- 1Department of Physical Medicine and Rehabilitation, Harvard Medical School, Boston, MA, United States
- 2Department of Physical Medicine and Rehabilitation, Spaulding Rehabilitation Hospital and the Schoen Adams Research Institute at Spaulding Rehabilitation, Charlestown, MA, United States
- 3Home Base, A Red Sox Foundation and Massachusetts General Hospital Program, Boston, MA, United States
- 4Faculty of Medicine and Health Technology, Tampere University and Tampere University Hospital, Tampere, Finland
- 5Department of Psychology, University of Kentucky, Lexington, KY, United States
- 6Medical Imaging Centre, Department of Radiology, Tampere University Hospital, Tampere, Finland
- 7Department of Psychiatry and Neurochemistry, Institute of Neuroscience and Physiology, Sahlgrenska Academy at the University of Gothenburg, Mölndal, Sweden
- 8Clinical Neurochemistry Laboratory, Sahlgrenska University Hospital, Mölndal, Sweden
- 9UK Dementia Research Institute at University College London, London, United Kingdom
- 10Department of Neurodegenerative Disease, University College London Queen Square Institute of Neurology, London, United Kingdom
- 11Hong Kong Center for Neurodegenerative Diseases, Hong Kong, Hong Kong SAR, China
- 12Neurocenter, Department of Neurosurgery, Turku University Hospital and University of Turku, Turku, Finland
- 13Turku Brain Injury Center, Turku University Hospital and University of Turku, Turku, Finland
- 14Department of Neurosurgery, Tampere University Hospital and Tampere University, Tampere, Finland
Blood-based biomarkers have been increasingly studied for diagnostic and prognostic purposes in patients with mild traumatic brain injury (MTBI). Biomarker levels in blood have been shown to vary throughout age groups. Our aim was to study four blood biomarkers, glial fibrillary acidic protein (GFAP), ubiquitin C-terminal hydrolase-L1 (UCH-L1), neurofilament light (NF-L), and total tau (t-tau), in older adult patients with MTBI. The study sample was collected in the emergency department in Tampere University Hospital, Finland, between November 2015 and November 2016. All consecutive adult patients with head injury were eligible for inclusion. Serum samples were collected from the enrolled patients, which were frozen and later sent for biomarker analyses. Patients aged 60 years or older with MTBI, head computed tomography (CT) imaging, and available biomarker levels were eligible for this study. A total of 83 patients (mean age = 79.0, SD = 9.58, range = 60–100; 41.0% men) were included in the analysis. GFAP was the only biomarker to show statistically significant differentiation between patients with and without acute head CT abnormalities [U(83) = 280, p < 0.001, r = 0.44; area under the curve (AUC) = 0.79, 95% CI = 0.67–0.91]. The median UCH-L1 values were modestly greater in the abnormal head CT group vs. normal head CT group [U (83) = 492, p = 0.065, r = 0.20; AUC = 0.63, 95% CI = 0.49–0.77]. Older age was associated with biomarker levels in the normal head CT group, with the most prominent age associations being with NF-L (r = 0.56) and GFAP (r = 0.54). The results support the use of GFAP in detecting abnormal head CT findings in older adults with MTBIs. However, small sample sizes run the risk for producing non-replicable findings that may not generalize to the population and do not translate well to clinical use. Further studies should consider the potential effect of age on biomarker levels when establishing clinical cut-off values for detecting head CT abnormalities.
Introduction
There is tremendous interest in developing blood-based biomarkers for both diagnostic and prognostic purposes for people who have sustained traumatic brain injuries (TBI) (1–4). Blood biomarkers have been studied in children (5–7) and adults (8–10) in the context of sport-related concussions (11–13) and in the emergency department (ED) setting (14, 15). There have been many studies that have employed blood-based biomarkers as a screening for trauma-related intracranial abnormalities visible on day-of-injury computed tomography (CT) (16–19). In 2018, the Food and Drug Administration (FDA) permitted the marketing of a panel of two biomarkers, glial fibrillary acidic protein (GFAP) and ubiquitin C-terminal hydrolase-L1 (UCH-L1), as a screening test for intracranial abnormalities in adults with head injuries presenting to the ED setting (20, 21). There have been multiple studies showing that GFAP is elevated in children (22, 23) and adults (14, 17–19) with structural neuroimaging evidence of TBI, and some studies have also illustrated elevations of UCH-L1 in those with macroscopic intracranial abnormalities (24, 25).
The purpose of this study was to examine the diagnostic usefulness of four serum biomarkers, from the Quanterix Simoa 4-plex assay (26), for identifying intracranial abnormalities in older adults presenting to the ED following suspected mild TBI (MTBI). Our goal was to examine the biomarkers individually—and not incorporate them into an existing clinical decision-making pathway or guideline. This 4-plex assay is used to measure four proteins: GFAP, neurofilament light (NF-L), total tau (t-tau), and UCH-L1. A description of these four biomarkers, and their temporal kinetics following TBI, is presented in Table 1. Among current trends in TBI research, there have been calls for more research focused on MTBI in older adults (27, 28), and there is evidence that biomarker results are different in older adults (29–31). Based on studies published to date, we hypothesized that, when studied in older patients, all four biomarkers would have statistically significant predictive associations with the presence of acute intracranial abnormalities on head CT, that GFAP would have the highest diagnostic accuracy, and that older patients would have higher biomarker levels.
Materials and methods
Subjects
The study sample was collected in the Tampere University Hospital ED (Tampere, Finland) between November 2015 and November 2016. The Tampere University Hospital is the only neurosurgical referral hospital in the hospital district, and the ED provides health services for a total of ~470,000 residents from 22 municipalities, both urban and rural. The Tampere University Hospital is comparable to a US level I trauma center. All adult patients aged 18 years or older, with an acute traumatic head injury, seen within 24 h of injury, were the population of interest. The minimum criteria for TBI were as follows: either blunt injury to the head or acceleration/deceleration type injury resulting in witnessed loss of consciousness, disorientation, or amnesia, and an initial Glasgow Coma Scale (GCS) score of 13–15 (32). An injury-ED admission delay of more than 24 h was an exclusion criterion. From that population, a sample of 325 patients was recruited for a prospective study (33) designed to validate the Scandinavian Guidelines for Initial Management of Minimal, Mild and Moderate Head Injuries in Adults (34). Of the 325 patients, 314 had blood drawn for the original study of S100B, and 225 of those samples were stored in the freezer for future use.
For the present study, the patients fulfilling the following criteria were included in the final sample: Glasgow Coma Scale (GCS) score from 14 to 15, age 60 years or over, blood sampling within 12 h or less from injury, lab results available for all four biomarkers, head CT scan performed, and no significant extracranial injury requiring surgery. Detailed case reports were obtained for each patient describing injury mechanism, symptoms following the head injury, and the findings of the physical examination performed in the ED by the on-call physician. The study enrolment process is presented in Figure 1. All enrolled patients provided an informed written consent according to the Declaration of Helsinki. The study protocol was approved by the Ethics Committee of the Pirkanmaa Hospital District, Tampere, Finland (ethical code: R15045).
Head CT imaging
Non-contrast head CT was performed with a 64-row CT scanner (GE, Lightspeed VCT, WI, USA). The findings were systematically coded by a neuroradiologist (K.B.), blinded to the clinical characteristics of the patients, based on the National Institute of Neurological Disorders and Stroke (NINDS) Common Data Elements (CDE) (63). The following traumatic lesions were coded as acute intracranial abnormalities according to the NINDS CDEs: skull fracture, epidural hematoma, subdural hematoma, subarachnoid hemorrhage, vascular dissection, traumatic aneurysm, venous sinus injury, midline shift, cisternal compression, fourth ventricle shift/effacement, contusion, intracerebral hemorrhage, intraventricular hemorrhage, diffuse axonal injury, penetrating injuries, craniocervical junction injury, brain swelling, ischemia/infarction/hypoxic-ischemic injury. No subject had an isolated skull fracture, and an isolated skull fracture, if present, would not have met our inclusion criterion for an intracranial abnormality.
Blood biomarkers and laboratory procedures
Venous blood samples were collected within 12 h of injury. Blood samples were centrifuged for 10 min at 10,000 rpm at room temperature. Part of the serum was analyzed at Tampere University Hospital (Tampere, Finland) as part of the hospital laboratory's on-call services for a prior study (33). The remaining serum was stored in Eppendorf tubes and immediately frozen at −70°C for future use. The blood samples were collected in Tampere between November 2015 and November 2016. Approximately 2 years later the serum was sent to the Sahlgrenska University Hospital (research laboratory) in Mölndal, Sweden for analysis. All the serum samples were transferred in 20 kilograms of dry ice from Tampere to Mölndal. The samples analyzed in Mölndal underwent one cycle of freezing and thawing. The serum samples were analyzed in March of 2018 using the Quanterix Simoa 4-plex assay on a Simoa HD-1 analyzer according to the manufacturer's instructions (Quanterix, Billerica, MA). The 4-plex assay measures four protein biomarkers in blood: GFAP, NF-L, t-tau, and UCH-L1. For GFAP, the lower limit of quantification (LLOQ) was 0.467 pg/mL, the lower limit of detection (LLOD) was 0.221 pg/mL, and the calibration range was 0–1,000 pg/mL. For NF-L, LLOQ was 0.241 pg/mL, LLOD was 0.104 pg/mL, and the calibration range was 0–500 pg/mL. For t-tau, LLOQ was 0.053 pg/mL, LLOD was 0.024 pg/mL, and the calibration range was 0–100 pg/mL. For UCH-L1, LLOQ was 5.45 pg/mL, LLOD 1.74 pg/mL, and the calibration range was 0–10 ng/mL. The mean interval in which the serum was frozen was 23.9 months (SD = 2.9, Range = 17–27). The laboratory technicians performing the analyses were blinded to the clinical data.
Statistical analyses
The primary dependent variables were the four blood biomarkers. The distributional characteristics for these biomarkers were examined using visual inspection of histograms for each biomarker in the total sample and the subgroups and Shapiro-Wilk tests of normality. Given that nearly all biomarkers in all groups were not normally distributed, non-parametric analyses were used. Group differences were examined using Mann-Whitney U tests. Bivariate associations were calculated using Spearman rho coefficients. Receiver operator characteristic curves, under non-parametric assumptions, were computed using the brain imaging result as the dependent variable and the biomarker values as the predictors. The statistical analyses were conducted with the Statistical Package for Social Sciences software program (IBM SPSS Statistics for Windows, Versions 24, Armonk, NY, USA). The statistical significance level was set at p < 0.05.
Results
Patient characteristics and blood sampling
The mean age of the total sample (n = 83) was 79.0 years (Median = 78.0, SD = 9.58, Range = 60–100; 41.0% men). The mechanism of injury was a fall in 96.4% of the sample. All had Glasgow Coma Scale scores (GCS) of 14 or 15 in the ED (GCS 15 = 94.0%). The median time between injury and blood sampling was 3.1 h (SD = 2.2; IQR = 1.8–4.7, Range = 0.8–11.7 h). The median time between blood sampling and head CT was 0.9 h (SD = 2.4; IQR = 0.2–2.1, Range = −1.3 to 13.9 h). Intracranial abnormalities were identified in 26.5% (n = 22). The imaging findings were as follows: skull fracture = 2.4% (n = 2), epidural hematoma = 0%, extra-axial hematoma=21.7% (n = 18), subdural hematoma (acute) = 12.0% (n = 10), subdural hematoma (subacute or chronic) = 3.6% (n = 3), traumatic subarachnoid hemorrhage = 10.8% (n = 9), intraventricular hemorrhage = 1.2 (n = 1), midline shift (supratentorial) = 2.4% (n = 2), contusion = 4.8% (n = 4), traumatic axonal injury = 3.6% (n = 3). No patient had an isolated skull fracture. The injury characteristics of the sample stratified by negative and positive imaging groups (i.e., uncomplicated vs. complicated MTBI) are presented in Table 2.
Descriptive statistics and group comparisons for the biomarkers
Descriptive statistics for the biomarkers, stratified by groups, are presented in Table 3 and Figure 2. Visual examination of the frequency distribution histograms and Shapiro-Wilk tests of normality revealed non-normal distributions for all biomarkers in the total sample and in the subgroups. As seen in the box and whisker plots in Figure 2, GFAP had the greatest separation between the groups, whereas the other biomarkers had similar distributional characteristics between groups. Those with abnormal CT scans had significantly greater levels of GFAP than those with normal CT scans [U(83) = 280, p < 0.001, r = 0.44]. There were no statistically significant differences between the two groups on the other three biomarkers (NF-L, t-tau, and UCH-L1).
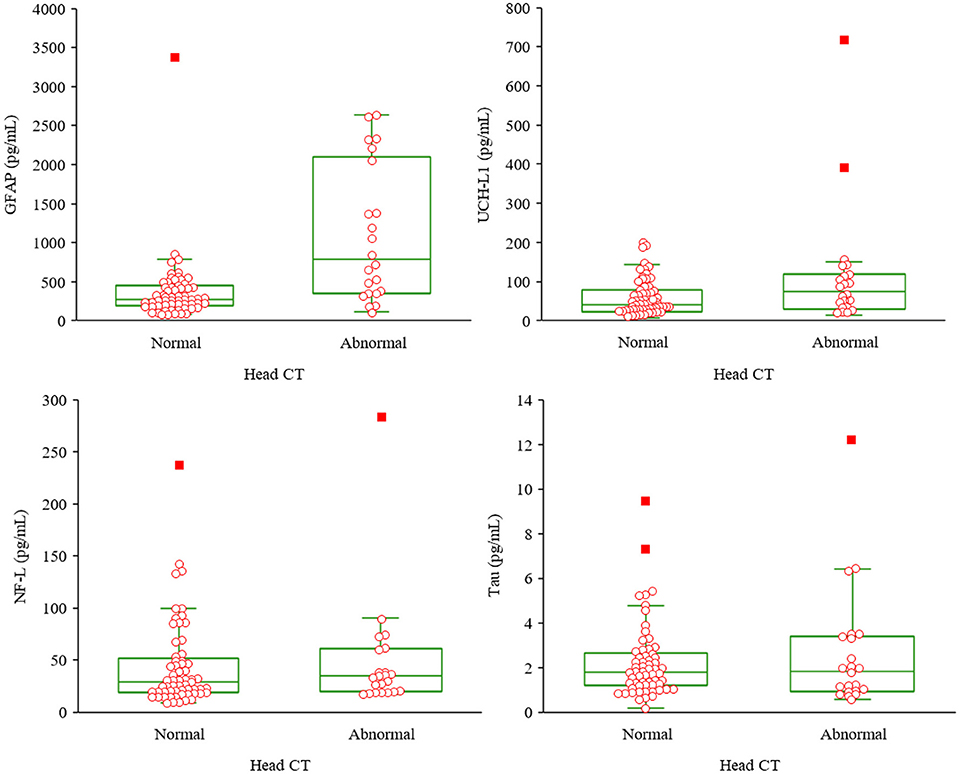
Figure 2. Box and whisker plots for the biomarkers by group. Upper left, glial fibrillary acidic protein; upper right, ubiquitin C-terminal hydrolase-L1; lower left, neurofilament light; lower right, total tau; CT; computed tomography. Normal head CT n = 61, abnormal head CT n = 22.
Biomarker intercorrelations and correlations with age and time to blood sampling
An intercorrelation matrix, stratified by group, is presented in Table 4. For those with a normal head CT, there were small to large correlations between age and GFAP, NF-L, t-tau, and UCH-LI. The most prominent age association was with NF-L (rho = 0.56) and GFAP (rho = 0.54), as illustrated in Table 4 and Figure 3. Due to small sample size in the abnormal head CT group, and inconsistent power across normal and abnormal head CT groupings, significance was not interpreted for the correlations. The correlations between age and the biomarkers were consistently negligible to small in the group with an abnormal head CT. The correlations between the biomarkers ranged from small to medium, apart from the large correlation between tau and UCH-L1 (rho = 0.57) in the abnormal head CT group and between GFAP and NF-L (rho = 0.53) in the normal head CT group. However, the correlation between GFAP and NF-L in the abnormal head CT group was negative (rho = −0.13). The correlations between the biomarkers and time to blood sampling varied from non-existent to weak in both groups, apart from GFAP in the abnormal head CT group (rho = 0.39).
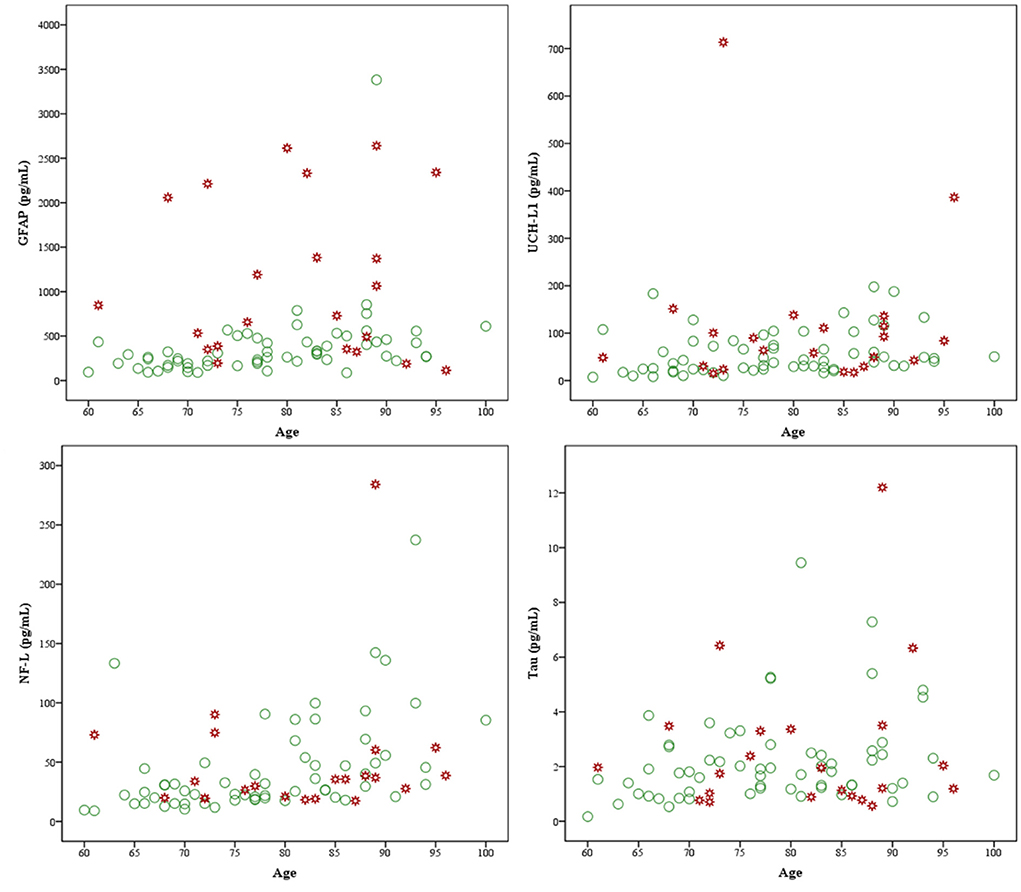
Figure 3. Scatterplots of biomarkers and age by group. GFAP, glial fibrillary acidic protein, upper left; UCH-L1, ubiquitin C-terminal hydrolase-L1, upper right; NF-L, neurofilament light, lower left; and tau, lower right. Markers in green are subjects with normal head computed tomography (CT) (n = 61) scans and markers in red are subjects with abnormal head CT scans (n = 22).
Discriminating abnormal from normal head CT scans
Receiver operator characteristic curves were computed for each biomarker. The results are presented in Figure 4. The only biomarker with a significant area under the curve was GFAP (AUC = 0.791, 95% CI = 0.669–0.914). All individuals' biomarker values are presented in the Table A1. To illustrate a possible future clinical algorithm for using two biomarkers, GFAP and UCH-L1, as a screening test for referring for head CT in older adults, we visually examined the frequency distributions and selected cutoff scores that would, in combination, identify all subjects with abnormal head CT scans. All subjects with abnormal head CT scans were correctly identified using the following algorithm: GFAP values are 323 pg/mL or greater or UCH-LI values are 42 pg/mL or greater. Applying that algorithm to those with negative head CT scans yielded the following: 36/61 (59.0%) had GFAP values of < 323 pg/mL, 31/61 (50.9%) had UCH-L1 values < 42 pg/mL, and 22/61 (36.1%) had both GFAP < 323 pg/mL and UCH-L1 < 42 pg/mL. The algorithm specificity for detecting abnormal head CT scans was 0.36 (95% CI = 0.24–0.49).
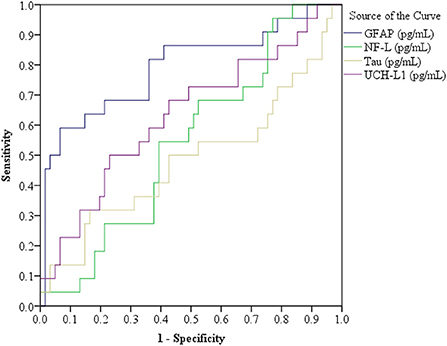
Figure 4. Receiver operator characteristic curves for the four biomarkers. Glial fibrillary acidic protein (GFAP), in the total sample (N = 83), area under the curve (AUC) = 0.791, standard error (SE) = 0.063, 95% confidence interval (CI) = 0.669–0.914; Ubiquitin C-terminal hydrolase-L1 (UCH-L1) AUC = 0.633, SE = 0.072, 95% CI = 0.493–0.774; Neurofilament light (NF-L) AUC = 0.544, SE = 0.067, 95% CI = 0.412–0.676; and t-Tau AUC = 0.487, SE = 0.079, 95% CI = 0.331–0.642. n = 83. A subgroup analysis was conducted for patients with Glasgow Coma Scale = 15 (n = 78, CT positive = 20 and normal head CT = 58), as follows: GFAP AUC = 0.776, SE = 0.068, 95% CI = 0.642–0.910; UCH-L1 AUC = 0.633, SE = 0.075, 95% CI = 0.486–0.780; NF-L AUC = 0.508, SE = 0.069, 95% CI = 0.373–0.642; and t-Tau AUC = 0.482, SE = 0.080, 95% CI = 0.325–0.639. Normal Head CT (n = 58): GFAP Median (Mdn) = 283.59, interquartile range (IQR) = 206.21–441.27; UCH-L1 Mdn = 41.80, IQR = 23.95–83.26; NF-L Mdn = 30.11, IQR = 19.56–54.37; and t-Tau Mdn = 1.79, IQR = 1.20–2.61. Positive Head CT (n = 20): GFAP Mdn = 787.84, IQR = 351.17–2174.14; UCH-L1 Mdn = 73.71, IQR = 32.83–129.61; NF-L Mdn = 32.70, IQR = 19.67–54.91; and t-Tau Mdn = 1.85, IQR = 0.95–3.35.
Discussion
There is tremendous interest in whether blood biomarkers can be used within an ED clinical pathway to determine if patients should undergo head CT following a suspected or confirmed MTBI (33, 64, 65). It is recognized that biomarker values differ in older adults (30, 31), so there is a need for research focused specifically on these patients. Nearly all older adults in this study presented to the ED following head trauma sustained in a fall. It is well-established that falls are a common cause of ED visits (66, 67) and TBI in older adults (68–70). The percentage of patients with traumatic abnormalities on their head CT was 26.5%, and, although substantially higher compared to many prior MTBI studies, a high proportion of abnormal scans has been reported in other MTBI studies focusing on older adults (71, 72).
Only one of the four biomarkers, GFAP, significantly differentiated older adults with abnormal head CT scans from those with normal head CT scans (Figures 2, 4). The median values for UCH-L1 were modestly greater in the complicated MTBI group vs. the uncomplicated MTBI group (Table 3), revealing a small separation between these groups (Figure 2), whereas there was very little difference between groups on levels of NF-L and t-tau. These results align with a prior study examining the added value of incorporating GFAP and UCH-L1 into clinical decision rules for detecting CT abnormalities in MTBI, which identified improved performance using GFAP independent of UCH-L1 (73).
The AUCs in the current study were substantially lower than in a prior TBI study using the same biomarker platform (GFAP: 0.79 vs. 0.88; UCH-L1: 0.63 vs. 0.86, NF-L: 0.54 vs. 0.84, and t-tau: 0.49 vs. 0.77, respectively) (43). The prior study, however, differed methodologically in that it included patients with mild, moderate, and severe TBIs (vs. MTBI only in this study), the median age of their sample was more than 30 years younger than in the present study, and they measured the biomarkers in plasma (vs. serum in this study). By including patients with moderate and severe TBIs, the prior study had higher biomarker levels for GFAP, UCH-L1, and t-tau than the present study and this might have contributed to better differentiation of those with abnormal head CT scans. Patients with moderate and severe TBI always undergo an acute head CT (72). Therefore, in this study, we wanted to focus on patients with MTBI. We also wanted to examine older patients, because higher prevalence of intracranial abnormalities has been reported among the elderly (74) and they experience more severe consequences and slower recovery than younger patients (28).
The intercorrelations between the biomarkers ranged from very small to large, indicating their variable relationships with each other (Table 4). Older age was associated with greater levels of all the biomarkers in those with uncomplicated MTBIs (Table 4), aligning with previous studies (29, 31). In contrast, for those with abnormal head CT scans, there was not an association between age and biomarker levels (Table 4; Figure 3). We found a large correlation between GFAP and NF-L (r = 0.53) in patients with normal head CT scans, while in patients with abnormal head CT scans, a similar correlation was found between UCH-L1 and t-tau (r = 0.57). The prior study with the same biomarker platform found the strongest correlation between NF-L and UCH-L1 (r = 0.71) and the weakest correlation between GFAP and t-tau (r = 0.06) (43). In their study cohort, the median age was 36 years lower and all TBI severities were included. While the neurobiology underlying this difference in results is unclear, the intercorrelations of biomarker concentrations are possibly also affected by age, sampling time point, extracranial injuries, and the severity of TBI.
Given that GFAP and UCH-L1 have been FDA-approved to screen for abnormal head CT scans following TBI in adults, we provided all individual subjects' biomarker values in the Table A1. Some authors have expressed concern that past researchers have not attempted to develop clinical cutoff scores or develop clinical algorithms for using blood biomarkers in the ED setting (75, 76). Therefore, we illustrated one example of an algorithm that can be used within 12 h of injury, optimized for the data presented in the Table A1, that yielded 100% sensitivity for identifying abnormal head CT scans. The algorithm produced 39 false positive and 22 true negative results, reflecting a specificity of 36.1% within the sample. Although the algorithm was developed using biomarker levels of older adults, the specificity of the algorithm matches that of the FDA-approved biomarker kit using the same biomarker combination (specificity 0.367) (20). A summary of the individual biomarkers follows.
GFAP
We found that GFAP was the only biomarker that significantly differentiated older adults with abnormal head CT scans with the AUC of 0.791. The use of this biomarker in detecting CT abnormalities in TBI patients has considerable support in the literature. A living systematic review summarized the existing literature on GFAP as a screening test for abnormal head CT findings (77). The sensitivities for predicting abnormal head CT findings were between 67 and 100% and the specificities between 0 and 89% (77). Different assays have been used in detecting GFAP levels, with various ELISA assays being the most often used (10, 14, 39, 41, 47, 48, 50, 78). Others have used assays by Randox Biochip (42), digital array from Quanterix™ (49), and the Quanterix Simoa 4-plex (8, 17, 43). GFAP is included in the FDA approved the Banyan BTI™ for clinical use (21). A study raised concern in the ability of GFAP to differentiate between patients with MTBI vs. orthopedic injury (79), which may be in part a result of GFAP not being elevated in some cases of uncomplicated MTBI (16, 80). However, it has been reported that serum GFAP levels may differentiate between patients with moderate to severe TBI and orthopedic controls 30 days after the injury (81). GFAP has been shown to be less accurate in discriminating complicated and uncomplicated MTBI patients in older vs. younger adults (30). The positive correlation between age and GFAP in our sample is consistent with a study (29) that reported greater GFAP concentrations in older patients with MTBIs.
UCH-L1
In the current study, we found that UCH-L1 did not statistically significantly differentiate older adults with abnormal head CT scans. Those with abnormal CT scans had modestly higher UCH-L1 levels, but the effect size was small. Prior studies illustrate that UCH-L1 levels differentiate, to some degree, patients with and without traumatic intracranial CT findings in isolated TBI, and in people with TBI and multitrauma (17, 18, 20, 24, 25). A living systematic review summarized the existing literature on serum and plasma UCH-L1 for predicting acute traumatic head CT findings. The AUC for serum was 0.77 (95% CI: 0.70–0.83, 347 cases, 179 controls) and for plasma was 0.67 (95% CI: 0.58–0.76, 595 cases, 256 control) (77). Several different assays for UCH-L1 have been used in TBI studies. Most studies have used ELISA, either custom made (25, 39, 40, 82–84) or commercially available by Banyan Biomarkers Inc. (6, 10, 20, 24, 41, 50, 85). Other studies have used Randox Biochip (42, 79, 86), the Quanterix Simoa 4-plex (17, 43), or electrochemiluminescence immunosassays (87). They differ in analysis techniques resulting in different limits of detection and quantification. UCH-L1 is included in the FDA approved the Banyan BTI™ for clinical use along with GFAP (21). Although considered brain-specific, a study detected elevated UCH-L1 serum levels in patients with orthopedic trauma compared to the healthy controls (25). Also, one study revealed that UCH-L1 levels did not distinguish patients with orthopedic injury without head trauma from patients with uncomplicated MTBI (79). In our study, there were modest differences in the median values of UCH-L1 (r = 0.20) between groups (Table 3), and we were statistically underpowered to detect a difference of this small magnitude. A recent study (29) showed the Banyan BTI™ to be 100% sensitive in detecting CT abnormalities in older patients but showed a stepwise reduction in specificity with age. The specificity for all patients over 65 years old was 13%. In our study, UCH-L1 was useful in an algorithm combined with GFAP designed to reduce the number of CT scans in the CT negative group while detecting all abnormalities in the CT positive group. The specificity of the algorithm was 36%.
Tau
In the current study, we found that t-tau did not statistically significantly differentiate older adults with abnormal head CT scans. There are discrepancies in other study results assessing the ability of tau to discriminate patients with and without head CT findings following milder spectrum brain injury (9, 17, 88). One concern expressed previously was that studies showing no significant differences in serum tau levels based on head CT findings involved an assay with a relatively high lower limit of detection, possibly obtaining negative findings due to less sensitivity of the assay (54). Studies using more sensitive technology have showed significant increases in blood tau levels in patients with MTBIs with abnormal findings on head CT (17, 43, 49). In addition, it should be noted, that there are studies suggesting that blood tau levels are affected by factors such as age in cognitively normal individuals (89) and presence of Alzheimer's disease (90, 91). In the current study, we used one of the more sensitive technologies for measuring t-tau with a lower limit of detection but found no group differences based on CT findings.
NF-L
In our study, NF-L did not statistically significantly differentiate older adults with abnormal head CT scans. In some studies, NF-L levels in blood discriminate patients with and without traumatic head CT findings (17, 43, 55). Elevated levels have been linked to diffuse axonal injury (92). However, due to its kinetically slow increase profile in blood, some suggest it might be more suitable for sampling during subacute and chronic stages of TBI for outcome prediction purposes (62). Also, blood NF-L levels have been reported to be higher in neurological conditions such as Alzheimer's disease (93, 94), multiple sclerosis (95), and Huntington's disease (96). A strong association between older age and increased NF-L levels has been reported both in MTBI patients and orthopedically injured trauma controls (31). Specifically, there was a high correlation between age and NF-L levels in a sample of adults with MTBIs and normal day-of-injury neuroimaging (r = 0.80), and within subgroups of patients who did (r = 0.068) and did not (r = 0.76) have pre-injury neurological diseases (31).
Limitations
This study was limited in its sample size, and especially, in the small number of patients with complicated MTBI. The sample was underpowered to detect smaller effects, but the overall differences in biomarker levels were quite modest with the exception of GFAP.
Small sample sizes run the risk for producing non-replicable findings that may not generalize to the population and do not translate well to clinical use. The results of this study require replication both in a larger sample of older adults and in younger patients. Moreover, GFAP levels might reflect, in part, aging-related inflammatory processes affecting astrocytes (97), higher levels of GFAP are associated with both aging and the development of dementia (98), and one study suggests that GFAP might be less sensitive to traumatic intracranial abnormalities in older adults compared to younger adults (30). The current approach of using the 4-plex to detect abnormal head CT should be replicated in diverse samples by future researchers to evaluate potential clinical usefulness.
Extracranial injuries were only grossly assessed based on the need for surgery other than neurosurgery, and more minor orthopedic injuries, such as conservatively treated fractures, were not noted. Therefore, it is possible that the biomarker concentrations are affected by these injuries. Our data is not suitable for analyzing the possible effect of extracranial injuries on biomarker concentrations.
Measuring biomarkers in cerebrospinal fluid (CSF) might yield different results. However, there are practical (e.g., time and cost), clinical (e.g., risks and possible need to discontinue anticoagulants), and ethical (e.g., suitable less invasive methods are available) challenges associated with measuring biomarkers in CSF.
Finally, our blood samples were stored in low temperature for ~2 years before the biomarker analysis. Although freezing blood samples in low temperatures has been shown to preserve proteins for years to decades (99), it is uncertain if the biomarker concentrations may have been affected by the storage period.
Conclusions
This study set out to determine if GFAP, UCH-L1, NF-L, and tau differentiated between older adult patients with and without traumatic intracranial abnormalities due to MTBI. GFAP was the only biomarker that significantly differentiated older adults with abnormal head CT scans from those with normal scans. The findings support the use of GFAP as a biomarker for detecting CT-positive intracranial abnormalities in older adults with MTBI. Further studies should consider the potential effect of age on biomarker levels when establishing clinical cut-off values for detecting head CT abnormalities.
Data availability statement
The original contributions presented in the study are included in the article/Supplementary material, further inquiries can be directed to the corresponding author.
Ethics statement
The studies involving human participants were reviewed and approved by Ethics Committee of the Pirkanmaa Hospital District, Tampere, Finland (ethical code: R15045). The patients/participants provided their written informed consent to participate in this study.
Author contributions
GI conceptualized the study, assisted with the literature review, conceptualized the statistical analyses, conducted the analyses, and wrote portions of the manuscript. MM assisted with conceptualizing the study, conducted the literature review, and wrote portions of the manuscript. JK assisted with conceptualizing the statistical analyses. KB reviewed and coded all the head CT scans. HZ and KajB conducted all the blood biomarker analyses, provided the results in a database, and wrote portions of the manuscript. JP assisted with conceptualizing the study and assisted with the literature review. TL assisted with conceptualizing the study, served as the overall study PI for the parent study and this research program, managed all regulatory requirements, and supervised the conduct of all aspects of data collection and database development. All authors critically reviewed and edited drafts of the manuscript and approved the final version for submission.
Funding
The study was financially supported by the Finnish State Research Funding, and the Finnish Medical Society Duodecim. TL and JP have received funding from Government's Special Financial Transfer tied to academic research in Health Sciences (Finland). JP has received funding from the Academy of Finland (#17379), Emil Aaltonen Foundation sr and the Maire Taponen Foundation. KajB acknowledges funding from the Torsten Söderberg Foundation, the Swedish Research Council, and the Swedish Brain Foundation. HZ is a Wallenberg Scholar supported by grants from the Swedish Research Council (#2018-02532), the European Research Council (#681712 and #101053962), Swedish State Support for Clinical Research (#ALFGBG-71320), the Alzheimer Drug Discovery Foundation (ADDF), USA (#201809-2016862), the AD Strategic Fund and the Alzheimer's Association (#ADSF-21-831376-C, #ADSF-21-831381-C and #ADSF-21-831377-C), the Olav Thon Foundation, the Erling-Persson Family Foundation, Stiftelsen för Gamla Tjänarinnor, Hjärnfonden, Sweden (#FO2019-0228), the European Union's Horizon 2020 research and innovation programme under the Marie Skłodowska-Curie grant agreement No 860197 (MIRIADE), the European Union Joint Programme—Neurodegenerative Disease Research (JPND2021-00694), and the UK Dementia Research Institute at UCL (UKDRI-1003). GI acknowledges unrestricted philanthropic support from the Mooney-Reed Charitable Foundation, ImPACT Applications, Inc., the Heinz Family Foundation, and the Schoen Adams Research Institute at Spaulding Rehabilitation. The above entities were not involved in the study design, collection, analysis, interpretation of data, the writing of this article, or the decision to submit it for publication.
Acknowledgments
The authors acknowledge research assistant Anne Simi for her assistance with the patient enrolment and data collection, and research coordinator Annamari Aitolahti for her assistance with blood sample logistics.
Conflict of interest
Author GI serves as a scientific advisor for NanoDX®, Sway Operations, LLC, and Highmark, Inc. He has a clinical and consulting practice in forensic neuropsychology, including expert testimony, involving individuals who have sustained mild TBIs. He has received research funding from several test publishing companies, including ImPACT Applications, Inc., CNS Vital Signs, and Psychological Assessment Resources (PAR, Inc.). He has received research funding as a principal investigator from the National Football League, and subcontract grant funding as a collaborator from the Harvard Integrated Program to Protect and Improve the Health of National Football League Players Association Members. Author JP has received a speaker's fee Finnish Medical Association. Author HZ has served at scientific advisory boards and/or as a consultant for Abbvie, Alector, Annexon, Apellis, Artery Therapeutics, AZTherapies, CogRx, Denali, Eisai, Nervgen, Novo Nordisk, Pinteon Therapeutics, Red Abbey Labs, Passage Bio, Roche, Samumed, Siemens Healthineers, Triplet Therapeutics, and Wave, has given lectures in symposia sponsored by Cellectricon, Fujirebio, Alzecure, Biogen, and Roche, and is a co-founder of Brain Biomarker Solutions in Gothenburg AB (BBS), which is a part of the GU Ventures Incubator Program. Author KajB has served as a consultant or at advisory boards for Alzheon, BioArctic, Biogen, Eli Lilly, Fujirebio Europe, IBL International, Merck, Novartis, Pfizer, and Roche Diagnostics, and is a co-founder of Brain Biomarker Solutions in Gothenburg AB, a GU Venture-based platform company at the University of Gothenburg.
The remaining authors declare that the research was conducted in the absence of any commercial or financial relationships that could be construed as a potential conflict of interest.
Publisher's note
All claims expressed in this article are solely those of the authors and do not necessarily represent those of their affiliated organizations, or those of the publisher, the editors and the reviewers. Any product that may be evaluated in this article, or claim that may be made by its manufacturer, is not guaranteed or endorsed by the publisher.
Supplementary material
The Supplementary Material for this article can be found online at: https://www.frontiersin.org/articles/10.3389/fneur.2022.960741/full#supplementary-material
References
1. Gan ZS, Stein SC, Swanson R, Guan S, Garcia L, Mehta D, Smith DH. Blood biomarkers for traumatic brain injury: a quantitative assessment of diagnostic and prognostic accuracy. Front Neurol. (2019) 10:446. doi: 10.3389/fneur.2019.00446
2. Wang KK, Yang Z, Zhu T, Shi Y, Rubenstein R, Tyndall JA, et al. An update on diagnostic and prognostic biomarkers for traumatic brain injury. Expert Rev Mol Diagn. (2018) 18:165–80. doi: 10.1080/14737159.2018.1428089
3. Edalatfar M, Piri SM, Mehrabinejad MM, Mousavi MS, Meknatkhah S, Fattahi MR, et al. Biofluid biomarkers in traumatic brain injury: a systematic scoping review. Neurocrit Care. (2021) 35:559–72. doi: 10.1007/s12028-020-01173-1
4. Amoo M, Henry J, O'Halloran PJ, Brennan P, Husien MB, Campbell M. S100B, GFAP, UCH-L1 and NSE as predictors of abnormalities on CT imaging following mild traumatic brain injury: a systematic review and meta-analysis of diagnostic test accuracy. Neurosurg Rev. (2021) 1:1–23. doi: 10.1007/s10143-021-01678-z
5. Stukas S, Higgins V, Frndova H, Gill J, Hubara E, Guerguerian AM, et al. Characterisation of serum total tau following paediatric traumatic brain injury: a case-control study. Lancet Child Adolesc Heal. (2019) 3:558–67. doi: 10.1016/S2352-4642(19)30194-4
6. Papa L, Mittal MK, Ramirez J, Silvestri S, Giordano P, Braga CF, et al. Neuronal biomarker ubiquitin C-terminal hydrolase detects traumatic intracranial lesions on computed tomography in children and youth with mild traumatic brain injury. J Neurotrauma. (2017) 34:2132–40. doi: 10.1089/neu.2016.4806
7. Lorton F, Simon-Pimmel J, Masson D, Launay E, Gras-Le Guen C, Scherdel P. Impact of routine S100B protein assay on CT scan use in children with mild traumatic brain injury. Clin Chem Lab Med. (2021) 59:875–82. doi: 10.1515/cclm-2020-1293
8. Posti JP, Takala RSK, Lagerstedt L, Dickens AM, Hossain I, Mohammadian M, et al. Correlation of blood biomarkers and biomarker panels with traumatic findings on computed tomography after traumatic brain injury. J Neurotrauma. (2019) 36:2178–89. doi: 10.1089/neu.2018.6254
9. Forouzan A, Motamed H, Delirrooyfard A, Zallaghi S. Serum cleaved tau protein and clinical outcome in patients with minor head trauma. Open Access Emerg Med. (2020) 12:7–12. doi: 10.2147/OAEM.S217424
10. Papa L, Brophy GM, Welch RD, Lewis LM, Braga CF, Tan CN, et al. Time course and diagnostic accuracy of glial and neuronal blood biomarkers GFAP and UCH-L1 in a large cohort of trauma patients with and without mild traumatic brain injury. JAMA Neurol. (2016) 73:551–60. doi: 10.1001/jamaneurol.2016.0039
11. Pattinson CL, Meier TB, Guedes VA, Lai C, Devoto C, Haight T, et al. Plasma biomarker concentrations associated with return to sport following sport-related concussion in collegiate athletes-A Concussion Assessment, Research, and Education (CARE) Consortium Study. JAMA Netw Open. (2020) 3:13191. doi: 10.1001/jamanetworkopen.2020.13191
12. McCrea M, Broglio SP, McAllister TW, Gill J, Giza CC, Huber DL, et al. Association of blood biomarkers with acute sport-related concussion in collegiate athletes: findings from the NCAA and Department of Defense CARE Consortium. JAMA Netw Open. (2020) 3:e1919771. doi: 10.1001/jamanetworkopen.2019.19771
13. Papa L, Ramia MM, Edwards D, Johnson BD, Slobounov SM. Systematic review of clinical studies examining biomarkers of brain injury in athletes after sports-related concussion. J Neurotrauma. (2015) 32:661–73. doi: 10.1089/neu.2014.3655
14. Anderson TN, Hwang J, Munar M, Papa L, Hinson HE, Vaughan A, et al. Blood-based biomarkers for prediction of intracranial hemorrhage and outcome in patients with moderate or severe traumatic brain injury. J Trauma Acute Care Surg. (2020) 89:80–6. doi: 10.1097/TA.0000000000002706
15. Yue JK, Upadhyayula PS, Avalos LN, Deng H, Wang KKW. The role of blood biomarkers for magnetic resonance imaging diagnosis of traumatic brain injury. Med. (2020) 56:87. doi: 10.3390/medicina56020087
16. Okonkwo DO, Puffer RC, Puccio AM, Yuh EL, Yue JK, Diaz-Arrastia R, et al. Point-of-care platform blood biomarker testing of glial fibrillary acidic protein versus S100 calcium-binding protein B for prediction of traumatic brain injuries: a transforming research and clinical knowledge in traumatic brain injury study. J Neurotrauma. (2020) 37:2460–7. doi: 10.1089/neu.2020.7140
17. Czeiter E, Amrein K, Gravesteijn BY, Lecky F, Menon DK, Mondello S, et al. Blood biomarkers on admission in acute traumatic brain injury: Relations to severity, CT findings and care path in the CENTER-TBI study. EBioMedicine. (2020) 56:102785. doi: 10.1016/j.ebiom.2020.102785
18. Mahan MY, Thorpe M, Ahmadi A, Abdallah T, Casey H, Sturtevant D, et al. Glial fibrillary acidic protein (GFAP) outperforms S100 calcium-binding protein B (S100B) and ubiquitin C-terminal hydrolase L1 (UCH-L1) as predictor for positive computed tomography of the head in trauma subjects. World Neurosurg. (2019) 128:e434–44. doi: 10.1016/j.wneu.2019.04.170
19. Gill J, Latour L, Diaz-Arrastia R, Motamedi V, Turtzo C, Shahim P, et al. Glial fibrillary acidic protein elevations relate to neuroimaging abnormalities after mild TBI. Neurology. (2018) 91:E1385–9. doi: 10.1212/WNL.0000000000006321
20. Bazarian JJ, Biberthaler P, Welch RD, Lewis LM, Barzo P, Bogner-Flatz V, et al. Serum GFAP and UCH-L1 for prediction of absence of intracranial injuries on head CT (ALERT-TBI): a multicentre observational study. Lancet Neurol. (2018) 17:782–9. doi: 10.1016/S1474-4422(18)30231-X
21. U.S. Food and Drug Administration. Evaluation of Automatic Class III Designation for Banyan Brain Trauma Indicator: Decision Memorandum. Silver Spring, MD: U.S. Food and Drug Administration (2018). p. 1–32.
22. Papa L, Mittal MK, Ramirez J, Ramia M, Kirby S, Silvestri S, et al. In children and youth with mild and moderate traumatic brain injury, glial fibrillary acidic protein out-performs s100β in detecting traumatic intracranial lesions on computed tomography. J Neurotrauma. (2016) 33:58–64. doi: 10.1089/neu.2015.3869
23. Papa L, Zonfrillo MR, Ramirez J, Silvestri S, Giordano P, Braga CF, et al. Performance of glial fibrillary acidic protein in detecting traumatic intracranial lesions on computed tomography in children and youth with mild head trauma. Acad Emerg Med. (2015) 22:1274–82. doi: 10.1111/acem.12795
24. Welch RD, Ayaz SI, Lewis LM, Unden J, Chen JY, Mika VH, et al. Ability of serum glial fibrillary acidic protein, ubiquitin C-terminal hydrolase-L1, and S100B to differentiate normal and abnormal head computed tomography findings in patients with suspected mild or moderate traumatic brain injury. J Neurotrauma. (2016) 33:203–14. doi: 10.1089/neu.2015.4149
25. Papa L, Lewis LM, Silvestri S, Falk JL, Giordano P, Brophy GM, et al. Serum levels of ubiquitin C-terminal hydrolase distinguish mild traumatic brain injury from trauma controls and are elevated in mild and moderate traumatic brain injury patients with intracranial lesions and neurosurgical intervention. J Trauma Acute Care Surg. (2012) 72:1335–44. doi: 10.1097/TA.0b013e3182491e3d
26. Simoa ® N4PA Advantage Kit Description-Human Nerology 4-Plex “A.”. Billerica, MA: Quanterix (2017).
27. Peters ME, Gardner RC. Traumatic brain injury in older adults: do we need a different approach? Concussion. (2018) 3:CNC56. doi: 10.2217/cnc-2018-0001
28. Gardner RC, Dams-O'Connor K, Morrissey MR, Manley GT. Geriatric traumatic brain injury: epidemiology, outcomes, knowledge gaps, and future directions. J Neurotrauma. (2018) 35:889–906. doi: 10.1089/neu.2017.5371
29. Ward MD, Weber A, Merrill VD, Welch RD, Bazarian JJ, Christenson RH. Predictive performance of traumatic brain injury biomarkers in high-risk elderly patients. J Appl Lab Med. (2020) 5:91–100. doi: 10.1093/jalm.2019.031393
30. Gardner RC, Rubenstein R, Wang KKW, Korley FK, Yue JK, Yuh EL, et al. Age-related differences in diagnostic accuracy of plasma glial fibrillary acidic protein and tau for identifying acute intracranial trauma on computed tomography: a TRACK-TBI Study. J Neurotrauma. (2018) 35:2341–50. doi: 10.1089/neu.2018.5694
31. Iverson GL, Reddi PJ, Posti JP, Kotilainen AK, Tenovuo O, Öhman J, et al. Serum neurofilament light is elevated differentially in older adults with uncomplicated mild traumatic brain injuries. J Neurotrauma. (2019) 36:2400–6. doi: 10.1089/neu.2018.6341
32. Carroll L, Cassidy J, Holm L, Kraus J, Coronado V. Methodological issues and research recommendations for mild traumatic brain injury: the WHO Collaborating Centre Task Force on Mild Traumatic Brain Injury. J Rehabil Med. (2004) 36:113–25. doi: 10.1080/16501960410023877
33. Minkkinen M, Iverson GL, Kotilainen A-K, Pauniaho S-L, Mattila VM, Lehtimäki T, et al. Prospective validation of the scandinavian guidelines for initial management of minimal, mild, and moderate head injuries in adults. J Neurotrauma. (2019) 36:2904–12. doi: 10.1089/neu.2018.6351
34. Undén J, Ingebrigtsen T, Romner B. Scandinavian guidelines for initial management of minimal, mild and moderate head injuries in adults: an evidence and consensus-based update. BMC Med. (2013) 11:50. doi: 10.1186/1741-7015-11-50
35. Doran JF, Jackson P, Kynoch PA, Thompson RJ. Isolation of PGP 9.5, a new human neurone-specific protein detected by high-resolution two-dimensional electrophoresis. J Neurochem. (1983) 40:1542–7. doi: 10.1111/j.1471-4159.1983.tb08124.x
36. Thompson RJ, Doran JF, Jackson P, Dhillon AP, Rode J. PGP 95—a new marker for vertebrate neurons and neuroendocrine cells. Brain Res. (1983) 278:224–8. doi: 10.1016/0006-8993(83)90241-X
37. Wilson PO, Barber PC, Hamid QA, Power BF, Dhillon AP, Rode J, et al. The immunolocalization of protein gene product 9.5 using rabbit polyclonal and mouse monoclonal antibodies. Br J Exp Pathol. (1988) 69:91–104.
38. Wilkinson K, Lee K, Deshpande S, Duerksen-Hughes P, Boss J, Pohl J. The neuron-specific protein PGP 95 is a ubiquitin carboxyl-terminal hydrolase. Science (80- ). (1989) 246:670–3. doi: 10.1126/science.2530630
39. Mondello S, Papa L, Buki A, Bullock MR, Czeiter E, Tortella FC, et al. Neuronal and glial markers are differently associated with computed tomography findings and outcome in patients with severe traumatic brain injury: a case control study. Crit Care. (2011) 15:R156. doi: 10.1186/cc10286
40. Brophy GM, Mondello S, Papa L, Robicsek SA, Gabrielli A, Tepas J, et al. Biokinetic analysis of ubiquitin C-terminal hydrolase-L1 (UCH-L1) in severe traumatic brain injury patient biofluids. J Neurotrauma. (2011) 28:861–70. doi: 10.1089/neu.2010.1564
41. Diaz-Arrastia R, Wang KKW, Papa L, Sorani MD, Yue JK, Puccio AM, et al. Acute biomarkers of traumatic brain injury: relationship between plasma levels of ubiquitin C-terminal hydrolase-L1 and glial fibrillary acidic protein. J Neurotrauma. (2014) 31:19–25. doi: 10.1089/neu.2013.3040
42. Posti JP, Takala RSK, Runtti H, Newcombe VF, Outtrim J, Katila AJ, et al. The levels of glial fibrillary acidic protein and ubiquitin C-terminal hydrolase-L1 during the first week after a traumatic brain injurycorrelations with clinical and imaging findings. Neurosurgery. (2016) 79:456–64. doi: 10.1227/NEU.0000000000001226
43. Korley FK, Yue JK, Wilson DH, Hrusovsky K, Diaz-Arrastia R, Ferguson AR, et al. Performance evaluation of a multiplex assay for simultaneous detection of four clinically relevant traumatic brain injury biomarkers. J Neurotrauma. (2018) 36:182–7. doi: 10.1089/neu.2017.5623
44. Thelin EP, Zeiler FA, Ercole A, Mondello S, Büki A, Bellander B-M, et al. Serial sampling of serum protein biomarkers for monitoring human traumatic brain injury dynamics: a systematic review. Front Neurol. (2017) 8:300. doi: 10.3389/fneur.2017.00300
45. Eng LF, Vanderhaeghen JJ, Bignami A, Gerstl B. An acidic protein isolated from fibrous astrocytes. Brain Res. (1971) 28:351–4. doi: 10.1016/0006-8993(71)90668-8
46. Bignami A, Eng LF, Dahl D, Uyeda CT. Localization of the glial fibrillary acidic protein in astrocytes by immunofluorescence. Brain Res. (1972) 43:429–35. doi: 10.1016/0006-8993(72)90398-8
47. Honda M, Tsuruta R, Kaneko T, Kasaoka S, Yagi T, Todani M, et al. Serum glial fibrillary acidic protein is a highly specific biomarker for traumatic brain injury in humans compared with S-100B and neuron-specific enolase. J Trauma. (2010) 69:104–9. doi: 10.1097/TA.0b013e3181bbd485
48. Metting Z, Wilczak N, Rodiger LA, Schaaf JM, Van Der Naalt J, GFAP. and S100B in the acute phase of mild traumatic brain injury. Neurology. (2012) 78:1428–33. doi: 10.1212/WNL.0b013e318253d5c7
49. Bogoslovsky T, Wilson D, Chen Y, Hanlon D, Gill J, Jeromin A, et al. Increases of plasma levels of glial fibrillary acidic protein, tau, and amyloid β up to 90 days after traumatic brain injury. J Neurotrauma. (2017) 34:66–73. doi: 10.1089/neu.2015.4333
50. Welch RD, Ellis M, Lewis LM, Ayaz SI, Mika VH, Millis S, et al. Modeling the kinetics of serum glial fibrillary acidic protein, ubiquitin carboxyl-terminal hydrolase-L1, and S100B concentrations in patients with traumatic brain injury. J Neurotrauma. (2017) 34:1957–71. doi: 10.1089/neu.2016.4772
51. Binder LI, Frankfurter A, Rebhun LI. The distribution of tau in the mammalian central nervous central nervous. J Cell Biol. (1985) 101:1371–8. doi: 10.1083/jcb.101.4.1371
52. Shahim P, Tegner Y, Wilson DH, Randall J, Skillbäck T, Pazooki D, et al. Blood biomarkers for brain injury in concussed professional ice hockey players. JAMA Neurol. (2014) 71:684–92. doi: 10.1001/jamaneurol.2014.367
53. Rubenstein R, Chang B, Yue JK, Chiu A, Winkler EA, Puccio AM, et al. Comparing plasma phospho tau, total tau, and phospho tau-total tau ratio as acute and chronic traumatic brain injury biomarkers. JAMA Neurol. (2017) 74:1063–72. doi: 10.1001/jamaneurol.2017.0655
54. Zetterberg H, Smith DH, Blennow K. Biomarkers of mild traumatic brain injury in cerebrospinal fluid and blood. Nat Rev Neurol. (2013) 9:201–10. doi: 10.1038/nrneurol.2013.9
55. Shahim P, Tegner Y, Marklund N, Blennow K, Zetterberg H. Neurofilament light and tau as blood biomarkers for sports-related concussion. Neurology. (2018) 90:E1780–8. doi: 10.1212/WNL.0000000000005518
56. Shahim P, Linemann T, Inekci D, Karsdal MA, Blennow K, Tegner Y, et al. Serum tau fragments predict return to play in concussed professional ice hockey players. J Neurotrauma. (2016) 33:1995–9. doi: 10.1089/neu.2014.3741
57. Lee MK, Xu Z, Wong PC, Cleveland DW. Neurofilaments are obligate heteropolymers in vivo. J Cell Biol. (1993) 122:1337–50. doi: 10.1083/jcb.122.6.1337
58. Trojanowski JQ, Schuck T, Schmidt ML, Lee VMY. Distribution of tau proteins in the normal human central and peripheral nervous system. J Histochem Cytochem. (1989) 37:209–15. doi: 10.1177/37.2.2492045
59. Friede RL, Samorajski T. Axon caliber related to neurofilaments and microtubules in sciatic nerve fibers of rats and mice. Anat Rec. (1970) 167:379–87. doi: 10.1002/ar.1091670402
60. Shahim P, Gren M, Liman V, Andreasson U, Norgren N, Tegner Y, et al. Serum neurofilament light protein predicts clinical outcome in traumatic brain injury. Sci Rep. (2016) 6:36791. doi: 10.1038/srep36791
61. Al Nimer F, Thelin E, Nyström H, Dring AM, Svenningsson A, Piehl F, et al. Comparative assessment of the prognostic value of biomarkers in traumatic brain injury reveals an independent role for serum levels of neurofilament light. PLoS ONE. (2015) 10:e0132177. doi: 10.1371/journal.pone.0132177
62. Thelin E, Al Nimer F, Frostell A, Zetterberg H, Blennow K, Nyström H, et al. Serum protein biomarker panel improves outcome prediction in human traumatic brain injury. J Neurotrauma. (2019) 36:2850–62. doi: 10.1089/neu.2019.6375
63. Haacke EM, Duhaime AC, Gean AD, Riedy G, Wintermark M, Mukherjee P, et al. Common data elements in radiologic imaging of traumatic brain injury. J Magn Reson Imaging. (2010) 32:516–43. doi: 10.1002/jmri.22259
64. Undén L, Calcagnile O, Undén J, Reinstrup P, Bazarian J. Validation of the Scandinavian guidelines for initial management of minimal, mild and moderate traumatic brain injury in adults. BMC Med. (2015) 13:292. doi: 10.1186/s12916-015-0533-y
65. Ananthaharan A, Kravdal G, Straume-Naesheim TM. Utility and effectiveness of the Scandinavian guidelines to exclude computerized tomography scanning in mild traumatic brain injury - a prospective cohort study. BMC Emerg Med. (2018) 18:44. doi: 10.1186/s12873-018-0193-2
66. Høidrup S, Sørensen TIA, Grønbæk M, Schroll M. Incidence and characteristics of falls leading to hospital treatment: a one-year population surveillance study of the Danish population aged 45 years and over. Scand J Public Health. (2003) 31:24–30. doi: 10.1080/14034940210134185
67. Johansen A, Dickens J, Jones M, Richmond P, Evans R. Emergency department presentation following falls: development of a routine falls surveillance system. Emerg Med J. (2011) 28:25–8. doi: 10.1136/emj.2009.086330
68. Helling TS, Watkins M, Evans LL, Nelson PW, Shook JW, Van Way CW. Low falls: an underappreciated mechanism of injury. J Trauma. (1999) 46:453–6. doi: 10.1097/00005373-199903000-00019
69. Sarani B, Temple-Lykens B, Kim P, Sonnad S, Bergey M, Pascual JL, et al. Factors associated with mortality and brain injury after falls from the standing position. J Trauma. (2009) 67:954–8. doi: 10.1097/TA.0b013e3181ae6d39
70. Pöyry T, Luoto T, Kataja A, Brander A, Tenovuo O, Iverson G, et al. Acute assessment of brain injuries in ground-level falls. J Head Trauma Rehabil. (2013) 28:89–97. doi: 10.1097/HTR.0b013e318250eadd
71. Haydel MJ, Preston CA, Mills TJ, Luber S, Blaudeau E, DeBlieux PM. Indications for computed tomography in patients with minor head injury. N Engl J Med. (2000) 343:100–5. doi: 10.1056/NEJM200007133430204
72. Stiell IG, Wells GA, Vandemheen K, Clement C, Lesiuk H, Laupacis A, et al. The Canadian CT Head Rule for patients with minor head injury. Lancet. (2001) 357:1391–6. doi: 10.1016/S0140-6736(00)04561-X
73. Papa L, Ladde JG, O'Brien JF, Thundiyil JG, Tesar J, Leech S, et al. Evaluation of glial and neuronal blood biomarkers compared with clinical decision rules in assessing the need for computed tomography in patients with mild traumatic brain injury. JAMA Netw Open. (2022) 5:e221302. doi: 10.1001/jamanetworkopen.2022.1302
74. Waganekar A, Sadasivan J, Prabhu As, Harichandrakumar K. Computed tomography profile and its utilization in head injury patients in emergency department: a prospective observational study. J Emerg Trauma Shock. (2018) 11:25. doi: 10.4103/JETS.JETS_112_17
75. Luoto TM, Raj R, Posti JP, Gardner AJ, Panenka WJ, Iverson GL. A systematic review of the usefulness of glial fibrillary acidic protein for predicting acute intracranial lesions following head trauma. Front Neurol. (2017) 8:652. doi: 10.3389/fneur.2017.00652
76. Maas AIR, Lingsma HF, ALERT-TBI. study on biomarkers for TBI: has science suffered? Lancet Neurol. (2018) 17:737–8. doi: 10.1016/S1474-4422(18)30275-8
77. Mondello S, Sorinola A, Czeiter E, Vámos Z, Amrein K, Synnot A, et al. Blood-based protein biomarkers for the management of traumatic brain injuries in adults presenting to emergency departments with mild brain injury: a living systematic review and meta-analysis. J Neurotrauma. (2021) 38:1086–106. doi: 10.1089/neu.2017.5182
78. Papa L, Silvestri S, Brophy GM, Giordano P, Falk JL, Braga CF, et al. GFAP out-performs S100β in detecting traumatic intracranial lesions on computed tomography in trauma patients with mild traumatic brain injury and those with extracranial lesions. J Neurotrauma. (2014) 31:1815–22. doi: 10.1089/neu.2013.3245
79. Posti JP, Hossain I, Takala RSK, Liedes H, Newcombe V, Outtrim J, et al. Glial fibrillary acidic protein and ubiquitin C-terminal hydrolase-L1 are not specific biomarkers for mild CT-negative traumatic brain injury. J Neurotrauma. (2017) 34:1427–38. doi: 10.1089/neu.2016.4442
80. Clarke GJB, Skandsen T, Zetterberg H, Einarsen CE, Feyling C, Follestad T, et al. One-year prospective study of plasma biomarkers from CNS in patients with mild traumatic brain injury. Front Neurol. (2021) 12:643743. doi: 10.3389/fneur.2021.643743
81. Shahim P, Politis A, van der Merwe A, Moore B, Ekanayake V, Lippa S, et al. Time course and diagnostic utility of NfL, tau, GFAP, and UCH-L1 in subacute and chronic TBI. Neurology. (2020) 95:e623–36. doi: 10.1212/WNL.0000000000009985
82. Papa L, Akinyi L, Liu MC, Pineda JA, Tepas JJ, Oli MW, et al. Ubiquitin C-terminal hydrolase is a novel biomarker in humans for severe traumatic brain injury. Crit Care Med. (2010) 38:138–44. doi: 10.1097/CCM.0b013e3181b788ab
83. Czeiter E, Mondello S, Kovacs N, Sandor J, Gabrielli A, Schmid K, et al. Brain injury biomarkers may improve the predictive power of the IMPACT outcome calculator. J Neurotrauma. (2012) 29:1770–8. doi: 10.1089/neu.2011.2127
84. Mondello S, Jeromin A, Buki A, Bullock R, Czeiter E, Kovacs N, et al. Glial neuronal ratio: a novel index for differentiating injury type in patients with severe traumatic brain injury. J Neurotrauma. (2012) 29:1096–104. doi: 10.1089/neu.2011.2092
85. Mondello S, Kobeissy F, Vestri A, Hayes RL, Kochanek PM, Berger RP. Serum concentrations of ubiquitin C-terminal hydrolase-L1 and glial fibrillary acidic protein after pediatric traumatic brain injury. Sci Rep. (2016) 6:28203. doi: 10.1038/srep28203
86. Takala RSK, Posti JP, Runtti H, Newcombe VF, Outtrim J, Katila AJ, et al. Glial fibrillary acidic protein and ubiquitin C-terminal hydrolase-L1 as outcome predictors in traumatic brain injury. World Neurosurg. (2016) 87:8–20. doi: 10.1016/j.wneu.2015.10.066
87. Kou Z, Gattu R, Kobeissy F, Welch RD, O'Neil BJ, Woodard JL, et al. Combining biochemical and imaging markers to improve diagnosis and characterization of mild traumatic brain injury in the acute setting: results from a pilot study. PLoS ONE. (2013) 8:e80296. doi: 10.1371/journal.pone.0080296
88. Kavalci C, Pekdemir M, Durukan P, Ilhan N, Yildiz M, Serhatlioglu S, et al. The value of serum tau protein for the diagnosis of intracranial injury in minor head trauma. Am J Emerg Med. (2007) 25:391–5. doi: 10.1016/j.ajem.2006.10.008
89. Lue LF, Pai MC, Chen TF, Hu CJ, Huang LK, Lin WC, et al. Age-dependent relationship between plasma Aβ40 and Aβ42 and total tau levels in cognitively normal subjects. Front Aging Neurosci. (2019) 11:292. doi: 10.3389/fnagi.2019.00292
90. Henriksen K, Byrjalsen I, Christiansen C, Karsdal MA. Relationship between serum levels of tau fragments and clinical progression of Alzheimer's disease. J Alzheimer's Dis. (2014) 43:1331–41. doi: 10.3233/JAD-140984
91. Mattsson N, Zetterberg H, Janelidze S, Insel PS, Andreasson U, Stomrud E, et al. Plasma tau in Alzheimer disease. Neurology. (2016) 87:1827–35. doi: 10.1212/WNL.0000000000003246
92. Ljungqvist J, Zetterberg H, Mitsis M, Blennow K, Skoglund T. Serum neurofilament light protein as a marker for diffuse axonal injury: results from a case series study. J Neurotrauma. (2017) 34:1124–7. doi: 10.1089/neu.2016.4496
93. Weston PSJ, Poole T, Ryan NS, Nair A, Liang Y, Macpherson K, et al. Serum neurofilament light in familial Alzheimer disease: a marker of early neurodegeneration. Neurology. (2017) 89:2167–75. doi: 10.1212/WNL.0000000000004667
94. Mattsson N, Andreasson U, Zetterberg H, Blennow K, Weiner MW, Aisen P, et al. Association of plasma neurofilament light with neurodegeneration in patients with Alzheimer disease. JAMA Neurol. (2017) 74:557–66. doi: 10.1001/jamaneurol.2016.6117
95. Disanto G, Barro C, Benkert P, Naegelin Y, Schädelin S, Giardiello A, et al. Serum neurofilament light: a biomarker of neuronal damage in multiple sclerosis. Ann Neurol. (2017) 81:857–70. doi: 10.1002/ana.24954
96. Byrne LM, Rodrigues FB, Blennow K, Durr A, Leavitt BR, Roos RAC, et al. Neurofilament light protein in blood as a potential biomarker of neurodegeneration in Huntington's disease: a retrospective cohort analysis. Lancet Neurol. (2017) 16:601–9. doi: 10.1016/S1474-4422(17)30124-2
97. Palmer AL, Ousman SS. Astrocytes and aging. Front Aging Neurosci. (2018) 10:337. doi: 10.3389/fnagi.2018.00337
98. Verberk IMW, Laarhuis MB, van den Bosch KA, Ebenau JL, van Leeuwenstijn M, Prins ND, et al. Serum markers glial fibrillary acidic protein and neurofilament light for prognosis and monitoring in cognitively normal older people: a prospective memory clinic-based cohort study. Lancet Heal Longev. (2021) 2:e87–95. doi: 10.1016/S2666-7568(20)30061-1
99. Shabihkhani M, Lucey GM, Wei B, Mareninov S, Lou JJ, Vinters H V, et al. The procurement, storage, and quality assurance of frozen blood and tissue biospecimens in pathology, biorepository, and biobank settings. Clin Biochem. (2014) 47:258–66. doi: 10.1016/j.clinbiochem.2014.01.002
Appendix
Keywords: traumatic brain injury, biomarkers, glial fibrillary acidic protein, ubiquitin C-terminal hydrolase-L1, total tau, neurofilament light, computed tomography
Citation: Iverson GL, Minkkinen M, Karr JE, Berghem K, Zetterberg H, Blennow K, Posti JP and Luoto TM (2022) Examining four blood biomarkers for the detection of acute intracranial abnormalities following mild traumatic brain injury in older adults. Front. Neurol. 13:960741. doi: 10.3389/fneur.2022.960741
Received: 03 June 2022; Accepted: 20 October 2022;
Published: 22 November 2022.
Edited by:
Linda Papa, Orlando Regional Medical Center, United StatesReviewed by:
Francisco Capani, Universidad Abierta Interamericana, ArgentinaParmenion P. Tsitsopoulos, Aristotle University of Thessaloniki, Greece
Robert D. Welch, Wayne State University, United States
Copyright © 2022 Iverson, Minkkinen, Karr, Berghem, Zetterberg, Blennow, Posti and Luoto. This is an open-access article distributed under the terms of the Creative Commons Attribution License (CC BY). The use, distribution or reproduction in other forums is permitted, provided the original author(s) and the copyright owner(s) are credited and that the original publication in this journal is cited, in accordance with accepted academic practice. No use, distribution or reproduction is permitted which does not comply with these terms.
*Correspondence: Teemu M. Luoto, teemu.luoto@pshp.fi