- 1Department of Neurology, State University of New York Downstate Health Sciences University, New York, NY, United States
- 2Department of Medicine, Vagelos College of Physicians and Surgeons, Columbia University Irving Medical Center, New York, NY, United States
- 3Department of Neurology and Rehabilitation and Regenerative Medicine, Columbia University, New York, NY, United States
- 4Division of Stroke and Cerebrovascular Diseases, Department of Neurology, Columbia University, New York, NY, United States
Post-stroke cognitive impairment affects more than one-third of patients after an ischemic stroke (IS). Identifying markers of potential cognitive recovery after ischemic stroke can guide patients' selection for treatments, enrollment in clinical trials, and cognitive rehabilitation methods to restore cognitive abilities in post-stroke patients. Despite the burden of post-stroke cognitive impairment, biomarkers of cognitive recovery are an understudied area of research. This narrative review summarizes and critically reviews the current literature on the use and utility of neuroimaging as a predictive biomarker of cognitive recovery after IS. Most studies included in this review utilized structural Magnetic Resonance Imaging (MRI) to predict cognitive recovery after IS; these studies highlighted baseline markers of cerebral small vessel disease and cortical atrophy as predictors of cognitive recovery. Functional Magnetic Resonance Imaging (fMRI) using resting-state functional connectivity and Diffusion Imaging are potential biomarkers of cognitive recovery after IS, although more precise predictive tools are needed. Comparison of these studies is limited by heterogeneity in cognitive assessments. For all modalities, current findings need replication in larger samples. Although no neuroimaging tool is ready for use as a biomarker at this stage, these studies suggest a clinically meaningful role for neuroimaging in predicting post-stroke cognitive recovery.
Introduction
The American Heart Association (AHA) and the American Stroke Association (ASA) define ischemic stroke (IS) as an episode of neurological dysfunction caused by focal cerebral, spinal, or retinal infarction (1). IS represents 80% of all stroke types (2) and is a major cause of disability (3). With increasing survival after stroke and population aging, the prevalence of stroke is projected to increase by 3.4 million in 2030 (4, 5). Many patients who survive a stroke live with a significant long-term disability that affects multiple functions, including motor, sensory, language, and cognitive abilities. An increasing number of studies have attempted to determine potential factors that can influence recovery after stroke (6). In a pivotal report from the Stroke Rehabilitation Roundtable, the importance of using a biomarker approach to identify the potential for recovery after stroke was outlined (6). The consensus introduced the term Stroke Recovery Biomarker (SRB), defined as “indicators of disease state that can be used clinically as a measure reflecting underlying processes that may be difficult to measure directly in humans and could be used to predict recovery or treatment response” (6, 7). The report referred to recovery for several stroke-type deficits, including motor, sensory, language, and cognition. The SRB approach aims to guide patients' treatment selection, enrollment in clinical trials, and rehabilitation interventions (6, 7).
IS recovery studies have focused mostly on motor recovery (8–20). Cognition is another important domain frequently affected by IS, resulting in post-stroke cognitive impairment (PSCI). A recent systematic review and meta-analysis identified a pooled prevalence of PSCI of 39%, measured within the first year post-stroke (21). Others report a PSCI prevalence ranging from 20 to 80% depending on factors such as race and methodology (22). Cognitive recovery remains an understudied aspect of stroke, and no biomarkers are currently ready for use in clinical trials (6, 23). Some studies reported spontaneous restoration of cognitive function after the subacute phase of IS (24–26). However, many patients have cognitive impairment beyond the subacute phase of IS. A recent, large, population-based study of first-ever stroke patients from the South London Stroke Register between 1995 and 2018 (n = 6,504, mean age = 73 years) found that one-third of patients cognitively improved during the first 3 months post-stroke, one-third deteriorated, and the rest remained cognitively unchanged (27). The study further reported that PSCI was associated with a 5-year increase in the risk of mortality (RR = 30%), dependency (RR = 90%), depression (RR = 60%) and institutionalization (RR = 50%) (27).
Imaging is a potential biomarker for cognitive recovery after IS (6). A systematic review evaluating all biological and imaging markers found that global atrophy and medial temporal lobe atrophy were the most consistent predictors of cognitive impairment after stroke; however, this review did not link cognitive recovery over time with neuroimaging (28). Given the accessibility and the wide use of neuroimaging as part of stroke workup, neuroimaging is a promising tool to study the potential for cognitive recovery after stroke. Neuroimaging techniques are currently being used to understand higher cortical function and recovery among comatose patients with the eventual goal to identify potential early and tailored rehabilitative interventions and underlying patient-specific characteristics that are most responsive to these interventions (29, 30). Cognitive aging is another area where neuroimaging is increasingly used to comprehend brain cognitive processes (31). Thus, our goal for this review was to summarize literature within the last 10 years describing neuroimaging as a predictive marker of cognitive recovery in IS. We focus on IS, the most common type of stroke associated with PSCI. We highlight important findings and limitations in the studies and discuss some of the challenges for future studies to consider.
Search methodology and literature selection
PubMed was used as the primary database for studies published in the last 10 years through September 6th, 2022. We used the Medical Subject Headings (MeSH) term “stroke” with the MeSH subheadings “complications” or “psychology” or the term “ischemic stroke” paired with both of the following terms in the abstract/title of each article or as MeSH terms:
• A cognition term (“cognition,” “cognitive,” “cognitive decline,” “cognition disorder,” or “dementia,” “neuropsychological,” or “neuropsychological tests”).
• A neuroimaging term (“neuroimaging,” “magnetic resonance imaging/MRI,” “functional magnetic resonance imaging/fMRI,” “diffusion tensor imaging/DTI,” “default mode network,” or “connectivity”).
Additional studies obtained through review of relevant article citations were included.
Studies examining other types of strokes—hemorrhagic stroke (HS), traumatic stroke, subarachnoid hemorrhage (SH), and transient ischemic attacks (TIA)—were excluded. HS were excluded due to their different recovery trajectories compared to IS (32, 33). Likewise, TIA were also excluded due to a lack of clear and persistent ischemic injury, which may result in a different recovery course (34, 35). Since cognitive recovery implies a change in cognitive performance over time, studies that reported only one cognitive assessment were excluded. Included studies associated a change in a cognitive assessment measure between at least two time points with baseline neuroimaging.
Finally, we required baseline imaging and cognitive assessments to be completed within 6 weeks of stroke. This is to ensure clinical relevance, as most imaging used to predict recovery would be completed during a hospital admission. A baseline cognitive assessment more than 6 weeks after an initial ischemic stroke may represent a different stage of stroke recovery and therefore not comparable to the other articles in this review.
Studies of potential relevance were selected, and 35 were excluded after careful full-text review based on the criteria detailed above (Figure 1, Supplementary Table 1). A total of 13 studies were included.
Neuroimaging modalities used as biomarkers for cognitive recovery after ischemic stroke
MRI: T1-weighted MRI, T2-weighted, and fluid-attenuated inversion recovery (FLAIR)
MRI as a means of characterizing or predicting cognitive impairment through structural biomarkers is well-documented (22, 36). These biomarkers include cerebral small vessel disease (SVD) such as white matter hyperintensities (WMH) and microinfarcts, cortical volume, and size and location of IS lesions (36). Researchers have attempted to use these same biomarkers to predict cognitive recovery following IS (37–39). Studies utilizing structural MRI that fit the criteria for this review are summarized in Table 1.
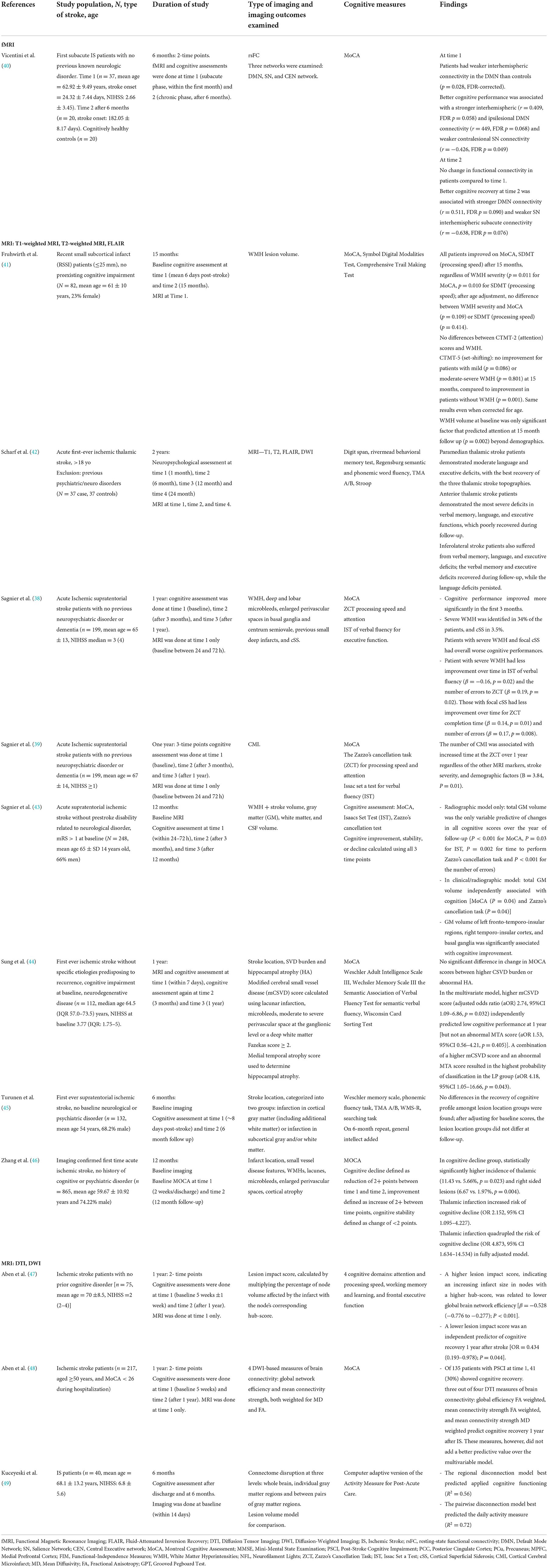
Table 1. Summary of key studies within the last 10 years on neuroimaging biomarkers of cognitive recovery after ischemic stroke.
Markers of cerebral small vessel disease
An important study by Sagnier et al. reported that first-time IS patients with pre-existing severe WMH on MRI had less improvement in verbal fluency tests at 3 months to 1 year after IS (38). Similarly, the presence of Cortical Superficial Siderosis (cSS) after first-time IS, another radiologic biomarker of cerebral SVD, was an indicator of worse cognitive recovery in tests of processing speed and attention, independent of IS volume/location, gray matter volume, other SVD biomarkers, and clinical severity, cardiovascular risk factors, and demographic confounders (38).
Similarly, Fruthwirth et al. found that deep WMH volume at baseline predicted recovery of set-shifting at 15 month follow up among patients with recent small subcortical infarcts: participants with no or mild deep WMH improved in set-shifting, while those with moderate to severe WMH showed no improvement (41). A similar pattern was seen for periventricular WMH (pWMH) at baseline and recovery of attention, although this interaction was no longer significant after controlling for age. For set-shifting, mild pWMH predicted improvement, whereas no pWMH and moderate-severe pWMH demonstrated no improvement. Of note, this study found that all patients, regardless of baseline WMH volume, improved in Montreal Cognitive Assessment (MoCA) scores, processing speed, and attention.
Another study examining cerebral small vessel disease (mCSVD) score—determined by MRI evaluation—and medial temporal atrophy (MTA) score—a measure of hippocampal atrophy—found that neither of these imaging markers predicted change in MoCA scores between baseline and 1 year follow up (44). Despite these imaging markers not being associated with a change in cognitive scores, this study did find that mCSVD and MTA scores could be used to predict low vs. high cognitive performance at 1 year, with higher mCSVD and MTA scores associated with increased likelihood of low MoCA scores at follow up.
Cortical volume
Sagnier found that total gray matter (GM) volume was the only radiographic factor predictive of cognitive improvement at 12 month follow up among IS patients. Specifically, GM volume of left fronto-temporo-insular regions, right temporo-insular cortex, and basal ganglia were significantly associated with cognitive improvement (43).
Infarct characteristic
Several studies examined the impact of infarct location on cognitive recovery using structural MRI. Turunen examined differences in cognitive recovery for cortical vs. subcortical lesions among patients with first-ever supratentorial ischemic stroke (45). While this study found that subcortical infarctions were associated with decreased verbal memory and psychomotor speed in the acute phase and persistent verbal memory differences at 6-month follow-up, there was no difference in recovery of cognition between the two lesion location groups. In contrast, a recent study by Zhang et al. found a significantly higher incidence of thalamic and right-sided lesions in the group that cognitively declined at follow up, determined by a difference in MoCA scores between baseline and 12-month exam (46). In their fully adjusted models, infarct in the thalamus more than quadrupled the risk of cognitive decline among these patients. A recent study further characterized cognitive recovery among patients with ischemic thalamic stroke: in this case control study with a 2-year follow-up period, patients with anterior and inferolateral thalamic strokes were found to have poorer recovery of language, memory, and executive function than those with paramedian strokes (42).
Together, these studies support the use of structural MRI sequences to predict changes in cognition after ischemic stroke. Baseline measures of cerebral SVD, including WMH, as well as baseline cortical volume may be important indicators of the potential for restoration of cognitive ability after stroke. In addition, these articles suggest that different infarct locations as seen on structural MRI may be used to predict differing cognitive recovery trajectories after stroke, although more studies are needed to fully elucidate this relationship.
Functional magnetic resonance imaging (fMRI)
Only one study reported post-IS cognitive recovery using resting-state fMRI (rs-fMRI) (Table 1) (40). Rs-fMRI utilizes Blood Oxygen Level Dependent (BOLD) to study spontaneous brain neural activity at rest in a specific functional brain region (rsfMRI activity) (50). In their study examining cognitive recovery after IS, Vincentini et al. found weaker interhemispheric spontaneous temporal correlations between different brain functional regions [rs-functional connectivity (rsFC)] within the Default Mode Network (DMN). Alterations of rsFc among IS patients have also been previously reported (51–53). Stroke injury has been shown to disrupt communications between hemispheres and results in both intra-and interhemispheric changes in rsFC (52). Vicentini et al. reported no change in rsFC from the subacute to the chronic phase in IS patients (40). This study did find, however, that better cognitive recovery at 6 months was correlated with rsFC in two networks: DMN and Executive Network (40).
This study argues in favor of the potential of rsFC to predict the course of cognitive changes post-IS and the involvement of DMN in the recovery process, although more studies are required to confirm these findings and further understand brain functional networks involved in the acute to chronic phases of cognitive recovery (20). Furthermore, there are still practical challenges to using rsFMRI in clinical settings, such as availability, time, and the need for interpretation expertise. The lack of technique standardization, including variability in rsfMRI data acquisition, preprocessing, and analytical methods, presents another challenge for clinical applicability (50, 54).
Diffusion tensor imaging (DTI) and diffusion-weighted imaging (DWI)
While fMRI examines the brain's functional integrity, DTI looks at the brain's white matter structural integrity. Fractional anisotropy (FA) is the most common parameter derived from DTI to assess brain structural connectivity. Other DTI measures are mean diffusivity (MD), radial diffusivity (RD), axial diffusivity (AD), independent of direction, and relative anisotropy.
Important studies using DTI/DWI to study cognitive recovery after IS are summarized in Table 1. A notable study by Aben et al. (47) used diffusion-weighted data after IS to create a lesion impact score that reflected the impact of IS size on the brain's network hubs. The authors demonstrated that a lower lesion impact score was an independent predictor of cognitive recovery 1 year after IS while controlling for WMH and infarct volumes (47). The authors showed that this score could also be calculated using structural MRI sequences (T1, FLAIR), which are routinely ordered as part of a stroke workup and therefore easier to implement in a clinical setting to predict long-term recovery after IS (47). A subsequent study compared DTI measures with a multivariable model, including age, education, and infarct size, and found that three out of four DTI measures of brain connectivity (global efficiency FA weighted, mean connectivity strength FA weighted, and mean connectivity strength MD weighted) predicted cognitive recovery 1 year after IS. These measures, however, did not improve prediction over the multivariable model that included education level and infarct size as significant predictors of cognitive recovery (48).
In a different study using DWI, Kuceyeski et al. (49) compared models of connectome disruption to determine which model best predicted recovery after IS. The authors found that the regional disconnection model, which reflects changes in structural connectivity of gray matter regions (WM tracts connecting brain regions) to the rest of the network, best predicted cognitive recovery. This regional disconnection model was found to be superior to models based on lesion volume and other disconnection models (whole brain and pairwise) (49).
The evidence for DTI and DWI as a means of predicting cognitive recovery after stroke is promising. More research is needed to determine the additional utility of this modality over structural MRI and to improve predictive value.
Study limitations and future directions
To the best of our knowledge, this review is among the first to focus on neuroimaging biomarkers of cognitive recovery among IS patients. This review is limited by inherent challenges in using keywords to search literature, including a lack of consistent use of terminology to characterize study subject matter. We carefully reviewed the literature to ensure our search was as robust as possible but may have inadvertently missed relevant studies. One key aspect of our study that necessitated the exclusion of multiple otherwise relevant articles was that we focused specifically on the association of neuroimaging with cognitive recovery after stroke; each included study reported on the association between baseline neuroimaging and a change in a cognitive measure over time. Articles that merely reported a cognitive outcome (e.g., post-stroke cognitive impairment vs. no post-stroke cognitive impairment) were therefore excluded from this review of neuroimaging biomarkers for cognitive recovery after IS.
In addition, there are several gaps in the current literature, as discussed below:
1. The best time to study cognitive recovery remains unclear and likely stems from uncertainty in the time frame of expected cognitive recovery post-stroke. In our review, we focused on baseline cognitive assessments done within 6 weeks of stroke to ensure clinical relevance and to adequately compare different articles. However, cognitive recovery studies among chronic stroke patients may help answer this question.
2. The ability to detect cognitive recovery depends largely on the sensitivity of the cognitive test used. The studies included in this review used a variety of different tests—all outlined in Table 1—which may limit our ability to compare them and to generalize their results. Screening tests such as the Montreal Cognitive Assessment (MoCA) and Mini-Mental State Exam (MMSE) are gross measures of cognition and may not capture subtle cognitive dysfunction nor subtypes of cognitive impairment (e.g., left neglect, aphasia). MoCA, for instance, is less sensitive to right-hemispheric lesion-based deficits (55). Adequate studies of cognitive recovery may require more detailed assessments tailored to the setting (e.g., brief baseline exams for inpatients, longer baseline exams for outpatients).
3. To be included in this review, we required at least one repeat measure of cognition. However, it should be noted that having longitudinal imaging is also important to show concordance between neuroimaging and cognitive testing parameters across the recovery course.
4. Some studies—which were excluded from this review—utilized cohorts of both ischemic (IS) and hemorrhagic strokes (HS) to study cognitive recovery. IS and IH have different pathophysiologies and different recovery processes (32, 33). Although the differences in recovery between the two-stroke types are not entirely known (56), future post-stroke cognitive recovery studies should analyze stroke types separately.
5. Only some of the reviewed studies explicitly assessed pre-stroke cognitive status. Pre-stroke cognitive status may influence the recovery process and should be taken into consideration (57). Similarly, not all studies mentioned if only first-time IS patients were included (49). In the future, it would be helpful to standardize screening of pre-stroke cognitive status to better allow comparison between studies of cognitive recovery.
6. Finally, many studies in this review combined neuroimaging with non-neuroimaging tools to improve the prediction of cognitive recovery after IS. For example, Sangier created models that incorporated demographic and clinical factors, including age, sex, education, cardiovascular risk factors, and modified Rankin score (38, 43). Such models are currently used to predict cognitive function after stroke, suggesting their utility in predicting cognitive recovery post-stroke; the SIGNAL2 score and the CHANGE score, both examining the risk of post-stroke cognitive impairment, incorporate age and education into their prediction tools, in addition to imaging variables (58, 59).
Conclusion
In summary, the current literature on cognitive recovery using neuroimaging as a predictive marker, although small, is promising. No imaging tool is ready for use as an established biomarker yet. Future studies should replicate current findings in larger samples using a consistent methodology.
Author contributions
MT and VK conducted the literature search and drafted the manuscript in equal contribution. MP reviewed the manuscript for intellectual content. IN conceptualized the project and reviewed the manuscript for intellectual content.
Funding
IN was currently supported by National Center for Advancing Translational Sciences, National Institutes of Health, through Grant Number KL2TR001874 and has received institutional support from Columbia University of Vagelos College of Physicians, Doris Duke Charitable Foundation COVID-19 Fund to Retain Clinical Scientists, and the American Heart Association.
Conflict of interest
The authors declare that the research was conducted in the absence of any commercial or financial relationships that could be construed as a potential conflict of interest.
Publisher's note
All claims expressed in this article are solely those of the authors and do not necessarily represent those of their affiliated organizations, or those of the publisher, the editors and the reviewers. Any product that may be evaluated in this article, or claim that may be made by its manufacturer, is not guaranteed or endorsed by the publisher.
Supplementary material
The Supplementary Material for this article can be found online at: https://www.frontiersin.org/articles/10.3389/fneur.2022.923942/full#supplementary-material
References
1. Sacco RL, Kasner SE, Broderick JP, Caplan LR, Connors JJ, Culebras A, et al. An updated definition of stroke for the 21st century: a statement for healthcare professionals from the American Heart Association/American Stroke Association. Stroke. (2013) 44:2064–89. doi: 10.1161/STR.0b013e318296aeca
2. Mehndiratta P, Chapman Smith S, Worrall BB. Etiologic stroke subtypes: updated definition and efficient workup strategies. Curr Treat Options Cardiovasc Med. (2015) 17:357. doi: 10.1007/s11936-014-0357-7
3. Virani SS, Alonso A, Aparicio HJ, Benjamin EJ, Bittencourt MS, Callaway CW, et al. Stroke statistics, heart disease and stroke statistics-2021 update: a report from the American Heart Association. Circulation. (2021) 143:e254–743. doi: 10.1161/CIR.0000000000000950
4. Ovbiagele B, Goldstein LB, Higashida RT, Howard VJ, Johnston SC, Khavjou OA, et al. Forecasting the future of stroke in the United States: a policy statement from the American Heart Association and American Stroke Association. Stroke. (2013) 44:2361–75. doi: 10.1161/STR.0b013e31829734f2
5. Benjamin EJ, Muntner P, Alonso A, Bittencourt MS, Callaway CW, Carson AP, et al. Heart disease and stroke statistics-2019 update: a report from the American Heart Association. Circulation. (2019) 139:e56–528. doi: 10.1161/CIR.0000000000000659
6. Boyd LA, Hayward KS, Ward NS, Stinear CM, Rosso C, Fisher RJ, et al. Biomarkers of stroke recovery: consensus-based core recommendations from the stroke recovery and rehabilitation roundtable. Neurorehabil Neural Repair. (2017) 31:864–76. doi: 10.1177/1545968317732680
7. Bernhardt J, Borschmann K, Boyd L, Carmichael ST, Corbett D, Cramer SC, et al. Moving rehabilitation research forward: developing consensus statements for rehabilitation and recovery research. Neurorehabil Neural Repair. (2017) 31:694–8. doi: 10.1177/1545968317724290
8. Hartwigsen G, Saur D. Neuroimaging of stroke recovery from aphasia - insights into plasticity of the human language network. Neuroimage. (2019) 190:14–31. doi: 10.1016/j.neuroimage.2017.11.056
9. Sinke MRT, van Tilborg GAF, Meerwaldt AE, van Heijningen CL, van der Toorn A, Straathof M, et al. Remote corticospinal tract degeneration after cortical stroke in rats may not preclude spontaneous sensorimotor recovery. Neurorehabil Neural Repair. (2021) 35:1010–19. doi: 10.1177/15459683211041318
10. Mattia D, Pichiorri F, Colamarino E, Masciullo M, Morone G, Toppi J, et al. The Promotoer, a brain-computer interface-assisted intervention to promote upper limb functional motor recovery after stroke: a study protocol for a randomized controlled trial to test early and long-term efficacy and to identify determinants of response. BMC Neurol. (2020) 20:254. doi: 10.1186/s12883-020-01826-w
11. Liu G, Tan S, Peng K, Dang C, Xing S, Xie C, et al. Network change in the ipsilesional cerebellum is correlated with motor recovery following unilateral pontine infarction. Eur J Neurol. (2019) 26:1266–73. doi: 10.1111/ene.13974
12. Wei W, Bai L, Wang J, Dai R, Tong RK, Zhang Y, et al. A longitudinal study of hand motor recovery after sub-acute stroke: a study combined FMRI with diffusion tensor imaging. PLoS ONE. (2013) 8:e64154. doi: 10.1371/journal.pone.0064154
13. Dang C, Liu G, Xing S, Xie C, Peng K, Li C, et al. Longitudinal cortical volume changes correlate with motor recovery in patients after acute local subcortical infarction. Stroke. (2013) 44:2795–801. doi: 10.1161/STROKEAHA.113.000971
14. Zarahn E, Alon L, Ryan SL, Lazar RM, Vry MS, Weiller C, et al. Prediction of motor recovery using initial impairment and fMRI 48 h poststroke. Cereb Cortex. (2011) 21:2712–21. doi: 10.1093/cercor/bhr047
15. Iorga M, Higgins J, Caplan D, Zinbarg R, Kiran S, Thompson CK, et al. Predicting language recovery in post-stroke aphasia using behavior and functional MRI. Sci Rep. (2021) 11:8419. doi: 10.1038/s41598-021-88022-z
16. Sebastian R, Long C, Purcell JJ, Faria AV, Lindquist M, Jarso S, et al. Imaging network level language recovery after left PCA stroke. Restor Neurol Neurosci. (2016) 34:473–89. doi: 10.3233/RNN-150621
17. Hartwigsen G. Adaptive plasticity in the healthy language network: implications for language recovery after stroke. Neural Plast. (2016) 2016:9674790. doi: 10.1155/2016/9674790
18. Jarso S, Li M, Faria A, Davis C, Leigh R, Sebastian R, et al. Distinct mechanisms and timing of language recovery after stroke. Cogn Neuropsychol. (2013) 30:454–75. doi: 10.1080/02643294.2013.875467
19. Lidzba K, Staudt M, Zieske F, Schwilling E, Ackermann H. Prestroke/poststroke fMRI in aphasia: perilesional hemodynamic activation and language recovery. Neurology. (2012) 78:289–91. doi: 10.1212/WNL.0b013e318243679a
20. Baldassarre A, Ramsey LE, Siegel JS, Shulman GL, Corbetta M. Brain connectivity and neurological disorders after stroke. Curr Opin Neurol. (2016) 29:706–13. doi: 10.1097/WCO.0000000000000396
21. Sexton EA-O, McLoughlin A, Williams DJ, Merriman NA, Donnelly N, Rohde DA-O, et al. Systematic review and meta-analysis of the prevalence of cognitive impairment no dementia in the first year post-stroke. Eur Stroke J. (2019) 4:160–71. doi: 10.1177/2396987318825484
22. Sun JH, Tan L, Yu JT. Post-stroke cognitive impairment: epidemiology, mechanisms and management. Ann Transl Med. (2014) 2:80. doi: 10.3978/j.issn.2305-5839.2014.08.05
23. Melkas S, Jokinen H, Hietanen M, Erkinjuntti T. Poststroke cognitive impairment and dementia: prevalence, diagnosis, and treatment. Degener Neurol Neuromuscul Dis. (2014) 4:21–7. doi: 10.2147/DNND.S37353
24. Ballard C, Rowan E, Stephens S, Kalaria R, Kenny RA. Prospective follow-up study between 3 and 15 months after stroke: improvements and decline in cognitive function among dementia-free stroke survivors >75 years of age. Stroke. (2003) 34:2440–4. doi: 10.1161/01.STR.0000089923.29724.CE
25. Liman TG, Heuschmann PU, Endres M, Flöel A, Schwab S, Kolominsky-Rabas PL. Changes in cognitive function over 3 years after first-ever stroke and predictors of cognitive impairment and long-term cognitive stability: the Erlangen Stroke Project. Dement Geriatr Cogn Disord. (2011) 31:291–9. doi: 10.1159/000327358
26. Desmond DW, Moroney JT, Sano M, Stern Y. Recovery of cognitive function after stroke. Stroke. (1996) 27:1798–803. doi: 10.1161/01.STR.27.10.1798
27. Obaid M, Flach C, Marshall I, C DAW, Douiri A. Long-term outcomes in stroke patients with cognitive impairment: a population-based study. Geriatrics. 5:32. (2020). doi: 10.3390/geriatrics5020032
28. Casolla B, Caparros F, Cordonnier C, Bombois S, Hénon H, Bordet R, et al. Biological and imaging predictors of cognitive impairment after stroke: a systematic review. J Neurol. (2019) 266:2593–604. doi: 10.1007/s00415-018-9089-z
29. Edlow BL, Claassen J, Schiff ND, Greer DM. Recovery from disorders of consciousness: mechanisms, prognosis and emerging therapies. Nat Rev Neurol. (2021) 17:135–56. doi: 10.1038/s41582-020-00428-x
30. Silva S, de Pasquale F, Vuillaume C, Riu B, Loubinoux I, Geeraerts T, et al. Disruption of posteromedial large-scale neural communication predicts recovery from coma. Neurology. (2015) 85:2036–44. doi: 10.1212/WNL.0000000000002196
31. Soch J, Richter A, Schütze H, Kizilirmak JM, Assmann A, Behnisch G, et al. A comprehensive score reflecting memory-related fMRI activations and deactivations as potential biomarker for neurocognitive aging. Hum Brain Mapp. (2021) 42:4478–96. doi: 10.1002/hbm.25559
32. Bhalla A, Wang Y, Rudd A, Wolfe CD. Differences in outcome and predictors between ischemic and intracerebral hemorrhage: the South London Stroke Register. Stroke. (2013) 44:2174–81. doi: 10.1161/STROKEAHA.113.001263
33. Wei JW, Heeley EL, Wang JG, Huang Y, Wong LK, Li Z, et al. Comparison of recovery patterns and prognostic indicators for ischemic and hemorrhagic stroke in China: the ChinaQUEST (QUality Evaluation of Stroke Care and Treatment) Registry study. Stroke. (2010) 41:1877–83. doi: 10.1161/STROKEAHA.110.586909
34. Sorensen AG, Ay H. Transient ischemic attack: definition, diagnosis, risk stratification. Neuroimaging Clin N Am. (2011) 21:303. doi: 10.1016/j.nic.2011.01.013
35. Abbott AL, Silvestrini M, Topakian R, Golledge J, Brunser AM, de Borst GJ, et al. Optimizing the definitions of stroke, transient ischemic attack, and infarction for research and application in clinical practice. Front Neurol. (2017) 8:537–7. doi: 10.3389/fneur.2017.00537
36. Gottesman RF, Hillis AE. Predictors and assessment of cognitive dysfunction resulting from ischaemic stroke. Lancet Neurol. (2010) 9:895–905. doi: 10.1016/S1474-4422(10)70164-2
37. Peng Y, Li Q, Qin L, He Y, Luo X, Lan Y, et al. Combination of serum neurofilament light chain levels and MRI markers to predict cognitive function in ischemic stroke. Neurorehabil Neural Repair. (2021) 35:247–55. doi: 10.1177/1545968321989354
38. Sagnier S, Catheline G, Munsch F, Bigourdan A, Poli M, Debruxelles S, et al. Severity of small vessel disease biomarkers reduces the magnitude of cognitive recovery after ischemic stroke. Cerebrovasc Dis. (2021) 50:456–63. doi: 10.1159/000513916
39. Sagnier S, Okubo G, Catheline G, Munsch F, Bigourdan A, Debruxelles S, et al. Chronic cortical cerebral microinfarcts slow down cognitive recovery after acute ischemic stroke. Stroke. (2019) 50:1430–36. doi: 10.1161/STROKEAHA.118.024672
40. Vicentini JE, Weiler M, Casseb RF, Almeida SR, Valler L, de Campos BM, et al. Subacute functional connectivity correlates with cognitive recovery six months after stroke. Neuroimage Clin. (2021) 29:102538. doi: 10.1016/j.nicl.2020.102538
41. Fruhwirth V, Enzinger C, Fandler-Höfler S, Kneihsl M, Eppinger S, Ropele S, et al. Baseline white matter hyperintensities affect the course of cognitive function after small vessel disease-related stroke: a prospective observational study. Eur J Neurol. (2021) 28:401–10. doi: 10.1111/ene.14593
42. Scharf AC, Gronewold J, Todica O, Moenninghoff C, Doeppner TR, de Haan B, et al. Evolution of neuropsychological deficits in first-ever isolated ischemic thalamic stroke and their association with stroke topography: a case-control study. Stroke. (2022) 53:1904–14. doi: 10.1161/STROKEAHA.121.037750
43. Sagnier S, Catheline G, Dilharreguy B, Munsch F, Bigourdan A, Poli M, et al. Admission brain cortical volume: an independent determinant of poststroke cognitive vulnerability. Stroke. (2017) 48:2113–20. doi: 10.1161/STROKEAHA.117.017646
44. Sung PS, Lee KP, Lin PY, Su HC, Yu RL, Tsai KJ, et al. Factors associated with cognitive outcomes after first-ever ischemic stroke: the impact of small vessel disease burden and neurodegeneration. J Alzheimers Dis. (2021) 83:569–79. doi: 10.3233/JAD-210587
45. Turunen KE, Kauranen TV, Laari SP, Mustanoja SM, Tatlisumak T, Poutiainen ET. Cognitive deficits after subcortical infarction are comparable with deficits after cortical infarction. Eur J Neurol. (2013) 20:286–92. doi: 10.1111/j.1468-1331.2012.03844.x
46. Zhang C, Wang Y, Li S, Pan Y, Wang M, Liao X, et al. Infarct location and cognitive change in patients after acute ischemic stroke: the ICONS study. J Neurol Sci. (2022) 438:120276. doi: 10.1016/j.jns.2022.120276
47. Aben HP, Biessels GJ, Weaver NA, Spikman JM, Visser-Meily JMA, de Kort PLM, et al. Extent to which network hubs are affected by ischemic stroke predicts cognitive recovery. Stroke. (2019) 50:2768–74. doi: 10.1161/STROKEAHA.119.025637
48. Aben HP, De Munter L, Reijmer YD, Spikman JM, Visser-Meily JMA, Biessels GJ, et al. Prediction of cognitive recovery after stroke: the value of diffusion-weighted imaging-based measures of brain connectivity. Stroke. (2021) 52:1983–92. doi: 10.1161/STROKEAHA.120.032033
49. Kuceyeski A, Navi BB, Kamel H, Raj A, Relkin N, Toglia J, et al. Structural connectome disruption at baseline predicts 6-months post-stroke outcome. Hum Brain Mapp. (2016) 37:2587–601. doi: 10.1002/hbm.23198
50. Lv H, Wang Z, Tong E, Williams LM, Zaharchuk G, Zeineh M, et al. Resting-state functional MRI: everything that nonexperts have always wanted to know. AJNR Am J Neuroradiol. (2018) 39:1390–9. doi: 10.3174/ajnr.A5527
51. Zhu Y, Bai L, Liang P, Kang S, Gao H, Yang H. Disrupted brain connectivity networks in acute ischemic stroke patients. Brain Imaging Behav. (2017) 11:444–53. doi: 10.1007/s11682-016-9525-6
52. Siegel JS, Ramsey LE, Snyder AZ, Metcalf NV, Chacko RV, Weinberger K, et al. Disruptions of network connectivity predict impairment in multiple behavioral domains after stroke. Proc Natl Acad Sci USA. (2016) 113:E4367–76. doi: 10.1073/pnas.1521083113
53. Tang C, Zhao Z, Chen C, Zheng X, Sun F, Zhang X, et al. Decreased functional connectivity of homotopic brain regions in chronic stroke patients: a resting state fMRI study. PLoS ONE. (2016) 11:e0152875. doi: 10.1371/journal.pone.0152875
54. Hohenfeld C, Werner CJ, Reetz K. Resting-state connectivity in neurodegenerative disorders: is there potential for an imaging biomarker? Neuroimage Clin. (2018) 18:849–70. doi: 10.1016/j.nicl.2018.03.013
55. Chan E, Altendorff S, Healy C, Werring DJ, Cipolotti L. The test accuracy of the Montreal Cognitive Assessment (MoCA) by stroke lateralisation. J Neurol Sci. (2017) 373:100–4. doi: 10.1016/j.jns.2016.12.028
56. Perna R, Temple J. Rehabilitation outcomes: ischemic versus hemorrhagic strokes. Behav Neurol. (2015) 2015:891651. doi: 10.1155/2015/891651
57. Reitz C, Bos MJ, Hofman A, Koudstaal PJ, Breteler MMB. Prestroke cognitive performance, incident stroke, and risk of dementia. Stroke. (2008) 39:36–41. doi: 10.1161/STROKEAHA.107.490334
58. Kandiah N, Chander RJ, Lin X, Ng A, Poh YY, Cheong CY, et al. Cognitive impairment after mild stroke: development and validation of the SIGNAL2 risk score. J Alzheimers Dis. (2016) 49:1169–77. doi: 10.3233/JAD-150736
Keywords: ischemic stroke, reperfusion, recovery, reorganization, neuroplasticity, neuroimaging, cognition
Citation: Tahmi M, Kane VA, Pavol MA and Naqvi IA (2022) Neuroimaging biomarkers of cognitive recovery after ischemic stroke. Front. Neurol. 13:923942. doi: 10.3389/fneur.2022.923942
Received: 19 April 2022; Accepted: 23 November 2022;
Published: 14 December 2022.
Edited by:
Keith Pennypacker, University of Kentucky, United StatesReviewed by:
Viktoria Fruhwirth, Medical University of Graz, AustriaCopyright © 2022 Tahmi, Kane, Pavol and Naqvi. This is an open-access article distributed under the terms of the Creative Commons Attribution License (CC BY). The use, distribution or reproduction in other forums is permitted, provided the original author(s) and the copyright owner(s) are credited and that the original publication in this journal is cited, in accordance with accepted academic practice. No use, distribution or reproduction is permitted which does not comply with these terms.
*Correspondence: Imama A. Naqvi, aWFuMjEwOEBjdW1jLmNvbHVtYmlhLmVkdQ==
†These authors share first authorship