- 1Department of Neurology, The First Affiliated Hospital of Zhengzhou University, Zhengzhou University, Zhengzhou, China
- 2The Academy of Medical Sciences of Zhengzhou University, Zhengzhou University, Zhengzhou, China
- 3Henan Key Laboratory of Cerebrovascular Diseases, The First Affiliated Hospital of Zhengzhou University, Zhengzhou University, Zhengzhou, China
- 4NHC Key Laboratory of Prevention and Treatment of Cerebrovascular Diseases, The First Affiliated Hospital of Zhengzhou University, Zhengzhou University, Zhengzhou, China
- 5Department of Neurology, Nanyang Central Hospital, Nanyang, China
- 6Institute of Neuroscience, Zhengzhou University, Zhengzhou, China
Background: Regarding the complexity of Parkinson's disease (PD), the identification of reliable biomarkers is of great significance for improving the accuracy of diagnosis and monitoring disease progression. Recently, some studies suggested that serum proline-rich protein 14 (PRR14), vascular cell adhesion molecule-1 (VCAM-1), and soluble CD163 (sCD163) factors may be associated with PD, even as potential biomarkers. However, the role of these serum factors is still unclear.
Objectives: This study aimed to explore the alterations of serum PRR14, VCAM-1, and sCD163 levels during PD progression, and their association with disease-related variables of PD.
Methods: We performed the assessment of scale tests and the detection of serum samples in patients with PD (n = 100) and healthy controls (HCs, n = 100). Furthermore, we investigated the association between serum factors and sex, cognitive impairments, H&Y (Hohn and Yahr), age at onset (AAO), and other variables in patients with PD.
Results: Patients with PD exhibited increased PRR14 and VCAM-1 serum levels compared with HCs. No significant differences were found in serum levels of sCD163. Subgroup analysis uncovered increased VCAM-1 in the female and male subgroups (PD and HCs). Among patients with PD, decreased PRR14 and increased VCAM-1 were associated with severer cognitive impairments and severer PD (H&Y), respectively. Bivariate correlation analysis revealed that there was a positive correlation between VCAM-1 and AAO.
Conclusions: Increased serum levels of PRR14 and VCAM-1 suggest that inflammation and defective autophagy may play vital roles in the pathogenesis of PD. However, the potential mechanisms remain to be elucidated.
Introduction
Parkinson's disease (PD) is a common neurodegenerative disorder characterized by the degeneration of the nigral dopaminergic (DA) neurons and clumped Lewy bodies. Patients with PD pathologically manifest aggregated α-synuclein (α-syn) in the central nervous system (CNS) and peripheral autonomic nervous system (1, 2). It was difficult to precisely diagnose and discriminate against PD owing to its similar clinical performance to other common neurodegenerative diseases (3). Considerable efforts have been made to identify reliable biomarkers for serum samples. Notably, previous studies had found that alterations in serum proline-rich protein 14 (PRR14), vascular cell adhesion molecule-1(VCAM-1), and soluble CD163 (sCD163) levels, were probably linked with the constipation status (4), the severity of disease (5), the cognition levels (6), and so on in patients with PD. Moreover, PRR14 was linked with the protection of DA neurons and the clearance of aggregated α-syn (4, 7), and the ongoing neuroinflammatory processes were correlated with the VCAM-1 and sCD163 in PD (5, 6). However, there is a lack of reliable evidence regarding whether their serum levels can sensitively discriminate PD from healthy controls (HCs).
A compelling etiology of PD was that impaired autophagy could not dump the aggregated α-syn in the brain sites, accelerating the deterioration of PD (8). In PD, the mammalian target of rapamycin (mTOR) is a typical autophagy-related signal pathway. Furthermore, increased mTOR protein expression levels were associated with the aggregation of α-syn by inhibiting autophagy in patients with PD (9, 10). In previous studies, PRR14 was regarded as an activator of the mTOR signal and was also used as a potential biomarker for the diagnosis of PD (4, 11). PRR14 is increased in the cerebrospinal fluid (CSF), serum, and plasma samples of patients with PD and animal models of PD (4, 7, 11, 12). Elevated serum PRR14 levels can inhibit autophagy and the clearance of α-syn in PD (10). Conversely, high serum PRR14 levels can also protect against the loss of DA neurons in PD (4). Among patients with PD, high serum PRR14 levels increased the risk of constipation (4). However, the study for serum PRR14 levels was sparse, and there is no other research to provide a profound understanding of the mechanisms of PRR14.
Another etiology of PD is the activation of microglia-induced neuroinflammation, which brings out the aggregated α-syn and defective nigral DA neurons in the CNS (13, 14). VCAM-1, as a distinct hint of the monocyte activation signal, impaired the blood–brain barrier (BBB) function and promoted neuroinflammation and loss of DA neurons (7). The latest in vitro studies found that genetic ablation of VCAM-1 can ameliorate neuroinflammation (15). However, decreased VCAM-1 was found in the 6-hydroxydopamine (6-OHDA) animal models of PD (16). For patients with PD, higher serum VCAM-1 level was correlated with worsening motor symptoms and disease severity, which provides further evidence to monitor PD progression (5). In addition, as another indicator of monocyte activation, shedding of sCD163 enhances the α-syn uptake, and α-syn in turn induces sCD163 shedding by activating monocytes (6). Noteworthy, increased CD163 in the brain tissues was found in 1-methyl-4-phenyl-1,2,3,6-tetrahydropyridine-induced (MPTP) animal models of PD (17), while Calvello et al. found the converse results (18). For patients with PD, sCD163 in CSF and serum positively correlated with CSF levels of α-syn and cognitive deficits, which supported that sCD163 plays a vital role in PD (6). However, rare studies showed whether VCAM-1 and sCD163 were associated with phenotypic related variables in PD, and their alterations of them were still discrepant in clinical samples (6, 7).
In this study, we identified alterations in serum PRR14 and VCAM-1 levels of patients with PD. Furthermore, we investigated the associations between altered factors and age at onset (AAO), Hohn and Yahr (H&Y), sex, cognitive impairments, and other variables to provide further insights into the pathogenesis of PD.
Patients and methods
Patient recruitment
This study randomly enrolled 100 patients with sporadic PD and 100 age- and sex-matched healthy individuals. Patients with PD were clinically diagnosed according to the Movement Disorders Society Clinical Diagnostic Criteria for Parkinson's disease (19). Patients with PD were recruited from the outpatient clinic and ward in the Department of Neurology, The First Affiliated Hospital of Zhengzhou University from 2018 to 2021. Simultaneously, healthy control subjects were randomly recruited from community-dwelling individuals, who are without any evidence of neurodegenerative disease or parkinsonian symptoms. Specialized neurologists assessed all participants. For patients with PD, participants were excluded with the initial diagnosis of PD Dementia (PDD) and dementia with Lewy body (DLB) according to the recommended standards by the Movement Disorder Society (20, 21). Patients who were taking corticosteroids, non-steroidal anti-inflammatory drugs (NSAIDs), or had been diagnosed with other disorders, such as tumors, infectious diseases, cardiovascular disease, diabetes mellitus, or systemic autoimmune diseases, were excluded from this study. This study involving human participants was reviewed and approved by the Medical Research Ethics Committee of the First Affiliated Hospital of Zhengzhou University. Written informed consent was obtained from all participants before their inclusion in this study.
Clinical assessments
We collected data on the demographic and clinical characteristics of patients with PD, including age, sex, age at PD onset, duration of PD, and level of education. The patients were evaluated to assess non-motor and motor symptoms during their “off state” using the Movement Disorder Society-Unified Parkinson's Disease Rating Scale (MDS-UPDRS). Simultaneously, the severity and stage of PD were obtained from the modified H&Y staging scale, and patients with PD were divided according to previous studies (22, 23). In detail, patients with PD with H&Y stage 1.0–2.0 were recognized as mild groups. H&Y stage 2.5–3.0 were divided into moderate groups, and H&Y stage 4.0–5.0 were severe groups. All participants underwent Mini-Mental State Examination (MMSE) assessment, accompanied by their level of education, to discern cognitive impairment (24). In detail, the established MMSE cutoff scores for the cognitive impairment of the participants are <17 for illiterate, <20 for individuals with 1–6 years of education, and <24 for individuals with 7 or more years of education (24). The cut-off values of disease duration and AAO were set according to the mean to distinguish discrete subgroups. The treatment of antiparkinsonian medication was also recorded. Simultaneously, levodopa-equivalent daily doses (LEDDs) were calculated as previously described (25). In particular, owing to the bad prognosis of the postural instability/gait difficulty (PIGD) group (26), we distinguished patients with PD with distinct clinical phenotypes, such as tremor dominant (TD), PIGD, and indeterminate (ID) phenotypes.
Measurement of factors in the serum
In the morning, venous blood samples were collected after overnight fasting using vacuum tubes, which minimizes confounding factors, such as diet and circadian rhythm. The samples were centrifuged at 1,000 g for 10 min at 4°C, and serum was collected and stored at –80°C for subsequent analysis. Specifically, serum levels of PRR14 (Jianglaibio, China) were detected using an Enzyme-linked Immunosorbent Assay (ELISA) kit, in accordance with the manufacturer's instructions. In addition, we used the Magnetic Luminex Assay-Human Premixed Multi-Analyte kit to determine the circulating levels of VCAM-1 and sCD163 according to the manufacturer's instructions (R&D Systems, Minneapolis, MN, USA). The minimum detectable dose of PRR14 was 2.5 ng/ml, VCAM-1 was 2,819.6 pg/ml, and sCD163 was 1,874.8 pg/ml.
Statistical analysis
All data were analyzed using the SPSS software (version 21.0; Armonk, NY, USA). Individual characteristics are displayed as the mean ± standard deviation (SD) for normally distributed variables and as the median along with the interquartile range for variables with skewed distributions. Fisher's t-test was used to compare binary variables. For continuous variables, the Shapiro–Wilk test was used to test the normality of the sample distribution. The two groups (patients with PD vs. HCs) were compared using the Mann–Whitney U-test or Student's t-test when the statistics were non-normally and normally distributed, respectively. Multiple group comparisons were used the Kruskal–Wallis non-parametric test and one-way ANOVA for non-parametric and parametric distributions, respectively. Of note, the post-hoc test (LSD) test or Kruskal–Wallis test was used for pairwise comparisons after comparisons of multiple groups. Bivariate correlations using Spearman's correlation analysis were performed to examine the relationship between serum factors and disease-related variables. All statistical tests were two-tailed, and the threshold for statistical significance was set at P < 0.05. Analyses were performed using the GraphPad Prism software (version 8.0.2; San Diego, CA, USA).
Results
Demographic and clinical characteristics
A total of 200 participants were enrolled in the study, including patients with PD (n = 100) and HCs (n = 100). Basic information about the participants in both groups is summarized in Table 1. No significant differences in age or sex were observed between the patient and the control groups. Among the 100 patients with PD, men occupied the major position (female/male =37/63), along with a mean age of 59.45 ± 8.58 (mean ± SD) years. The mean duration of illness was 4.44 ± 3.17 years, and the mean AAO was 54.76 ± 9.03 years. For PD participants, the UPDRS III and MMSE scores were 39.31 ± 19.16 (7–116) (mean ± SD (range) and 24.30 ± 5.50 (6–30), respectively. The years of education in patients with PD were 9.46 ± 4.44 (0–16). The percentage of individuals taking antiparkinsonian medication was 80% in patients with PD. Among the medicated patients, the percentage of using L-dopamine and dopamine agonists was 86.25 and 48.75%, respectively. Especially, the LEDDs were 381.70 ± 339.99 (0–2,060), along with the denoted H&Y stage was 2.10 ± 0.85 (1–5).
Altered factors in the serum
For 200 participants, significant results showed that higher serum PRR14 levels (37.82 vs. 30.26 ng/ml, P = 0.009; Figure 1A; Table 2) and VCAM-1 levels (1,098,200.00 vs. 440,188.30 pg/ml, P = 0.001; Figure 1B; Table 2) were observed in patients with PD than in HCs. However, serum levels of sCD163 showed no significant difference between patients with PD and HCs (269,202.18 vs. 191,443.60 pg/ml, P = 0.114; Supplementary Figure S1A; Table 2).
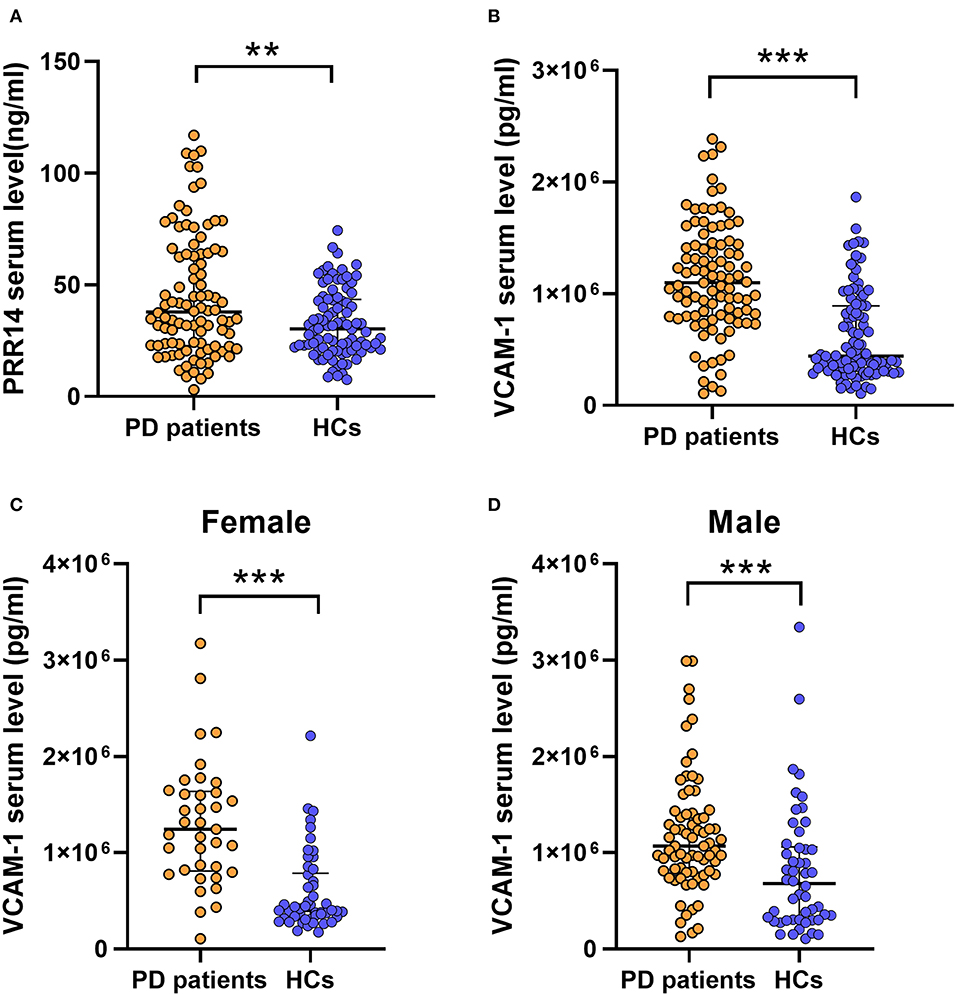
Figure 1. Significantly serum factors implies that inflammation and defective autophagy might play a vital role in the pathophysiology of PD. Higher serum PRR14 (A) and VCAM-1 (B) levels were observed in PD patients compared with HCs. In sex subgroup analysis, PD patients exhibited increased VCAM-1 serum levels compared with HCs both in female subgroups (C) and male subgroups (D). **P < 0.01; ***P < 0.001, Mann–Whitney U test. PD, Parkinson's disease; HCs, healthy controls; PRR14, proline-rich protein 14; VCAM-1, Vascular cell adhesion molecule-1.
Subgroup analyses and correlation analyses among patients with PD
For further analysis, we divided the patients with PD into discrete subgroups according to sex, cognitive impairment status, H&Y stage, and other variables. In sex subgroup analysis, patients with PD exhibited increased VCAM-1 serum levels compared with HCs in both female subgroups (P < 0.001; Figure 1C; Supplementary Table 1) and male subgroups (P = 0.001; Figure 1D; Supplementary Table 1). And the PPR14 and sCD163 serum levels (Supplementary Figures S1B–E; Supplementary Table 1) were observed without some sex differences throughout the sex subgroup analysis. For two groups (with or without cognitive impairment) based on the MMSE test score and the level of education, it proved that lower serum PRR14 levels were linked with severer cognitive impairments (P = 0.048; Figure 2A; Supplementary Table 2). As for three distinctive groups (Mild, Moderate, and Severe groups) defined from the H&Y stage, increased VCAM-1 was associated with severer PD (H&Y) (P =0.036; Figure 2B; Supplementary Tables 3, 4). Further pairwise comparisons implied that there was a significance in serum VCAM-1 level between the Mild groups vs. Severe groups or Moderate groups vs. Severe groups (P=0.034; Figure 2B; Supplementary Table 4). However, other residual subgroup analyses did not reveal a significant difference (Supplementary Tables 5–7), regardless of the potential tendency.
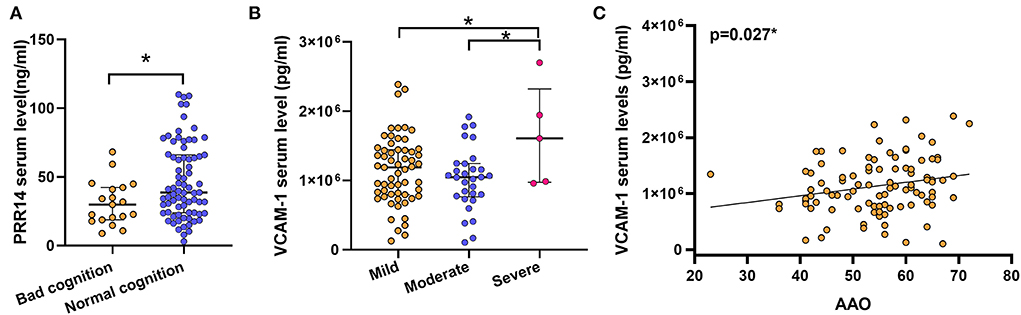
Figure 2. Probable prognostic variables affecting altered factors were uncovered in the subgroup analyses. Lower serum PRR14 levels were linked with severer cognitive impairments (A). *P < 0.05, Mann–Whitney U test. Increased VCAM-1 was associated with severer PD (B). *P < 0.05, Kruskal–Wallis test. Possible relationship between the altered serum factors and clinical related variables were examined by Bivariate association analysis. The results revealed that a later AAO was associated with higher serum VCAM-1 levels (C). *P < 0.05, Spearman correlation test. PD, Parkinson's disease; PRR14, proline-rich protein 14; VCAM-1, Vascular cell adhesion molecule-1; AAO, Age at onset.
Apart from subgroups analysis, bivariate correlation analysis only revealed that there was a positive correlation between VCAM-1 and AAO, but not with other disease-related variables (r = 0.252, P = 0.008; Figure 2C; Table 3). Nevertheless, serum levels of PRR14 were irrelevant to other clinical variables (Table 3). These novel findings need to be confirmed by further experiments.
Discussion
Based on the similar clinical properties of PD compared with other neurodegenerative diseases, it is significant to find an accessible biomarker to effectively diagnose PD with high sensitivity and specificity. An accumulating body of research has focused on alterations in serum factors which plays a crucial role in the pathophysiology of PD (10, 14, 27, 28). Previous studies have shown inconsistent results for factors (PRR14, VCAM-1, and sCD163) that are linked with PD (4, 6, 29). Generally, PRR14 can slow down the clearance of aggregated α-syn or protect the DA neurons (4, 7), while VCAM-1 and sCD163 were involved with the ongoing neuroinflammatory processes in PD (5, 6). In this study, we found that serum levels of PRR14 and VCAM-1 increased in patients with PD. However, no significant difference was observed in the serum sCD163 levels. Furthermore, we found that decreased PRR14 and increased VCAM-1 were linked with severer cognitive impairments and severer PD (H&Y), respectively. As for the bivariate correlation analysis, we uncovered a positive correlation between VCAM-1 and AAO.
Depending on two separable modular domains, PRR14 bridged the nuclear lamina or nuclear membrane and heterochromatin, which plays a role in the potentiation of mTOR signal and inhibition of autophagy (30, 31). As a well-known oncogene, many studies have demonstrated the upregulation of PRR14 in breast cancer (32), lung cancer (33), colon cancer (31), and so on. This sign was regarded as carcinogenesis and progression, which relies on the activation of the PI3-kinase/Akt/mTOR pathway (4). This common pathway also participates in PD and other degenerative diseases via autophagy (10, 34). Of note, as an activator for the mTOR pathway, increased PRR14 is detected in the CSF, serum, and plasma samples of patients with PD and relevant animal models (4, 7, 11, 12), which may result in the suppression of autophagy, accumulation of α-syn, and neuronal death (4). Conversely, some studies suggested that increased serum PRR14 levels were linked with the protection of DA neurons (4). Interestingly, among patients with PD, high serum PRR14 levels increased the risk of constipation (4). In our study, increased PRR14 was similar to other studies in patients with PD (4). Noteworthy, our serum PRR14 levels for HCs were different compared with previous studies, which could attribute to the regional distinction and difference in the size of samples (4). As for the subgroup analysis, we first found that lower PRR14 levels were associated with severer cognitive impairments (P = 0.048; Figure 2A, Supplementary Table 2). Further explorations are required to fully understand the increased PRR14 effect on cognitive impairments during PD.
Vascular cell adhesion molecule-1is a vascular inflammation factor that plays an important role in BBB dysfunction and neoangiogenesis (5, 35). With the initiation of inflammation, activated microglia and astrocytes release typical pro-inflammatory cytokines to disrupt the BBB. Hence, VCAM-1 was released from brain endothelial cells (BECs), resulting in the migration of peripheral immune cells from the peripheral blood into the CNS (5, 36–38). Thus, neuroinflammation was aggravated in patients with PD (36). Increased VCAM-1 was demonstrated in the serum and plasma of patients with PD (7, 29), and in the CSF of diabetes patients (39). Interestingly, CSF levels of VCAM-1 positively correlated with BBB permeability (39), and VCAM-1 can directly increase BBB permeability (35). Genetic ablation of VCAM-1 in BECs can ameliorate neuroinflammation via the VCAM-1-very late antigen 4 (VLA4)-axis (15). In a 6-OHDA animal model of PD, decreased VCAM-1 was found in the midbrain, instead of the striatum (16). Of note, higher VCAM-1 levels were positively linked with cognitive impairment in a large cohort of elderly participants (40). For patients with PD, higher serum VCAM-1 level was positively correlated with worsening motor symptoms (MDS-UPDRS score) and disease severity (H&Y stage), which supported that VCAM-1 is a potential biomarker (5, 7). In addition, higher VCAM-1 levels were found in fatigued patients with PD compared with non-fatigued patients with PD (29), and the factors were negatively correlated with the gray matter volume of some brain regions (left parahippocampus, cerebellum, etc.) in patients with PD, which uncovered the relationship between vascular inflammatory and brain atrophy during the neurodegenerative process in PD (5). In our study, serum VCAM-1 levels were increased in patients with PD. Subgroup analyses indicated that higher VCAM-1 was associated with severer PD (Mild groups vs. Severe groups; Figure 2B; Supplementary Tables 1–7) and later AAO (Figure 2C; Table 3). We speculated that later AAO of patients with PD had defective adaptive immune systems and were sensitive to stressors, which increased the risk of developing PD (5, 41). The mechanisms underlying our results remain to be explored in the future.
It is well-known that increased sCD163 is a distinct sign of monocyte activation, which is exclusively shed from the surface of monocytes and macrophages to produce soluble products (6, 42, 43). Notably, sCD163 shedding enhances α-syn uptake, and α-syn in turn induces sCD163 shedding by activating monocytes in myeloid cell lines and monocyte-derived macrophages, respectively (6). In typical MPTP PD animal models, CD163 was increased in the striatum and substantia nigra, while other studies displayed converse results (17). Corresponding studies suggested that sCD163 was a novel biomarker in PD because elevated CSF and serum of sCD163 were positively correlated with α-syn levels and cognitive impairments in patients with PD (6, 43). In addition, previous studies found that serum sCD163 levels were elevated in late PD women, but not in men, which implied the sex differences (6). However, our study showed no significant difference in serum sCD163 levels of patients with PD and HCs (Supplementary Figure S1A; Table 2). These discordant results could be attributed to regional differences or differences in sample sizes among the participants, which requires more evidence.
In conclusion, we found that serum levels of PRR14 and VCAM-1 were increased in patients with PD compared to HCs. Furthermore, decreased PRR14 was associated with severer cognitive impairments, and increased VCAM-1 was linked with severer PD and later AAO. However, our studies had some limitations, such as the small sample size (patients with PD and HCs), higher percentages of using antiparkinsonian medication (80%; Table 1), and lower education level, which may slightly influence the values of results. Therefore, future studies will increase the sample size and recruit more matched and general patients (the whole medication-naïve patients) and control subjects to gain a comprehensive understanding of the mechanisms of PD. Overall, these results suggest that the initiation of the inflammation cascade and defective autophagy might play a vital role in the pathophysiology of PD.
Data availability statement
The datasets to this article are available from the corresponding author upon reasonable request.
Ethics statement
The studies involving human participants were reviewed and approved by Medical Research Ethics Committee of the First Affiliated Hospital of Zhengzhou University. The patients/participants provided their written informed consent to participate in this study.
Author contributions
HZ: designed the research project, organized the study, executed the research, designed the statistical analysis, and wrote the first draft. TW: executed the research and designed the statistical analysis. CS: executed the research, reviewed the manuscript, and provided funding. LF: organized the study and executed the statistical analysis. YS and XL: organized the study. YF: executed the statistical analysis and reviewed the statistics. JY: reviewed the manuscript. CM: designed the research project, reviewed the manuscript, and provided funding. YX: provided funding. All authors contributed to the article and approved the submitted version.
Funding
This work was supported by the National Natural Science Foundation of China (Grant U1904207 and 91849115 to YX, Grant 81771290 and 81974211 to CS, Grant 82271277 and 81901300 to CM), Provincial Key Scientific and Technological Projects (Grant SBGJ202003020 to CM), the Innovative and Scientific and Technological Talent Training Project of Henan Province (Grant YXKC2021062 to CM), and the Non-Profit Central Research Institute Fund of Chinese Academy of Medical Sciences.
Acknowledgments
The authors would like to gratefully acknowledge the participation of all our patient volunteers. We are indebted to the support and assistance of The First Affiliated Hospital of Zhengzhou University. We also unfeignedly acknowledge the logical critiques and professional technical assistance in our collaborative research team.
Conflict of interest
The authors declare that the research was conducted in the absence of any commercial or financial relationships that could be construed as a potential conflict of interest.
Publisher's note
All claims expressed in this article are solely those of the authors and do not necessarily represent those of their affiliated organizations, or those of the publisher, the editors and the reviewers. Any product that may be evaluated in this article, or claim that may be made by its manufacturer, is not guaranteed or endorsed by the publisher.
Supplementary material
The Supplementary Material for this article can be found online at: https://www.frontiersin.org/articles/10.3389/fneur.2022.993940/full#supplementary-material
Supplementary Figure 1. For the alteration of PD patients and HCs groups, no significance was found in the serum sCD163 levels between two groups (A). For sex subgroup analysis, PRR14 and sCD163 serum levels were observed without the sex differences among the female (B,D) and male subgroups (C,E). Mann–Whitney U test. PD, Parkinson's disease; PRR14, proline-rich protein 14; sCD163, soluble CD163.
Abbreviations
PD, Parkinson's disease; DA neurons, dopaminergic neurons; α-syn, α-synuclein; CNS, central nervous system; PRR14, proline-rich protein 14; VCAM-1, vascular cell adhesion molecule-1; sCD163, soluble CD163; HCs, healthy controls; mTOR, the mammalian target of rapamycin; CSF, cerebrospinal fluid; BBB, blood–brain barrier; 6-OHDA, 6-hydroxydopamine; MPTP, 1-methyl-4-phenyl-1,2,3,6-tetrahydropyridine; AAO, age at onset; H&Y, Hohn and Yahr; PDD, PD Dementia; DLB, dementia with Lewy body; NSAIDs, non-steroidal anti-inflammatory drugs; MDS-UPDRS, Movement Disorder Society-Unified Parkinson's Disease Rating Scale; MMSE, Mini-Mental State Examination; LEDDs, levodopa-equivalent daily doses; PIGD, postural instability/gait difficulty; ID, Indeterminate; TD, tremor dominant; ELISA, Enzyme-linked Immunosorbent Assay; LSD test, post-hoc test (LSD) test; BECs, brain endothelial cells; VLA4, very late antigen 4.
References
1. Shults CW. Lewy bodies. Proc Natl Acad Sci U S A. (2006) 103:1661–8. doi: 10.1073/pnas.0509567103
2. Kim S, Kwon SH, Kam TI, Panicker N, Karuppagounder SS, Lee S, et al. Transneuronal propagation of pathologic α-synuclein from the gut to the brain models Parkinson's disease. Neuron. (2019) 103:627-641.e7. doi: 10.1016/j.neuron.2019.05.035
3. Luan M, Sun Y, Chen J, Jiang Y, Li F, Wei L, et al. Diagnostic value of salivary real-time quaking-induced conversion in Parkinson's disease and multiple system atrophy. Mov Disord. (2022) 37:1059–63. doi: 10.1002/mds.28976
4. Jin T, Tan X, Shi X, Lv L, Peng X, Zhang H, et al. Preliminary findings on proline-rich protein 14 as a diagnostic biomarker for Parkinson's disease. Neuromolecular Med. (2020) 23:285–91. doi: 10.1007/s12017-020-08617-z
5. Yu CC, Chen HL, Chen MH, Lu CH, Tsai NW, Huang CC, et al. Vascular inflammation is a risk factor associated with brain atrophy and disease severity in Parkinson's disease: a case-control study. Oxid Med Cell Longev. (2020) 2020:2591248. doi: 10.21203/rs.2.19756/v1
6. Nissen SK, Ferreira SA, Nielsen MC, Schulte C, Shrivastava K, Hennig D, et al. Soluble CD163 changes indicate monocyte association with cognitive deficits in Parkinson's disease. Mov Disord. (2021) 36:963–76. doi: 10.1002/mds.28424
7. Perner C, Perner F, Gaur N, Zimmermann S, Witte OW, Heidel FH, et al. Plasma VCAM1 levels correlate with disease severity in Parkinson's disease. J Neuroinflammation. (2019) 16:94. doi: 10.1186/s12974-019-1482-8
8. Levy JMM, Towers CG, Thorburn A. Targeting autophagy in cancer. Nat Rev Cancer. (2017) 17:528–42. doi: 10.1038/nrc.2017.53
9. Gao S, Duan C, Gao G, Wang X, Yang H. Alpha-synuclein overexpression negatively regulates insulin receptor substrate 1 by activating mTORC1/S6K1 signaling. Int J Biochem Cell Biol. (2015) 64:25–33. doi: 10.1016/j.biocel.2015.03.006
10. Zhu Z, Yang C, Iyaswamy A, Krishnamoorthi S, Sreenivasmurthy SG, Liu J, et al. Balancing mTOR signaling and autophagy in the treatment of Parkinson's disease. Int J Mol Sci. (2019) 20:728. doi: 10.3390/ijms20030728
11. Sinha A, Srivastava N, Singh S, Singh AK, Bhushan S, Shukla R, et al. Identification of differentially displayed proteins in cerebrospinal fluid of Parkinson's disease patients: a proteomic approach. Clin Chim Acta. (2009) 400:14–20. doi: 10.1016/j.cca.2008.09.026
12. Soreq L, Ben-Shaul Y, Israel Z, Bergman H, Soreq H. Meta-analysis of genetic and environmental Parkinson's disease models reveals a common role of mitochondrial protection pathways. Neurobiol Dis. (2012) 45:1018–30. doi: 10.1016/j.nbd.2011.12.021
13. Ferreira SA, Romero-Ramos M. Microglia response during parkinson's disease: alpha-synuclein intervention. Front Cell Neurosci. (2018) 12:247. doi: 10.3389/fncel.2018.00247
14. Nagatsu T, Sawada M. Inflammatory process in Parkinson's disease: role for cytokines. Curr Pharm Des. (2005) 11:999–1016. doi: 10.2174/1381612053381620
15. Yousef H, Czupalla CJ, Lee D, Chen MB, Burke AN, Zera KA, et al. Aged blood impairs hippocampal neural precursor activity and activates microglia via brain endothelial cell VCAM1. Nat Med. (2019) 25:988–1000. doi: 10.1038/s41591-019-0440-4
16. Armentero MT, Levandis G, Bazzini E, Cerri S, Ghezzi C, Blandini F. Adhesion molecules as potential targets for neuroprotection in a rodent model of Parkinson's disease. Neurobiol Dis. (2011) 43:663–8. doi: 10.1016/j.nbd.2011.05.017
17. De Nuccio F, Cianciulli A, Porro C, Kashyrina M, Ruggiero M, Calvello R, et al. Inflammatory response modulation by vitamin C in an MPTP mouse model of Parkinson's disease. Biology. (2021) 10:1155. doi: 10.3390/biology10111155
18. Calvello R, Cianciulli A, Nicolardi G, De Nuccio F, Giannotti L, Salvatore R, et al. Vitamin D treatment attenuates neuroinflammation and dopaminergic neurodegeneration in an animal model of Parkinson's disease, shifting M1 to M2 microglia responses. J Neuroimmune Pharmacol. (2017) 12:327–39. doi: 10.1007/s11481-016-9720-7
19. Postuma RB, Berg D, Stern M, Poewe W, Olanow CW, Oertel W, et al. MDS clinical diagnostic criteria for Parkinson's disease. Mov Disord. (2015) 30:1591–601. doi: 10.1002/mds.26424
20. McKeith IG, Boeve BF, Dickson DW, Halliday G, Taylor JP, Weintraub D, et al. Diagnosis and management of dementia with Lewy bodies: fourth consensus report of the DLB consortium. Neurology. (2017) 89:88–100. doi: 10.1212/WNL.0000000000004058
21. Emre M, Aarsland D, Brown R, Burn DJ, Duyckaerts C, Mizuno Y, et al. Clinical diagnostic criteria for dementia associated with Parkinson's disease. Mov Disord. (2007) 22:1689-707. doi: 10.1002/mds.21507
22. Hardoff R, Sula M, Tamir A, Soil A, Front A, Badarna S, et al. Gastric emptying time and gastric motility in patients with Parkinson's disease. Mov Disord. (2001) 16:1041–7. doi: 10.1002/mds.1203
23. Kouchaki E, Daneshvar Kakhaki R, Tamtaji OR, Dadgostar E, Behnam M, Zaribaf A, et al. Correlation of serum levels and gene expression of tumor necrosis factor-α-induced protein-8 like-2 with Parkinson disease severity. Metab Brain Dis. (2018) 33:1955–9. doi: 10.1007/s11011-018-0302-7
24. Li H, Jia J, Yang Z. Mini-mental state examination in elderly chinese: a population-based normative study. J Alzheimers Dis. (2016) 53:487–96. doi: 10.3233/JAD-160119
25. Tomlinson CL, Stowe R, Patel S, Rick C, Gray R, Clarke CE. Systematic review of levodopa dose equivalency reporting in Parkinson's disease. Mov Disord. (2010) 25:2649–53. doi: 10.1002/mds.23429
26. Shen B, Pan Y, Jiang X, Wu Z, Zhu J, Dong J, et al. Altered putamen and cerebellum connectivity among different subtypes of Parkinson's disease. CNS Neurosci Ther. (2020) 26:207–14. doi: 10.1111/cns.13259
27. Tansey MG, Goldberg MS. Neuroinflammation in Parkinson's disease: its role in neuronal death and implications for therapeutic intervention. Neurobiol Dis. (2010) 37:510–8. doi: 10.1016/j.nbd.2009.11.004
28. Whitton PS. Inflammation as a causative factor in the aetiology of Parkinson's disease. Br J Pharmacol. (2007) 150:963–76. doi: 10.1038/sj.bjp.0707167
29. Herlofson K, Heijnen CJ, Lange J, Alves G, Tysnes OB, Friedman JH, et al. Inflammation and fatigue in early, untreated Parkinson's disease. Acta Neurol Scand. (2018) 138:394–9. doi: 10.1111/ane.12977
30. Dunlevy KL, Medvedeva V, Wilson JE, Hoque M, Pellegrin T, Maynard A, et al. The PRR14 heterochromatin tether encodes modular domains that mediate and regulate nuclear lamina targeting. J Cell Sci. (2020) 133:jcs240416. doi: 10.1242/jcs.240416
31. Li F, Zhang C, Fu L. PRR14 overexpression promotes cell growth, epithelial to mesenchymal transition and metastasis of colon cancer via the AKT pathway. PLoS ONE. (2019) 14:e0218839. doi: 10.1371/journal.pone.0218839
32. Ren X, Long M, Li Z, Wu B, Jin T, Tu C, et al. Oncogene PRR14 promotes breast cancer through activation of PI3K signal pathway and inhibition of CHEK2 pathway. Cell Death Dis. (2020) 11:464. doi: 10.1038/s41419-020-2640-8
33. Yang M, Lewinska M, Fan X, Zhu J, Yuan ZM. PRR14 is a novel activator of the PI3K pathway promoting lung carcinogenesis. Oncogene. (2016) 35:5527–38. doi: 10.1038/onc.2016.93
34. Maiese K, Chong ZZ, Shang YC, Wang S. mTOR: on target for novel therapeutic strategies in the nervous system. Trends Mol Med. (2013) 19:51–60. doi: 10.1016/j.molmed.2012.11.001
35. Haarmann A, Nowak E, Deiß A, van der Pol S, Monoranu CM, Kooij G, et al. Soluble VCAM-1 impairs human brain endothelial barrier integrity via integrin α-4-transduced outside-in signalling. Acta Neuropathol. (2015) 129:639–52. doi: 10.1007/s00401-015-1417-0
36. Reale M, Iarlori C, Thomas A, Gambi D, Perfetti B, Di Nicola M, et al. Peripheral cytokines profile in Parkinson's disease. Brain Behav Immun. (2009) 23:55–63. doi: 10.1016/j.bbi.2008.07.003
37. Nelson AR, Sweeney MD, Sagare AP, Zlokovic BV. Neurovascular dysfunction and neurodegeneration in dementia and Alzheimer's disease. Biochim Biophys Acta. (2016) 1862:887–900. doi: 10.1016/j.bbadis.2015.12.016
38. Troncoso MF, Ortiz-Quintero J, Garrido-Moreno V, Sanhueza-Olivares F, Guerrero-Moncayo A, Chiong M, et al. VCAM-1 as a predictor biomarker in cardiovascular disease. Biochim Biophys Acta Mol Basis Dis. (2021) 1867:166170. doi: 10.1016/j.bbadis.2021.166170
39. Janelidze S, Hertze J, Nägga K, Nilsson K, Nilsson C, Wennström M, et al. Increased blood-brain barrier permeability is associated with dementia and diabetes but not amyloid pathology or APOE genotype. Neurobiol Aging. (2017) 51:104–12. doi: 10.1016/j.neurobiolaging.2016.11.017
40. Tchalla AE, Wellenius GA, Sorond FA, Gagnon M, Iloputaife I, Travison TG, et al. Elevated soluble vascular cell adhesion molecule-1 is associated with cerebrovascular resistance and cognitive function. J Gerontol A Biol Sci Med Sci. (2017) 72:560–6. doi: 10.1093/gerona/glw099
41. Tansey MG, Wallings RL, Houser MC, Herrick MK, Keating CE, Joers V. Inflammation and immune dysfunction in Parkinson disease. Nat Rev Immunol. (2022) 4:1–17. doi: 10.1038/s41577-022-00684-6
42. Bian Z, Gong Y, Huang T, Lee CZW, Bian L, Bai Z, et al. Deciphering human macrophage development at single-cell resolution. Nature. (2020) 582:571–6. doi: 10.1038/s41586-020-2316-7
Keywords: Parkinson's disease, proline-rich protein 14, vascular cell adhesion molecule-1, soluble CD163, serum
Citation: Zheng H, Wang T, Shi C, Fan L, Su Y, Fan Y, Li X, Yang J, Mao C and Xu Y (2022) Increased PRR14 and VCAM-1 level in serum of patients with Parkinson's disease. Front. Neurol. 13:993940. doi: 10.3389/fneur.2022.993940
Received: 14 July 2022; Accepted: 07 September 2022;
Published: 30 September 2022.
Edited by:
Katerina Markopoulou, NorthShore University HealthSystem, United StatesReviewed by:
Michele Sandre, University Hospital of Padua, ItalyKeisuke Suzuki, Dokkyo Medical University, Japan
Copyright © 2022 Zheng, Wang, Shi, Fan, Su, Fan, Li, Yang, Mao and Xu. This is an open-access article distributed under the terms of the Creative Commons Attribution License (CC BY). The use, distribution or reproduction in other forums is permitted, provided the original author(s) and the copyright owner(s) are credited and that the original publication in this journal is cited, in accordance with accepted academic practice. No use, distribution or reproduction is permitted which does not comply with these terms.
*Correspondence: Chengyuan Mao, bWFvY2hlbmd5dWFuMjAxNSYjeDAwMDQwOzEyNi5jb20=; Yuming Xu, eHV5dW1pbmcmI3gwMDA0MDt6enUuZWR1LmNu
†These authors have contributed equally to this work and share first authorship