- 1Comprehensive Stroke Center, Institute of Neuroscience, Hospital Clinic of Barcelona, Barcelona, Spain
- 2Neurosurgery Department, Institute of Neuroscience, Hospital Clinic of Barcelona, Barcelona, Spain
- 3Institut d'Investigacions Biomèdiques Agustí Pi i Sunyer (IDIBAPS), Barcelona, Spain
- 4Department of Medicine, Faculty of Medicine and Health Sciences, University of Barcelona, Barcelona, Spain
Hyperglycaemia, hypoglycaemia and higher glucose variability during the Early Brain Injury (EBI) period of aneurysmal subarachnoid hemorrhage (aSAH) have been associated with poor clinical outcome. However, it is unclear whether these associations are due to direct glucose-driven injury or if hyperglycaemia simply acts as a marker of initial severity. Actually, strict glucose control with intensive insulin therapy has not been demonstrated as an effective strategy for improving clinical outcomes after aSAH. Currently published studies describing an association between hyperglycaemia and prognosis in aSAH patients have been based on isolated glucose measurements and did not incorporate comprehensive dynamic evaluations, such as those derived from subcutaneous continuous glucose monitoring devices (CMG). Arguably, a more accurate knowledge on glycaemic patterns during the acute phase of aSAH could increase our understanding of the relevance of glycaemia as a prognostic factor in this disease as well as to underpin its contribution to secondary focal and diffuse brain injury. Herein, we have summarized the available evidence on the diagnostic and prognostic relevance of glucose metrics during the acute phase of cerebrovascular diseases, focusing in the EBI period after aSAH. Overall, obtaining a more precise scope of acute longitudinal glucose profiles could eventually be useful for improving glucose management protocols in the setting of acute aSAH and to advance toward a more personalized management of aSAH patients during the EBI phase.
Introduction
Hyperglycaemia occurs in about three out of four aSAH patients within the first 72 h after the initial bleeding and it has been associated with poor clinical outcomes and secondary brain injury (1–7). However, it remains to be clarified whether these observed associations are due to direct deleterious effects induced by high blood sugar or if hperglycaemia is simply a marker of stroke severity. Although hyperglycemia has been related to worse outcome in several pathologies, there is conflicting evidence about the benefits of intensive glucose management for improving clinical outcomes in different critical settings (8, 9). Thus, the potential clinical benefit of strict control with intensive insulin therapy has not yet been validated by clinical trials (7, 10–13). Of note, intensive management of blood glucose may result in hypoglycaemia, and both hyperglycaemia and hypoglycaemia have been associated with higher risk of complications during the acute phase of aSAH and with poorer clinical recovery at long-term. Hyperglycaemia is, in part, a consequence of an acute stress response to brain injury and may enhance neuroinflammatory mechanisms and increase the risk of ischemic complications such as delayed cerebral ischemia (DCI) (5–7). Conversely, hypoglycaemia is also dangerous for the brain, which relies on glucose for maintaining its function, and low glucose levels may eventually increase secondary brain injury through a myriad of mechanisms that include a poorer tissue tolerance to ischemia (5–7). Besides, acute fluctuations in serum glucose concentrations are common after aSAH and may also result detrimental (1–7, 14–16). In this review, we will summarize the available evidence on the diagnostic and relevance of glucose metrics during the acute phase of cerebrovascular diseases, focusing in the EBI period after aSAH.
Glycaemic status in acute cerebrovascular illnesses: How to tackle the problem
Glycaemic dysregulation decisively affects vital and functional prognosis of patients across several cerebrovascular diseases, although it is still unclear how to effectively address the glycaemic status in the acute phase of critical diseases such as aSAH. Traditionally, glycaemic evaluation has relied on static parameters, like glycaemia on admission or premorbid glycaemic status including known-diabetes or glycated hemoglobin (HbA1c) on admission. Nonetheless, glycaemia is a rapidly changing parameter due to both disease evolution and acute management-related factors that are only partially understood. Therefore, static markers may be inadequate to address the true relevance of glycaemic status and to guide insulin therapy in the acute setting. To overcome this limitation, several studies addressed glycaemia as a dynamic variable by means of evaluating the glycaemic variability (GV) (17, 18). In this context, subcutaneous Continuous Glucose Monitoring (CGM) devices have emerged as a potential substitute to point of care standards in the inpatient setting. In brief, CGM devices are usually placed on the thigh, abdomen or arm, and measure interstitial fluid glucose generally through an oxidase-peroxidase reaction. That information is sent to an external device, then allowing a remote, non-invasive, high temporal resolution evaluation of the glycaemic status. Common technical limitations of CGM in an ICU setting include biofilm formation, need for calibration, measurement lag and the interaction of drugs such as acetaminophen or vasopressors (19, 20). Initial studies evaluating the feasibility of CGM in the ICU setting have mainly focused on the accuracy of the technique, before assessing its ability to guide treatments. In general, and in spite of the technical limitations cited above, the device accuracy seems to be acceptable and reproducible across different series and acute clinical settings, with no major safety issues. From a logistic and economic point of view, some reports indicate that CGM devices might significantly reduce nurse workload regarding glycaemic control and might be cost-effective (21, 22).
The exhaustive information obtained through CGM includes static measures such as mean, maximum and minimum glucose levels, the amount of time spent above or below a predefined threshold, and also several dynamic features accounting for GV. CGM also allows the identification of different longitudinal glucose profiles, as well as performing complex analyses of glucose homeostasis, which would be not feasible with conventional testing. The main advantages of CGM, compared to conventional finger prick testing, are the ability to comprehensively analyse a multitude of parameters and to obtain their longitudinal trajectory profiles. Reports on CGM in acute cerebrovascular diseases showed similar-to-better detection of dysglycaemic events, mainly hypoglycaemia, compared to the standard of care. Interestingly, some of these events show circadian variability and nocturnal preference (23, 24), being easily overlooked with the conventional scheduled capillary glucose measurements.
However, the information derived from CGM devices should be interpreted carefully, since definitions and thresholds of the novel glycaemic parameters could be complex and heterogeneous. Beyond the extraction of the aforementioned predefined key metrics, the comprehensive analysis of CGM-derived repeated measurements may require sophisticated analytic tools and pre-planned analytical strategies (19, 20). In addition, evidence regarding which subsets of patients would benefit from wearing a CGM device is still scarce (19).
The ability of CGM to guide intensive insulin treatments (IIT) is also a matter of debate. Although the use of CGM has spread in the outpatient setting for certain indications, its utility in the inpatient setting remains to be demonstrated. Thus, interventional clinical trials based on CGM are scarce in comparison with accuracy and safety studies. A recent report in a cardiac ICU demonstrated good patient and caregivers' acceptance, accuracy and reliability of subcutaneous CGM measurements (25). Indeed, CGM could be of potential great value in the management of acute illnesses, especially in patients at risk for high GV and hypoglycaemia, including those affected by vascular brain injury (19). A retrospective analysis of two randomized controlled trials including critically ill patients that compared CGM-driven IIT vs. conventional arterial point of care (POC)-driven IIT showed that CGM driven-IIT did not result in a significantly reduced GV. However, glucose complexity measures calculated with real time CGM data were predictive of the risk of mortality (26). In one of those trials, real-time CGM-driven IIT was associated with a reduced risk of hypoglycaemic events, an observation that was also replicated in a similarly designed trial including postcardiac surgery patients (27, 28). In the same line, a recent trial comparing insulin dosing driven by CGM measurements vs. standard POC glucose testing in a cohort of hospitalized patients receiving IIT or bolus basal therapy showed earlier hypoglycaemia detection in the CGM group (29).
In summary, CGM technology seems safe, accurate and might be cost-effective in the acute inpatient setting, although its clinical value for guiding IIT or for prognostic purposes in patients who need tight glycaemic control, as those affected by acute brain lesions, remains to be demonstrated. Importantly, the benefit of strict glycaemic control with IIT for improving clinical outcomes in aSAH remains to be validated in clinical trials before an eventual implementation of CGM for guiding IIT (7, 10–13).
Diagnostic and prognostic relevance of static and dynamic glucose metrics in the acute phase of aSAH
Patients with aSAH may suffer focal and diffuse brain lesions in addition to the initial bleeding, a fact that dramatically impacts the functional and cognitive outcomes at long term. According to the moment of appearance, within or after the first 72 h from the initial bleeding, these brain lesions are classified as part of EBI or DCI, respectively (30–33). Traditionally, DCI secondary to vasospasm has been considered one of the main complications related to poor prognosis in this disease, although therapeutic approaches aimed to control angiographic vasospasm have not shown reliable clinical benefits (33, 34). On the other hand, the severity of EBI, both via acute ischemic lesions or microstructural diffuse lesions, is associated with an increased risk of systemic complications and with poorer clinical, cognitive and affective outcomes at long-term (35–37). There is growing evidence of the role that physiopathological changes play in the promotion of EBI after aSAH. These mechanisms include loss of cerebral autoregulation, microthrombosis, enhanced exposure to inflammation and oxidative stress, blood brain barrier (BBB) disruption and apoptotic cell death, among others (38–43).
Hyperglycaemia has been associated with a prothrombotic state, increased neuroinflammation, oxidative stress and BBB disruption in several clinical and preclinical models of central nervous system diseases. A plausible underlying mechanism is the overproduction of mitochondrial reactive oxygen species, which occurs preferentially in tissues that are insulin-independent like the brain (44–49). In experimental SAH, hyperglycaemia promotes neuronal apoptosis and is associated to higher incidence of vasospasm (43, 50). In the vulnerable brain, hyperglycaemia also promotes anaerobic glycolysis leading to the accumulation of lactate, a process thought to contribute to increased brain injury (6, 51–53).
From a clinical standpoint, dysglycaemia is a major prognostic factor in both early and late phases of spontaneous aSAH, as summarized in Table 1. First, hyperglycaemia has been associated with worse clinical and radiological presentation, as well as with higher in-hospital mortality, higher rates of neurological and systemic complications, and ultimately worse short-term and long-term functional outcomes (3, 5, 7, 14, 16, 54–62, 64–66). On the other hand, hypoglycaemia has been strongly linked to in-hospital mortality and incidence of vasospasm (55, 59, 61–63). Otherwise, poor premorbid metabolic control estimated by HbA1c upon admission does not seem to consistently correlate with the acute neurological status nor with DCI or neurological outcome at long-term (64, 65). Some authors have proposed the use of ratios to increase the predictive value of admission blood glucose levels. In this line, the glucose-potassium and glucose-phosphate ratios on admission proved to be a significant predictor of vasospasm, rebleeding, along with acute and mid-term functional outcomes (58, 60, 66, 67).
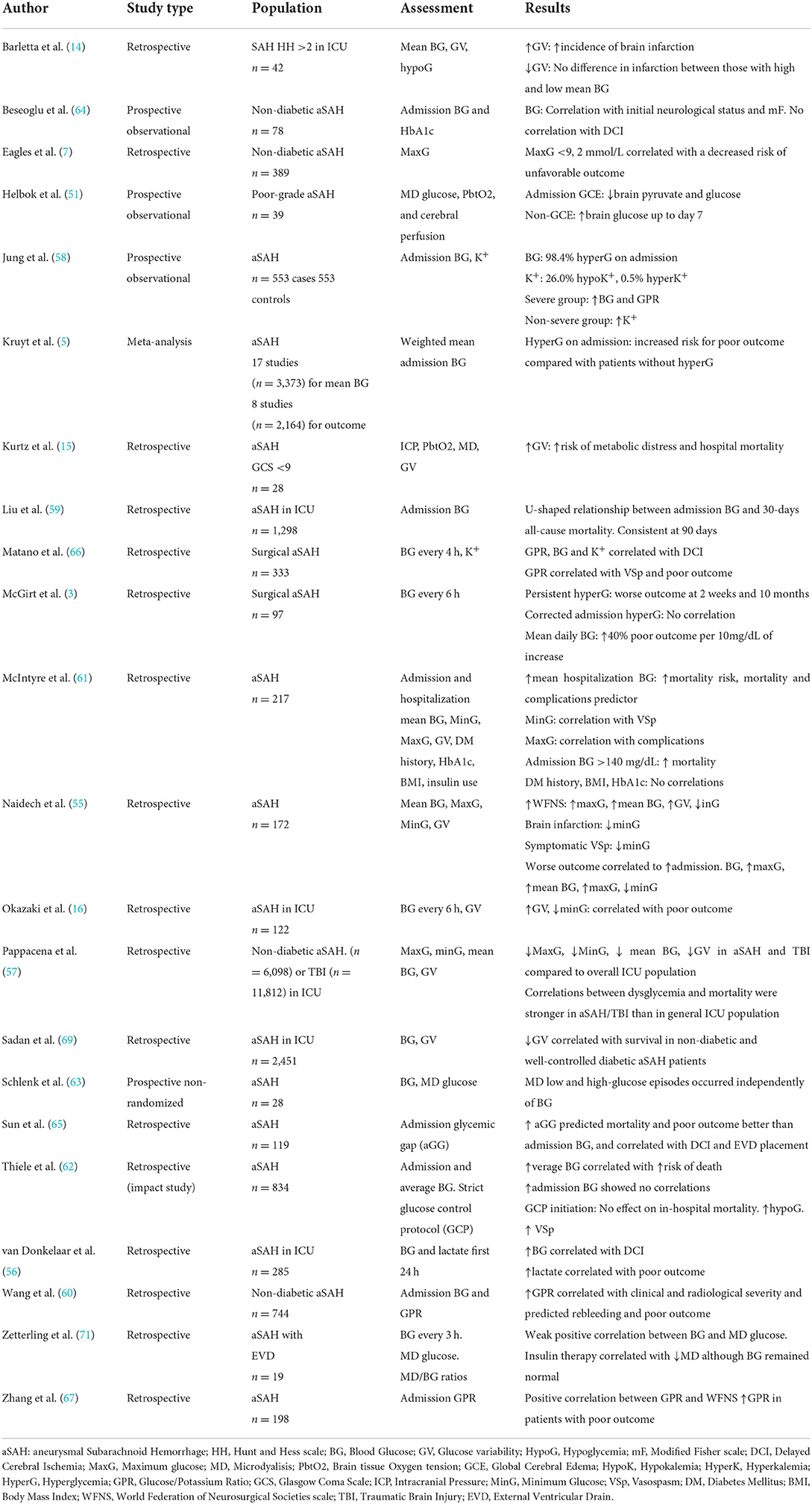
Table 1. Main studies assessing the impact of glucose and brain metabolism in prognosis and complications after spontaneous SAH.
Even when hyperglycaemia on admission is a very sensible biomarker, it has been suggested that GV is a more reliable tool to predict outcomes (3, 14–16, 68, 69). Several formulas have been proposed to express the GV, yet they usually rely on comparison of low temporal resolution assessments of capillary glucose. A remarkable observation in terms of GV implications is the differential behavior between diabetic and non-diabetic patients during the acute phase of aSAH. Prior studies have suggested that the association between GV and outcome occurs in non-diabetic and well-controlled diabetic patients, but not in those with poorly controlled diabetes (69). Therefore, tolerance to high or low glucose levels may vary according to the premorbid glycaemic status.
Importantly, blood glucose levels might not always reflect those present in the brain interstitium as assessed with mycrodyalisis catheters (70, 71). In acute aSAH, glucose transport through the BBB is impaired, causing that even normal levels of blood glucose result relatively insufficient to supply the raised brain metabolic demand that occurs during the EBI period (71). This phenomenon poses patients with aSAH at a vulnerable state, where glycaemic fluctuations may provoke brain metabolic distress and secondary injury. Remarkably, systemic GV even within normal limits of blood glucose levels has also been associated with the incidence of cerebral metabolic distress, evaluated though multimodal monitoring with cerebral microdialysis probes (15, 51). Henceforth, GV instead of absolute glucose levels might better reflect the clinical course of severe aSAH, and eventually guide glycaemic-control interventions.
To our knowledge no studies regarding the prognostic relevance of glucose temporal profiles have used GCM devices in the specific setting of aSAH. Indeed, there is no sufficient evidence to conclude that longitudinal evaluation of glycaemic patterns have a greater prognostic value during the EBI period of aSAH. The vast majority of published data on hyperglycaemia and prognosis in aSAH patients are based on scheduled capillary glucose measurements as implemented in standard clinical practice (e.g., capillary glucose determinations every 6 h within the first days after bleeding) and have not considered including comprehensive dynamic evaluations, such as those derived from CGM devices. Arguably, using CGM to obtain accurate glycaemic profiles during the acute phase of aSAH could help define the relevance of glycaemia as a prognostic factor in this disease, as well as underpin its contribution to secondary focal and diffuse brain injury.
Diagnostic and prognostic yield of glucose monitoring in acute cerebrovascular diseases
Studies focused on hyperacute glucose monitoring in cerebrovascular diseases have included mainly patients affected by acute ischemic stroke (AIS). In this setting, both admission and acute-phase hyperglycaemia have been associated with mid-term and long-term morbidity and mortality following a J-shaped fashion, as well as with higher incidence of infarct growth, ischemic recurrence risk and post-stroke cognitive impairment (72–76). After alteplase infusion, hyperglycaemia correlates with worse recanalization status, higher odds of symptomatic haemorrhagic transformation (sICH) and poorer clinical recovery (77–80). Higher GV after alteplase administration has also been associated with higher concentrations of circulating markers of inflammation and with worse clinical outcomes (81). In the same line, after mechanical thrombectomy, glycaemic disarrangements have been associated with worse outcomes, higher frequency of sICH and higher risk of death, although no link was found with recanalization rates (82–85). In intracerebral hemorrhage (ICH), hyperglycaemia at admission has been related to worse functional outcomes and death, in both diabetic and non-diabetic populations (86, 87). Reassuringly, a recent meta-analysis that evaluated the prognostic relevance of GV in both AIS and ICH showed a significant association between lower GV and reduced mortality at 30 and 90 days after stroke (88).
Nonetheless, studies using CGM in stroke are still scarce and have been based in modest population sizes. Disglycaemia measured by CGM is frequent after acute cerebrovascular events and has been linked to infarct growth in AIS (89, 90) and with poor prognosis after AIS and ICH (91), as shown in Table 2. It is noteworthy that, in these populations, CGM devices significantly improved hypoglycemia detection compared to standard clinical practice, thus opening a window of opportunity for CGM in patients that require aggressive glycaemic control (23, 24). However, to date, intensive glucose lowering in the acute phase of AIS has not reliably proven a clinical benefit, pointing the lack of consensus on hyperglycaemia definition or blood glucose targets (92, 93).
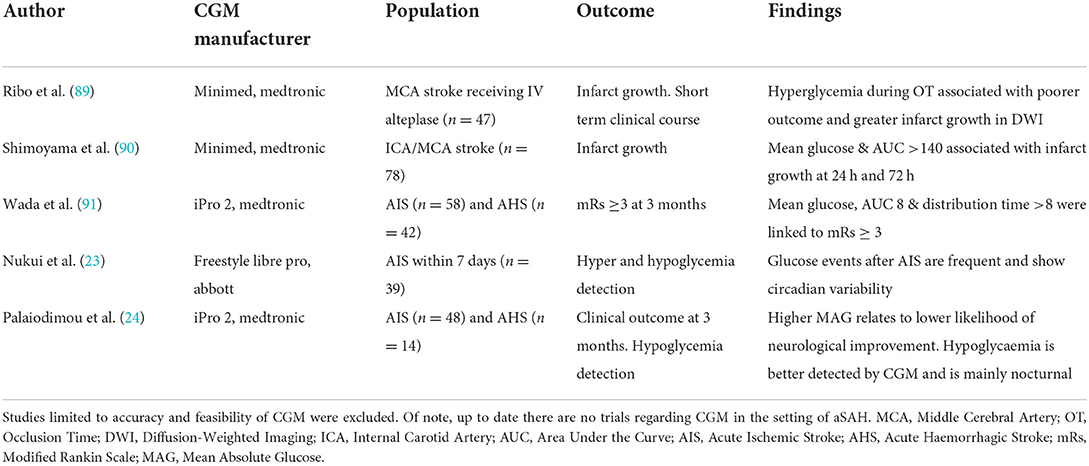
Table 2. Studies assessing potential clinical impact of CGM in cerebrovascular acute disease management.
Conclusions and future directions
Hyperglycaemia, hypoglycemia and glucose variability during the acute phase of aSAH have been associated with an increased risk of in hospital complications and with poor long-term functional recovery. However, it is unclear whether these associations are due to direct deleterious effects induced by glucose dysregulation or if hyperglycaemia is an epiphenomenon related to the initial bleeding severity. In addition, it remains inconclusive whether there are specific longitudinal glycaemic profiles during the EBI period with special prognostic implications and/or more prone to lead to secondary brain injury. Arguably, the use of comprehensive dynamic evaluations, such as those derived from CGM devices, could aid to obtain a more precise understanding of such a highly dynamic process that is conditioned by both systemic complications and specific therapeutic approaches. A more accurate knowledge on glycaemic patterns during the acute phase of aSAH could increase our understanding of the relevance of glycaemia as a prognostic factor in this disease and to underpin its contribution to secondary focal and diffuse brain injury. In this context, the evaluation of biomarkers related with brain microvascular, metabolic and microstructural integrity using quantitative advanced MRI along with the quantification of circulating and cerebrospinal fluid (CSF) molecules related with brain injury could be highly informative for identifying glucose profiles associated with higher brain damage after aSAH. More research is needed to understand the most appropriate and informative timing for glucose monitoring, and how to combine glucose metrics with surrogate markers of acute brain injury. Eventually this information may lead to improve glucose management protocols in the setting of acute aSAH, to optimize the design of clinical trials aimed to modulate glucose levels and to advance toward a more personalized management of aSAH sufferers during the EBI period.
Author contributions
DS, AM, LP, LL, RT, and SA participated in writing the manuscript and editing critical parts of this review. LL, RT, and SA gave the final approval for this submission. All authors contributed to the article and approved the submitted version.
Funding
We thank the Spanish Ministry of Economy and Competitiveness for the grant given to SA and RT [project PI19/00936 funded by Instituto de Salud Carlos III and co-funded by the European Regional Development Fund (ERDF)], the Fundació la Marató de TV3 for the grant given to SA (grant number 17/C/2017). DS is supported by a grant from Hospital Clinic de Barcelona (Contracte Clínic de Recerca Emili Letang-Josep Font).
Conflict of interest
The authors declare that the research was conducted in the absence of any commercial or financial relationships that could be construed as a potential conflict of interest.
Publisher's note
All claims expressed in this article are solely those of the authors and do not necessarily represent those of their affiliated organizations, or those of the publisher, the editors and the reviewers. Any product that may be evaluated in this article, or claim that may be made by its manufacturer, is not guaranteed or endorsed by the publisher.
References
1. Dorhout Mees SM, van Dijk GW, Algra A, Kempink DR, Rinkel GJ. Glucose levels and outcome after subarachnoid hemorrhage. Neurology. (2003) 61:1132–3. doi: 10.1212/01.WNL.0000090466.68866.02
2. Badjatia N, Topcuoglu MA, Buonanno FS, Smith EE, Nogueira RG, Rordorf GA, et al. Relationship between hyperglycemia and symptomatic vasospasm after subarachnoid hemorrhage. Crit Care Med. (2005) 33:1603–9. doi: 10.1097/01.CCM.0000168054.60538.2B
3. McGirt MJ, Woodworth GF, Ali M, Than KD, Tamargo RJ, Clatterbuck RE. Persistent perioperative hyperglycemia as an independent predictor of poor outcome after aneurysmal subarachnoid hemorrhage. J Neurosurg. (2007) 107:1080–5. doi: 10.3171/JNS-07/12/1080
4. Dungan KM, Braithwaite SS, Preiser JC. Stress hyperglycaemia. Lancet. (2009) 373:1798–807. doi: 10.1016/S0140-6736(09)60553-5
5. Kruyt ND, Biessels GJ, de Haan RJ, Vermeulen M, Rinkel GJ, Coert B, et al. Hyperglycemia and clinical outcome in aneurysmal subarachnoid hemorrhage: a meta-analysis. Stroke. (2009) 40:e424–30. doi: 10.1161/STROKEAHA.108.529974
6. Kruyt ND, Biessels GJ, DeVries JH, Luitse MJ, Vermeulen M, Rinkel GJ, et al. Hyperglycemia in aneurysmal subarachnoid hemorrhage: a potentially modifiable risk factor for poor outcome. J Cereb Blood Flow Metab. (2010) 30:1577–87. doi: 10.1038/jcbfm.2010.102
7. Eagles ME, Newton BD, Rosgen BK, Ayling OGS, Muram S, Tso MK, et al. Optimal glucose target after aneurysmal subarachnoid hemorrhage: a matched cohort study. Neurosurgery. (2021) 90:340–6. doi: 10.1227/NEU.0000000000001823
8. van den Berghe G, Wouters P, Weekers F, Verwaest C, Bruyninckx F, Schetz M, et al. Intensive insulin therapy in critically ill patients. N Engl J Med. (2001) 345:1359–67. doi: 10.1056/NEJMoa011300
9. Finfer S, Chittock DR, Su SY, Blair D, Foster D, Dingra V, et al. Intensive versus conventional glucose control in critically ill patients. N Engl J Med. (2009) 360:1283–97. doi: 10.1056/NEJMoa0810625
10. Bilotta F, Spinelli A, Giovannini F, Doronzio A, Delfini R, Rosa G. The effect of intensive insulin therapy on infection rate, vasospasm, neurologic outcome, and mortality in neurointensive care unit after intracranial aneurysm clipping in patients with acute subarachnoid hemorrhage: a randomized prospective pilot trial. J Neurosurg Anesthesiol. (2007) 19:156–60. doi: 10.1097/ANA.0b013e3180338e69
11. Latorre JG, Chou SH, Nogueira RG, Singhal AB, Carter BS, Ogilvy CS, et al. Effective glycemic control with aggressive hyperglycemia management is associated with improved outcome in aneurysmal subarachnoid hemorrhage. Stroke. (2009) 40:1644–52. doi: 10.1161/STROKEAHA.108.535534
12. Schmutzhard E, Rabinstein AA. Participants in the international multi-disciplinary consensus conference on the critical care management of subarachnoid hemorrhage. Spontaneous subarachnoid hemorrhage and glucose management. Neurocrit Care. (2011) 15:281–6. doi: 10.1007/s12028-011-9601-0
13. Kramer AH, Roberts DJ, Zygun DA. Optimal glycemic control in neurocritical care patients: a systematic review and meta-analysis. Crit Care. (2012) 16:R203. doi: 10.1186/cc11812
14. Barletta JF, Figueroa BE, DeShane R, Blau SA, McAllen KJ. High glucose variability increases cerebral infarction in patients with spontaneous subarachnoid hemorrhage. J Crit Care. (2013) 28:798–803. doi: 10.1016/j.jcrc.2012.12.012
15. Kurtz P, Claassen J, Helbok R, Schmidt J, Fernandez L, Presciutti M, et al. Systemic glucose variability predicts cerebral metabolic distress and mortality after subarachnoid hemorrhage: a retrospective observational study. Crit Care. (2014) 18:R89. doi: 10.1186/cc13857
16. Okazaki T, Hifumi T, Kawakita K, Shishido H, Ogawa D, Okauchi M, et al. Blood glucose variability: a strong independent predictor of neurological outcomes in aneurysmal subarachnoid hemorrhage. J Intensive Care Med. (2018) 33:189–95. doi: 10.1177/0885066616669328
17. Hill NR, Oliver NS, Choudhary P, Levy JC, Hindmarsh P, Matthews DR. Normal reference range for mean tissue glucose and glycemic variability derived from continuous glucose monitoring for subjects without diabetes in different ethnic groups. Diabetes Technol Ther. (2011) 13:921–8. doi: 10.1089/dia.2010.0247
19. Wallia A, Umpierrez GE, Rushakoff RJ, Klonoff DC, Rubin DJ, Hill Golden S, et al. Consensus statement on inpatient use of continuous glucose monitoring. J Diabetes Sci Technol. (2017) 11:1036–44. doi: 10.1177/1932296817706151
20. Danne T, Nimri R, Battelino T, Bergenstal RM, Close KL, DeVries JH, et al. International consensus on use of continuous glucose monitoring. Diabetes Care. (2017) 40:1631–40. doi: 10.2337/dc17-1600
21. Aragon D. Evaluation of nursing work effort and perceptions about blood glucose testing in tight glycemic control. Am J Crit Care. (2006) 15:370–7. doi: 10.4037/ajcc2006.15.4.370
22. Boom DT, Sechterberger MK, Rijkenberg S, Kreder S, Bosman RJ, Wester JP, et al. Insulin treatment guided by subcutaneous continuous glucose monitoring compared to frequent point-of-care measurement in critically ill patients: a randomized controlled trial. Crit Care. (2014) 18:453. doi: 10.1186/s13054-014-0453-9
23. Nukui S, Akiyama H, Soga K, Takao N, Tsuchihashi Y, Iijima N, et al. Risk of hyperglycemia and hypoglycemia in patients with acute ischemic stroke based on continuous glucose monitoring. J Stroke Cerebrovasc Dis. (2019) 28:104346. doi: 10.1016/j.jstrokecerebrovasdis.2019.104346
24. Palaiodimou L, Lioutas VA, Lambadiari V, Theodorou A, Themistocleous M, Aponte L, et al. Glycemic variability of acute stroke patients and clinical outcomes: a continuous glucose monitoring study. Ther Adv Neurol Disord. (2021) 14:17562864211045876. doi: 10.1177/17562864211045876
25. Kosiborod M, Gottlieb RK, Sekella JA, Peterman D, Grodzinsky A, Kennedy P, et al. Performance of the medtronic sentrino continuous glucose management (CGM) system in the cardiac intensive care unit. BMJ Open Diabetes Res Care. (2014) 2:e000037. doi: 10.1136/bmjdrc-2014-000037
26. Brunner R, Adelsmayr G, Herkner H, Madl C, Holzinger U. Glycemic variability and glucose complexity in critically ill patients: a retrospective analysis of continuous glucose monitoring data. Crit Care. (2012) 16:R175. doi: 10.1186/cc11657
27. Holzinger U, Warszawska J, Kitzberger R, Wewalka M, Miehsler W, Herkner H, et al. Real-time continuous glucose monitoring in critically ill patients: a prospective randomized trial. Diabetes Care. (2010) 33:467–72. doi: 10.2337/dc09-1352
28. Kopecký P, Mráz M, Bláha J, Lindner J, Svačina S, Hovorka R, et al. The use of continuous glucose monitoring combined with computer-based eMPC algorithm for tight glucose control in cardiosurgical ICU. Biomed Res Int. (2013) 2013:186439. doi: 10.1155/2013/186439
29. Price C, Ditton G, Russell GB, Aloi J. Reliability of inpatient CGM: comparison to standard of care. J Diabetes Sci Technol. (2021) 15:19322968211062168. doi: 10.1177/19322968211062168
30. Terpolilli NA, Brem C, Bühler D, Plesnila N. Are we barking up the wrong vessels? Cerebral microcirculation after subarachnoid haemorrhage. Stroke. (2015) 46:3014–9. doi: 10.1161/STROKEAHA.115.006353
31. van der Kleij LA, De Vis JB, Olivot JM, Calviere L, Cognard C, Zuithoff NP, et al. Magnetic resonance imaging and cerebral ischemia after aneurysmal subarachnoid hemorrhage: a systematic review and meta-analysis. Stroke. (2017) 48:239–45. doi: 10.1161/STROKEAHA.116.011707
32. Frontera JA, Ahmed W, Zach V, Jovine M, Tanenbaum L, Sehba F, et al. Acute ischaemia after subarachnoid haemorrhage, relationship with early brain injury and impact on outcome: a prospective quantitative MRI study. J Neurol Neurosurg Psychiatry. (2015) 86:71–8. doi: 10.1136/jnnp-2013-307313
33. Macdonald RL. Delayed neurological deterioration after subarachnoid haemorrhage. Nat Rev Neurol. (2014) 10:44–58. doi: 10.1038/nrneurol.2013.246
34. Neifert SN, Chapman EK, Martini ML, Shuman WH, Schupper AJ, Oermann EK, et al. Aneurysmal subarachnoid hemorrhage: the last decade. Transl Stroke Res. (2021) 12:428–46. doi: 10.1007/s12975-020-00867-0
35. Geraghty JR, Davis JL, Testai FD. Neuroinflammation and microvascular dysfunction after experimental subarachnoid hemorrhage: emerging components of early brain injury related to outcome. Neurocrit Care. (2019) 31:373–89. doi: 10.1007/s12028-019-00710-x
36. Rass V, Helbok R. Early brain injury after poor-grade subarachnoid hemorrhage. Curr Neurol Neurosci Rep. (2019) 19:78. doi: 10.1007/s11910-019-0990-3
37. Osgood ML. Aneurysmal subarachnoid hemorrhage: review of the pathophysiology and management strategies. Curr Neurol Neurosci Rep. (2021) 21:50. doi: 10.1007/s11910-021-01136-9
38. Marzatico F, Gaetani P, Cafè C, Spanu G, Baena RRy. Antioxidant enzymatic activities after experimental subarachnoid hemorrhage in rats. Acta Neurol Scand. (1993) 87:62–6. doi: 10.1111/j.1600-0404.1993.tb04077.x
39. Gaetani P, Pasqualin A, Baena RRy, Borasio E, Marzatico F. Oxidative stress in the human brain after subarachnoid haemorrhage. J Neurosurg. (1998) 89:748–54. doi: 10.3171/jns.1998.89.5.0748
40. Kamii H, Kato I, Kinouchi H, Chan PH, Epstein CJ, Akabane A, et al. Amelioration of vasospasm after subarachnoid hemorrhage in transgenic mice overexpressing CuZn-superoxide dismutase. Stroke. (1999) 30:867–71. doi: 10.1161/01.STR.30.4.867
41. Endo H, Nito C, Kamada H, Yu F, Chan PH. Reduction in oxidative stress by superoxide dismutase overexpression attenuates acute brain injury after subarachnoid hemorrhage via activation of Akt/glycogen synthase kinase-3beta survival signaling. J Cereb Blood Flow Metab. (2007) 27:975–82. doi: 10.1038/sj.jcbfm.9600399
42. Lee JY, Keep RF, He Y, Sagher O, Hua Y, Xi G. Hemoglobin and iron handling in brain after subarachnoid hemorrhage and the effect of deferoxamine on early brain injury. J Cereb Blood Flow Metab. (2010) 30:1793–803. doi: 10.1038/jcbfm.2010.137
43. Huang YH, Chung CL, Tsai HP, Tzou RD, Wu SC, Chai CY, et al. Impact of hyperglycemia on neuronal apoptosis after subarachnoid hemorrhage in rodent brain: an experimental research. Int J Surg. (2020) 83:246–52. doi: 10.1016/j.ijsu.2020.07.009
44. Li W, Maloney RE, Aw TY. High glucose, glucose fluctuation and carbonyl stress enhance brain microvascular endothelial barrier dysfunction: implications for diabetic cerebral microvasculature. Redox Biol. (2015) 5:80–90. doi: 10.1016/j.redox.2015.03.005
45. Stentz FB, Umpierrez GE, Cuervo R, Kitabchi AE. Proinflammatory cytokines, markers of cardiovascular risks, oxidative stress, and lipid peroxidation in patients with hyperglycemic crises. Diabetes. (2004) 53:2079–86. doi: 10.2337/diabetes.53.8.2079
46. Yu T, Robotham JL, Yoon Y. Increased production of reactive oxygen species in hyperglycemic conditions requires dynamic change of mitochondrial morphology. Proc Natl Acad Sci USA. (2006) 103:2653–8. doi: 10.1073/pnas.0511154103
47. Cipolla MJ, Huang Q, Sweet JG. Inhibition of protein kinase Cβ reverses increased blood-brain barrier permeability during hyperglycemic stroke and prevents edema formation in vivo. Stroke. (2011) 42:3252–7. doi: 10.1161/STROKEAHA.111.623991
48. Shah GN, Price TO, Banks WA, Morofuji Y, Kovac A, Ercal N, et al. Pharmacological inhibition of mitochondrial carbonic anhydrases protects mouse cerebral pericytes from high glucose-induced oxidative stress and apoptosis. J Pharmacol Exp Ther. (2013) 344:637–45. doi: 10.1124/jpet.112.201400
49. Shah GN, Morofuji Y, Banks WA, Price TO. High glucose-induced mitochondrial respiration and reactive oxygen species in mouse cerebral pericytes is reversed by pharmacological inhibition of mitochondrial carbonic anhydrases: implications for cerebral microvascular disease in diabetes. Biochem Biophys Res Commun. (2013) 440:354–8. doi: 10.1016/j.bbrc.2013.09.086
50. Huang YH, Chung CL, Tsai HP, Wu SC, Chang CZ, Chai CY, et al. Hyperglycemia aggravates cerebral vasospasm after subarachnoid hemorrhage in a rat model. Neurosurgery. (2017) 80:809–15. doi: 10.1093/neuros/nyx016
51. Helbok R, Schmidt JM, Kurtz P, Hanafy KA, Fernandez L, Stuart RM, et al. Systemic glucose and brain energy metabolism after subarachnoid hemorrhage. Neurocrit Care. (2010) 12:317–23. doi: 10.1007/s12028-009-9327-4
52. Katsura K, Ekholm A, Siesjö BK. Coupling among changes in energy metabolism, acid-base homeostasis, and ion fluxes in ischemia. Can J Physiol Pharmacol. (1992) 70 Suppl. S170–5. doi: 10.1139/y92-259
53. Parsons MW, Barber PA, Desmond PM, Baird TA, Darby DG, Byrnes G, et al. Acute hyperglycemia adversely affects stroke outcome: a magnetic resonance imaging and spectroscopy study. Ann Neurol. (2002) 52:20–8. doi: 10.1002/ana.10241
54. Frontera JA, Fernandez A, Claassen J, Schmidt M, Schumacher HC, Wartenberg K, et al. Hyperglycemia after SAH: predictors, associated complications, and impact on outcome. Stroke. (2006) 37:199–203. doi: 10.1161/01.STR.0000194960.73883.0f
55. Naidech AM, Levasseur K, Liebling S, Garg RK, Shapiro M, Ault ML, et al. Moderate hypoglycemia is associated with vasospasm, cerebral infarction, and 3-month disability after subarachnoid hemorrhage. Neurocrit Care. (2010) 12:181–7. doi: 10.1007/s12028-009-9311-z
56. van Donkelaar CE, Dijkland SA, van den Bergh WM, Bakker J, Dippel DW, Nijsten MW, et al. Early circulating lactate and glucose levels after aneurysmal subarachnoid hemorrhage correlate with poor outcome and delayed cerebral ischemia: a two-center cohort study. Crit Care Med. (2016) 44:966–72. doi: 10.1097/CCM.0000000000001569
57. Pappacena S, Bailey M, Cabrini L, Landoni G, Udy A, Pilcher DV, et al. Early dysglycemia and mortality in traumatic brain injury and subarachnoid hemorrhage. Minerva Anestesiol. (2019) 85:830–9. doi: 10.23736/S0375-9393.19.13307-X
58. Jung HM, Paik JH, Kim SY, Hong DY. Association of plasma glucose to potassium ratio and mortality after aneurysmal subarachnoid hemorrhage. Front Neurol. (2021) 12:661689. doi: 10.3389/fneur.2021.661689
59. Liu D, Tang Y, Zhang Q. Admission hyperglycemia predicts long-term mortality in critically ill patients with subarachnoid hemorrhage: a retrospective analysis of the MIMIC-III database. Front Neurol. (2021) 12:678998. doi: 10.3389/fneur.2021.678998
60. Wang J, Feng Q, Zhang Y, Qiu W, Gao H. Elevated glucose-potassium ratio predicts preoperative rebleeding in patients with aneurysmal subarachnoid hemorrhage. Front Neurol. (2022) 12:795376. doi: 10.3389/fneur.2021.795376
61. McIntyre MK, Halabi M, Li B, Long A, Van Hoof A, Afridi A, et al. Glycemic indices predict outcomes after aneurysmal subarachnoid hemorrhage: a retrospective single center comparative analysis. Sci Rep. (2021) 11:158. doi: 10.1038/s41598-020-80513-9
62. Thiele RH, Pouratian N, Zuo Z, Scalzo DC, Dobbs HA, Dumont AS, et al. Strict glucose control does not affect mortality after aneurysmal subarachnoid hemorrhage. Anesthesiology. (2009) 110:603–10. doi: 10.1097/ALN.0b013e318198006a
63. Schlenk F, Graetz D, Nagel A, Schmidt M, Sarrafzadeh AS. Insulin-related decrease in cerebral glucose despite normoglycemia in aneurysmal subarachnoid hemorrhage. Crit Care. (2008) 12:R9. doi: 10.1186/cc6776
64. Beseoglu K, Steiger HJ. Elevated glycated hemoglobin level and hyperglycemia after aneurysmal subarachnoid hemorrhage. Clin Neurol Neurosurg. (2017) 163:128–32. doi: 10.1016/j.clineuro.2017.10.037
65. Sun PY, Poblete RA, Nguyen PL, Bulic SF, Kim-Tenser MA, Marehbian J, et al. Association between glycemic gap and in-hospital outcomes in aneurysmal subarachnoid hemorrhage. Front Neurol. (2021) 12:714341. doi: 10.3389/fneur.2021.714341
66. Matano F, Fujiki Y, Mizunari T, Koketsu K, Tamaki T, Murai Y, et al. Serum glucose and potassium ratio as risk factors for cerebral vasospasm after aneurysmal subarachnoid hemorrhage. J Stroke Cerebrovasc Dis. (2019) 28:1951–7. doi: 10.1016/j.jstrokecerebrovasdis.2019.03.041
67. Zhang D, Zhuang Z, Wei Y, Liu X, Li W, Gao Y, et al. Association of admission serum glucose-phosphate ratio with severity and prognosis of aneurysmal subarachnoid hemorrhage. World Neurosurg. (2019) 127:e1145–51. doi: 10.1016/j.wneu.2019.04.071
68. Egi M, Bellomo R, Stachowski E, French CJ, Hart G. Variability of blood glucose concentration and short-term mortality in critically ill patients. Anesthesiology. (2006) 105:244–52. doi: 10.1097/00000542-200608000-00006
69. Sadan O, Feng C, Vidakovic B, Mei Y, Martin K, Samuels O, et al. Glucose variability as measured by inter-measurement percentage change is predictive of in-patient mortality in aneurysmal subarachnoid hemorrhage. Neurocrit Care. (2020) 33:458–67. doi: 10.1007/s12028-019-00906-1
70. Helbok R, Kofler M, Schiefecker AJ, Gaasch M, Rass V, Pfausler B, et al. Clinical use of cerebral microdialysis in patients with aneurysmal subarachnoid hemorrhage-state of the art. Front Neurol. (2017) 8:565. doi: 10.3389/fneur.2017.00565
71. Zetterling M, Hillered L, Enblad P, Karlsson T, Ronne-Engström E. Relation between brain interstitial and systemic glucose concentrations after subarachnoid hemorrhage. J Neurosurg. (2011) 115:66–74. doi: 10.3171/2011.3.JNS10899
72. Williams LS, Rotich J, Qi R, Fineberg N, Espay A, Bruno A, et al. Effects of admission hyperglycemia on mortality and costs in acute ischemic stroke. Neurology. (2002) 59:67–71. doi: 10.1212/WNL.59.1.67
73. Ntaios G, Egli M, Faouzi M, Michel P. J-shaped association between serum glucose and functional outcome in acute ischemic stroke. Stroke. (2010) 41:2366–70. doi: 10.1161/STROKEAHA.110.592170
74. Baird TA, Parsons MW, Phan T, Butcher KS, Desmond PM, Tress BM, et al. Persistent poststroke hyperglycemia is independently associated with infarct expansion and worse clinical outcome. Stroke. (2003) 34:2208–14. doi: 10.1161/01.STR.0000085087.41330.FF
75. Lim JS, Kim C, Oh MS, Lee JH, Jung S, Jang MU, et al. Effects of glycemic variability and hyperglycemia in acute ischemic stroke on post-stroke cognitive impairments. J Diabetes Complications. (2018) 32:682–7. doi: 10.1016/j.jdiacomp.2018.02.006
76. Otero-Ortega L, Gutiérrez-Fernández M, Gutiérrez-Zúñiga R, Madero-Jarabo R, Alonso de Leciñana M, Laso-García F, et al. The effect of post-stroke hyperglycaemia on the levels of brain damage and repair-related circulating biomarkers: the glycaemia in acute stroke study II. Eur J Neurol. (2019) 26:1439–46. doi: 10.1111/ene.14010
77. Paciaroni M, Agnelli G, Caso V, Corea F, Ageno W, Alberti A, et al. Acute hyperglycemia and early hemorrhagic transformation in ischemic stroke. Cerebrovasc Dis. (2009) 28:119–23. doi: 10.1159/000223436
78. Poppe AY, Majumdar SR, Jeerakathil T, Ghali W, Buchan AM, Hill MD. Admission hyperglycemia predicts a worse outcome in stroke patients treated with intravenous thrombolysis. Diabetes Care. (2009) 32:617–22. doi: 10.2337/dc08-1754
79. Saqqur M, Shuaib A, Alexandrov AV, Sebastian J, Khan K, Uchino K. The correlation between admission blood glucose and intravenous rt-PA-induced arterial recanalization in acute ischemic stroke: a multi-centre TCD study. Int J Stroke. (2015) 10:1087–92. doi: 10.1111/ijs.12517
80. Osei E, Fonville S, Zandbergen AAM, Koudstaal PJ, Dippel DWJ, den Hertog HM. Impaired fasting glucose is associated with unfavorable outcome in ischemic stroke patients treated with intravenous alteplase. J Neurol. (2018) 265:1426–31. doi: 10.1007/s00415-018-8866-z
81. Cai Y, Zhang H, Li Q, Zhang P. Correlation between blood glucose variability and early therapeutic effects after intravenous thrombolysis with alteplase and levels of serum inflammatory factors in patients with acute ischemic stroke. Front Neurol. (2022) 13:806013. doi: 10.3389/fneur.2022.806013
82. Kim JT, Jahan R, Saver JL. SWIFT investigators. Impact of glucose on outcomes in patients treated with mechanical thrombectomy: a post hoc analysis of the solitaire flow restoration with the intention for thrombectomy study. Stroke. (2016) 47:120–7. doi: 10.1161/STROKEAHA.115.010753
83. Osei E, den Hertog HM, Berkhemer OA, Fransen PSS, Roos YBWEM, Beumer D, et al. Admission glucose and effect of intra-arterial treatment in patients with acute ischemic stroke. Stroke. (2017) 48:1299–305. doi: 10.1161/STROKEAHA.116.016071
84. Goyal N, Tsivgoulis G, Pandhi A, Dillard K, Katsanos AH, Magoufis G, et al. Admission hyperglycemia and outcomes in large vessel occlusion strokes treated with mechanical thrombectomy. J Neurointerv Surg. (2018) 10:112–7. doi: 10.1136/neurintsurg-2017-012993
85. Chamorro Á, Brown S, Amaro S, Hill MD, Muir KW, Dippel DWJ, et al. Glucose modifies the effect of endovascular thrombectomy in patients with acute stroke. Stroke. (2019) 50:690–6. doi: 10.1161/STROKEAHA.118.023769
86. Godoy DA, Piñero GR, Svampa S, Papa F, Di Napoli M. Hyperglycemia and short-term outcome in patients with spontaneous intracerebral hemorrhage. Neurocrit Care. (2008) 9:217–29. doi: 10.1007/s12028-008-9063-1
87. Saxena A, Anderson CS, Wang X, Sato S, Arima H, Chan E, et al. Prognostic significance of hyperglycemia in acute intracerebral hemorrhage: the INTERACT2 study. Stroke. (2016) 47:682–8. doi: 10.1161/STROKEAHA.115.011627
88. Lin J, Cai C, Xie Y, Yi L. Acute glycemic variability and mortality of patients with acute stroke: a meta-analysis. Diabetol Metab Syndr. (2022) 14:69. doi: 10.1186/s13098-022-00826-9
89. Ribo M, Molina CA, Delgado P, Rubiera M, Delgado-Mederos R, Rovira A, et al. Hyperglycemia during ischemia rapidly accelerates brain damage in stroke patients treated with tPA. J Cereb Blood Flow Metab. (2007) 27:1616–22. doi: 10.1038/sj.jcbfm.9600460
90. Shimoyama T, Kimura K, Uemura J, Saji N, Shibazaki K. Post stroke dysglycemia and acute infarct volume growth: a study using continuous glucose monitoring. Eur Neurol. (2016) 76:167–74. doi: 10.1159/000448329
91. Wada S, Yoshimura S, Inoue M, Matsuki T, Arihiro S, Koga M, et al. Outcome prediction in acute stroke patients by continuous glucose monitoring. J Am Heart Assoc. (2018) 7:e008744. doi: 10.1161/JAHA.118.008744
92. Palaiodimou L, Lioutas VA, Lambadiari V, Paraskevas GP, Voumvourakis K, Tsivgoulis G. Glycemia management in acute ischemic stroke: current concepts and novel therapeutic targets. Postgrad Med. (2019) 131:423–37. doi: 10.1080/00325481.2019.1651206
Keywords: subarachnoid hemorrhage, early brain injury, continuous glucose monitoring, stress hyperglycemia, glycemic variability, glucose profile
Citation: Santana D, Mosteiro A, Pedrosa L, Llull L, Torné R and Amaro S (2022) Clinical relevance of glucose metrics during the early brain injury period after aneurysmal subarachnoid hemorrhage: An opportunity for continuous glucose monitoring. Front. Neurol. 13:977307. doi: 10.3389/fneur.2022.977307
Received: 24 June 2022; Accepted: 25 August 2022;
Published: 12 September 2022.
Edited by:
Isabel Fragata, New University of Lisbon, PortugalReviewed by:
Sandra Magnoni, IRCCS Ca 'Granda Foundation Maggiore Policlinico Hospital, ItalyCopyright © 2022 Santana, Mosteiro, Pedrosa, Llull, Torné and Amaro. This is an open-access article distributed under the terms of the Creative Commons Attribution License (CC BY). The use, distribution or reproduction in other forums is permitted, provided the original author(s) and the copyright owner(s) are credited and that the original publication in this journal is cited, in accordance with accepted academic practice. No use, distribution or reproduction is permitted which does not comply with these terms.
*Correspondence: Ramón Torné, torne@clinic.cat; Sergi Amaro, samaro@clinic.cat
†These authors have contributed equally to this work and share first authorship