- 1Guang'anmen Hospital, China Academy of Chinese Medical Sciences, Beijing, China
- 2Graduate School of China Academy of Chinese Medical Sciences, Beijing, China
- 3Institute of Acupuncture and Moxibustion, China Academy of Chinese Medical Sciences, Beijing, China
- 4Dongzhimen Hospital, Beijing University of Chinese Medicine, Beijing, China
- 5Affiliated Hospital of Traditional Chinese Medicine, Southwest Medical University, Luzhou, China
Objective: Transcutaneous auricular vagus nerve stimulation (taVNS) is effective for treatment-resistant depression (TRD). In the current study, we observed the immediate modulating brain effect of taVNS in patients with TRD using rest-state functional magnetic resonance imaging (rs-fMRI).
Method: Forty patients with TRD and forty healthy controls (HCs) were recruited. Rs-fMRI was performed before and after 30 min of taVNS at baseline. The brain regions that presented significantly different the Regional Homogeneity (ReHo) between the TRD patients and HCs were selected as the ROI to calculate the functional connectivity (FC) of full brain. The correlations were estimated between the clinical scales' score and the functional brain changes.
Results: Following taVNS stimulation treatment, TRD patients showed significantly reduced ReHo in the medial orbital frontal cortex (mOFC) (F = 18.06, P < 0.0001), ANCOVA of the mOFC-Based FC images revealed a significant interaction effect on the left inferior parietal gyrus (IPG) and left superior marginal gyrus (SMG) (F = 11.6615, P < 0.001, F = 16.7520, P < 0.0001). Among these regions, the HAMD and HAMA scores and ReHo/FC changes were not correlated.
Conclusion: This study applied rs-fMRI technology to examine the effect of taVNS stimulation treatment on the brain activity of TRD. These results suggest that the brain response of TRD patients to taVNS treatment may be associated with the functional modulation of cortical regions including the medial orbital frontal cortex, the left inferior parietal gyrus, and the left superior marginal regions. Changes in these neuroimaging indices may represent the neural mechanisms underlying taVNS Immediate Stimulation treatment in TRD.
Introduction
Major depressive disorder (MDD) is a common clinical disorder of the psychiatric system, characterized by persistent depressed mood, reduced interest and cognitive function, anhedonia, and somatic disturbances (1). MDD contributes significantly to the global disease burden, with up to one-third being treatment-resistant patients (2). In clinical treatment, about 35% of patients with depression exhibit poor efficacy even after a complete course of treatment with two or more antidepressants that possess different chemical structures; this type of depression is categorized as treatment-resistant depression (TRD) (3). In addition, the disability and fatality rates of TRD patients are significantly higher than those of ordinary depression patients (4). Serretti et al. (5) reported six most likely risk factors for TRD, including long course of disease, slow onset, comorbid anxiety, advanced age, episode severity, and depressive characteristics. Therefore, TRD is a hot but difficult research topic for psychiatrists at present.
TRD is a complex disorder for which the pathogenesis is not fully understood. Studies have demonstrated TRD is associated with functional abnormalities in brain neural circuits related to emotional and cognition processing, self-representation, and reward processing, these brain regions include the medial orbital frontal cortex (mOFC), amygdala, inferior parietal gyrus (IPG), and superior marginal gyrus (SMG) (6–9). It was reported that anhedonia is associated with neurological dysfunctions in the reward system (10). Additional studies (11–13) revealed the reward loop nervous system carries emotional or cognitive information and decision-making information in the prefrontal cortex. The mOFC is a key part that mediates pain experience and motivation to avoid pain.
TRD treatment is mainly based on drug therapy combined with non-drug treatment. Most antidepressants cause adverse reactions, such as cardiovascular disease and metabolic syndrome (14). Non-drug treatments mainly include psychotherapy, electroconvulsive therapy (ECT), repetitive transcranial magnetic stimulation (rTMS), deep brain stimulation (DBS), and vagus nerve stimulation (VNS). VNS is an FDA-approved somatic treatment for treatment-resistant depression (TRD) that can produce clinically significant antidepressant effects (15). However, the application is limited by the involvement of surgery and potential side effects. To overcome the potential barriers to applying VNS, a non-invasive transcutaneous vagus nerve stimulation (taVNS) method has been developed. The rationale for using taVNS on the ear is based on anatomical studies that suggest the ear is the only place on the surface of the human body where there is afferent vagus nerve distribution (16, 17). Thus, direct stimulation of the afferent nerve fibers on the ear should produce an effect similar to classic VNS in reducing depressive symptoms but without the burden of surgical intervention (18, 19). Our previous research group (20–22) discovered that taVNS is clinically effective in treating TRD and further observed that taVNS has a significant synergistic effect on TRD patients in maintaining drug treatment. The taVNS therapeutic mechanism may be related to the modulating brain default mode network (DMN), reward network and salience network. However, the mechanism of the immediate effect of taVNS in the treatment of TRD remains unclear.
Resting-state functional magnetic resonance imaging (rs-fMRI) is a neuroimaging technique based on blood oxygenation level dependent (BOLD) levels to detect brain activity patterns and is one of the main methods to study the brain effects of acupuncture (23). Additionally, rs-fMRI has been gradually applied in the field of bipolar disorder (24), schizophrenia (25), autism (26), and other psychiatric disorders. Also, rs-fMRI has been applied to study the subtypes of depression (27–29). ReHo is used to assess the level of coordination of neural activity in local brain regions by calculating ReHo values, which indirectly reflect the spontaneous activity of local neurons in time synchronization (30). Functional connectivity (FC), which is a fMRI method for observing the functional association between different brain regions by analyzing the statistical correlation between the time series of different brain regions (31), has also been used in major depressive disorder research.
Materials and methods
Recruitment of participants
Forty adult patients aged between 18 and 70 years with a Diagnostic and Statistical Manual of Mental Disorders-IV-Text Revision or 5 (DSM-IV-TR or 5) diagnosis of major depressive disorder who had failed to respond to at least two different antidepressants with adequate dosage and treatment duration (i.e., fluoxetine ≥ 20 mg/day for ≥ 60 consecutive days) were included in our study. Forty healthy controls (HCs) were recruited and matched with patients in sex, age, and education. The HCs had no lifetime history of major or minor psychiatric disorders. In addition, the TRD patients and HCs did not have major medical or neurological illnesses, or a history of alcohol or substance abuse. All participants were right-handed. Before the study, they were all informed of the study protocol and volunteered to participate in the study. Participants with fMRI contraindications and severe organic or mental diseases were excluded.
Ethical review and registration
The present study was reviewed and approved by the Ethics Committee of Guang'anmen Hospital, China Academy of Chinese Medical sciences (No. 2017-021-SQ) and registered at the Chinese Clinical Trial Registry (No. ChiCTR-1800014277).
Transcutaneous auricular vagus nerve stimulation
The electro-acupuncture stimulator (SDZ-IIB, Hwato brand, manufactured in Su Zhou, China) was attached to the bilateral cymba conchae through electrodes on the skin surface (Figure 1). Parameters were set according to previous studies of taVNS (32, 33): Dilatational wave of 4/20 Hz and pulse width of 0.2 ms ± 30%. The current intensity was adjusted according to each patient s subjective feeling. Each taVNS session lasted for 30 min. Before treatment, the patient's ear armor was routinely disinfected with 75% alcohol.
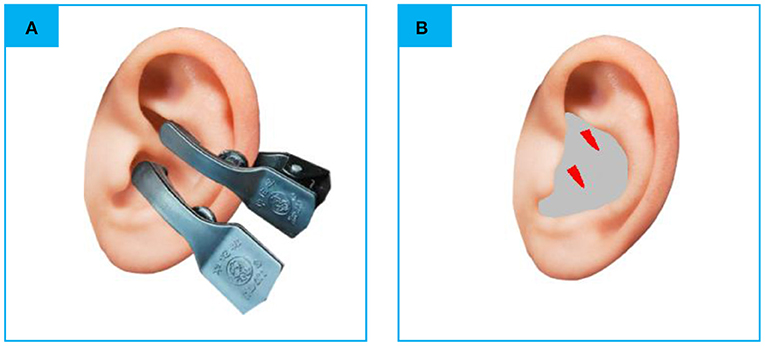
Figure 1. (A) The electrodes were attached to the surface of cymba conchae. (B) The stimulating place of taVNS. rs-fMRI, rest-state functional Magnetic Resonance Imaging; taVNS, Transcutaneous Auricular Vagus Nerve Stimulation (the red color areas).
Clinical assessments
All participants accepted Hamilton Rating Scale for Depression (HAMD) and Hamilton Anxiety Rating Scale (HAMA) to estimate the mental status of all the participants. Inclusion in the current study required patients to score >17, and the HCs would be excluded with a total score of HAMD or HAMA >7. The process of the current study is shown in Figure 2. In addition, we screened all patients' T2-weighted and structural images to rule out most of the severe metabolic or immune-related neuropsychiatric diseases, cerebrovascular diseases, inflammatory diseases of the central never system, and intracranial tumors.
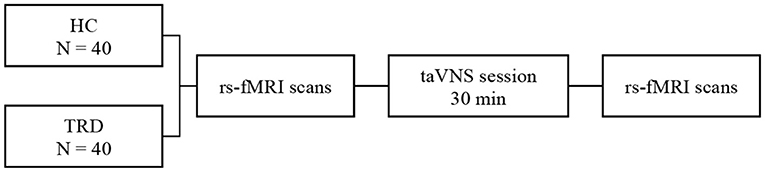
Figure 2. fMRI for instant taVNS were measured before and after the first treatment on the two groups.
Scan acquisition
Rest-state functional magnetic resonance imaging (rs-fMRI) was performed before and after the first 30 min taVNS session. Participants were told to keep their eyes closed and not fall asleep during the scan. The fMRI data were acquired by Siemens 3.0T Skyra scanner (Siemens; Munich, Germany). The scanning parameters were as follows. The BOLD gradient Echo Planar Imaging (EPI) sequence was used in functional images. Two hundred volumes lasted 6 min and 10 s, repeat time/echo time: 2,000/30 ms, flip angle = 90°, scanning field of view: 224 mm × 224 mm, matrix: 64 × 64, number of layers: 32, layer thickness/spacing: 3.5/0.6 mm. In a high-definition structure image, three-dimensional magnetization was used to prepare a fast gradient-echo sequence, repeat time/echo time: 2,530/2.98 ms, flip angle: 7 degrees, field of view: 256 mm × 256 mm, matrix: 64 × 64, and Layer thickness/spacing: 1.0/0 mm. We obtained 192 images.
Image processing
fMRI data pre-processing
DPABI (http://rfmri.org/DPABI) software (34), an SPM-based functional MRI pre-processing pipeline, was used for data pre-processing. The pre-processing steps were as follows. DICOM file was converted into NIFTI, and the first 10 time points were removed. The remaining 190 time points were slice-time corrected and realigned according to the Friston 24-parameter model. The nuisance signals, including linear trend, head-motion, signals of cerebrospinal fluid, and white matter, were regressed from the data (35). Then, the functional images were co-registered to the T1-weighted structural images, segmented through Voxel-Based Morphometry (VBM). Derived images were normalized to Montreal Neurological Institute (MNI) space according to transformation parameters estimated by VBM. All data used in this study satisfied the criteria of spatial movement in any direction <1.5 mm or degree. Subjects demonstrated no significant group differences in head-motion parameters. In this study, we failed to find significant differences in FD between groups (F = 0.4939, P = 0.4843).
The limitations of the signal-to-noise ratio and disputes in sampling and pre-processing strategies for fMRI data in the existing voxel-based analysis studies are sometimes contradictory. To better present the short-time intervention, we employed ReHo and FC to reveal the reproductive results.
Regional homogeneity (ReHo) is calculated using voxel-based Kendall's coefficient of concordance (KCC) for the time series of a given voxel with its nearest neighbors (24). ReHo maps were calculated using the unsmoothed and filtered (0.01–0.08Hz) images to remove physiological signals, such as heartbeat and respiration. Then ReHo maps were taken to mean ReHo maps by subtracting the mean voxel-wise ReHo in the entire brain and standardized into Z-value (zReHo Maps). Calculated zReHo maps were smoothed to MNI space with 6 mm Gaussian kernel full width at the half maximum smooth nucleus.
FC is the Pearson's correlations of the temporal fMRI signals between a Region of Interest (ROI) and all brain. Positive brain regions after ReHo statistics found by the above voxel based analyses would be used as the ROI for seed to voxel FC analysis. The AAL template of the WFU_Pick Atlas_v3.0 software was used to extract the seed points of the differential brain regions (36), Calculate the correlation coefficient (r) between the average time series of different brain regions and the time series of other voxels in the whole brain, Pearson's correlation coefficients were transformed into normally distributed scores according to the Fisher's R- to -Z transformation. Statistical inferences were the same as in the ReHo analysis.
Statistics
Clinical data analysis
Clinical data were analyzed using the SPSS 23.0 statistical software (IBM Corporation, Somers, New York). One-way analysis of variance (ANOVA) was used to compare age and education level among the groups, and the chi-square test was used to compare sex. A two-sample t-test was used to compare HAMD-17 and HAMA scores between the two groups, with P < 0.05 (two-tailed) as the threshold for statistical significance.
fMRI data analysis
In SPSS 25 (SPSS Inc., Chicago, IL, USA), two-sample t-tests and χ2 tests were applied to compare the baseline characteristics between the TRD and HC groups.
For the fMRI data, to determine the group × stimulation interaction effect between the two groups and the two scans, the main effects of group (the TRD group and the HC group) and time (baseline and post taVNS stimulation period), Covariates in the repeated measures ANCOVA and post hoc analyses were performed. Gender, age, education level, and framewise displacement (FD) metric (derived from Jenkinson's formula) of the four groups of subjects were used as covariates. The brain regions showing significant time differences in the HC group were excluded (37). The result for ANCOVA was the performance in Gaussian random field correction (GRF), combined voxel-wise P-value < 0.01 with cluster P-value < 0.05 (two-tailed). We performed post-hoc t-test analysis using DPARSF 5.1 software for two-by-two comparisons between groups, and Bonferroni correction was applied to the results, setting a threshold of P < 0.0125(0.05/4) for statistical significance.
To clarify the behavioral associations of brain function, we performed Pearson correlation analyses between the fMRI values and clinical scales in SPSS 25.
Results
Demographic characteristics and clinical results
The demographic and behavioral data are provided in Table 1, in which no significant differences in age and sex between TRD patients and HCs were observed. However, the HAMD and HAMA scores were higher for the TRD patients group (n = 40:40, P < 0.01).
fMRI results
ReHo
Group × stimulation interaction differences in ReHo are shown in Figure 3A and Tables 2, 3. Significant group × stimulation interactions on ReHo were observed in the right medial orbital frontal cortex.
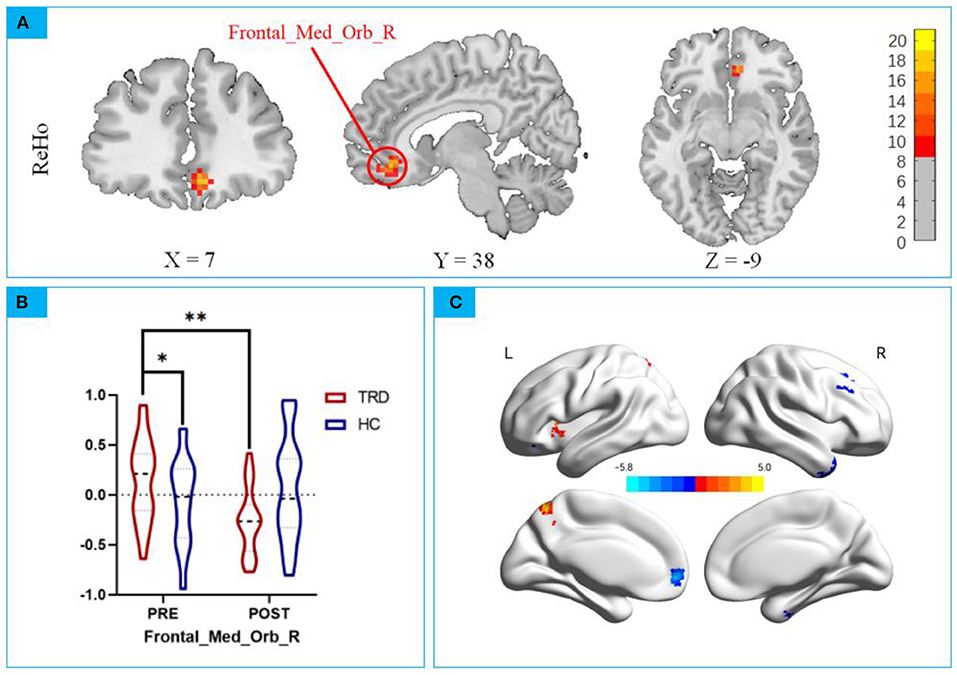
Figure 3. (A) Significant group × stimulation interactions on ReHo were observed in the right medial orbital frontal cortex; (B) post hoc analysis showed taVNS decreased lower activation in the TRD group than baseline. * p < 0.01; ** p < 0.001. (C) Main Effect of Time on ReHo. Blue colors represent decreased ReHo in after taVNS stimulation compared to before, while the hot colors represent the opposite.
Repeated measures ANCOVA revealed a significant interaction effect on the right medial orbital frontal cortex (F = 18.06, P < 0.0001, Figure 3A), post hoc analyses confirmed that the Reho value in the mOFC of the TRD group was significantly higher in the HC group in the baseline (t = 2.402, P < 0.001; Figure 3B). After instant taVNS stimulation, the ReHo value was significantly decreased (t = −4.314, P < 0.001; Figure 3B), Before and after treatment in the HC group, the difference was not statistically significant (t = 1.155, P = 0.2515; Figure 3B).
Significant main effect on time was found, Compared to before taVNS stimulation, in the right posterior lobes of the cerebellum, temporal inferior gyrus, left medial orbital frontal, and right superior frontal gyrus of the ReHo value decreased, Left precentral gyrus of the ReHo value increased (Table 4). No significant main effect on group effect was found. The 3D map is produced by the BrainNet Viewer toolbox (38) (Figure 3C).
FC
According to the ReHo results, we defined the right medial orbital frontal regions as ROI for the FC analyses (39). Repeated measures ANCOVA revealed a significant interaction effect on the left inferior parietal gyrus and left superior marginal gyrus (F = 11.6615, P < 0.001, F = 16.7520, P < 0.0001; Figure 4A; Tables 5, 6).
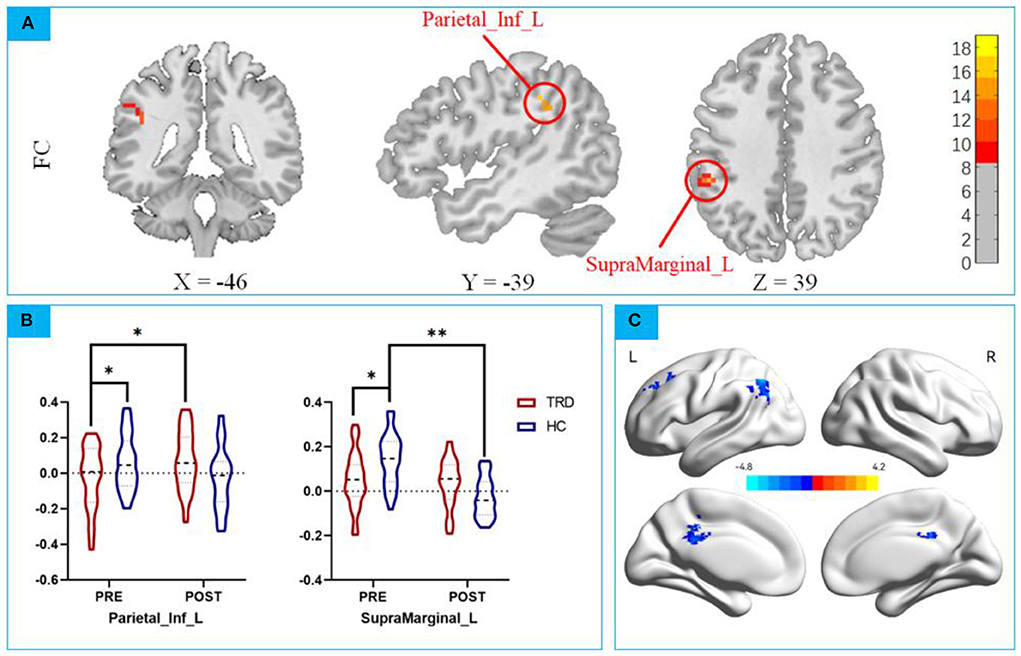
Figure 4. (A) Repeated measures ANCOVA revealed a significant interaction effect on the left inferior parietal gyrus and left superior marginal gyrus; (B) post hoc analysis mOFC-Based FC between the mOFC and IPG; post hoc analysis mOFC-Based FC between the mOFC and SMG. * p < 0.01; ** p < 0.001; (C) Main Effect of Time on FC of mOFC and SMG. Blue colors represent decreased FC of mOFC and SMG in POST-taVNS stimulation compared to PRE-taVNS.
ANCOVA of the mOFC-Based FC images showed that the group × time interaction effect of the mOFC with IPG showed statistical significance. Post hoc analyses confirmed that the FC strength in the TRD group was significantly lower in the HC group in the baseline (t = 2.133, P < 0.001; Figure 4B), after instant taVNS stimulation, the FC strength was significantly increased (t = −4.314, P < 0.001; Figure 4B). Before and after treatment in the HC group, the difference was not statistically significant (t = 1.155, P = 0.2515; Figure 4B).
ANCOVA of the mOFC-Based FC showed that the group × time interaction effect of the mOFC with the SMG showed statistical significance. Post hoc analyses confirmed that the FC strength in the TRD group was significantly lower in the HC group in the baseline (t = 3.236, P < 0.01; Figure 4B), after instant taVNS stimulation, the FC strength was increased (t = 1.623, P = 0.11339; Figure 4B), but the difference was not statistically significant. Furthermore, after treatment in the HC group, the FC strength was significantly lower in the baseline (t = 8.704, P < 0.001; Figure 4B).
No significant main effect was found in functional connection of mOFC and IPG. However Significant main effect on time was found in mOFC and SMG (Table 7), Compare before taVNS stimulation in the left middle Cingulate Gyrus, left middle frontal gyrus, and left Inferior parietal of the FC strength decreased. No significant main effect on group effect was found. The 3D map is produced by the BrainNet Viewer toolbox (38) (Figure 4C).
Correlation analyses
The HAMD and HAMA scores and ReHo/FC changes in the above-mentioned brain regions were not correlated (Figure 5).
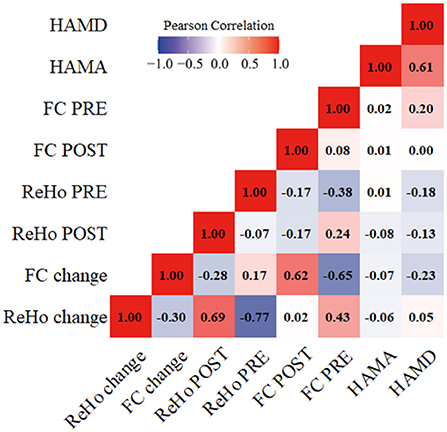
Figure 5. Correlations between the clinical scales' scores and the fMRI values. HAMD, Hamilton rating scale for depression; HAMA, Hamilton anxiety rating scale. ReHo, Regional homogeneity; FC, Functional connectivity; ReHo PRE/FC PRE, before taVNS treatment; ReHo POST/FC POST, after taVNS treatment; ReHo change/FC change, the difference in value before and after treatment.
Discussion
This study applied rs-fMRI technology to examine the effect of taVNS stimulation treatment on the brain activity of TRD. Our current study revealed that following taVNS stimulation treatment, TRD patients showed significantly reduced ReHo in the medial orbital frontal cortex (mOFC). ANCOVA of the mOFC-Based FC images revealed a significant interaction effect on the left inferior parietal gyrus (IPG) and left superior marginal gyrus (SMG). Among these regions, the HAMD and HAMA scores and ReHo/FC changes were not correlated.
taVNS can immediately regulate the synchrony of neuronal activity in the mOFC brain region of TRD patients
Several studies have confirmed that patients with TRD tend to have lower reward sensitivity (40). The mOFC is an integral part of the reward network and is associated with emotional information and sensory stimuli (41, 42). Fang et al. (43) illustrated that abnormal OFC-default network functional connection regulation was significantly related to relieving depressive symptoms. Studies have also demonstrated that the gray matter volume of OFC and the functional connection of OFC-amygdala in TRD patients are positively correlated, reflecting that TRD patients may suffer from greater stress and depression, and must call OFC more frequently to regulate the amygdala response to negative emotions (44). Compared with the HC group, it was found that ReHo in the mOFC brain region of TRD patients was decreased by taVNS immediate treatment. Based on previous studies (20–22), taVNS may have an immediate regulation effect on the spontaneous brain activity of mOFC in TRD patients to improve the status of the limbic system and reward circuit. The mOFC is also a key brain region involved in safety and risk decision-making. When TRD shows overactivation of OFC in the resting state, it will overreact to social rejection signals, thus increasing the risk of suicidal behavior. In addition, from the perspective of neural circuits, mOFC is also involved in the motivation control of punishment avoidance conditions, suggesting that its significant activation may simultaneously mediate the process of individuals' high avoidance motivation for pain (45). More than half of TRD patients report suicidal thoughts (46). After taVNS treatment, TRD patients' ReHo value immediately decreased, and the synchronization of neuronal activity was significantly reduced, which means that taVNS can effectively inhibit negative emotions such as suicidal tendencies in TRD patients. In conclusion, taVNS treatment may activate the emotion cognitive regulation function involved in mOFC and jointly regulate the negative emotions of TRD patients.
taVNS has an immediate regulatory effect on brain regions and brain networks related to the regulation of emotion
In this study, ANCOVA of the mOFC-Based FC images revealed a significant interaction effect on the left inferior parietal gyrus (IPG) and left superior marginal gyrus (SMG). Furthermore, Main Effect of Time on ReHo, Compared before taVNS stimulation, in the right posterior lobes of the cerebellum, temporal inferior gyrus, left medial orbital frontal and right superior frontal gyrus of the ReHo value decreased, left precentral gyrus of the ReHo value increased. Main Effect of Time on FC of mOFC and SMG, compare before taVNS stimulation, in the left middle Cingulate Gyrus, left middle frontal gyrus, and left Inferior parietal of the FC strength decreased. Abnormalities in these regions have also been extensively reported in previous studies, and the present study has accumulated more evidence for the relevant results (47–49). Previous studies have posited that rumination may play a pivotal role in the psychopathology of TRD (50, 51), Default mode network (DMN), such as the medial prefrontal cortex (MPFC) and posterior cingulate cortex/precuneus. Frontoparietal control network (FPCN) regions, including the inferior parietal lobule (IPL), dorsal lateral prefrontal cortex (DLPFC), and superior marginal gyrus (SMG) (52). DMN and FPCN are closely related to emotion and cognitive processing (53), Silani et al. (54) showed that the SMG is a key brain area for emotion control. The FPCN anatomically connects the DMN and the dorsal attention network (DAN), and its function is to integrate stored internal representations with external environmental information, and to simultaneously resolve multiple interdependent emergencies and response mappings to conflicting stimuli, assigning work. Memory and attention resources. Our study found that the FC strength in the TRD group was significantly lower in the HC group in the baseline that the top-down regulation of TRD emotion is abnormal. And taVNS treatment can reduce the neural activity level of the mOFC and increase the neural activity intensity of the SMG and the IPL, which has a dynamic regulatory effect on the brain function of TRD patients. This suggests that taVNS can regulate the negative emotions of TRD from bottom to top (55). In conclusion, taVNS has an immediate regulatory effect on brain regions and brain networks related to the regulation of emotion.
Interestingly, we also found that after taVNS intervention in the HC group, the FC strength was significantly lower in the baseline. The taVNS immediate stimulation also had modulating effects in healthy individuals. Previous studies have also found short-term antidepressant therapy for healthy individuals reduce activity in the amygdala, OFC, superior frontal gyrus, and precentral gyrus, and SMG during emotional stimulation. These brain regions are associated with the negative affective of depression (56–64). Our taVNS treatment is consistent with studies on the regulatory effect of antidepressants on healthy individuals, which is worthy of further study.
Limitations
First, the study's sample size is small, and thus the results may be biased. Fewer brain areas are immediately adjusted to cause changes, which may be different from those after long-term treatment. Second, this study only used the commonly used research indicators of resting-state fMRI to observe the changes in the immediate effect mechanism of the brain, and the indicators used are not comprehensive enough.
In our future studies, the sample size will be expanded, and a variety of functional imaging research methods will be used to further explore the brain mechanism of the efficacy of taVNS on TRD patients. More indicators, including arterial spin labeling (ASL), GABA and other indicators of TRD patients, will need to be carried out for statistical analysis to improve the scientific value of this study.
Conclusions
In this study, we found taVNS can immediately regulate the synchrony of neuronal activity in the mOFC brain region of TRD patients. ANCOVA of the mOFC-Based FC images revealed a significant interaction effect on the IPG and SMG. In summary, the potential mechanism of taVNS treatment for TRD may be to enhance the function of emotion regulation circuits, monitor and manage negative emotions. Activity of emotion-processing networks, reduces the processing of negative emotions in TRD. Through taVNS treatment, the abnormal brain regions in TRD can be normalized, or even reversed, which may play a compensatory role in the reduction of depressive symptoms and involving DMN, FPCN and Reward Network.
Data availability statement
The original contributions presented in the study are included in the article/supplementary material, further inquiries can be directed to the corresponding authors.
Ethics statement
The studies involving human participants were reviewed and approved by Ethics Committee of Guang'anmen Hospital, China Academy of Chinese Medical Sciences, China (No. 2017-021-SQ). The patients/participants provided their written informed consent to participate in this study. Written informed consent was obtained from the individual(s) for the publication of any potentially identifiable images or data included in this article.
Author contributions
JF conceived and designed this experiment. This article was written mainly by YM and ZW. Patients were recruited and assessed by JS and CG. fMRI data were collected by ZW and JH. YM and ZD drew the diagrams and carried out the statistical analysis of data. YH and LZ performed fMRI on the subjects. JF and YLi reviewed the article. Text correction was done by YLu and LC. All authors contributed to the article and approved the submitted version.
Funding
This research was supported by the Science and Technology Innovation Project of China Academy of Chinese Medical Sciences (CI2021A03301), National Natural Science Foundation of China (82174282 and 81774433), and National Key Research and Development Program of China (2018YFC1705800).
Acknowledgments
The authors would like to thank TopEdit (www.topeditsci.com) for its linguistic assistance during the preparation of this manuscript.
Conflict of interest
The authors declare that the research was conducted in the absence of any commercial or financial relationships that could be construed as a potential conflict of interest.
Publisher's note
All claims expressed in this article are solely those of the authors and do not necessarily represent those of their affiliated organizations, or those of the publisher, the editors and the reviewers. Any product that may be evaluated in this article, or claim that may be made by its manufacturer, is not guaranteed or endorsed by the publisher.
References
1. Hasin DS, Sarvet AL, Meyers JL, Saha TD, Ruan WJ, Stohl M, et al. Epidemiology of adult DSM-5 major depressive disorder and its specifiers in the United States. JAMA Psychiatry. (2018) 75:336–46. doi: 10.1001/jamapsychiatry.2017.4602
2. Ahmed HU, Hossain MD, Aftab A, Soron TR, Alam MT, Chowdhury MW, et al. Suicide and depression in the World Health Organization South-East Asia Region: a systematic review. WHO South-East Asia J Public Health. (2017) 6:60:6. doi: 10.4103/2224-3151.206167
3. Gaynes BN, Lux L, Gartlehner G, Asher G, Forman-Hoffman V, Green J, et al. Defining treatment-resistant depression. Depress Anxiety. (2020) 37:134–45. doi: 10.1002/da.22968
4. Bergfeld IO, Mantione M, Figee M, Schuurman PR, Lok A, Denys D, et al. Treatment-resistant depression and suicidality. J Affect Disord. (2018) 235:362–7. doi: 10.1016/j.jad.2018.04.016
5. Serretti A, Fabbri C. 6 Signs your patient is at risk for treatment-resistant depression. Psychiatric Times. (2017). Available online at: https://www.psychiatrictimes.com/view/6-signs-your-patient-risk-treatment-resistant-depression (accessed August 30, 2017).
6. Murphy JA, Sarris J, Byrne GJA. Review of the conceptualisation and risk factors associated with treatment-resistant depression. Depress Res Treat. (2017) 2017:4176825. doi: 10.1155/2017/4176825
7. Bennabi D, Aouizerate B, El-Hage W, Doumy O, Moliere F, Courtet P, et al. Risk factors for treatment resistance in unipolar depression: a systematic review. J Affect Disord. (2015) 171:137–41. doi: 10.1016/j.jad.2014.09.020
8. Fife D, Blacketer C, Reps JM, Ryan P. Database Studies of Treatment-Resistant Depression Should Take Account of Adequate Dosing. Prim Care Companion CNS Disord. (2018) 20:18m02274. doi: 10.4088/PCC.18m02274
9. Cepeda MS, Reps J, Ryan P. Finding factors that predict treatment-resistant depression: results of a cohort study. Depress Anxiety. (2018) 35:668–73. doi: 10.1002/da.22774
10. Der-Avakian A, Markou A. The neurobiology of anhedonia and other reward-related deficits. Trends Neurosci. (2012) 35:68–77. doi: 10.1016/j.tins.2011.11.005
11. Bracht T, Mertse N, Walther S, Lüdi K, Breit S, Federspiel A, et al. Link between structural connectivity of the medial forebrain bundle, functional connectivity of the ventral tegmental area, and anhedonia in unipolar depression. NeuroImage Clin. (2022) 34:102961. doi: 10.1016/j.nicl.2022.102961
12. Yee DM, Crawford JL, Lamichhane B, Braver TS. Dorsal anterior cingulate cortex encodes the integrated incentive motivational value of cognitive task performance. J Neurosci. (2021) 41:3707–20. doi: 10.1523/JNEUROSCI.2550-20.2021
13. Jackson SA, Horst NK, Pears A, Robbins TW, Roberts AC. Role of the perigenual anterior cingulate and orbitofrontal cortex in contingency learning in the marmoset. Cerebral Cortex (New York, NY). (2016) 26:3273–84. doi: 10.1093/cercor/bhw067
14. Khawam EA, Laurencic G, Malone DA. Side effects of antidepressants: an overview. Cleve Clin J Med. (2006) 73:351–61. doi: 10.3949/ccjm.73.4.351
15. Chen LM, Li XJ, Xu K, Guo CL, Zhang GL, Han M, et al. Functional MRI-based study on neuromechanism of trans-auricular vagus nerve stimulation for treatment-resistant depression. Acupuncture Res. (2021) 46:869–74. doi: 10.13702/j.1000-0607.20210241
16. Henry TR. Therapeutic mechanisms of vagus nerve stimulation.Neurology. (2002) 59:S3–14. doi: 10.1212/WNL.59.6_suppl_4.S3
17. Peuker ET, Filler TJ. The nerve supply of the human auricle. Clin Anat. (2002) 15:35–7. doi: 10.1002/ca.1089
18. Hein E Nowak M Kiess O Biermann T and Bayerlein K. Kornhuber J,Kraus T. Auricular transcutaneous electrical nerve stimulation in depressed patients: a randomized controlled pilot study. J Neural Transm. (2013) 120:821–7. doi: 10.1007/s00702-012-0908-6
19. Rong PJ, Fang JL, Wang LP, Meng H, Liu J, Ma YG, et al.Transcutaneous vagus nerve stimulation for the treatment of depres-sion: A study protocol for a double blinded randomized clinical trial. BMC Complement Altern Med. (2012) 12:255. doi: 10.1186/1472-6882-12-255
20. Rong P, Liu J, Wang L, Liu R, Fang J, Zhao J, et al. Effect of transcutaneous auricular vagus nerve stimulation on major depressive disorder: A nonrandomized controlled pilot study. J Affect Disord. (2016) 195:172–9. doi: 10.1016/j.jad.2016.02.031
21. Fang J, Hong Y, Fan Y, Liu J. Brain response to transcutaneous electronical stimulation on auricular concha of the healthy subjects using fmri. Chin J Magnetic Reson Imaging. (2014) 5:416–22. doi: 10.3969/j.issn.1674-8034.2014.06.003
22. Xu K, Li XJ, Fang JL, Chen LM, Gao DQ, Hong Y. Clinical observation on effect in auricular electro-acupuncture on treatment-resistant depression. Medica-World Sci Technol. (2019) 21:2266–71. doi: 10.11842/wst.20190718004
23. Pandarakalam JP. Challenges of treatment-resistant depression. Psychiatr Danub. (2018) 30:273–84. doi: 10.24869/psyd.2018.273
24. Chen CH, Suckling J, Lennox BR, Ooi C, Bullmore ET. A quantitative meta-analysis of fMRI studies in bipolar disorder. Bipolar Disord. (2011) 13:1–15. doi: 10.1111/j.1399-5618.2011.00893.x
25. Mwansisya TE, Hu A, Li Y, Chen X, Wu G, Huang X, et al. Task and resting-state fMRI studies in first-episode schizophrenia: a systematic review. Schizophr Res. (2017) 189:9–18. doi: 10.1016/j.schres.2017.02.026
26. Dapretto M, Davies MS, Pfeifer JH, Scott AA, Sigman M, Bookheimer SY, et al. Understanding emotions in others: mirror neuron dysfunction in children with autism spectrum disorders. Nat Neurosci. (2006) 9:28–30. doi: 10.1038/nn1611
27. Drysdale AT, Grosenick L, Downar J, Dunlop K, Mansouri F, Meng Y, et al. Resting-state connectivity biomarkers define neurophysiological subtypes of depression. Nat Med. (2017) 23:28–38. doi: 10.1038/nm.4246
28. Borserio BJ, Sharpley CF, Bitsika V, Sarmukadam K, Fourie PJ, Agnew LL, et al. Default mode network activity in depression subtypes. Rev Neurosci. (2021) 32:597–613. doi: 10.1515/revneuro-2020-0132
29. Guo CC, Hyett MP, Nguyen VT, Parker GB, Breakspear MJ. Distinct neurobiological signatures of brain connectivity in depression subtypes during natural viewing of emotionally salient films. Psychol Med. (2016) 46:1535–45. doi: 10.1017/S0033291716000179
30. Zang Y, Jiang T, Lu Y, He Y, Tian L. Regional homogeneity approach to fMRI data analysis. Neuroimage. (2004) 22:394–400. doi: 10.1016/j.neuroimage.2003.12.030
31. Friston KJ, Frith CD, Liddle PF, Frackowiak RSJ. Functional connectivity: the principal-component analysis of large (PET) data sets. J Cereb Blood Flow Metab. (1993) 13:5–14. doi: 10.1038/jcbfm.1993.4
32. Jiao Y, Guo X, Luo M, Li S, Liu A, Zhao Y, et al. Effect of transcutaneous vagus nerve stimulation at auricular concha for insomnia: a randomized clinical trial. Evid Based Complement Altern Med. (2020) 2020:6049891. doi: 10.1155/2020/2536573
33. Liu J, Fang J, Wang Z, Rong P, Hong Y, Fan Y, et al. Transcutaneous vagus nerve stimulation modulates amygdala functional connectivity in patients with depression. J Affect Disord. (2016) 205:319–26. doi: 10.1016/j.jad.2016.08.003
34. Yan CG, Wang XD, Zuo XN, Zang YF. DPABI: Data Processing and Analysis for (Resting-State) Brain Imaging. Neuroinformatics. (2016) 14:339–51. doi: 10.1007/s12021-016-9299-4
35. Friston KJ, Williams S, Howard R, Frackowiak RS, Turner R. Movement-related effects in fMRI time-series. Magnetic Resonan Med. (1996) 35:346–55. doi: 10.1002/mrm.1910350312
36. Maldjian JA, Laurienti PJ, Kraft RA, Burdette JH. An automated method for neuroanatomic and cytoarchitectonic atlas-based interrogation of fMRI data sets. Neuroimage. (2003) 19:1233–9. doi: 10.1016/S1053-8119(03)00169-1
37. Wang L, Li X, Li K, Su Y, Zeng Y, Zhang Q, et al. Mapping the effect of escitalopram treatment on amplitude of low-frequency fluctuations in patients with depression: a resting-state fMRI study. Metab Brain Dis. (2017) 32:147–54. doi: 10.1007/s11011-016-9871-5
38. Xia M, Wang J, He Y. BrainNet Viewer: A Network Visualization Tool for Human Brain Connectomics. PLoS ONE. (2013) 8. doi: 10.1371/journal.pone.0068910
39. Deng R, Yang X, Meng Y, Tao Y, Wang H, Li X, et al. Data-driven study on resting-state functional magnetic resonance imaging during early abstinence of alcohol dependence in male patients and its predictive value for relapse. BMC Psychiatry. (2022) 22. doi: 10.1186/s12888-022-03782-w
40. Klawohn J, Joyner K, Santopetro N, Brush CJ, Hajcak G. Depression reduces neural correlates of reward salience with increasing effort over the course of the progressive ratio task. J Affect Disord. (2022) 307:294–300. doi: 10.1016/j.jad.2022.03.051
41. Klawohn J, Burani K, Bruchnak A, Santopetro N, Hajcak G. Reduced neural response to reward and pleasant pictures independently relate to depression. Psychol Med. (2021) 51:741–9. doi: 10.1017/S0033291719003659
42. Rappaport BI, Kandala S, Luby JL, Barch DM. Brain reward system dysfunction in adolescence: current, cumulative, and developmental periods of depression. Am J Psychiatry. (2020) 177:754–63. doi: 10.1176/appi.ajp.2019.19030281
43. Fang J, Rong P, Hong Y, Fan Y, Liu J, Wang H, et al. Transcutaneous vagus nerve stimulation modulates default mode network in major depressive disorder. Biol Psychiatry. (2016) 79:266–73. doi: 10.1016/j.biopsych.2015.03.025
44. Mao Y, Zuo XN, Ding C, Qiu J. OFC and its connectivity with amygdala as predictors for future social anxiety in adolescents. Dev Cogn Neurosci. (2020) 44:100804. doi: 10.1016/j.dcn.2020.100804
45. Taylor WD, Zald DH, Felger JC, Christman S, Claassen DO, Horga G, et al. Influences of dopaminergic system dysfunction on late-life depression. Mol Psychiatry. (2022) 27:180–91. doi: 10.1038/s41380-021-01265-0
46. Kalin NH. Insights into suicide and depression. Am J Psychiatry. (2020) 177:877–80. doi: 10.1176/appi.ajp.2020.20081207
47. Gong J, Wang J, Qiu S, Chen P, Luo Z, Wang J, et al. Common and distinct patterns of intrinsic brain activity alterations in major depression and bipolar disorder: voxel-based meta-analysis. Transl Psychiatry. (2020) 10. doi: 10.1038/s41398-020-01036-5
48. Zhang Y, Shao J, Wang X, Chen Z, Liu H, Pei C, et al. Functional impairment-based segmentation of anterior cingulate cortex in depression and its relationship with treatment effects. Human Brain Mapp. (2021) 42:4035–47. doi: 10.1002/hbm.25537
49. Zhang B, Qi S, Liu S, Liu X, Wei X, Ming D, et al. Altered spontaneous neural activity in the precuneus, middle and superior frontal gyri, and hippocampus in college students with subclinical depression. BMC Psychiatry. (2021) 21. doi: 10.1186/s12888-021-03292-1
50. Vidal S, Jermann F, Aubry J, Richard-Lepouriel H, Kosel M. Effect of Ketamine on Rumination in Treatment-Resistant Depressive Patients. J Clin Psychopharmacol. (2020) 40:607–10. doi: 10.1097/JCP.0000000000001305
51. Foroughi A, Sadeghi K, Parvizifard A, Parsa Moghadam A, Davarinejad O, Farnia V, et al. The effectiveness of mindfulness-based cognitive therapy for reducing rumination and improving mindfulness and self-compassion in patients with treatment-resistant depression. Trends Psychiatry Psychother. (2020) 42:138–46. doi: 10.1590/2237-6089-2019-0016
52. Rai S, Griffiths KR, Breukelaar IA, Barreiros AR, Chen W, Boyce P, et al. Default-mode and fronto-parietal network connectivity during rest distinguishes asymptomatic patients with bipolar disorder and major depressive disorder. Transl Psychiatry. (2021) 11:547. doi: 10.1038/s41398-021-01660-9
53. Dai Z, Shao J, Zhou H, Chen Z, Zhang S, Wang H, et al. Disrupted fronto-parietal network and default-mode network gamma interactions distinguishing suicidal ideation and suicide attempt in depression. Progr Neuro-Psychopharmacol Biol Psychiatry. (2022) 113:110475. doi: 10.1016/j.pnpbp.2021.110475
54. Silani G, Bird G, Brindley R, Singer T, Frith CD, Frith U, et al. Levels of emotional awareness and autism: An fMRI study. Soc Neurosci. (2008) 3:112–97. doi: 10.1080/17470910701577020
55. Kaiser R, Andrews-Hanna JR, Wager TD, Pizzagalli DA. Large-scale network dysfunction in major depressive disorder: a meta-analysis of resting-state functional connectivity. JAMA Psychiatry. (2015) 72:603–11. doi: 10.1001/jamapsychiatry.2015.0071
56. Del-Ben CM, Deakin JF, McKie S, Delvai NA, Williams SR, Elliott R, et al. The effect of citalopram pretreatment on neuronal responses to neuropsychological tasks in normal volunteers: an FMRI study. Neuropsychopharmacology. (2005) 30:1724–34. doi: 10.1038/sj.npp.1300728
57. Takahashi H, Yahata N, Koeda M, Takano A, Asai K, Suhara T, et al. Effects of dopaminergic and serotonergic manipulation on emotional processing: a pharmacological fMRI study. Neuroimage. (2005) 27:991–1001. doi: 10.1016/j.neuroimage.2005.05.039
58. Harmer CJ, Mackay CE, Reid CB, Cowen PJ, Goodwin GM. Antidepressant drug treatment modifies the neural processing of nonconscious threat cues. Biol Psychiatry. (2006) 59:816–20. doi: 10.1016/j.biopsych.2005.10.015
59. Anderson IM, Del-Ben CM, Mckie S, Richardson P, Williams SR, Elliott R, et al. Citalopram modulation of neuronal responses to aversive face emotions: a functional MRI study. Neuroreport. (2007) 18:1351–5. doi: 10.1097/WNR.0b013e3282742115
60. Bigos KL, Pollock BG, Aizenstein HJ, Fisher PM, Bies RR, Hariri AR, et al. Acute 5-HT reuptake blockade potentiates human amygdala reactivity. Neuropsychopharmacology. (2008) 33:3221–5. doi: 10.1038/npp.2008.52
61. Simmons AN, Arce E, Lovero KL, Stein MB, Paulus MP. Subchronic SSRI administration reduces insula response during affective anticipation in healthy volunteers. Int J Neuropsychopharmacol. (2009) 12:1009–20. doi: 10.1017/S1461145709990149
62. Murphy SE, Norbury R, O'Sullivan U, Cowen PJ, Harmer CJ. Effect of a single dose of citalopram on amygdala response to emotional faces. Br J Psychiatry. (2009) 194:535–40. doi: 10.1192/bjp.bp.108.056093
63. Brühl AB, Kaffenberger T, Herwig U. Serotonergic and noradrenergic modulation of emotion processing by single dose antidepressants. Neuropsychopharmacology. (2010) 35:521–33. doi: 10.1038/npp.2009.159
Keywords: treatment-resistant depression, transcutaneous auricular vagus nerve stimulation, rest-state functional magnetic resonance imaging (rs-fMRI), amplitude of low-frequency fluctuations, regional homogeneity, functional connectivity, orbital frontal cortex
Citation: Ma Y, Wang Z, He J, Sun J, Guo C, Du Z, Chen L, Luo Y, Gao D, Hong Y, Zhang L, Liu Y and Fang J (2022) Transcutaneous auricular vagus nerve immediate stimulation treatment for treatment-resistant depression: A functional magnetic resonance imaging study. Front. Neurol. 13:931838. doi: 10.3389/fneur.2022.931838
Received: 29 April 2022; Accepted: 19 July 2022;
Published: 01 September 2022.
Edited by:
Wei Zhang, Peking University, ChinaReviewed by:
Jie Yang, Central South University, ChinaIvan V. Brak, State Scientific Research Institute of Physiology and Basic Medicine, Russia
Copyright © 2022 Ma, Wang, He, Sun, Guo, Du, Chen, Luo, Gao, Hong, Zhang, Liu and Fang. This is an open-access article distributed under the terms of the Creative Commons Attribution License (CC BY). The use, distribution or reproduction in other forums is permitted, provided the original author(s) and the copyright owner(s) are credited and that the original publication in this journal is cited, in accordance with accepted academic practice. No use, distribution or reproduction is permitted which does not comply with these terms.
*Correspondence: Yong Liu, MTkwOTc2ODEzOSYjeDAwMDQwO3FxLmNvbQ==; Jiliang Fang, RmFuZ21naCYjeDAwMDQwOzE2My5jb20=
†These authors have contributed equally to this work and share first authorship