- 1School of Human Sciences, The University of Western Australia, Perth, WA, Australia
- 2Ear Science Institute Australia, Subiaco, WA, Australia
- 3Centre for Healthy Ageing, College of Science, Health, Engineering and Education, Murdoch University, Perth, WA, Australia
- 4School of Medical and Health Sciences, Edith Cowan University, Joondalup, WA, Australia
- 5Department of Biomedical Sciences, Faculty of Medicine and Health Sciences, Macquarie University, Sydney, NSW, Australia
- 6Ear Science Centre, School of Surgery, The University of Western Australia, Perth, WA, Australia
Behavioral measures, such as pure-tone audiometry (PTA), are commonly used to determine hearing thresholds, however, PTA does not always provide reliable hearing information in difficult to test individuals. Therefore, objective measures of hearing sensitivity that require little-to-no active participation from an individual are needed to facilitate the detection and treatment of hearing loss in difficult to test people. Investigation of the reliability of the auditory steady-state response (ASSR) for measuring hearing thresholds in older adults is limited. This study aimed to investigate if ASSR can be a reliable, objective measure of frequency specific hearing thresholds in older adults. Hearing thresholds were tested at 500 Hz, 1000 Hz, 2000 Hz, and 4000 Hz in 50 participants aged between 60 and 85 years old, using automated PTA and ASSR. Hearing thresholds obtained from PTA and ASSR were found to be significantly correlated (p < .001) in a cohort consisting of participants with normal hearing or mild hearing loss. ASSR thresholds were significantly higher as compared to PTA thresholds, but for the majority of cases the difference remained within the clinically acceptable range (15 dB). This study provides some evidence to suggest that ASSR can be a valuable tool for estimating objective frequency-specific hearing thresholds in older adults and indicate that ASSR could be useful in creating hearing treatment plans for older adults who are unable to complete behavioral PTA. Further research on older adults is required to improve the methodological features of ASSR to increase consistency and reliability, as well as minimize some of the limitations associated with this technique.
Introduction
Sensory processing declines across adulthood, with one third of those over the age of 65 being affected by disabling hearing loss (HL) (1). It is estimated that around 466 million people worldwide have disabling HL, accounting for over 5% of the world's population (1). The World Health Organization estimates that untreated hearing loss has an annual global cost of approximately US$750 billion. The identification of and addressing HL can be cost-effective and beneficial at an individual and societal level. Hearing loss can have great impact on quality of daily living and on communication (2–4). In addition, untreated HL is associated with multiple co-morbidities, including anxiety (5), depression (6), social isolation (7), loneliness (8) and poor physical health (9).
Assessing auditory acuity is frequently obtained using pure-tone audiometry (PTA), the gold standard for evaluating hearing threshold status, however, PTA does not always provide reliable hearing information in difficult to test individuals (10). This can be due to lack of cooperation during the assessment, inability to maintain attention and focus or limited understanding of test instructions (11). Older adults with cognitive impairment, particularly at moderate-to-severe levels, can have difficulties in performing behavioral hearing assessments, due to their diminished ability to maintain attention and understand test instructions (12). However, detecting and treating HL in those with cognitive impairment can have positive implications on their cognitive performance (13–16), social interaction (15) and overall quality of life (2). Therefore, being able to objectively measure hearing function in older adults who are unable to complete behavioral PTA is of great interest.
The auditory brainstem response (ABR), a far-field auditory electrophysiological test conducted using surface electrodes, provides an objective alternative method for measuring hearing function and is used particularly in infants and children not suited for behavioral PTA. ABR includes auditory evoked potentials from the eighth cranial nerve (auditory nerve) and neurons along the brainstem auditory pathway after presentation of an acoustic stimulus (17). ABR evoked using click stimuli provides a high degree of information regarding the integrity of the central and peripheral auditory pathways, particularly due to the reproducibility and stability of the waveform (18, 19).
However, a major limitation of the click-evoked ABR for assessing hearing sensitivity is its inability to determine frequency-specific hearing thresholds (20). As click-evoked ABR collects whole basilar membrane responses, it is difficult to accurately determine participating frequency ranges, which limits its effectiveness in providing accurate information for hearing loss intervention and rehabilitation. Commonly, ABR recordings are also dependent on the subjective interpretation of a recorded waveform by the examiner in order to evaluate if a response is present or not, and therefore, ABR results can be influenced by the examiner's experience and expertise (21, 22). Additionally, research has suggested that ABR testing cannot be used to evaluate severe-to-profound hearing loss, as it provides inadequate measures at thresholds >90 dB eHL (23, 24).
Tone-evoked (tone-burst) ABR can be used to assess responses in one ear to one frequency at a time, however, this is time consuming and, like click-evoked ABR, does not provide responses in cases of severe and profound hearing loss (21). Recently, a new ABR testing paradigm, parallel ABR (pABR), has been proposed to provide frequency-specific hearing threshold measures for multiple octave frequencies in both ears simultaneously (25). This new ABR technique uses independently randomized sequences of tone-burst stimuli to acquire ABR waveforms. pABR has been suggested to acquire waveforms with similar morphology of traditional ABR in a fraction of the recording time (25, 26). However, pABR technique still requires examiners to subjectively interpret recorded waveforms and its performance in assessing participants with HL or from different age groups has not been established yet.
Auditory steady-state responses (ASSR) has been suggested to be another objective audiometry test that can overcome some of the limitations associated with ABR (27). Similar to ABR, ASSR is a scalp-recorded auditory evoked potential (28). ASSR is a periodic electrical response evoked by periodically modulated tones, which is used to assess hearing sensitivity in patients of all ages and various degrees of sensorineural hearing loss without the need for patient participation (29, 30). Unlike ABR, which is evoked by a short stimuli at a relatively low repetition rate, ASSR is evoked using repeated pure tones at high repetition rates. ASSR uses amplitudes and phases in a spectral domain and is dependent on peak detection across a spectrum, meaning that the response is periodic and phase-locked to a modulation envelope (28). ASSR can be detected using frequency, time or spectral based analyses (28, 31). The neural generators of ASSR are dependent on the modulation frequencies used in the testing. Higher cortical and subcortical structures are suggested to generate responses to slower modulation rates (<50 Hz), while the auditory nerve and brainstem are suggested to respond to faster modulation rates (>80 Hz) (32, 33).
ASSR can be used to evaluate hearing sensitivity at a range of frequencies similar to behavioral PTA, using simultaneous stimulation and evaluation of multiple frequencies binaurally (33). ASSR results are presented as an electrophysiological audiogram, allowing for easy interpretation of hearing quality and for the preparation of medical reports (34). ASSR has also been suggested to provide better hearing data in comparison to ABR, in cases with severe-to-profound sensorineural hearing loss of 90 dB HL or greater (22, 35). Moreover, ASSR thresholds (spectrum of the response) are predicted by the stimulus spectrum and do not require subjective interpretations of the recorded responses, therefore overcoming some of the common limitations associated with other clinical audiometric tests, e.g., ABR.
Previous research suggests that ASSR can be a reliable predictor of hearing thresholds when compared to PTA in both children and adults (10, 11, 30, 35–37). However, there is no research comparing hearing threshold measures between PTA and ASSR in a cohort consisting of only older adults (aged 60 years and over), to date, research has only included older adults as part of a mixed aged (ranging from children to older adults) cohort when comparing PTA and ASSR thresholds (11, 30, 37). There is evidence to suggest that age-related changes in neural envelope processing and phase-locking may result in decreased ASSR responses in older adults compared to young adults or children (38–41). Therefore, the reliability of ASSR as a measure of hearing acuity specifically for older adults remains unclear. The aim of this study is to investigate if ASSR can be a reliable objective measure of frequency-specific hearing thresholds in older adults.
Methods
Participants
Community-dwelling (i.e., from the general population) older adults (aged 60 years and over) were recruited from an ongoing longitudinal research project known as the Western Australia Memory Study (WAMS). All procedures undertaken in this study were conducted in accordance with ethical approval (HPH-139) from the Ramsay Health Care WA| SA Human Research Ethics Committee (previously, the Hollywood Private Hospital Ethics Committee, Western Australia). As part of the WAMS, participants underwent comprehensive neuropsychological assessments, using self-reports and informant-reports questionnaires and surveys. All participants completed a demographic questionnaire and provided informed consent. Participants with current or previous diagnosis of a neurodegenerative disease, stroke or psychotic disorders were excluded from this study. Only older adults who performed within the normal range on cognitive measures were included in this study. More information on the neuropsychological and psychological assessments used in the WAMS can be found in Sohrabi et al. (42). Participants with unilateral deafness or already wearing hearing aids were excluded from this study. All participants underwent an otoscopic examination, a PTA and an ASSR, in the order given, in the same session/day. Only participants with normal otoscopic findings were included in the study.
PTA Recording
Pure tone audiometry was conducted (air-conduction) bilaterally at 500, 1000, 2000, 4000, 8000 Hz using the KUDUwave 5000 system, Type 2 clinical audiometer (Emoyo, Johannesburg, South Africa). Tones were presented via insert earphones which were inserted in the ear canals with circumaural headphones placed over the ears. An automated threshold-seeking paradigm was used to establish hearing threshold. At each frequency, threshold levels were determined using the Hughson-Westlake (43) procedure, by increasing increments of 10 dB followed by decreasing increments of 5 dB. Participants were required to press a button in response to any tones they heard during the assessment. Degree of HL was classified based on the American Speech-Language-Hearing Association (ASHA) classification system adapted from Clark 1981 (44).
ASSR Recording Parameters
ASSR was performed in an electrically shielded and sound attenuated room. Participants were tested while awake and in a relaxed Fowler's position (45). Air-conducted stimuli were presented to the left and right ear simultaneously via ER-3A insert earphones. Acoustic stimuli were generated and presented by the Chartr EP system (Version 5.3, GN Otometrics). Four carrier frequencies: 500, 1000, 2000, and 4000 Hz, were tested using an automated multiple ASSR technique that utilizes an algorithm that uses a Fourier Linear Combiner with an adaptive filter and circular statistical analysis (46). This means that the four carrier frequencies were tested simultaneously in both ears at each modulation frequency. 100% amplitude modulation and 20% frequency modulation were used for all carrier frequencies, with the response confidence set at 95% as predefined by the system manufacturer. The modulation frequency varied for each carrier frequency: modulation rates were 88, 80, 96, and 92 Hz for the right and 90, 82, 98, and 94 Hz for the left ear, for 500, 1000, 2000, and 4000 Hz carrier frequencies, respectively. A gain of 200 k, a low-pass filter at 105 Hz and a high-pass filter at 65 Hz were used.
ASSR recordings were obtained using four Ag/AgCl disc electrodes which were placed according to the International Electrode System (IES) 10-20; two inverting (reference) electrodes on each mastoid (one behind left ear and one behind right ear) behind the ear, non-inverting (active/recording) electrode at vertex (Cz) and ground electrode on the lower forehead. Prior to recording, the skin was prepared for electrode placement with a mild abrasive to obtain electrode impedances under 5 KΩ. ASSR measurements were performed using a descending procedure, by recording electrical responses while reducing the intensity of the acoustic signal in 10 dB steps until the threshold. The threshold was defined as the minimum intensity of detected responses, with a maximum of 7 min search time for each frequency allowed. Participants were not required to actively participate during ASSR recordings. Default correction factors (500 Hz−20 dB HL, 1000 Hz−10 dB HL, 2000 Hz−10 dB HL, 4000 Hz– 10 dB HL) on the Chartr EP system were applied to all final audiograms obtained from ASSR. To minimize artifacts and noise interference as a result of body movement, participants were instructed to stay still during the recording.
Statistical Analysis
After the PTA and ASSR measurements, statistical analysis was performed using IBM SPSS Statistics, version 25.0 (IBM Corp, Armonk, NY). Continuous variables were presented as a mean with standard deviation, and categorical variables were presented as absolute numbers and percentages. A Student t-test was used to compare normally distributed continuous variables between PTA and ASSR measures. PTA and ASSR threshold measures were also compared with an assessment of the correlation using Pearson's correlation analysis. Frequencies in which ASSR testing did not elicit a response were excluded from the final statistical analysis. A p-value of < 0.05 was considered statistically significant.
Results
A total of 50 (100 ears) community-dwelling older adults (14 male and 36 female) took part in this study (Table 1). Participants were aged between 61–84 years, average age for males was 72.9 ± 6 years and for females 71.6 ± 5.1 years (combined mean age 72.1 ± 5.4 years). On average, participant depression, anxiety, and stress scores were within normal levels (depression: 0–4, anxiety: 0–3, and stress: 0–7) according to the DASS 21 (47) severity scale: 2.2 ± 2.1, 2.2 ± 2.2 and 4.3 ± 2.9, respectively (Table 1). There was no significant correlation between psychological status (depression, anxiety, and stress) and participant age or gender (Pearson's correlation).
Behavioral PTA
Behavioral hearing thresholds using PTA were obtained for the whole sample. In this study, hearing range between 0–25 dB HL was considered normal hearing, 26–40 dB HL was considered mild HL, 41–55 dB HL was considered moderate HL, 56–70 dB HL was moderately severe HL, 71–90 was considered severe HL and 91 dB HL and above was considered profound HL. According to average 4-point PTA threshold measures, 78% (39/50) of participants had normal hearing thresholds (0–25 dB HL) and 22% (11/50) had mild hearing loss (26–40 dB HL). There was no significant correlation between PTA threshold measures and participants' depression, anxiety, or stress scores (Pearson's correlation). On average, males (24.6 dB ± 10.3, n = 14) had significantly higher hearing thresholds (p < 0.05) when compared to females (16.3 dB ± 7.2, n = 36), t(48) = 3.26; p = 0.002. There was a low, however significant, correlation between PTA thresholds and participant age, r(49) = 0.28; p < 0.05, showing increased thresholds with age.
ASSR
ASSR thresholds could not be measured in 34%, 10%, 1% and 27% of ears for 500, 1000, 2000, and 4000 Hz frequencies, respectively. These cases were excluded from further statistical analysis for the frequency in which no response was measured; hence the number of data points differs between frequencies. ASSR testing took on average 20 min to complete, with the shortest time recorded to achieve threshold measures at all tested carrier frequencies being 3.5 min and the longest time being 30.5 min. Threshold measures for all four carrier frequencies were obtained in 36% (18/50) of participants. Of those participants the average 4-point hearing thresholds indicate 72.2% (13/18) had normal hearing, 22.2% (4/18) had mild hearing loss and 5.5% (1/18) had moderate hearing loss. There was no significant correlation between ASSR thresholds and participants' gender, depression, anxiety, and stress scores (Pearson's correlation). There was a moderate, and significant, linear positive correlation between ASSR thresholds and participant age r(17) = 0.48; p < 0.05.
Comparison of ASSR and PTA in Older Adults
Table 2 and Figure 1 provide a summary of the mean thresholds for each carrier frequency for both PTA and ASSR. ASSR thresholds were significantly higher as compared to PTA thresholds based on the paired sample t-test analyses. The significant difference between the two procedures (i.e., PTA and ASSR) was seen at all frequencies, 500 Hz (7.5 dB ± 11.2, t(65) = 5.49; p < 0.001), 1000 Hz (6 dB ± 10.2, t(89) = 5.58; p < 0.001), 2000 Hz (5.7 dB ± 8.2, t(98) = 6.87; p < 0.001) and 4000 Hz (4.7 dB ± 9.2, t(70) = 4.28; p < 0.001), in order from highest to lowest threshold difference (Table 2). Mean PTA and ASSR hearing threshold values and differences were similar for each carrier frequency when analyzed for left and right ears separately, as noted in Table 2 and Figure 1.

Table 2. Mean (± SD) pure tone audiometry (PTA) and auditory steady-state response (ASSR) hearing threshold values (in decibels dBHL) in normal hearing older adults.
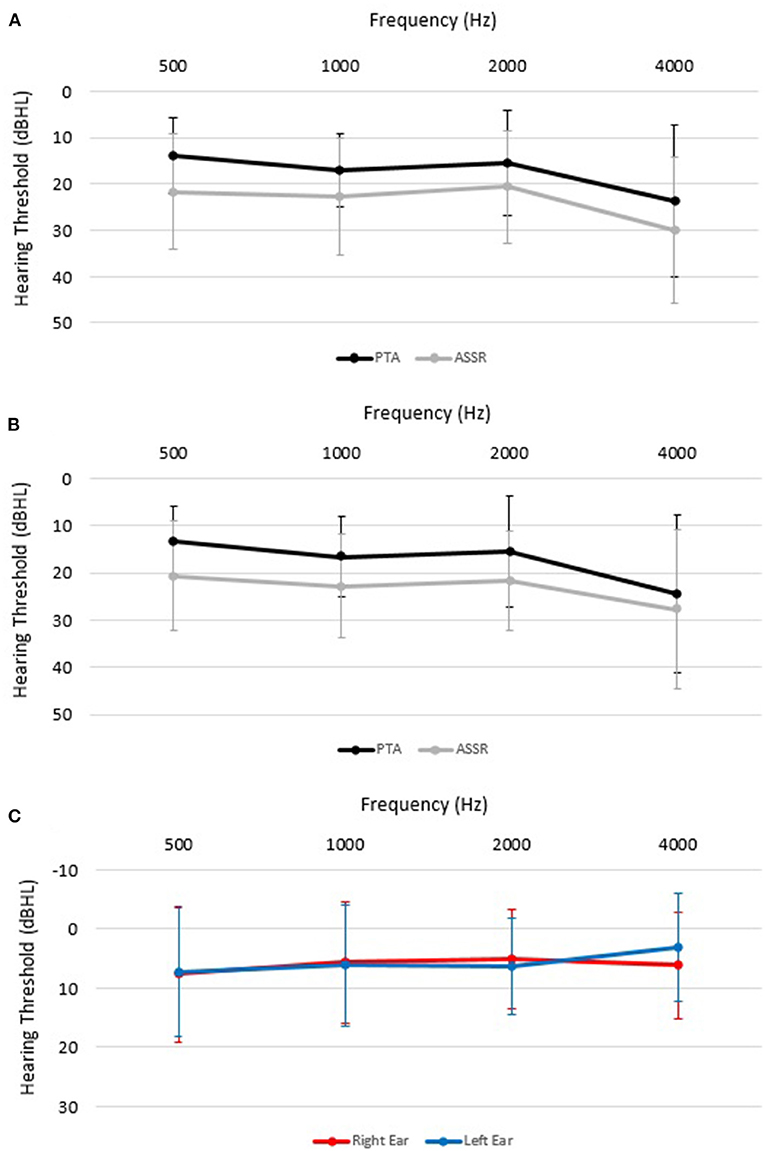
Figure 1. Mean (± SD) pure tone audiometry (PTA; black lines A, B) and auditory steady-state response (ASSR; gray lines A, B) hearing thresholds (in decibels dBHL) for each carrier frequency in the right ear (A), left ear (B). (C) shows threshold differences between PTA and ASSR in left and right ears.
The majority of all thresholds measured using ASSR were higher than thresholds measured using PTA for the same ear. Overall, 59% of ASSR thresholds overestimated (were higher than) PTA thresholds, 18% underestimated PTA thresholds and 23% were the same as the PTA thresholds. A similar trend can be seen when looking at each carrier frequency separately, with the majority of ASSR thresholds overestimating the PTA threshold (Figure 2). Over 80% of hearing thresholds measured using ASSR were within ± 15 dB from thresholds measured using PTA at 500 (80.3%), 1000 (85.5%), 2000 (90.9%) and 4000 (88.7%) Hz. In total, 63.6% of thresholds measured using ASSR were within ±10 dB from PTA thresholds at 500 Hz, 72.2% at 1000 Hz, 79.8% at 2000 Hz and 78.9% at 4000 Hz. Distribution of ASSR and PTA threshold differences (dB HL) for each carrier frequency are presented in Figure 3.
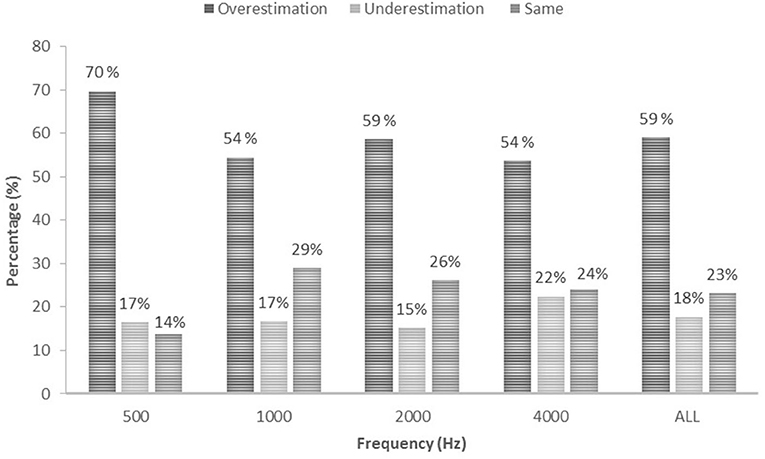
Figure 2. Accuracy of ASSR estimations in percentage (%) presented for each frequency and for all frequencies combined.
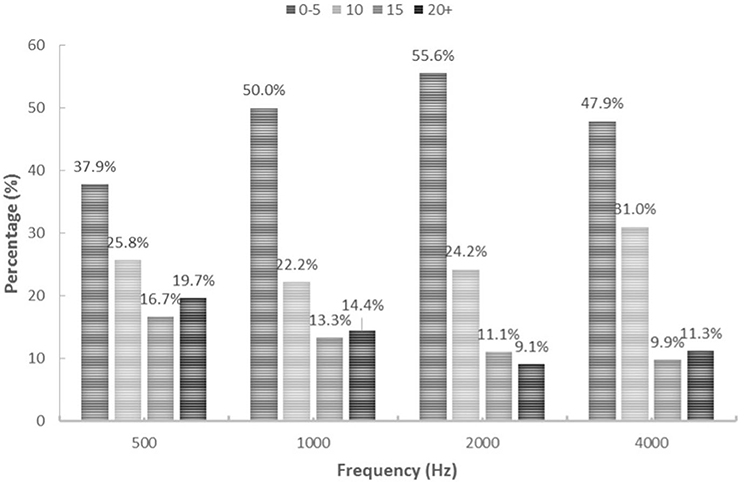
Figure 3. Distribution of ASSR and PTA differences for each frequency. Percentages presented for 0–5 dB, 10 dB, 15 dB and 20 dB+ (and over) threshold differences.
Correlation analysis, as presented in Figure 4, revealed strong significant (p < 0.001) linear correlations between hearing threshold measures from ASSR and PTA at 1000 Hz, 2000 Hz and 4000 Hz at r(89) = 0.53, r(98) = 0.74, and r(70) = 0.84, respectively. A moderate, yet significant, correlation between thresholds for ASSR and PTA was seen for the 500 Hz carrier frequency (r(65) = 0.42; p < 0.001). Similarly, correlation analysis of each ear separately resulted in strong correlations for threshold measures between ASSR and PTA at 1000 Hz (right ear only), 2000 Hz and 4000 Hz and moderate correlations at 500 Hz and 1000 Hz (left ear only). See Supplementary Figures S1, S2 and Supplementary Table S1.
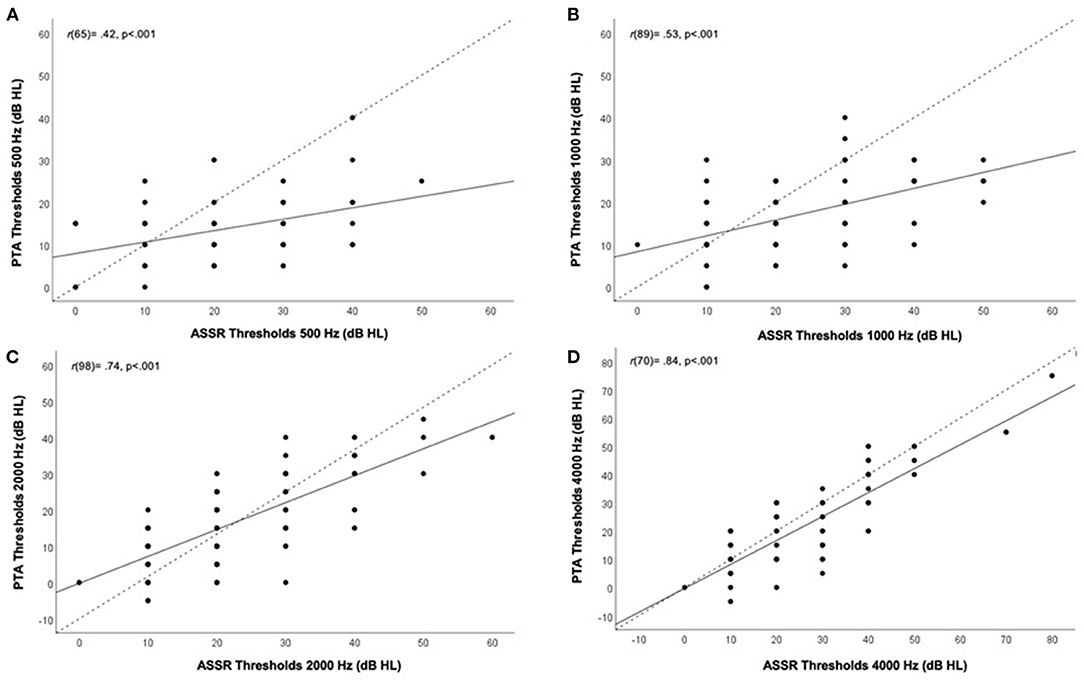
Figure 4. Correlation between hearing thresholds (both ears combined) obtained from auditory steady-state response (ASSR) (x-axis) and pure-tone audiometry (PTA) (y-axis) in dB HL according to carrier frequency. Different panels show different frequencies. (A) 500Hz, (B) 1000 Hz, (C) 2000 Hz, and (D) 4000 Hz. Correlation coefficients (r) and p-value are presented on the top left corner of each panel for all tested frequencies. Black line indicates line of best fit, dotted line indicates 1:1 ratio line.
Discussion
Hearing thresholds obtained from PTA and ASSR in the current study were found to be significantly correlated in a cohort consisting of elderly participants with normal hearing or mild hearing loss. This is in agreement with other studies that also reported a significant correlation between hearing thresholds obtained using PTA and ASSR (10, 37, 48). However, in the present study, there was a statistically significant increase in thresholds measured with ASSR as compared to PTA at all tested carrier frequencies. Mean threshold differences between PTA and ASSR were largest at 500 Hz with a difference of 7.5 dB HL and lowest at 4000 Hz with a difference of 4.7 dB HL. Nonetheless, we showed that the majority (varying between 64 and 79% dependent on carrier frequency) of threshold differences between ASSR and PTA were within 10 dB of each other and over 80% of ASSR thresholds were within 15 dB of PTA thresholds. Ten to fifteen dB differences in threshold measures are considered to be clinically acceptable and are tolerable when making hearing intervention plans (37, 49). Therefore, the results of this study indicate that threshold measures recorded using ASSR have the potential to provide useful objective estimations of hearing thresholds in “difficult to test” older adults for the timely detection and treatment of hearing loss. It should be noted that the present cohort of older adults presented with normal cognitive function and psychological status (i.e., depression, anxiety and stress), therefore, these factors would have no influence on test outcomes. Additionally, there was no statistical correlation between cognitive, psychological or gender status and hearing acuity.
In most cases, ASSR over estimated PTA thresholds. Over estimation of hearing thresholds could lead to an increased risk of false positives (identifying someone with HL even if hearing is normal) as well as over estimation of HL severity. Variations in the analysis algorithm and application of correction factors used to obtain ASSR and PTA thresholds could be contributing factors to the variation in threshold measures between the two techniques (49). In commercial acquisition systems, to counteract differences between ASSR and PTA, correction factors are applied based on the carrier frequency. Correction factors are set based on the difference between PTA and ASSR thresholds in subjects with varied hearing and age range (50). These correction factors can differ from one system manufacturer to another and are set as standard for most groups (i.e., adults, children, those with and without HL), which can result in variations in the threshold measures from one commercial system to another for the same subject (50). It may be that the correction factors applied to other age ranges may be suboptimal for older adults as used in the present study. Indeed, it has previously been suggested that ASSR can be a more useful technique in children, adults and older adults if the correction factors applied are defined specifically for each age group (50).
One factor that may influence the observed variations is the modulation rate used. In this study, for ASSR, default modulation rates were set for each carrier frequency according to manufacturer [Chartr EP (44)] recommendations. Modulation rates ranged from 80–98 Hz across the carrier frequencies. These modulation rates are considered fast modulations as they are over 50 Hz. Previous research that has informed acquisition system manufacturers, has been on participants in other age ranges [i.e., infants (35), adults under 35 (48), or a combination of children and adults (10, 11, 30, 36)] and this has yielded inconsistent ASSR protocols/recommendations. Previous research revealed that ASSRs are difficult to record in infants at low modulation frequencies (~40 Hz), therefore, high modulation frequencies have become a standard for ASSR testing regardless of the age of the subject (51). However, there is evidence to suggest that age-related changes in neural envelope processing may result in decreased ASSR responses for faster modulation rates in older adults compared to young adults or children (39, 40). Specifically, for high gamma frequencies (≥ 80 Hz) ASSRs decrease with age, which in turn suggests age-related decline in synchronized activity of high gamma oscillations (52–54). Therefore, this could contribute to the significant increase in thresholds seen when using ASSR compared to PTA in the present study.
Additionally, phase-locking of ASSR is suggested to be lower at high modulation rates in middle-aged and older adults in comparison to young adults (39). This has also been demonstrated by a number of animal studies, which show decline in phase-locking in fast modulations in both near- and far-field recordings with aging (55–57). The effect of aging on phase-locking is not reported for slow modulation frequencies (<50 Hz) (39). Reduced ASSR strength and lower phase-locking to fast modulation frequencies with aging is in line with reports of reduced temporal precision in encoding rapidly modulated stimuli as a result of loss of functional inhibition across the central auditory pathway with aging (38, 39, 41). Therefore, the use of high modulation rates for older adults may have resulted in no thresholds being established through ASSR in some cases and larger variations (less accuracy) in threshold differences between PTA and ASSR.
In this study ASSR thresholds were obtained for all tested carrier frequencies in only 36% of the participant sample (n = 50). Similarly, a study conducted on children (n = 20) found that ASSR thresholds were obtained for all frequencies tested (500, 1000, 2000, and 4000 Hz) in only 45% of the sample (35). Although, ASSR provides an objective measure of hearing thresholds without the need for active participation of the subject, it can be affected by patient movement, behavioral status (e.g., awake vs. asleep) and patient preparation (electrode impedance). Therefore, variations in these factors can result in inaccurate measures or no thresholds being established (11, 30). Additionally, a number of studies suggest that ASSR threshold measures at 500 Hz should be interpreted with caution (10, 30, 58). Similar to previous research, in the present study ASSR threshold measures at 500 Hz were the most variable among all carrier frequencies and also had the highest percentage of thresholds that were not established during the testing time limits (36, 59). This has been suggested to be due to the higher EEG noise and internal jittering as a result of neurologic asynchronicity (60). More research is required to establish strategies to overcome patient and equipment factors than may have negative impact on test results and accuracy.
Study Limitations
One experimental protocol limitation that could have contributed to larger variations in threshold differences between ASSR and PTA is the difference in step sizes used for establishing thresholds in the two tests. For PTA in this study threshold levels were determined by increasing increments of 10 dB followed by decreasing increments of 5 dB, and for ASSR, measurements were performed using a descending procedure, by reducing the intensity of the acoustic signal in 10 dB steps until the threshold. The differences in step size and protocols used in PTA and ASSR can easily result in at least 5 dB difference in thresholds between the two tests. Additionally, this study used an automated KUDUwave audiometer to establish PTA and compared them to ASSR. There is a ± 5–10 dB difference between KUDUwave and clinician obtained (in an audiological clinical setting) hearing thresholds in frequencies between 1000 and 4000 Hz and more than 10 dB difference for 500 Hz (47). Therefore future research should compare ASSR thresholds with KUDUwave PTA and clinician obtained PTA.
In this study, a maximum threshold search time of 7 min was set for ASSR due to testing time constraints. However, in some participants no thresholds were establish during this 7 min time frame and ASSR thresholds were obtained at all four carrier frequencies for only 36% of the participant sample. For ASSR, it is unknown how much search time should be allowed for thresholds to be established, highlighting another ASSR protocol element that requires refining and further research to improve methodological quality and clinical application.
Furthermore, this study only included participants with normal hearing or mild hearing loss, which does not provide full insight into the use of ASSR for testing hearing acuity in older adults. Previous reports indicate that ASSR thresholds are closer to PTA thresholds in participants with sensorineural hearing loss in comparison to normal hearing participants (30, 36, 61). It has been suggested that such smaller threshold differences between ASSR and PTA in HL participants could be due to abnormal increase in the response amplitude as a result of recruitment for damage to outer hair cells (36, 61). Therefore, generalizing ASSR findings to normal hearing and HL groups could result in incorrect threshold estimation. Due to the limited sample size in this study and difficulties obtaining ASSR thresholds in some participants, exploring the reliability of ASSR threshold measures in participants based on hearing thresholds (those with normal hearing and those with mild HL) was not suitable. Future research would benefit from investigating ASSR threshold measures in older adults with different degrees of HL.
Conclusion
The findings of the present study provide evidence to indicate that ASSR may be a valuable tool in estimating objective frequency-specific hearing thresholds in older adults. Though there is increased risk of false positive, due to over estimation of HL, ASSR is still reliable in assessing HL. Threshold differences between ASSR and the gold standard PTA were, for the majority of participants, within clinically acceptable ranges, thus ASSR can be useful in identifying HL in order to create hearing treatment plans for older adults who are unable to complete behavioral PTA. However, additional research is required to determine optimal parameters of ASSR for threshold estimation in older adults in order to increase its consistency and reliability, as well as eliminate some of the limitations associated with this technique. More research is also required to define modulation frequencies that are more suitable for older adults, which could provide valuable information to inform ASSR testing protocols for them as well as acquisition system manufacturers. Defining specific correction factors that take into account the patient's age, degree of HL and are specific for the carrier frequency can also help improve the methodological quality of ASSR.
Author Contributions
HT, DJ, WM, HS, and RM conceived the idea for this study. HT collected hearing data with the supervision of DJ. HT prepared the initial draft with input from DJ, WM, and HS. All authors contributed to the development of the idea of this manuscript, as well as contributed to the revision of the manuscript. All authors approved for the manuscript to be submitted.
Funding
This work was supported by the Australian Government Research Training Program Scholarship at The University of Western Australia; and Australian Alzheimer's Research Foundation.
Conflict of Interest
The authors declare that the research was conducted in the absence of any commercial or financial relationships that could be construed as a potential conflict of interest.
Publisher's Note
All claims expressed in this article are solely those of the authors and do not necessarily represent those of their affiliated organizations, or those of the publisher, the editors and the reviewers. Any product that may be evaluated in this article, or claim that may be made by its manufacturer, is not guaranteed or endorsed by the publisher.
Supplementary Material
The Supplementary Material for this article can be found online at: https://www.frontiersin.org/articles/10.3389/fneur.2022.924096/full#supplementary-material
References
1. Organization WH. Deafness and Hearing Loss. World Health Organization (2020). Available online at: https://www.who.int/news-room/fact-sheets/detail/deafness-and-hearing-loss
2. Manrique-Huarte R, Calavia D, Huarte Irujo A, Girón L, Manrique-Rodríguez M. Treatment for hearing loss among the elderly: auditory outcomes and impact on quality of life. Audiology & neurotology. (2016) 21:29–35. doi: 10.1159/000448352
3. Dalton DS, Cruickshanks KJ, Klein BEK, Klein R, Wiley TL, Nondahl DM. The impact of hearing loss on quality of life in older adults. Gerontologist. (2003) 43:661–8. doi: 10.1093/geront/43.5.661
4. Chia E-M, Wang JJ, Rochtchina E, Cumming RR, Newall P, Mitchell P. Hearing impairment and health-related quality of life: the blue mountains hearing study. Ear Hear. (2007) 28:187–95. doi: 10.1097/AUD.0b013e31803126b6
5. Shoham N, Lewis G, Favarato G, Cooper C. Prevalence of anxiety disorders and symptoms in people with hearing impairment: a systematic review. Soc Psychiatry Psychiatr Epidemiol. (2019) 54:649–60. doi: 10.1007/s00127-018-1638-3
6. Jayakody DMP, Almeida OP, Speelman CP, Bennett RJ, Moyle TC, Yiannos JM, et al. Association between speech and high-frequency hearing loss and depression, anxiety and stress in older adults. Maturitas. (2018) 110:86–91. doi: 10.1016/j.maturitas.2018.02.002
7. Mick PT, Lin FR. Social Isolation and Hearing Loss in the Elderly. Otolaryngol Head Neck Surg. (2013) 149(2_suppl):110. doi: 10.1177/0194599813495815a228
8. Mick P, Parfyonov M, Wittich W, Phillips N, Pichora-Fuller MK. Associations between sensory loss and social networks, participation, support, and loneliness: analysis of the Canadian longitudinal study on aging. Can Fam Physician. (2018) 64:e33–41. doi: 10.1093/geroni/igx004.2643
9. Hogan A, O'Loughlin K, Miller P, Kendig H. The health impact of a hearing disability on older people in Australia. J Aging Health. (2009) 21:1098–111. doi: 10.1177/0898264309347821
10. Wadhera R, Hernot S, Gulati SP, Kalra V. A Controlled comparison of auditory steady-state responses and pure-tone audiometry in patients with hearing loss. Ear Nose Throat J. (2017) 96:e47–52. doi: 10.1177/0145561317096010-1109
11. Swami H, Kumar S. Comparison of frequency-specific hearing thresholds between pure-tone audiometry and auditory steady-state response. Indian J otology. (2019) 25:59–65. doi: 10.4103/indianjotol.INDIANJOTOL_97_18
12. Bott A, Meyer C, Hickson L, Pachana NA. Can adults living with dementia complete pure-tone audiometry? a systematic review. Int J Audiology. (2019) 58:185–92. doi: 10.1080/14992027.2018.1550687
13. Sarant J, Harris D, Busby P, Maruff P, Schembri A, Lemke U, et al. The effect of hearing aid use on cognition in older adults: can we delay decline or even improve cognitive function? J Clin Med. (2020) 9:254. doi: 10.3390/jcm9010254
14. Maharani A, Dawes P, Nazroo J. Tampubolon G. Longitudinal relationship between hearing aid use and cognitive function in older Americans. J Am Geriatr Soc. (2018) 66:1130–6. doi: 10.1111/jgs.15363
15. Dawes P, Emsley R, Cruickshanks KJ, Moore DR, Fortnum H, Edmondson-Jones M, et al. Hearing loss and cognition: the role of hearing aids, social isolation and depression. PLoS ONE. (2015) 10:e0119616. doi: 10.1371/journal.pone.0119616
16. Deal JA, Sharrett AR, Albert MS, Coresh J, Mosley TH, Knopman D, et al. Hearing impairment and cognitive decline: a pilot study conducted within the atherosclerosis risk in communities neurocognitive study. Am J Epidemiol. (2015) 181:680–90. doi: 10.1093/aje/kwu333
17. Jewett DL, Williston JS. Auditory-Evoked far fields averaged from the scalp of humans. Brain (London, England: 1878). (1971) 94:681–96. doi: 10.1093/brain/94.4.681
18. Eggermont JJ. The inadequacy of click-evoked auditory brainstem responses in audiological applications. Ann N Y Acad Sci. (1982) 388:707–9. doi: 10.1111/j.1749-6632.1982.tb50839.x
19. Peterein JL, Neely JG. Auditory brainstem response testing in neurodiagnosis: structure versus function. J Am Acad Audiol. (2012) 23:269–75. doi: 10.3766/jaaa.23.4.5
20. Stapells DR, Oates P. Estimation of the pure-tone audiogram by the auditory brainstem response: a review. Audiol Neurootol. (1997) 2:257–80. doi: 10.1159/000259252
21. Canale A, Lacilla M, Cavalot AL, Albera R. Auditory steady-state responses and clinical applications. Eur Arch Otorhinolaryngol. (2006) 263:499–503. doi: 10.1007/s00405-006-0017-y
22. Gorga MP, Neely ST, Hoover BM, Dierking DM, Beauchaine KL, Manning C. Determining the upper limits of stimulation for auditory steady-state response measurements. Ear Hear. (2004) 25:302–7. doi: 10.1097/01.AUD.0000130801.96611.6B
23. Brookhouser PE, Gorga MP, Kelly WJ. Auditory brainstem response results as predictors of behavioral auditory thresholds in severe and profound hearing impairment. Laryngoscope. (1990) 100:803–10. doi: 10.1288/00005537-199008000-00002
24. Arlinger S. Audiologic diagnosis of infants. Semin Hear. (2000) 21:379–88. doi: 10.1055/s-2000-13469
25. Polonenko MJ, Maddox RK. The parallel auditory brainstem response. Trends Hear. (2019) 23:2331216519871395. doi: 10.1177/2331216519871395
26. Polonenko MJ, Maddox RK. Optimizing parameters for using the parallel auditory brainstem response to quickly estimate hearing thresholds. Ear Hear. (2022) 43:646–58. doi: 10.1097/AUD.0000000000001128
27. Dimitrijevic A, John MS, Van Roon P, Purcell DW, Adamonis J, Ostroff J, et al. Estimating the audiogram using multiple auditory steady-state responses. J Am Acad Audiol. (2002) 13:205–24. doi: 10.1055/s-0040-1715964
28. Picton TW, John MS, Dimitrijevic A, Purcell D. Human auditory steady-state responses. Int J Audiol. (2003) 42:177–219. doi: 10.3109/14992020309101316
29. Celesia GG. Brainstem auditory evoked responses. In: Disorders of Peripheral and Central Auditory Processing. Amsterdam: Elsevier Health Sciences (2013). p. 137–54.
30. Ahn JH, Lee H-S, Kim Y-J, Yoon TH, Chung JW. Comparing pure-tone audiometry and auditory steady state response for the measurement of hearing loss. Otolaryngol Head Neck Surg. (2007) 136:966–71. doi: 10.1016/j.otohns.2006.12.008
31. Rodriguez R, Picton T, Linden D, Hamel G, Laframboise G. Human auditory steady state responses: effects of intensity and frequency. Ear Hear. (1986) 7:300–13. doi: 10.1097/00003446-198610000-00003
32. Kuwada S, Anderson JS, Batra R, Fitzpatrick DC, Teissier N, D'Angelo WR. Sources of the scalp-recorded amplitude-modulation following response. J Am Acad Audiol. (2002) 13:188–204. doi: 10.1055/s-0040-1715963
33. Van Maanen A, Stapells DR. Comparison of multiple auditory steady-state responses (80 Versus 40 Hz) and slow cortical potentials for threshold estimation in hearing-impaired adults. Int J Audiol. (2005) 44:613–24. doi: 10.1080/14992020500258628
34. Valdes JL, Perez-Abalo MC, Martin V, Savio G, Sierra C, Rodriguez E, et al. Comparison of statistical indicators for the automatic detection of 80 hz auditory steady state responses. Ear Hear. (1997) 18:420–9. doi: 10.1097/00003446-199710000-00007
35. Kandogan T, Dalgic A. Reliability of auditory steady-state response (Assr): comparing thresholds of auditory steady-state response (Assr) with auditory brainstem response (abr) in children with severe hearing loss. Indian J Otolaryngol Head Neck Surg. (2012) 65:604–7. doi: 10.1007/s12070-012-0581-y
36. Ozdek A, Karacay M, Saylam G, Tatar E, Aygener N, Korkmaz MH. Comparison of pure tone audiometry and auditory steady-state responses in subjects with normal hearing and hearing loss. Eur Arch Otorhinolaryngol. (2010) 267:43–9. doi: 10.1007/s00405-009-1014-8
37. Komazec Z, Lemajić-Komazec S, Jović R, Nadj C, Jovancević L, Savović S. Comparison between auditory steady-state responses and pure-tone audiometry. Vojnosanit Pregl. (2010) 67:761–5. doi: 10.2298/vsp1009761k
38. Anderson S, Parbery-Clark A, White-Schwoch T, Kraus N. Aging affects neural precision of speech encoding. J Neurosci. (2012) 32:14156–64. doi: 10.1523/JNEUROSCI.2176-12.2012
39. Farahani ED, Wouters J, van Wieringen A. Neural generators underlying temporal envelope processing show altered responses and hemispheric asymmetry across age. Front Aging Neurosci. (2020) 12:596551. doi: 10.3389/fnagi.2020.596551
40. Tlumak AI, Durrant JD, Delgado RE. The effect of advancing age on auditory middle- and long-latency evoked potentials using a steady-state-response approach. Am J Audiol. (2015) 24:494–507. doi: 10.1044/2015_AJA-15-0036
41. Roque L, Karawani H, Gordon-Salant S, Anderson S. Effects of age, cognition, and neural encoding on the perception of temporal speech cues. Front Aging Neurosci. (2019) 13:749. doi: 10.3389/fnins.2019.00749
42. Sohrabi HR, Weinborn M, Laske C, Bates KA, Christensen D, Taddei K, et al. Subjective Memory complaints predict baseline but not future cognitive function over three years: results from the Western Australia Memory Study. Int Psychogeriatr. (2018) 31:513–25. doi: 10.1017/S1041610218001072
43. Hughson W, Westlake H. Manual for program outline for rehabilitation of aural casualties both military and civilian. Trans Am Acad Ophthalmol Otolaryngol. (1944) 48(Suppl):1–15.
45. Armstrong M, Moore RA. Anatomy, Patient Positioning. Statpearls. Treasure Island (FL): StatPearls Publishing LLC (2022).
46. Hidayat SH. Otometrics Ics Chartr Ep 200, Instrumen Abr/Bera/Assr. Hearing Vision. (2015). Available online at: https://madsen.hu/pdf/utmutato/Chartr_EP_Operation_Manual_7-60-0820-EN.pdf
47. Swanepoel de W, Biagio L. Validity of diagnostic computer-based air and forehead bone conduction audiometry. J Occup Environ Hyg. (2011) 8:210–4. doi: 10.1080/15459624.2011.559417
48. Beck RM, Ramos BF, Grasel SS, Ramos HF, de Moraes MFBB, de Almeida ER, et al. Comparative Study between pure tone audiometry and auditory steady-state responses in normal hearing subjects. Braz J Otorhinolaryngol. (2014) 80:35–40. doi: 10.5935/1808-8694.20140009
49. Hatzopoulos S, Petruccelli J, Sliwa L, Jedrzejczak WW, Kochanek K, Skarzyński H. Hearing threshold prediction with auditory steady state responses and estimation of correction functions to compensate for differences with behavioral data, in adult subjects. Part 1: Audera and Chartr Ep Devices. Med Sci Monit. (2012) 18:Mt47–53. doi: 10.12659/msm.883195
50. Ghasemahmad Z, Farahani S. Validity of correction factors applied to auditory steady-state responses (Assrs) in normal hearing adults in Chartr Ep system. Eur Arch Otorhinolaryngol. (2019) 276:2171–80. doi: 10.1007/s00405-019-05451-3
51. Stapells DR, Galambos R, Costello JA, Makeig S. Inconsistency of auditory middle latency and steady-state responses in infants. Electroencephalogr Clin Neurophysiol. (1988) 71:289–95. doi: 10.1016/0168-5597(88)90029-9
52. Dimitrijevic A, John MS, Picton TW. Auditory steady-state responses and word recognition scores in normal-hearing and hearing-impaired adults. Ear Hear. (2004) 25:68–84. doi: 10.1097/01.AUD.0000111545.71693.48
53. Parthasarathy A, Cunningham PA, Bartlett EL. Age-Related differences in auditory processing as assessed by amplitude-modulation following responses in quiet and in noise. Front Aging Neurosci. (2010) 2:152. doi: 10.3389/fnagi.2010.00152
54. Grose JH, Mamo SK, Hall JW. Age effects in temporal envelope processing: speech unmasking and auditory steady state responses. Ear Hear. (2009) 30:568–75. doi: 10.1097/AUD.0b013e3181ac128f
55. Parthasarathy A, Bartlett E. Two-Channel recording of auditory-evoked potentials to detect age-related deficits in temporal processing. Hear Res. (2012) 289:52–62. doi: 10.1016/j.heares.2012.04.014
56. Herrmann B, Parthasarathy A, Bartlett EL, Foxe J. Ageing affects dual encoding of periodicity and envelope shape in rat inferior colliculus neurons. Eur J Neurosci. (2017) 45:299–311. doi: 10.1111/ejn.13463
57. Parthasarathy A, Herrmann B, Bartlett EL. Aging alters envelope representations of speech-like sounds in the inferior colliculus. Neurobiol Aging. (2019) 73:30–40. doi: 10.1016/j.neurobiolaging.2018.08.023
58. D'Haenens W, Dhooge I, Vel ED, Maes L, Bockstael A, Vinck BM. Auditory steady-state responses to mm and exponential envelope am2/fm stimuli in normal-hearing adults. Int J Audiol. (2007) 46:399–406. doi: 10.1080/14992020701347329
59. Casey K-A, Small SA. Comparisons of auditory steady state response and behavioral air conduction and bone conduction thresholds for infants and adults with normal hearing. Ear Hear. (2014) 35:423–39. doi: 10.1097/AUD.0000000000000021
60. Lins OG, Picton TW. Auditory Steady-State Responses to Multiple Simultaneous Stimuli. Electroencephalogr Clin Neurophysiol. (1995) 96:420–32. doi: 10.1016/0168-5597(95)00048-W
61. Perez-Abalo MC, Savio G, Torres A, Martín V, Rodríguez E, Galán L. Steady state responses to multiple amplitude-modulated tones: an optimized method to test frequency-specific thresholds in hearing-impaired children and normal-hearing subjects. Ear Hear. (2001) 22:200–11. doi: 10.1097/00003446-200106000-00004
Keywords: auditory steady-state response (ASSR), pure-tone audiometry (PTA), hearing, older adult, objective audiometry
Citation: Tarawneh HY, Sohrabi HR, Mulders WHAM, Martins RN and Jayakody DMP (2022) Comparison of Auditory Steady-State Responses With Conventional Audiometry in Older Adults. Front. Neurol. 13:924096. doi: 10.3389/fneur.2022.924096
Received: 20 April 2022; Accepted: 08 June 2022;
Published: 04 July 2022.
Edited by:
Helen Henshaw, University of Nottingham, United KingdomReviewed by:
Anthea Bott, GN Hearing A/S, DenmarkRebecca Millman, The University of Manchester, United Kingdom
Copyright © 2022 Tarawneh, Sohrabi, Mulders, Martins and Jayakody. This is an open-access article distributed under the terms of the Creative Commons Attribution License (CC BY). The use, distribution or reproduction in other forums is permitted, provided the original author(s) and the copyright owner(s) are credited and that the original publication in this journal is cited, in accordance with accepted academic practice. No use, distribution or reproduction is permitted which does not comply with these terms.
*Correspondence: Hadeel Y. Tarawneh, hadeel.tarawneh@research.uwa.edu.au