- 1Department of Neurology, Kiel University, Kiel, Germany
- 2Department of Neuroscience, Mental Health and Sensory Organs (NESMOS), Sapienza University of Rome, Rome, Italy
- 3Division of Surgery, Saarland University, Homburg, Germany
- 4Faculty of Engineering, Kiel University, Kiel, Germany
- 5Santa Lucia Foundation, Istituto di Ricovero e Cura a Carattere Scientifico (IRCCS), Rome, Italy
Introduction: Dual-tasking (DT) while walking is common in daily life and can affect both gait and cognitive performance depending on age, attention prioritization, task complexity and medical condition. The aim of the present study was to investigate the effects of DT on cognitive DT cost (DTC) (i) in a dataset including participants of different age groups, with different neurological disorders and chronic low-back pain (cLBP) (ii) at different levels of cognitive task complexity, and (iii) in the context of a setting relevant to daily life, such as combined straight walking and turning.
Materials and methods: Ninety-one participants including healthy younger and older participants and patients with Parkinson's disease, Multiple Sclerosis, Stroke and cLBP performed a simple reaction time (SRT) task and three numerical Stroop tasks under the conditions congruent (StC), neutral (StN) and incongruent (StI). The tasks were performed both standing (single task, ST) and walking (DT), and DTC was calculated. Mixed ANOVAs were used to determine the effect of group and task complexity on cognitive DTC.
Results: A longer response time in DT than in ST was observed during SRT. However, the response time was shorter in DT during StI. DTC decreased with increasing complexity of the cognitive task. There was no significant effect of age and group on cognitive DTC.
Conclusion: Our results suggest that regardless of age and disease group, simple cognitive tasks show the largest and most stable cognitive effects during DT. This may be relevant to the design of future observational studies, clinical trials and for clinical routine.
Introduction
In daily life, it is very common to encounter situations that require performing two tasks simultaneously, such as walking and talking on the phone. In research conditions, this is referred to as dual-task (DT), as opposed to single-task (ST). In particular, a large body of research has focused on the interaction between cognitive tasks (e.g., serial subtractions) and motor tasks (e.g., walking), showing that DT can affect the performance of one or even both of the tasks involved compared to ST (1). The effect of DT can be calculated as the percentage change in the parameters on interest between DT and ST and has been termed DT cost (DTC). A positive value of DTC means that performance worsens in DT compared with ST and vice versa (1). In motor-cognitive DT paradigms, DTC can be calculated for both cognitive and motor tasks, reflecting an improvement or decrease in either motor or cognitive performance or both. The DTC has been widely used to study the interaction between movement and cognition as a reliable measure of the effect of DT in both physiological (2) and pathological conditions (3–5). In addition, it has been shown to be a useful outcome measure for assessing response to training programs and rehabilitation interventions (6–10). Indeed, a reduction in DTC reflects better motor-cognitive integration and attentional capacity (1).
Walking is one of the most common motor activities performed daily, and a large number of situations involving DT occur during walking. In addition, factors that negatively affect the ability to walk, such as neurological disorders, orthopedic impairments, and balance disorders, have a profound impact on people's independence and quality of life (11). Several studies have reported deterioration in gait parameters, such as reduced gait speed (4, 12–14), cadence (4, 12) and increased gait variability (4, 12, 15, 16), in DT compared with ST. These effects can be observed in different age groups such as healthy older (12) and younger adults (16), and disease populations such as Parkinson's Disease (PD) (14), Multiple Sclerosis (MS) (13), stroke (4) and chronic low-back pain (cLBP) patients (15). Several mechanisms have been proposed to explain the effect of DT, such as attention allocation theory and the bottleneck theory (1). The attention allocation theory states that people have a limited attention capacity. When, as in multitasking, attention demands exceed this capacity, performance on one or more of the simultaneously performed tasks decreases. The bottleneck theory states that above a certain threshold of processing load, only one task can be completed at a time due to limited cognitive resources, resulting in a decline in performance (1). However, many studies have reported no relevant or even negative cognitive DTC, both in healthy participants (17–23) and in patients with cLBP (15) and neurological conditions (24–31). Based on these observations, Plummer and colleagues proposed a classification of the motor-cognitive interactions of DT into 9 possible outcomes based on the combination of effects (i.e., improvement, no change or worsening compared with ST) in cognitive and motor performance, respectively (32). The variability in the results reflects the complexity of interactions between motor and cognitive tasks, and several explanations have been proposed. One possible explanation is that the two tasks do not compete for the same resource pool, resulting in little or no interference between the two. Another hypothesis suggests that a cross-talk interaction might exist and that the two tasks interact in a facilitative manner when they derive from similar cognitive domains and use similar neural populations (1, 33).
Another factor involved in the interaction between gait and cognitive tasks is task complexity, but only a few studies have investigated its influence on cognitive DTC, with variable results. Some studies have reported higher cognitive DTC as cognitive task complexity increases (34–36). The effect seems to be influenced by age and cognitive domains involved (35–39). However, another study did not find this effect (40).
Requiring demanding attentional skills and the integrity of executive functions (1), DT has been used to study cognitive and motor interaction in various physiological and pathological conditions, such as aging, neurological disorders and cLBP. It has been reported that older participants have a reduced ability to process multiple tasks simultaneously, resulting in a higher cognitive DTC compared with younger adults (2) and this is probably related to reduced processing speed and cognitive reserve (37, 41, 42). Some studies have also investigated the effect of motor-cognitive DT on cognitive DTC in various neurological disorders such as PD (43), MS (6, 44) and stroke (4). However, these studies have focused only on single neurologic conditions or age groups.
Recently, cLBP has been shown to be associated with impaired attention and working memory (45) and altered connectivity of the attentional network (46), and DT have led to impairment of gait and balance parameters in these patients (15, 47, 48). Only one study has reported data on cognitive DTC in patients with cLBP, showing little or no effect of DT on verbal fluency (15). However, it is unclear whether more challenging concomitant cognitive tasks can unmask an effect of DT on cognitive performance. Based on clinical experience, we hypothesized that this cohort might show low DTC or even an improvement in cognitive performance in DT with more challenging cognitive tasks due to a pain distraction effect.
Therefore, the aim of the present study was to investigate the effects of DT on cognitive DTC (i) in a dataset including participants of different age groups, with different neurological conditions and cLBP, (ii) at different levels of cognitive task complexity, and (iii) in the context of a setting relevant to daily life, such as combined straight walking and turning. The idea for the experimental paradigm was based on the fact that short walking episodes combined with turns better reflect everyday situations than the straight walking paradigms used in previous studies (49) and that we assumed that such combined straight walking and turning movements are often performed under DT conditions in daily life.
Methods
In the present study we analyzed a dataset from a cross-sectional study designed to develop and validate mobility algorithms. This included participants with different age groups, with different neurological disorders and cLBP. Detail of the dataset protocol can be found in (50).
Population
Participants were longitudinally recruited through flyers placed in public facilities (healthy participants) and in neurology departments and outpatient clinics at University Hospital Schleswig-Holstein (UKSH), Kiel Campus, Germany (neurological patients). Inclusion criteria were (i) age 18 years or older and (ii) ability to walk independently without walking aids. Exclusion criteria were (i) Montreal Cognitive Assessment score <15 and (ii) other movement disorders affecting mobility performance, as judged by the assessor. Participants were divided into 6 groups according to age and diagnosis. Healthy participants were divided into “younger” (age 18–45 years) and “older” (age ≥ 60 years). Participants with neurological disorders included patients with PD [according to the UK Brain Bank criteria (51)], MS [according to McDonalds criteria (52)], recent symptomatic stroke (<4 weeks), and cLBP, diagnosed on the basis of the patient's medical history and examination (53).
Ethics
The study was approved by the ethical committee of the Medical Faculty of Kiel University (D438/18) and was conducted in accordance with the principles of the Declaration of Helsinki. All participants provided written informed consent before the start of measurements. The study is registered in the German Clinical Trials Register (DRKS00022998).
Demographic and clinical data
Demographic data including age, sex, weight, and height were collected. Overall cognitive function was assessed with the Montreal Cognitive Assessment (54). Mobility was assessed with the Short Physical Performance Battery (SPPB) (55). Disease-specific evaluations included the MDS Unified Parkinson's Disease Rating Scale part III (MDS-UPDRS-III) (56) and the Hoehn and Yahr Scale (57) for PD patients; the Expanded Disability Status Scale (58) for MS patients; the NIH Stroke Scale (59) for patients with stroke; a visual analog scale of pain (60) and the German Funktionsfragenbogen Hannover Scale (61) for patients with cLBP.
Experimental procedure
Participants performed two smartphone-based cognitive tasks of different complexity. The first task consisted of a simple reaction time test (SRT) in which participants had to tap the screen as fast as possible after the appearance of a black square. Six time intervals ranging from 1,000 to 2,000 ms (in 200 ms increments) were used for the appearance of the black square. Each condition appeared 4 times for a total of 24 trials, administered in random order. The response time in ms was recorded for each trial, and the average of all trials was calculated for each participant. The second task consisted of a numerical Stroop test in which participants had to choose the higher number from two options (62). The Stroop test was administered in 3 conditions: (i) Congruent, in which the number with a higher value had a larger character size (StC); (ii) Neutral, in which the character size of both numbers was equal (StN); (iii) Incongruent, in which the number with a higher value had a smaller character size (StI). Each condition consisted of 8 trials for a total of 24 total trials in random order. The SRT and Stroop tasks were performed consecutively. Response times were recorded in ms and the average for each condition was calculated for each participant. Both the SRT and Stroop numerical tests were performed on a smartphone while standing quietly (ST condition) and while walking up and down a 5-meter walkway (DT condition). For the DT condition, participants were not given any instruction regarding task prioritization.
Movement and rotation analysis
Participants were measured with a 3D optical motion capture system (Qualisys AB, Gothenburg, Sweden). Reflective markers were placed on the head, sternum, pelvis, and feet. The exact placement of the markers is described elsewhere (50). Data were recorded at 200 Hz. To detect turns during walking, pelvis angles were calculated according to Euler's method. The beginning and end of a turn were detected by the change in the standard deviation of the angular signal around the vertical axis, using the Matlab's built-in function findchangepts.
Data analyses
Turning phases were extracted by the method described above, the total time spent during turning was computed and used to calculate the turning ratio according to the following formula:
To assess the impact of DT on cognitive performance, DTC was calculated using the following formula (63, 64):
Statistical analysis
Statistical analyses were performed using JASP v0.16.1 (JASP Team, University of Amsterdam), R v4.0.3 and RStudio v2022.02.2+433 for Windows (R Foundation for Statistical Computing, Vienna, Austria). Descriptive statistics were calculated for the examined variables. To assess the difference in DTC across the 6 groups and different cognitive tasks, a mixed ANOVA test was used, with the factors “task” and “group.” To assess the difference in response times across the 6 groups between ST and DT, separate mixed ANOVA tests were used, one for each cognitive task, with the factors “condition” and “group.” Post-hoc t-tests with Bonferroni's correction for multiple tests were performed in case of significant ANOVA main effects. If necessary, Greenhouse-Geisser's correction for non-sphericity were applied. Partial eta squared () were reported as measure of effect size. The significance threshold was set at α <0.05. All data were reported as mean ± SD or median (Q1–Q3) for numerical data and N (%) for categorical variables.
Results
A total of 91 participants were enrolled in the study. Table 1 shows the demographic and clinical data of the included population. The average turning ratio was 23%.
Effect of complexity of the cognitive tasks
Mixed ANOVA showed a significant effect of factor “task” [F(3, 177) = 48.630; p < 0.001; = 0.452] (Figure 1) and post-hoc analysis showed significantly higher cognitive DTC in SRT than in all Stroop conditions (all p < 0.001), with no significant differences among these in the overall group and in all subgroups except in patients with cLBP (Figure 2).
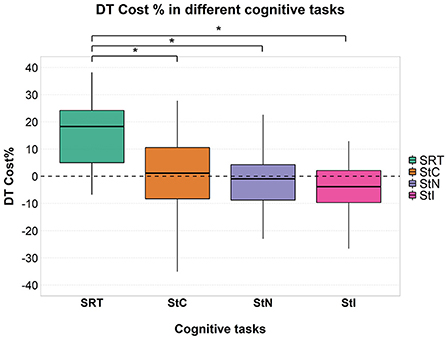
Figure 1. Cognitive DTC in each cognitive task. A negative value means a reduced response time and a consequently better performance and vice versa. A dashed line to mark the zero was added. Significant pairwise comparisons are marked with an asterisk. SRT, Simple Reaction Time; StC, Congruent Stroop Test; StN, Neutral Stroop Test; StI, Incongruent Stroop Test.
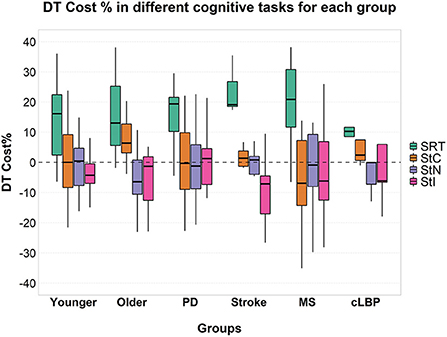
Figure 2. Cognitive DTC across the different cognitive tasks in each group. A negative value means a reduced response time and a consequently better performance and vice versa. A dashed line to mark the zero was added. SRT, Simple Reaction Time; StC, Congruent Stroop Test; StN, Neutral Stroop Test; StI, Incongruent Stroop Test.
A significant effect of factor “condition” [F(1, 85) = 71.999; p < 0.001; = 0.459] was found on the response time for the SRT task with an overall higher response time in DT than in ST condition. Considering Stroop test, a significant effect of factor “condition” was found only for StI [F(1, 71) = 9.411; p = 0.003; = 0.117] with an overall decrease in response time in DT compared to ST condition. Post-hoc analysis showed that response time was significantly higher in DT than in ST in all groups in SRT. On the other hand, in StI, response time was significantly lower in DT than in ST only in the younger participants, while no significant difference was found in the other groups (Figure 3).
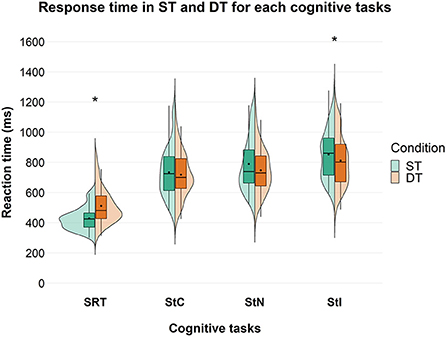
Figure 3. Response time in ms between ST and DT, across the different cognitive tasks in the overall group. Significant differences between ST and DT are marked with an asterisk. SRT, Simple Reaction Time; StC, Congruent Stroop Test; StN, Neutral Stroop Test; StI, Incongruent Stroop Test.
Cognitive DTC values for all groups in each cognitive task are shown in Supplementary Table 1.
Effect of age and disease group
No significant effects of factor “group” or interaction between the two factors were found on cognitive DTC (Figure 4).
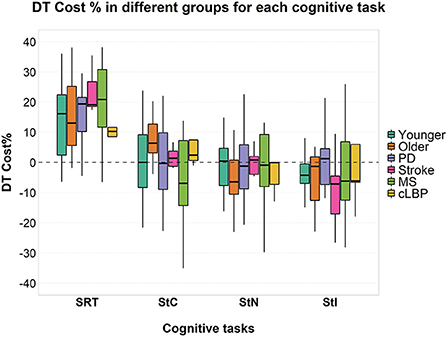
Figure 4. Cognitive DTC across the different groups in each cognitive task. A negative value means a reduced response time and a consequently better performance and vice versa. A dashed line to mark the zero was added. SRT, Simple Reaction Time; StC, Congruent Stroop Test; StN, Neutral Stroop Test; StI, Incongruent Stroop Test.
A significant effect of factor “group” was found [F(5, 85) = 8.000; p < 0.001; = 0.320] on the response time for the SRT task and a significant interaction between factor “condition” and “group” [F(5, 85) = 3.022; p = 0.015; = 0.151]. A significant effect of factor “group” was found for StC [F(5, 70) = 10.626; p < 0.001; = 0.432], StN [F(5, 68) = 10.663; p < 0.001; = 0.439] and StI [F(5, 71) = 15.337; p < 0.001; = 0.519]. No significant interaction was found between the two factors for all three conditions of Stroop test (Figure 4). Post-hoc pairwise comparisons for factor “group” and details of response time in ST and DT are shown in detail in Supplementary Table 2.
Discussion
This analysis on a cross-sectional dataset aimed to evaluate the effect of motor-cognitive DT at different cognitive task complexities on cognitive DTC during turning and walking in healthy participants, patients with different neurological conditions, and patients with cLBP. We found a significant effect of cognitive task complexity on cognitive DTC while age and disease did not have a significant effect on cognitive DTC in the population studied here. These results are discussed in detail in the following.
Effect of complexity of the cognitive tasks
Cognitive DTC depended on cognitive task complexity, both in the overall group and at the subgroup level, with DTC decreasing as task complexity increased. This is in contrast to what we expected. A significant difference in response time between ST and DT was found during SRT and StI, but not during StC and StN. During SRT, a higher response time was observed in DT, compared with ST (Figure 3) and this can be seen in all subgroups (Supplementary Table 2). In contrast, during StI, we observed a lower response time in DT, compared to ST (Figure 3). Our results on SRT are in line with previous studies showing a deterioration in reaction time when performing another task simultaneously, such as contralateral movements (65), a secondary cognitive task (66), a postural task (67) or walking (68, 69). However, previous studies have reported that cognitive DTC is highly variable depending on several factors such as attention (70), age and gender (18, 35, 36, 38, 71–74), task type and difficulty (35, 36, 39, 63, 70, 75), and these variables show a complex interaction. In addition, several studies have shown that cognitive performance in DT did not change compared to ST (17, 19–21) or even improved (17, 18, 20, 29). This has been demonstrated in both healthy participants and patients with neurological conditions and cLBP (15, 24–28, 30, 31). The opposite results between SRT and StI, found in this study, could be due to several mechanisms, such as (i) attention prioritization; (ii) the different cognitive functions involved in the two tasks; and (iii) the interaction between the learning processes within sessions and task complexity.
Attention and task prioritization have been shown to have an important effect on motor-cognitive DT involving walking (18, 24, 64, 67, 76). In our study, the task context was similar for each condition, but participants were not instructed to prioritize the cognitive task or walking and turning, thus they may have adopted a “cognitive first” strategy in StI and a “mobility first” strategy in SRT. A similar strategy adaptation has already been described for older adults (77). In accordance with our results, previous studies have also suggested a role of task complexity in the choice of different attentional strategies, with more complex cognitive tasks resulting in lower DTC and simpler cognitive tasks resulting in higher DTC (78).
SRT and Stroop tasks require different cognitive functions. The SRT is a measure of processing speed (79), while the Stroop test is a more complex task involving executive functions (80, 81). Previous studies have demonstrated an important role of executive functions in gait, and their impairment has been associated with reduced performance in walking tasks (82). According to the cross-talk model (1), the greater involvement of executive functions in both StI and walking could explain the lower DTC during this condition. In contrast, during SRT, the different cognitive functions involved might have led to a negative effect and a higher DTC with a significant difference compared to all Stroop conditions, both in the overall group (Figure 1) and at a the subgroup level (Figure 2).
As SRT is a simple task, it is also possible that the learning curve was so fast that DT effects were well-presented with this paradigm, but that the learning curve was substantially lower for the Stroop tasks which may have led to better results during the DT sessions (which were always performed after the ST sessions).
Effect of age and disease group
Differently from what we expected, we found no significant differences across groups in cognitive DTC (Figure 4). This result suggests that neurologic diseases and cLBP, at the level of severity and age included in the present study, might have no relevant effect on cognitive DTC. Previous findings have shown higher cognitive DTC in older adults compared with younger ones (2). This has been linked to reduced cognitive reserve and slowed processing capacity, with some cognitive domains (e.g., executive functions) more involved than others (37, 41, 42). The greater cognitive demands of walking in older participants may also play a role in these observations. Indeed, at a younger age, walking is a task with a high level of automaticity, whereas this decreases with age, leading to more active control and, consequently, a higher cognitive load (42). This is also demonstrated by the higher level of activation of the prefrontal cortex during walking with increasing age (83). Previous studies also reported higher cognitive DTC in PD (43, 84) and stroke patients (4) compared with healthy participants. This has been linked to physical and cognitive impairment related to these disorders and to reduced attention and cognitive reserve (85). In MS patients, on the other hand, studies have shown a negative effect of DT on cognitive performance (6, 86), but only a few studies have reported data on cognitive DTC and it remains unclear whether this differs between MS patients and healthy participants (3). Finally, only one study reported data on cognitive DTC in patients with cLBP showing little or no effect of DT on cognitive performance (15).
Finally, we found no interaction between the factor “complexity” and “group” on cognitive DTC. Previous studies have reported an influence of age on the effect of task complexity on DTC, with younger participants showing a significant DTC on cognitive performance only in the most challenging cognitive tasks, whereas older participants were reported to be more prone to DT effect at a lower level of cognitive task complexity (37). The domain-specific effect of DT on cognitive performance also appears to differ between younger and older adults (38, 39). The discrepancies between the previous results and ours might lie in the low disease severity of our patients and their overall good cognitive and physical function. Therefore, we might hypothesize that at the disease severity included in our study, the specific features of the disorders in terms of physical and cognitive impairment have little impact in cognitive DTC. However, most studies have focused on single conditions or age groups and, to our knowledge, there has been no study in which participants with different neurological conditions, cLBP and of different ages have been included in the same experimental protocol.
Limitations
We acknowledge that this study has limitations. First, the sample size of some groups, in particular the cLBP group, is low. This is explained by the basic design of the cross-sectional study, which provided the dataset used in the present article. However, we believe it is still useful to show data on the entire spectrum of diseases studied to open the possibility of generating new hypotheses for future studies. Second, participants had, on average, relatively high levels of physical and cognitive abilities. This may limit the generalizability of our results, and further studies including participants with lower functional scores may be needed. Third, in DT, participants were not instructed to prioritize any of the tasks. This could have led to different attentional strategies. However, we argue that this problem is difficult to avoid. For example, we cannot exclude that the instruction “Please do not prioritize any of the tasks” does not lead to the activation of another (cognitive) task during the experiment. Fourth, standing while performing a cognitive task might be considered an (additional) resource-consuming task. Indeed, this paradigm has been used in previous studies as a DT postural paradigm (87–89). In future studies, the possibility of performing cognitive tasks while sitting could be considered. Fifth, although none of the participants reported attentional deficit as a specific symptom, we did not assess this aspect in a standardized and structured way, thus an effect cannot theoretically be ruled out. Sixth, we focused our analysis on disease and age groups therefore we did not test the independent effect of age across neurological diseases and cLBP. However, we do not expect this to have substantially affected our data, as we consistently compared disease groups with both older and younger adults in our calculations. Similarly, we focused on the differences across groups without assessing the independent effect of disease severity or functional scores. Future studies will be useful to address this issue. Finally, ST and DT, as well as SRT and Stroop conditions, were performed consecutively. Thus, we cannot rule out that at least some improvement between ST and DT may be explained by a learning effect.
Conclusion
DT while walking has a different effect on cognition depending on the complexity of the cognitive task. Cognitive tasks that are more complex and involve executive functions showed lower cognitive DTC and even better cognitive performance in DT than in ST. The underlying mechanisms could involve task prioritization, cross-talk interaction, or a combination of learning processes and interference between the two tasks. Age and disease group did not have a relevant effect on cognitive DTC in our cohort. These findings could help movement disorder specialists, neurologists, general practitioners, allied health therapists and patients to interpret the interaction between cognitive abilities and walking in a context that more closely reflects everyday walking conditions, and could be useful to industry and academic principal investigators to design effective assessment batteries that focus on DTC.
Data availability statement
The raw data supporting the conclusions of this article will be made available by the authors, without undue reservation.
Ethics statement
The studies involving human participants were reviewed and approved by Ethical Committee of the Medical Faculty of Kiel University (D438/18). The patients/participants provided their written informed consent to participate in this study.
Author contributions
EW, CH, and WM designed the study. EW collected data. EW and EB performed data analyses. EB and WM wrote the first draft of the manuscript. EW, RR, CH, and FP reviewed manuscript draft. All authors contributed to the article and approved the submitted version.
Funding
This study was funded by the Open Access Publication fund of the Christian-Albrechts-University of Kiel for scientists of the CAU by Schleswig-Holstein state government. We acknowledge the financial support by DFG within the funding programme Open Access-Publikationskosten.
Acknowledgments
The authors sincerely thank all people that were involved in the data collection, from study participants to students who aided and assisted during assessments.
Conflict of interest
The authors declare that the research was conducted in the absence of any commercial or financial relationships that could be construed as a potential conflict of interest.
Publisher's note
All claims expressed in this article are solely those of the authors and do not necessarily represent those of their affiliated organizations, or those of the publisher, the editors and the reviewers. Any product that may be evaluated in this article, or claim that may be made by its manufacturer, is not guaranteed or endorsed by the publisher.
Supplementary material
The Supplementary Material for this article can be found online at: https://www.frontiersin.org/articles/10.3389/fneur.2022.964207/full#supplementary-material
References
1. Leone C, Feys P, Moumdjian L, D'Amico E, Zappia M, Patti F. Cognitive-motor dual-task interference: a systematic review of neural correlates. Neurosci Biobehav Rev. (2017) 75:348–60. doi: 10.1016/j.neubiorev.2017.01.010
2. Brustio PR, Magistro D, Zecca M, Rabaglietti E, Liubicich ME. Age-related decrements in dual-task performance: comparison of different mobility and cognitive tasks. A cross sectional study. PLoS ONE. (2017) 12:e0181698. doi: 10.1371/journal.pone.0181698
3. Learmonth YC, Ensari I, Motl RW. Cognitive motor interference in multiple sclerosis: insights from a systematic quantitative review. Arch Phys Med Rehabil. (2017) 98:1229–40. doi: 10.1016/j.apmr.2016.07.018
4. Deblock-Bellamy A, Lamontagne A, Blanchette AK. Cognitive-locomotor dual-task interference in stroke survivors and the influence of the tasks: a systematic review. Front Neurol. (2020) 11:882. doi: 10.3389/fneur.2020.00882
5. Johansson H, Ekman U, Rennie L, Peterson DS, Leavy B, Franzén E. Dual-task effects during a motor-cognitive task in Parkinson's disease: patterns of prioritization and the influence of cognitive status. Neurorehabil Neural Repair. (2021) 35:356–66. doi: 10.1177/1545968321999053
6. Veldkamp R, Baert I, Kalron A, Tacchino A, D'hooge M, Vanzeir E, et al. Structured cognitive-motor dual task training compared to single mobility training in persons with multiple sclerosis, a multicenter RCT. J Clin Med. (2019) 8:E2177. doi: 10.3390/jcm8122177
7. Jung SH, Hasegawa N, Mancini M, King LA, Carlson-Kuhta P, Smulders K, et al. Effects of the agility boot camp with cognitive challenge (ABC-C) exercise program for Parkinson's disease. NPJ Park Dis. (2020) 6:31. doi: 10.1038/s41531-020-00132-z
8. Ali N, Tian H, Thabane L, Ma J, Wu H, Zhong Q, et al. The effects of dual-task training on cognitive and physical functions in older adults with cognitive impairment; a systematic review and meta-analysis. J Prev Alzheimers Dis. (2022) 9:359–70. doi: 10.14283/jpad.2022.16
9. Baek CY, Chang WN, Park BY, Lee KB, Kang KY, Choi MR. Effects of dual-task gait treadmill training on gait ability, dual-task interference, and fall efficacy in people with stroke: a randomized controlled trial. Phys Ther. (2021) 101:pzab067. doi: 10.1093/ptj/pzab067
10. Schneider N, Dagan M, Katz R, Thumm PC, Brozgol M, Giladi N, et al. Combining transcranial direct current stimulation with a motor-cognitive task: the impact on dual-task walking costs in older adults. J Neuroengineering Rehabil. (2021) 18:23. doi: 10.1186/s12984-021-00826-2
11. Davis JC, Bryan S, Best JR Li LC, Hsu CL, Gomez C, Vertes KA, et al. Mobility predicts change in older adults' health-related quality of life: evidence from a vancouver falls prevention prospective cohort study. Health Qual Life Outcomes. (2015) 13:101. doi: 10.1186/s12955-015-0299-0
12. Smith E, Cusack T, Cunningham C, Blake C. The influence of a cognitive dual task on the gait parameters of healthy older adults: a systematic review and meta-analysis. J Aging Phys Act. (2017) 25:671–86. doi: 10.1123/japa.2016-0265
13. Leone C, Patti F, Feys P. Measuring the cost of cognitive-motor dual tasking during walking in multiple sclerosis. Mult Scler Houndmills Basingstoke Engl. (2015) 21:123–31. doi: 10.1177/1352458514547408
14. Raffegeau TE, Krehbiel LM, Kang N, Thijs FJ, Altmann LJP, Cauraugh JH, et al. meta-analysis: Parkinson's disease and dual-task walking. Parkinsonism Relat Disord. (2019) 62:28–35. doi: 10.1016/j.parkreldis.2018.12.012
15. Hamacher D, Hamacher D, Schega L. A cognitive dual task affects gait variability in patients suffering from chronic low back pain. Exp Brain Res. (2014) 232:3509–13. doi: 10.1007/s00221-014-4039-1
16. Yogev-Seligmann G, Giladi N, Brozgol M, Hausdorff JM. A training program to improve gait while dual tasking in patients with Parkinson's disease: a pilot study. Arch Phys Med Rehabil. (2012) 93:176–81. doi: 10.1016/j.apmr.2011.06.005
17. Schaefer S, Lövdén M, Wieckhorst B, Lindenberger U. Cognitive performance is improved while walking: differences in cognitive–sensorimotor couplings between children and young adults. Eur J Dev Psychol. (2010) 7:371–89. doi: 10.1080/17405620802535666
18. Yogev-Seligmann G, Rotem-Galili Y, Mirelman A, Dickstein R, Giladi N, Hausdorff JM. How does explicit prioritization alter walking during dual-task performance? Effects of age and sex on gait speed and variability. Phys Ther. (2010) 90:177–86. doi: 10.2522/ptj.20090043
19. Alderman BL, Olson RL, Mattina DM. Cognitive function during low-intensity walking: a test of the treadmill workstation. J Phys Act Health. (2014) 11:752–8. doi: 10.1123/jpah.2012-0097
20. Tomporowski PD, Audiffren M. Dual-task performance in young and older adults: speed-accuracy tradeoffs in choice responding while treadmill walking. J Aging Phys Act. (2014) 22:557–63. doi: 10.1123/JAPA.2012-0241
21. Larson MJ, LeCheminant JD, Carbine K, Hill KR, Christenson E, Masterson T, et al. Slow walking on a treadmill desk does not negatively affect executive abilities: an examination of cognitive control, conflict adaptation, response inhibition, and post-error slowing. Front Psychol. (2015) 6:723. doi: 10.3389/fpsyg.2015.00723
22. Larson MJ, LeCheminant JD, Hill K, Carbine K, Masterson T, Christenson E. Cognitive and typing outcomes measured simultaneously with slow treadmill walking or sitting: implications for treadmill desks. PLoS ONE. (2015) 10:e0121309. doi: 10.1371/journal.pone.0121309
23. Ehmann PJ, Brush CJ, Olson RL, Bhatt SN, Banu AH, Alderman BL. Active workstations do not impair executive function in young and middle-age adults. Med Sci Sports Exerc. (2017) 49:965–74. doi: 10.1249/MSS.0000000000001189
24. Ohzuno T, Usuda S. Cognitive-motor interference in post-stroke individuals and healthy adults under different cognitive load and task prioritization conditions. J Phys Ther Sci. (2019) 31:255–60. doi: 10.1589/jpts.31.255
25. Hsiu-Chen C, Chiung-Chu C, Jiunn-Woei L, Wei-Da C, Yi-Hsin W, Ya-Ju C, et al. The effects of dual-task in patients with Parkinson's disease performing cognitive-motor paradigms. J Clin Neurosci. (2020) 72:72–8. doi: 10.1016/j.jocn.2020.01.024
26. Penko AL, Streicher MC, Dey T, Rosenfeldt AB, Alberts JL. Parkinson's gait kinematics deteriorates across multiple cognitive domains under dual-task paradigms. Clin Neurol Neurosurg. (2020) 197:106083. doi: 10.1016/j.clineuro.2020.106083
27. Fearon C, Butler JS, Waechter SM, Killane I, Kelly SP, Reilly RB, et al. Neurophysiological correlates of dual tasking in people with Parkinson's disease and freezing of gait. Exp Brain Res. (2021) 239:175–87. doi: 10.1007/s00221-020-05968-8
28. Feld JA, Plummer P. Patterns of cognitive-motor dual-task interference post stroke: an observational inpatient study at hospital discharge. Eur J Phys Rehabil Med. (2021) 57:327–36. doi: 10.23736/S1973-9087.20.06273-5
29. Marshall MR, Duckworth SC, Currie MR, Schmid D, Rogers RR. Role of age and sex on dual tasking using a treadmill desk while performing cognitive tests. Gait Posture. (2021) 90:148–53. doi: 10.1016/j.gaitpost.2021.08.013
30. Ozkul C, Guclu-Gunduz A, Eldemir K, Apaydin Y, Gulsen C, Yazici G, et al. Dual-task cost and related clinical features in patients with multiple sclerosis. Motor Control. (2021) 25:211–33. doi: 10.1123/mc.2020-0035
31. Rice J, Corp DT, Swarowsky A, Cahalin LP, Cabral DF, Nunez C, et al. Greater cognitive-motor interference in individuals post-stroke during more complex motor tasks. J Neurol Phys Ther JNPT. (2022) 46:26–33. doi: 10.1097/NPT.0000000000000379
32. Plummer P, Eskes G, Wallace S, Giuffrida C, Fraas M, Campbell G, et al. Cognitive-motor interference during functional mobility after stroke: state of the science and implications for future research. Arch Phys Med Rehabil. (2013) 94:2565–74.e6. doi: 10.1016/j.apmr.2013.08.002
33. Papegaaij S, Hortobágyi T, Godde B, Kaan WA, Erhard P, Voelcker-Rehage C. Neural correlates of motor-cognitive dual-tasking in young and old adults. PLoS ONE. (2017) 12:e0189025. doi: 10.1371/journal.pone.0189025
34. Forte R, Pesce C, Di Baldassarre A, Shea J, Voelcker-Rehage C, Capranica L, et al. How older adults cope with cognitive complexity and environmental constraints during dual-task walking: the role of executive function involvement. Int J Environ Res Public Health. (2019) 16:E1835. doi: 10.3390/ijerph16101835
35. Oliveira AS, Reiche MS, Vinescu CI, Thisted SAH, Hedberg C, Castro MN, et al. The cognitive complexity of concurrent cognitive-motor tasks reveals age-related deficits in motor performance. Sci Rep. (2018) 8:6094. doi: 10.1038/s41598-018-24346-7
36. Vila-Chã C, Vaz C, Oliveira AS. Electrocortical activity in older adults is more influenced by cognitive task complexity than concurrent walking. Front Aging Neurosci. (2021) 13:718648. doi: 10.3389/fnagi.2021.718648
37. Srygley JM, Mirelman A, Herman T, Giladi N, Hausdorff JM. When does walking alter thinking? Age and task associated findings. Brain Res. (2009) 1253:92–9. doi: 10.1016/j.brainres.2008.11.067
38. Vaportzis E, Georgiou-Karistianis N, Stout JC. Dual task performance in normal aging: a comparison of choice reaction time tasks. PLoS ONE. (2013) 8:e60265. doi: 10.1371/journal.pone.0060265
39. Goh H-T, Pearce M, Vas A. Task matters: an investigation on the effect of different secondary tasks on dual-task gait in older adults. BMC Geriatr. (2021) 21:510. doi: 10.1186/s12877-021-02464-8
40. Plummer-D'Amato P, Brancato B, Dantowitz M, Birken S, Bonke C, Furey E. Effects of gait and cognitive task difficulty on cognitive-motor interference in aging. J Aging Res. (2012) 2012:583894. doi: 10.1155/2012/583894
41. Hobert MA, Niebler R, Meyer SI, Brockmann K, Becker C, Huber H, et al. Poor trail making test performance is directly associated with altered dual task prioritization in the elderly–baseline results from the TREND study. PLoS ONE. (2011) 6:e27831. doi: 10.1371/journal.pone.0027831
42. Beurskens R, Bock O. Age-related deficits of dual-task walking: a review. Neural Plast. (2012) 2012:131608. doi: 10.1155/2012/131608
43. Wild LB, de Lima DB, Balardin JB, Rizzi L, Giacobbo BL, Oliveira HB, et al. Characterization of cognitive and motor performance during dual-tasking in healthy older adults and patients with Parkinson's disease. J Neurol. (2013) 260:580–9. doi: 10.1007/s00415-012-6683-3
44. Wajda DA, Sosnoff JJ. Cognitive-motor interference in multiple sclerosis: a systematic review of evidence, correlates, and consequences. BioMed Res Int. (2015) 2015:720856. doi: 10.1155/2015/720856
45. Corti EJ, Gasson N, Loftus AM. Cognitive profile and mild cognitive impairment in people with chronic lower back pain. Brain Cogn. (2021) 151:105737. doi: 10.1016/j.bandc.2021.105737
46. Mao CP, Yang HJ, Zhang QJ, Yang QX Li XH. Altered effective connectivity within the Cingulo-frontal-parietal cognitive attention networks in chronic low back pain: a dynamic causal modeling study. Brain Imaging Behav. (2022) 16:1516–27. doi: 10.1007/s11682-021-00623-4
47. Sherafat S, Salavati M, Takamjani IE, Akhbari B, Rad SM, Mazaheri M, et al. Effect of dual-tasking on dynamic postural control in individuals with and without nonspecific low back pain. J Manipulative Physiol Ther. (2014) 37:170–9. doi: 10.1016/j.jmpt.2014.02.003
48. Shih H-JS, Winstein CJ, Kulig K. Young adults with recurrent low back pain demonstrate altered trunk coordination during gait independent of pain status and attentional demands. Exp Brain Res. (2021) 239:1937–49. doi: 10.1007/s00221-021-06106-8
49. Hillel I, Gazit E, Nieuwboer A, Avanzino L, Rochester L, Cereatti A, et al. Is every-day walking in older adults more analogous to dual-task walking or to usual walking? Elucidating the gaps between gait performance in the lab and during 24/7 monitoring. Eur Rev Aging Phys Act. (2019) 16:6. doi: 10.1186/s11556-019-0214-5
50. Warmerdam E, Romijnders R, Geritz J, Elshehabi M, Maetzler C, Otto JC, et al. Proposed mobility assessments with simultaneous full-body inertial measurement units and optical motion capture in healthy adults and neurological patients for future validation studies: study protocol. Sensors. (2021) 21:5833. doi: 10.3390/s21175833
51. Gibb WR, Lees AJ. The relevance of the Lewy body to the pathogenesis of idiopathic Parkinson's disease. J Neurol Neurosurg Psychiatry. (1988) 51:745–52. doi: 10.1136/jnnp.51.6.745
52. Thompson AJ, Banwell BL, Barkhof F, Carroll WM, Coetzee T, Comi G, et al. Diagnosis of multiple sclerosis: 2017 revisions of the McDonald criteria. Lancet Neurol. (2018) 17:162–73. doi: 10.1016/S1474-4422(17)30470-2
53. Otto JC, Gierthmühlen J, Kirchhofer V, Borzikowsky C, Baron R. Validation of the questionnaire for symptom assessment in pain disorders for back pain patients (Q-SAP). Eur J Pain Lond Engl. (2021) 25:513–28. doi: 10.1002/ejp.1690
54. Nasreddine ZS, Phillips NA, Bédirian V, Charbonneau S, Whitehead V, Collin I, et al. The Montreal cognitive assessment, MoCA: a brief screening tool for mild cognitive impairment. J Am Geriatr Soc. (2005) 53:695–9. doi: 10.1111/j.1532-5415.2005.53221.x
55. Guralnik JM, Simonsick EM, Ferrucci L, Glynn RJ, Berkman LF, Blazer DG, et al. short physical performance battery assessing lower extremity function: association with self-reported disability and prediction of mortality and nursing home admission. J Gerontol. (1994) 49:M85–94. doi: 10.1093/geronj/49.2.M85
56. Goetz CG, Tilley BC, Shaftman SR, Stebbins GT, Fahn S, Martinez-Martin P, et al. Movement disorder society-sponsored revision of the unified Parkinson's disease rating scale (MDS-UPDRS): scale presentation and clinimetric testing results. Mov Disord. (2008) 23:2129–70. doi: 10.1002/mds.22340
57. Hoehn MM, Yahr MD. Parkinsonism: onset, progression and mortality. Neurology. (1967) 17:427–42. doi: 10.1212/WNL.17.5.427
58. Kurtzke JF. Rating neurologic impairment in multiple sclerosis: an expanded disability status scale (EDSS). Neurology. (1983) 33:1444–52. doi: 10.1212/WNL.33.11.1444
59. Brott T, Adams HP, Olinger CP, Marler JR, Barsan WG, Biller J, et al. Measurements of acute cerebral infarction: a clinical examination scale. Stroke. (1989) 20:864–70. doi: 10.1161/01.STR.20.7.864
60. Williamson A, Hoggart B. Pain: a review of three commonly used pain rating scales. J Clin Nurs. (2005) 14:798–804. doi: 10.1111/j.1365-2702.2005.01121.x
61. Haase I, Schwarz A, Burger A, Kladny B. [Comparison of Hannover functional ability questionnaire (FFbH) and the SF-36 subscale “physical functioning”]. Rehabil. (2001) 40:40–2. doi: 10.1055/s-2001-12127
62. Henik A, Tzelgov J. Is three greater than five: the relation between physical and semantic size in comparison tasks. Mem Cognit. (1982) 10:389–95. doi: 10.3758/BF03202431
63. Bock O. Dual-task costs while walking increase in old age for some, but not for other tasks: an experimental study of healthy young and elderly persons. J Neuroengineering Rehabil. (2008) 5:27. doi: 10.1186/1743-0003-5-27
64. Kelly VE, Janke AA, Shumway-Cook A. Effects of instructed focus and task difficulty on concurrent walking and cognitive task performance in healthy young adults. Exp Brain Res. (2010) 207:65–73. doi: 10.1007/s00221-010-2429-6
65. Begeman M, Kumru H, Leenders K, Valls-Sole J. Unilateral reaction time task is delayed during contralateral movements. Exp Brain Res. (2007) 181:469–75. doi: 10.1007/s00221-007-0944-x
66. Godefroy O, Roussel M, Despretz P, Quaglino V, Boucart M. Age-related slowing: perceptuomotor, decision, or attention decline? Exp Aging Res. (2010) 36:169–89. doi: 10.1080/03610731003613615
67. Jehu DA, Desponts A, Paquet N, Lajoie Y. Prioritizing attention on a reaction time task improves postural control and reaction time. Int J Neurosci. (2015) 125:100–6. doi: 10.3109/00207454.2014.907573
68. Sparrow WA, Begg RK, Parker S. Aging effects on visual reaction time in a single task condition and when treadmill walking. Motor Control. (2006) 10:201–11. doi: 10.1123/mcj.10.3.201
69. Faulkner KA, Redfern MS, Rosano C, Landsittel DP, Studenski SA, Cauley JA, et al. Reciprocal influence of concurrent walking and cognitive testing on performance in older adults. Gait Posture. (2006) 24:182–9. doi: 10.1016/j.gaitpost.2005.08.004
70. Remaud A, Boyas S, Lajoie Y, Bilodeau M. Attentional focus influences postural control and reaction time performances only during challenging dual-task conditions in healthy young adults. Exp Brain Res. (2013) 231:219–29. doi: 10.1007/s00221-013-3684-0
71. Kaur M, Nagpal S, Singh H, Suhalka ML. Effect of dual task activity on reaction time in males and females. Indian J Physiol Pharmacol. (2014) 58:389–94.
72. Balasubramaniam M, Sivapalan K, Nishanthi V, Kinthusa S, Dilani M. Effect of dual-tasking on visual and auditory simple reaction times. Indian J Physiol Pharmacol. (2015) 59:194–8.
73. Tsang WW-N, Chan VW-L, Wong HH, Yip TW-C, Lu X. The effect of performing a dual-task on postural control and selective attention of older adults when stepping backward. J Phys Ther Sci. (2016) 28:2806–11. doi: 10.1589/jpts.28.2806
74. Samulski B, Prebor J, Armitano-Lago C, Morrison S. Age-related changes in neuromotor function when performing a concurrent motor task. Exp Brain Res. (2020) 238:565–74. doi: 10.1007/s00221-020-05736-8
75. Remaud A, Boyas S, Caron GAR, Bilodeau M. Attentional demands associated with postural control depend on task difficulty and visual condition. J Mot Behav. (2012) 44:329–40. doi: 10.1080/00222895.2012.708680
76. Postigo-Alonso B, Galvao-Carmona A, Conde-Gavilán C, Jover A, Molina S, Peña-Toledo MA, et al. The effect of prioritization over cognitive-motor interference in people with relapsing-remitting multiple sclerosis and healthy controls. PLoS ONE. (2019) 14:e0226775. doi: 10.1371/journal.pone.0226775
77. Salkovic D, Hobert MA, Bellut C, Funer F, Renno S, Haertner L, et al. Evidence for a selectively regulated prioritization shift depending on walking situations in older adults. Front Aging Neurosci. (2017) 9:75. doi: 10.3389/fnagi.2017.00075
78. Patel N, Jankovic J, Hallett M. Sensory aspects of movement disorders. Lancet Neurol. (2014) 13:100–12. doi: 10.1016/S1474-4422(13)70213-8
79. Jensen AR. The theory of intelligence and its measurement. Intelligence. (2011) 39:171–7. doi: 10.1016/j.intell.2011.03.004
80. Stroop JR. Studies of interference in serial verbal reactions. J Exp Psychol. (1935) 18:643–62. doi: 10.1037/h0054651
81. Scarpina F, Tagini S. The Stroop color and word test. Front Psychol. (2017) 8:557. doi: 10.3389/fpsyg.2017.00557
82. Yogev-Seligmann G, Hausdorff JM, Giladi N. The role of executive function and attention in gait. Mov Disord. (2008) 23:329–42; quiz 472. doi: 10.1002/mds.21720
83. Nóbrega-Sousa P, Gobbi LTB, Orcioli-Silva D, Conceição NR da, Beretta VS, Vitório R. Prefrontal cortex activity during walking: effects of aging and associations with gait and executive function. Neurorehabil Neural Repair. (2020) 34:915–24. doi: 10.1177/1545968320953824
84. Morenilla L, Márquez G, Sánchez JA, Bello O, López-Alonso V, Fernández-Lago H, et al. Postural stability and cognitive performance of subjects with Parkinson's disease during a dual-task in an upright stance. Front Psychol. (2020) 11:1256. doi: 10.3389/fpsyg.2020.01256
85. Kim H, Fraser S. Neural correlates of dual-task walking in people with central neurological disorders: a systematic review. J Neurol. (2022) 269:2378–402. doi: 10.1007/s00415-021-10944-5
86. Rooney S, Ozkul C, Paul L. Correlates of dual-task performance in people with multiple sclerosis: a systematic review. Gait Posture. (2020) 81:172–82. doi: 10.1016/j.gaitpost.2020.07.069
87. Boisgontier MP, Beets IAM, Duysens J, Nieuwboer A, Krampe RT, Swinnen SP. Age-related differences in attentional cost associated with postural dual tasks: increased recruitment of generic cognitive resources in older adults. Neurosci Biobehav Rev. (2013) 37:1824–37. doi: 10.1016/j.neubiorev.2013.07.014
88. Brustio PR, Rainoldi A, Petrigna L, Rabaglietti E, Pizzigalli L. Postural stability during dual- and triple-task conditions: the effect of different levels of physical fitness in older adults. Sci Sports. (2021) 36:143–51. doi: 10.1016/j.scispo.2020.02.012
Keywords: dual task cost, Parkinson's disease, stroke, Multiple Sclerosis, low back pain, Stroop test, reaction time, dual task
Citation: Bianchini E, Warmerdam E, Romijnders R, Hansen C, Pontieri FE and Maetzler W (2022) Cognitive dual-task cost depends on the complexity of the cognitive task, but not on age and disease. Front. Neurol. 13:964207. doi: 10.3389/fneur.2022.964207
Received: 08 June 2022; Accepted: 12 September 2022;
Published: 03 October 2022.
Edited by:
Anderson Souza Oliveira, Aalborg University, DenmarkReviewed by:
Carolina Vila-Chã, Instituto Politécnico da Guarda, PortugalPaolo Riccardo Brustio, University of Turin, Italy
Rita Chiaramonte, University of Catania, Italy
Copyright © 2022 Bianchini, Warmerdam, Romijnders, Hansen, Pontieri and Maetzler. This is an open-access article distributed under the terms of the Creative Commons Attribution License (CC BY). The use, distribution or reproduction in other forums is permitted, provided the original author(s) and the copyright owner(s) are credited and that the original publication in this journal is cited, in accordance with accepted academic practice. No use, distribution or reproduction is permitted which does not comply with these terms.
*Correspondence: Walter Maetzler, dy5tYWV0emxlciYjeDAwMDQwO25ldXJvbG9naWUudW5pLWtpZWwuZGU=