of normal childhood motor and language development and was University educated. He presented at age 36 years with a several year history of frequent falls, initially attributed to pelvic fractures sustained from a previous mining accident. He experienced slowly progressive limb weakness with elevated CK (400–600 U/L; normal range <171 U/L). Cognitive issues manifested in his mid-60 s, with family members reporting poor decision making, reduced insight and memory problems. There were no visual hallucinations. Progressive cognitive impairment and severe weakness necessitated high-level nursing home care. Tonic-clonic epileptic seizures, without clear focal onset, began in his late 60 s. Magnetic resonance imaging (MRI) of the brain aged 64 showed confluent, bilateral white matter T2 hyperintensity with involvement of the corpus callosum (Figure 2). There was no significant cerebral or hippocampal atrophy. At around 70 years of age the proband became dependent upon the use of a wheelchair, non-verbal and incontinent.
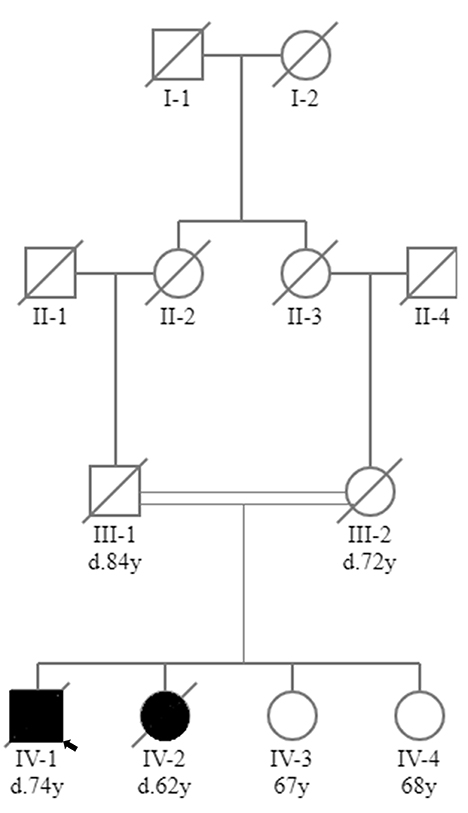
Figure 1. Family tree. Family tree showing that the proband (IV-1) and his affected sister (IV-2) were born to consanguineous first cousin parents (III-1 and III-2). Two sisters in their 60s were unaffected (IV-3 and IV-4). Square, male; circle, female; black circle or square, clinically affected; white circle or square, clinically unaffected; line through circle or square, deceased (age of death, where known, given below circle or square as denoted by d.); double line, consanguinity; small black arrow, proband.
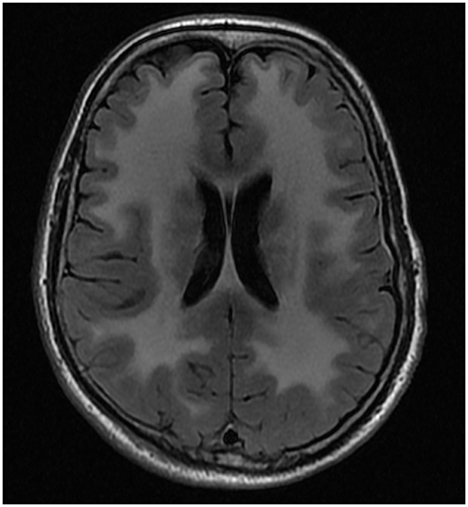
Figure 2. MRI brain of proband. Axial FLAIR* image from MRI brain of proband at 64 years of age showing confluent, bilateral white matter T2# hyper intensity with involvement of the corpus callosum *FLAIR, Fluid-attenuated inversion recovery; #T2, transverse relaxation time.
The proband had a history of well-controlled type II diabetes mellitus, hypothyroidism, depression, paroxysmal atrial fibrillation, and recurrent squamous cell carcinomas. His regular medications at age 72 included sodium valproate 600 mg twice daily, aspirin 100 mg daily, thyroxine 50 mcg 5/7 days and 100 mcg 2/7 days, metformin 500 mg daily, vitamin D 25 mcg daily, insulin glargine 25 units subcutaneously daily, Movicol sachet daily, docusate sodium with senna two tablets twice a day, paracetamol 500 mg two tablets four times a day and risperidone 2 mg as needed.
Examination at the age of 72 showed that he could only follow basic one-step commands and was non-verbal precluding more formal cognitive testing. There was diffuse limb muscle wasting with fixed flexion contractures of the elbows, fingers, hips, and knees. There was Medical Research Council (MRC) grade ≤3 weakness of the proximal and distal limb muscles that could be tested. There was calf pseudohypertrophy. Limb tone was difficult to assess due to the presence of contractures, but pathologic clonus was elicited at the ankles. The reflexes were brisk, and the plantar responses were equivocal. Sensory examination was challenging but the patient appeared to feel sharp sensation in all four limbs. His pupils were equal and reactive to light. Detailed examination of eye movements was not possible due to his difficulty in following instructions and sustaining attention. No facial weakness was observed. The proband was unable to stand or walk.
There was no record of a previous electroencephalogram, nerve conduction study/electromyogram (EMG), transthoracic echocardiogram or respiratory function test. Given the degree of his disability and advanced dementia these were not pursued as the results would not have changed management. The proband continued to decline and poor control of his tonic-clonic seizures resulted in an escalation of his anti-seizure medications. The proband succumbed to aspiration pneumonia at the age of 74.
His younger sister (IV-2) died at the age of 62 from pneumonia in the setting of early onset dementia. She presented to another facility with slowly progressive limb weakness with elevated CK (400-650 U/L) and gait dysfunction from the age of 50. Poor decision making and impairments in working memory were noted in her late 50 s. A limited EMG of her biceps muscle showed myopathic changes characterized by small amplitude motor units without abnormal spontaneous activity. Neuroimaging showed extensive cerebral white matter disease (not shown). From the age of 59 there was a more rapid deterioration in her cognitive and physical function culminating in her being bedbound, mute, and doubly incontinent for the 12 months prior to her death. She did not have a history of epilepsy. The other two sisters in their 60 s (IV-3 and IV-4) were unaffected. There was no other family history of neuromuscular disease or dementia.
Genetic testing
Genetic testing, including targeted sequencing of FKRP, DNM2 and LARGE genes, failed to identify causative genetic variants. Whole exome sequencing, performed from blood of proband (IV-1) identified a previously unreported homozygous missense variant [NM_000426.3:c.2906G>A;p.(Cys969Tyr)] in exon 21 of LAMA2 (Table 1) [submitted to ClinVar–https://www.ncbi.nlm.nih.gov/clinvar/variation/1693116/?oq=LAMA2%5bgene%5+p.Cys969Tyr&m=NM_000426.4(LAMA2):c.2906G>A%20(p.Cys969Tyr)]. Sanger sequencing confirmed the LAMA2 variant was homozygous in the proband and his affected sister (IV-2). The two unaffected sisters (IV-3 and IV-4) were not available for genetic testing for segregation analysis. A chromosomal microarray detected multiple long contiguous stretches of homozyosity (LCSH), including the LAMA2 locus on chromosome 6.
Muscle histopathology
A quadriceps muscle biopsy obtained from the proband at age 56 years showed myopathic changes with marked replacement of muscle with adipose tissue (Figure 3Ai). The remaining muscle fibers showed marked variation in myofibre diameter with numerous severely atrophic fibers.
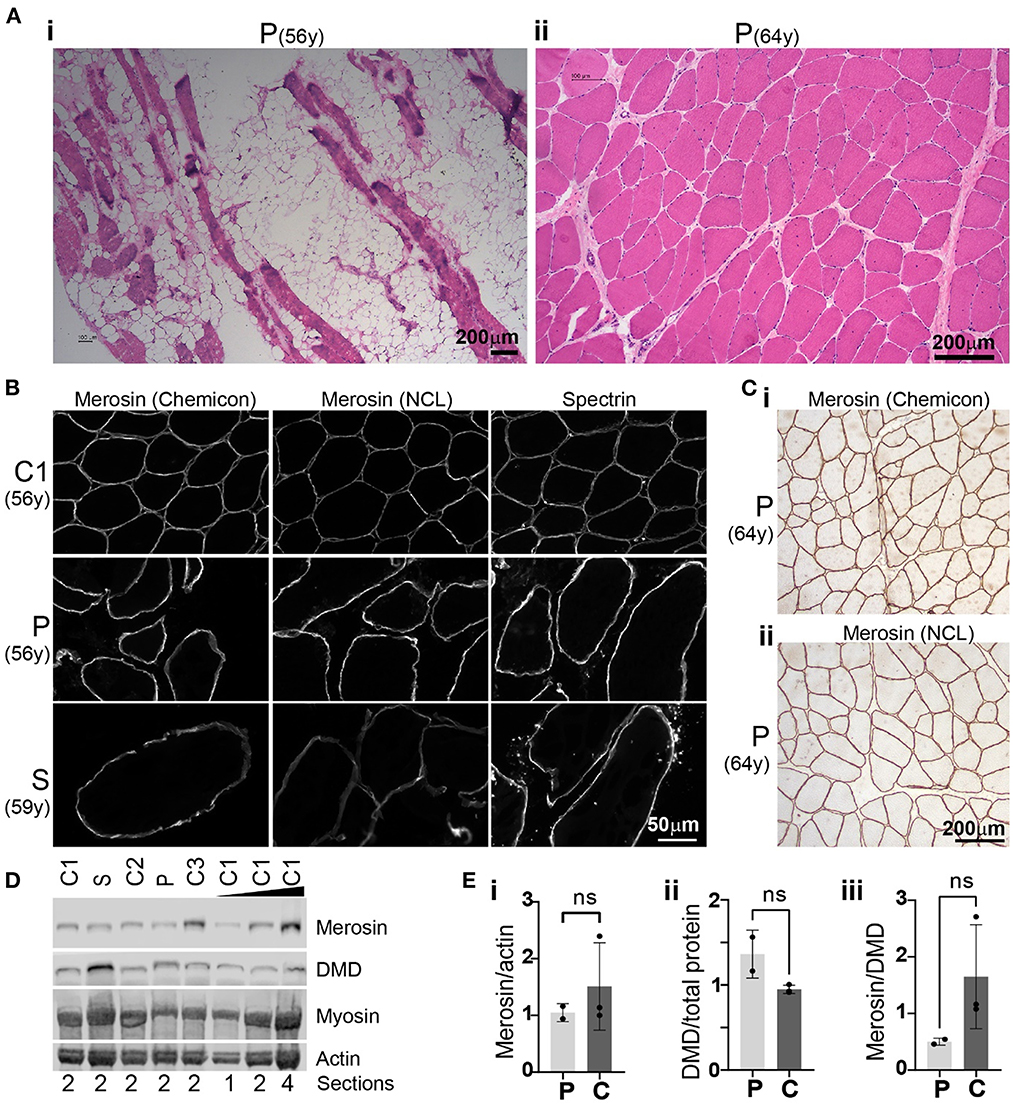
Figure 3. Histology, immunohistochemistry and Western blot analysis. (A) Haematoxylin and eosin (H&E) staining of muscle biopsies obtained from the proband (P) at age 56 years (i; quadriceps) and 64 years (ii; biceps). Unstained areas observed in (i) represent adipose tissue infiltrates while muscle fibers appear pink in H&E-stained sections. (B) Merosin immunohistochemical staining of proband (P) quadriceps muscle biopsies and a muscle biopsy obtained from the proband's sister (S) at 59 years demonstrating membrane-staining comparable to control 1 (C1). Immunostaining for spectrin, another membrane-associated protein, showed a staining pattern similar to merosin. (C) Immunohistochemical staining of proband biceps muscle obtained at age 64 years with two merosin antibodies (i: Chemicon MAb1922; ii: NCL-merosin Novocastra) demonstrated membrane-associated staining pattern of merosin similar to (B). (D) Western blot of muscle from the proband (P; 56y) and his sister (S; 59y) showed merosin protein levels similar to those detected in three control samples: C1 = 56y (female), C2 = 57y (female), C3 = 59y (male). (E) Bar graphs of merosin protein levels normalized to actin (measured from total protein stain) (i), dystrophin protein levels normalized to total protein (ii) and merosin protein levels normalized to dystrophin (iii). Average ± standard deviation for proband and sister (collectively denoted as P) and controls (C) are shown. Groups were compared by using a t-test and found to be not significantly different (ns, i; p = 0.4848, ii; 0.0734, iii; 0.1926).
A biceps muscle biopsy taken subsequently, aged 64 years, showed less severe myopathic changes compared to his quadriceps biopsy with increased internal nuclei, variation in myofibre diameters including hypertrophic and atrophic fibers, but no adipose tissue infiltration (Figure 3Aii). A quadriceps muscle biopsy of the proband's sister at age 59 years showed myopathic changes similar to the quadriceps muscle biopsy from her brother at the age of 56 with severe variation in myofibre diameter, numerous atrophic fibers and significant replacement of skeletal muscle with fibroadipose tissue (not shown).
To determine whether the homozygous missense variant in LAMA2 results in abnormal subcellular localization and abnormal protein levels of the encoded protein merosin, we performed immunohistochemistry and western blot analysis. Merosin immunohistochemistry on two biopsies from the proband (age 56 and 64 years) and one biopsy from the sister (age 59 years) demonstrated membrane-associated staining pattern comparable to control muscle (Figures 3B, C). Merosin protein levels as determined by Western blot were also comparable to controls (three age matched controls were used) in both subjects (Figure 3D). Merosin protein levels were found to be lower in the proband and sister compared to controls when normalized to actin and dystrophin but this difference was not statistically significant (Figure 3Ei, iii). There was a small increase in dystrophin protein levels when normalized to total protein in the proband and sister compared to controls but this difference was also not statistically significant (Figure 3Eii).
Discussion
The phenotypic and genotypic spectrum of LAMA2 muscular dystrophy is wide. Here we report a novel homozygous LAMA2 variant in two siblings born to consanguineous parents. The LAMA2 variant was not reported in several large reference population databases (7, 11, 12) and in silico analyses (13, 14) predicted the variant to have a deleterious impact on protein function (Table 1). The affected sister harbored the same variant in a homozygous state. Whilst genetic testing of the parents was not possible the LCSH on chromosomal microarray would support the novel variant occurring in trans due to known parental consanguinity. Collective clinical and genetic evidence supports classification of NM_000426.3:c.2906G>A;p.(Cys969Tyr) as likely pathogenic as per the ACMG-AMP criteria (15) (Table 1). A limitation of our report is the lack of in vitro or in vivo functional studies to assess for any damaging effect of this variant on the gene product. The variant identified in our study affects a highly conserved cysteine residue within the laminin-type epidermal growth factor (EGF)-like domain (16). Cysteines in this domain are critical for disulfide bond formation (16) and the variant is thus likely to affect the integrity of this rigid rod-like structure, which could destabilize the sarcolemma-extracellular matrix connections facilitated by the laminin-α2 protein (17). A study reporting 61 new LAMA2 variants and reviewing LAMA2 variants in the Leiden Open Variation Database (18) (https://www.LOVD.nl/LAMA2) found that 25% of deleterious missense variants affect cysteine residues within EGF-like repeats (17). A very rare missense variant [p.(Cys969Ser)] in the same amino acid position as our variant has been reported in the gnomAD database in the hetereozygous state without clinical disease (7).
The proband's clinical presentation was notable for profound weakness, severe joint contractures, extensive cerebral white matter changes, UMN findings, epilepsy, and dementia. Cerebral white matter changes are seen in nearly all patients with LAMA2 muscular dystrophy (1, 2). In patients with adult onset LGMDR23, epilepsy is uncommon and cognition is usually intact despite the striking white matter abnormalities on brain MRI (1, 2). Mild deficits in executive function and mild cognitive impairment have been reported in a few older patients with adult onset LGMDR23 (19, 20). More significant cognitive impairment, in the form of intellectual disability, has been reported in LGMDR23 but only in younger patients with childhood onset disease (20, 21). The dementia seen in the proband and his sister could represent an extension of the LGMDR23 phenotype. However, as they were born to consanguineous parents it is possible that the dementia is due to the inheritance of another, albeit unrecognized, recessive pathogenic variant, especially given the LCSH seen on chromosomal microarray.
The finding of brisk reflexes and pathologic ankle clonus in the proband implies co-existent UMN pathology. This could be related to the extensive white matter changes, but UMN signs have not been described in other patients with LAMA2 muscular dystrophy most of whom have similar white matter abnormalities on brain MRI.
The muscle biopsies from our subjects showed non-specific myopathic and dystrophic features consistent with the muscle biopsy findings in other LGMD23 cases (2, 4, 19, 20). However, most LGMD23 cases show at least partial merosin deficiency on immunohistochemistry and Western blot (2). Significant variability of merosin immunostaining in cases of LGMDR23 is well recognized and often depends on the antibodies used (22). However, the finding of normal merosin immunostaining, as seen in our subjects, is atypical. Normal merosin immunostaining is not unprecedented and has been described in two cases of genetically confirmed LGMDR23 using the same antibodies (MAb1922, Chemicon and NCL-merosin, Novocastra) (20, 23). Like our subjects, both cases had a late onset of symptoms with one case developing LGMD in their forties (20). In one of these cases, a subtle reduction of merosin immunostaining was only detected when the antibody directed against the N-terminal 300-kDa fragment of laminin-α2 (4H8, Alexis) was used (23). This differential immunostaining is consistent with the findings in other cases of LGMDR23 where staining with the N-terminal antibody often shows more abnormal results compared to staining with the antibody against the C-terminal 80-kDa fragment (MAb1922, Chemicon) (22). This could provide a possible explanation for the “normal” merosin immunostaining seen in our subjects as the N-terminal antibody was not used.
To our knowledge the findings of normal merosin Western blot in muscle despite clinical and genetic evidence of LAMA2 muscular dystrophy has not been described. It is possible that a small change in merosin protein levels is not detected by our Western blot. Merosin protein levels were lower in the proband and his sister compared to controls when normalized to actin and dystrophin but these differences were not statistically significant (Figure 3Ei, iii). It is possible that the LAMA2 variant [p.(Cys969Tyr)] could cause disease by conferring a toxic gain-of-function effect at the protein level, although this phenomenon would be extremely rare in the setting of a recessive disorder. Alternatively, the variant may reduce the activity of the laminin type EGF-like domain as postulated above but not significantly impact overall protein levels.
In the absence of published diagnostic criteria for LAMA2 muscular dystrophy the finding of rare predicted to be pathogenic recessive variants in the LAMA2 gene is currently considered to be the gold standard for establishing a diagnosis (1, 2). The presence of limb girdle muscle weakness, contractures, raised CK and cerebral white matter changes in the proband and his sister combined with likely pathogenic LAMA2 variants are highly suggestive of LGMDR23. In particular, the finding of cerebral white matter changes in both subjects provides a compelling argument that the recessive LAMA2 variants are the cause for their muscle weakness, as cerebral white matter changes appear to be unique to this sub-type of LGMD.
Conclusion
This case report expands the genotypic and phenotypic spectrum of LGMDR23 through the identification of a novel homozygous LAMA2 variant in a sibling pair with typical LGMD23 features including white matter signal changes–along with upper motor neuron findings and dementia. This finding highlights the utility of WES in aiding the diagnosis of rare neuromuscular disorders, particularly where the muscle biopsy results are inconclusive.
Data availability statement
The datasets presented in this study can be found in online repositories. The names of the repository/repositories and accession number(s) can be found in the article/ Supplementary material.
Ethics statement
The studies involving human participants were reviewed and approved by Human Research Ethics Committees of the Children's Hospital at Westmead, Australia (2019/ETH11736). The patients/participants provided their written informed consent to participate in this study.
Author contributions
MK was responsible for conception, organization, and writing of manuscript. LW and SB were responsible for obtaining initial muscle and genetic data and assisted with editing of the manuscript. MY assisted preparation of the methods section on muscle immunohistochemistry and western blot and provided Figure 3 on muscle histopathology results and helped edit the manuscript. FG assisted with Table 1 on genetic variant, submission of genetic variant to ClinVar database, and helped edit the manuscript. SC was responsible for supervising collection, interpretation of muscle and genetic data, and assisted with editing of the manuscript. EO, TR, RH, and PM helped with editing of the manuscript. All authors contributed to the article and approved the submitted version.
Acknowledgments
Acknowledgment to the Queensland Medical Laboratory (QML) for performing the chromosomal microarray and interpreting the results.
Conflict of interest
The authors declare that the research was conducted in the absence of any commercial or financial relationships that could be construed as a potential conflict of interest.
Publisher's note
All claims expressed in this article are solely those of the authors and do not necessarily represent those of their affiliated organizations, or those of the publisher, the editors and the reviewers. Any product that may be evaluated in this article, or claim that may be made by its manufacturer, is not guaranteed or endorsed by the publisher.
Supplementary material
The Supplementary Material for this article can be found online at: https://www.frontiersin.org/articles/10.3389/fneur.2023.1055639/full#supplementary-material
References
1. Oliveira J, Freixo JP, Santos M, Coelho T. LAMA2 Muscular Dystrophy. GeneReviews® (2012). Available online at: https://www.ncbi.nlm.nih.gov/books/NBK97333/
2. Sarkozy A, Foley AR, Zambon AA, Bönnemann CG, Muntoni F. LAMA2-related dystrophies: clinical phenotypes, disease biomarkers, and clinical trial readiness. Front Mol Neurosci. (2020) 13:1–11. doi: 10.3389/fnmol.2020.00123
3. Sframeli M, Sarkozy A, Bertoli M, Astrea G, Hudson J, Scoto M, et al. Congenital muscular dystrophies in the UK population: Clinical and molecular spectrum of a large cohort diagnosed over a 12-year period. Neuromuscul Disord. (2017) 27:793–803. doi: 10.1016/j.nmd.2017.06.008
4. Løkken N, Born AP, Duno M, Vissing J. LAMA2-related myopathy: Frequency among congenital and limb-girdle muscular dystrophies. Muscle and Nerve. (2015) 52:547–53. doi: 10.1002/mus.24588
5. Ghaoui R, Cooper ST, Lek M, Jones K, Corbett A, Reddel SW, et al. Use of whole-exome sequencing for diagnosis of limb-girdle muscular dystrophy: outcomes and lessons learned. JAMA Neurol. (2015) 72:1424–32. doi: 10.1001/jamaneurol.2015.2274
6. Pais L, Snow H, Weisburd S, B, Zhang Baxter S, Ditroia S, et al. seqr: a web-based analysis and collaboration tool for rare disease genomics. Hum Mutat. (2022) 43:698–707. doi: 10.1002/humu.24366
7. Karczewski KJ, Francioli LC, Tiao G, Cummings BB, Alföldi J, Wang Q, et al. The mutational constraint spectrum quantified from variation in 141,456 humans. Nature. (2020) 581:434–43. doi: 10.1038/s41586-020-2308-7
8. Cohen E, Bonne G, Rivier F, Hamroun D. The 2022 version of the gene table of neuromuscular disorders (nuclear genome). Neuromuscul Disord. (2021) 31:1313–57. doi: 10.1016/j.nmd.2021.11.004
9. Lo HP, Cooper ST, Evesson FJ, Seto JT, Chiotis M, Tay V, et al. Limb-girdle muscular dystrophy: Diagnostic evaluation, frequency and clues to pathogenesis. Neuromuscul Disord. (2008) 18:34–44. doi: 10.1016/j.nmd.2007.08.009
10. Cooper ST, Lo HP, North KN. Single section Western blot: Improving the molecular diagnosis of the muscular dystrophies. Neurology. (2003) 61:93–7. doi: 10.1212/01.WNL.0000069460.53438.38
11. Auton A, Abecasis GR, Altshuler DM, Durbin RM, Bentley DR, Chakravarti A, et al. A global reference for human genetic variation. Nature. (2015) 526:68–74. doi: 10.1038/nature15393
12. Lek M, Karczewski KJ, Minikel E V, Samocha KE, Banks E, Fennell T, et al. Analysis of protein-coding genetic variation in 60,706 humans. Nature. (2016) 536:285–91. doi: 10.1038/nature19057
13. Dong C, Wei P, Jian X, Gibbs R, Boerwinkle E, Wang K, et al. Comparison and integration of deleteriousness prediction methods for nonsynonymous SNVs in whole exome sequencing studies. Hum Mol Genet. (2015) 24:2125–37. doi: 10.1093/hmg/ddu733
14. Jagadeesh KA, Wenger AM, Berger MJ, Guturu H, Stenson PD, Cooper DN, et al. M-CAP eliminates a majority of variants of uncertain significance in clinical exomes at high sensitivity. Nat Genet. (2016) 48:1581–6. doi: 10.1038/ng.3703
15. Richards S, Aziz N, Bale S, Bick D, Das S, Gastier-Foster J, et al. Standards and guidelines for the interpretation of sequence variants: a joint consensus recommendation of the American College of Medical Genetics and Genomics and the Association for Molecular Pathology. Genet Med. (2015) 17:405–24. doi: 10.1038/gim.2015.30
16. Abe Y, Odaka M, Inagaki F, Lax I, Schlessinger J, Kohda D. Disulfide bond structure of human epidermal growth factor receptor. J Biol Chem. (1998) 273:11150–7. doi: 10.1074/jbc.273.18.11150
17. Oliveira J, Gruber A, Cardoso M, Taipa R, Fineza I, Gonçalves A, et al. LAMA2 gene mutation update: Toward a more comprehensive picture of the laminin-α2 variome and its related phenotypes. Hum Mutat. (2018) 39:1314–37. doi: 10.1002/humu.23599
18. Fokkema IFAC, Taschner PEM, Schaafsma GCP, Celli J, Laros JFJ, den Dunnen JT, et al. v. 20: The next generation in gene variant databases. Hum Mutat. (2011) 32:557–63. doi: 10.1002/humu.21438
19. Gavassini BF, Carboni N, Nielsen JøE, Danielsen ER, Thomsen C, Svenstrup K, et al. Clinical and molecular Characterization of limb-girdle muscular dystrophy due to LAMA2 mutations. Muscle and Nerve. (2011) 44:703–9. doi: 10.1002/mus.22132
20. Magri F, Brusa R, Bello L, Peverelli L, Del BR, Govoni A, et al. Limb girdle muscular dystrophy due to LAMA2 gene mutations: new mutations expand the clinical spectrum of a still challenging diagnosis. Acta Myol. (2020) 39:67–82. doi: 10.36185/2532-1900-009
21. Deodato F, Sabatelli M, Ricci E, Mercuri E, Muntoni F, Sewry C, et al. Hypermyelinating neuropathy, mental retardation and epilepsy in a case of merosin deficiency. Neuromuscul Disord. (2002) 12:392–8. doi: 10.1016/S0960-8966(01)00312-1
22. Jones KJ, Morgan G, Johnston H, Tobias V, Ouvrier RA, Wilkinson I, et al. The expanding phenotype of laminin α2 chain (merosin) abnormalities: case series and review. J Med Genet. (2001) 38:649–57. doi: 10.1136/jmg.38.10.649
Keywords: limb girdle muscular dystrophy, genotype, phenotype, LAMA2, whole exome sequencing
Citation: Katz M, Waddell LB, Yuen M, Bryen SJ, Oates E, Garton FC, Robertson T, Henderson RD, Cooper ST and McCombe PA (2023) Case report: Adult-onset limb girdle muscular dystrophy in sibling pair due to novel homozygous LAMA2 missense variant. Front. Neurol. 14:1055639. doi: 10.3389/fneur.2023.1055639
Received: 05 October 2022; Accepted: 12 January 2023;
Published: 27 January 2023.
Edited by:
Giovanni Meola, University of Milan, ItalyReviewed by:
Edoardo Malfatti, Hôpitaux Universitaires Henri Mondor, FranceAndrea Legati, IRCCS Carlo Besta Neurological Institute Foundation, Italy
Xin-Ming Shen, Mayo Clinic, United States
Kaihui Zhang, Fudan University, China
Copyright © 2023 Katz, Waddell, Yuen, Bryen, Oates, Garton, Robertson, Henderson, Cooper and McCombe. This is an open-access article distributed under the terms of the Creative Commons Attribution License (CC BY). The use, distribution or reproduction in other forums is permitted, provided the original author(s) and the copyright owner(s) are credited and that the original publication in this journal is cited, in accordance with accepted academic practice. No use, distribution or reproduction is permitted which does not comply with these terms.
*Correspondence: Matthew Katz, matthew.katz@health.qld.gov.au