- Department of Biology, Basic Sciences School, Shahed University, Tehran, Iran
Methadone is the most frequently used opioid therapy worldwide, with controversial effects on oxidative stress homeostasis. This study investigated the effects of intraperitoneal (i.p.) co-administration of methadone (0.1, 0.3, 1, and 3 mg/kg) and valproate sodium (300 mg/kg) or gabapentin (50 mg/kg) in the mice maximal electroshock (MES)-induced seizure model. The adverse effect of drugs was assessed using the chimney test. The levels of tumor necrosis factor-alpha (TNF-α) and malondialdehyde (MDA) contents were measured in mice brains after a single seizure. Administration of methadone alone resulted in a significant reduction in the duration of hind limb extension (HLE) than that in the control group. Methadone pretreatment at doses of 0.1 and 0.3 mg/kg i.p. decreased, and at doses of 1 and 3 mg/kg i.p. had an increasing effect on anticonvulsant efficacy of gabapentin. Pretreatment with all doses of methadone significantly decreased the valproate anticonvulsive efficacy. At doses of 1 and 3 mg/kg i.p. methadone per se increased brain MDA levels after MES-induced seizure. Administration of methadone (0.3 mg/kg i.p.) enhanced and at 3 mg/kg decreased gabapentin effect on brain MDA level, but their co-treatment did not lead to further increase in MDA. Methadone at 0.3–3 mg/kg enhanced the effect of sodium valproate on MDA levels in the brain, but at all doses significantly potentiated its effect on brain TNF-α levels. The drugs did not produce any side effects on motor coordination in experimental animals. In conclusion, methadone showed different effects on anticonvulsant actions of gabapentin and valproate through regulation of brain levels of MDA and TNF-α.
Introduction
Despite acceptable progress, complete seizure control is not achieved in >30% of patients with epilepsy (1). Polytherapy is a promising approach recently proposed to treat resistant epilepsy and the actual prevention of the disease (2). Animal models were used to preselect suitable combinations of drugs with anticonvulsants that could finally be evaluated in clinics. Targeting endogenous opioid systems due to their anticonvulsant and neuroprotective properties has been proposed as a new therapeutic approach for epilepsy treatment (3). The literature review indicated that opioid drugs, either directly or in combination with other anticonvulsant medications, might lead to contradictory results in terms of seizure control (4–6). It was suggested that the accumulation of cytokines and lipid peroxidation products in the brain might lead to abnormal and excessive simultaneous firing in a group of neurons and epilepsy (7). Therefore, exploring the role of lipid peroxidation and cytokines in the regulation epilepsy-related processes may help us understand the molecular mechanisms behind epilepsy and also predict the success of this combination therapy in the treatment of epilepsy (8).
Previously, the combination of opioids and gabapentin prescription was used to treat pain beyond the usual medical condition treatment, and valproate was used as an analgesic adjuvant to reduce the development of morphine side effects (9, 10). The opioid agonists have been recognized for having potential for both proconvulsant and anticonvulsant effects in experimental models of epilepsy (11–14). Methadone is a synthetic mu receptors agonist used for opioid maintenance therapy. Furthermore, it has several pharmacological activities in addition to opioid activity, such as the antitumor, anti-inflammatory, and antioxidant activities (15, 16). This compound, as a neuromodulator, can suppress intrinsic nerve excitability (17, 18). It has also shown inhibitory effects on both T- and L-type calcium channels in neuroblastoma cells (19, 20). In addition, prenatal exposure of neonate rats with methadone caused a reduction in the levels of GABA proteins in the brain, reversible inhibitory effect on NMDA receptors (21), and acts as a regulator of excitation/inhibition balance in neuronal networks (22–25). GABA proteins are proteins that are directly connected through the intracellular domain to GABA-A receptors and regulate the membrane traffic of these receptors (26).
It prolonged the inactivation period of the neuronal sodium channel and suppressed the action potential firing in peripheral nerves (26, 27). It can be expected that the drug will also be able to influence the anticonvulsant activity of these drugs. So, studying the effect of methadone in combination with gabapentin or valproate in experimental model could significantly increase our knowledge about the mechanisms underlying seizure control in the brain.
Although the pathogenesis of epilepsy has not been fully clarified, the activation of neuroinflammatory and oxidative stress is an important perpetuating factor for epileptogenesis in drug-resistant patients (28). Tumor necrosis factor-alpha (TNF-α), one of the inflammatory markers, is produced and released from epileptic neurons (29). It induces a rapid and persistent decrease in inhibitory synaptic strength and downregulates GABA receptors in mature mouse hippocampal neurons (30). Several lines of evidence suggest that TNF-α potentiates excitatory transmission in both physiological and pathological conditions (31). Indeed, TNF-α plays an essential role in the maximal electroshock (MES)-induced seizure model (32). It was shown that lipid peroxidation products, together with TNF-α, lead to the development of epilepsy by induction of irreversible damage to phospholipids in neuronal cells (33). It has been shown that malondialdehyde (MDA), a lipid peroxidation product, can modify neuronal excitability by regulating the balance between excitatory and inhibitory neurotransmitters in the brain (34). Thus, there is a need for more in-depth knowledge about oxidative stress and inflammatory factors in the pathogenesis of seizures. Further analysis of these factors may better address the effects of anticonvulsant drugs on preventing seizure.
Accordingly, we hypothesized that methadone administration might improve the response to anticonvulsant therapy. We also examine the possible role of brain MDA and TNF-α in these interactions. The effects of treatments on motor coordination were also investigated using the chimney test.
Materials and methods
Experimental procedures
Drugs
Methadone hydrochloride was purchased from TEMAD Company, Tehran, Iran. Sodium valproate and methadone were received as gifts from Raha Daru and Meher Daru pharmaceutical companies, Tehran, Iran, respectively. Drugs were dissolved in phosphate-buffered saline (PBS) and serially diluted to require doses. Methadone, sodium valproate, and gabapentin were injected intraperitoneally (i.p.) 30, 45, and 60 min, respectively, before the MES-induced seizures based on previous data in the literature (35–37).
Animals
Adult male NMRI mice, procured from the Animal House of Shahed University, were used. The animals were housed four per cage in standard cages under environmental conditions with temperature: 22 ± 1°C; humidity: 60 ± 5%; reversed 12-h light/dark cycle with lights turned on at 19:00, and free access to food palate and water ad libitum. Mice were only used once in each experiment, and a new group of mice was used for each dose and drug tested. The experiments were performed from 08:00 to 14:00. All experiments were performed in accordance with the NIH Guide for the Care and Use of Laboratory Animals (NIH, 1996). The research protocol was approved by the local ethical committee of Shahed University with the code number IR.SHAHED.REC.1400.072. An effort was made to reduce animal numbers and suffering.
Behavioral evaluations
MES-induced seizure model
Electroconvulsions were generated by alternating current (10 Hz, 37.2 mA, and 0.2 s) delivered via ear-clip electrodes connected to a stimulator apparatus (Borj Sanat Co. Tehran-Iran) as described by Jahani et al. (38). Electrodes were moistened with saline solution before each application, and the mice were observed in the duration of hind limb extension (HLE) in mice. The reduction in HLE duration was an index of anticonvulsant success. The percentages of protection were calculated according to the following formula:
Protection % = 100–(number of mice showing HLE/total number of mice in each group) × 100 (35).
The chimney test
Acute adverse effects produced by the drugs alone and in combination were assessed using the chimney (motor performance) test (37). In this test, mice had to climb back up a vertical cylinder (30 cm in length and 3 cm in diameter) with a rough surface inside. The inability of the mouse to climb the cylinder in 60 s was considered as impaired locomotor capacity.
Treatment plan
In experiment 1, the duration of HLE was assessed after the administration of different doses of methadone (0.1, 0.3, 1, and 3 mg/kg i.p.), and its vehicles after MES. The methadone doses were chosen according to a previous report (22).
In experiment 2, the duration of HLE was assessed after the administration of saline (5 ml/kg i.p.), valproate (300 mg/kg i.p.), and gabapentin (50 mg/kg i.p.) alone, after MES.
In experiment 3, the duration of HLE was determined after the administration of methadone + valproate sodium and methadone + gabapentin. Mice were first treated with saline or methadone before administration of valproate or gabapentin, and MES (22, 36).
Each animal was individually placed in the center of the plexiglass chamber during each trial.
Biochemical measurements
At the end of the experiments, animals were sacrificed by decapitation under deep anesthesia. Next, the brain was immediately removed, weighed, and washed with 50 mM (pH 7.4) ice-cold PBS solution. Then, it was placed in 1/5 (w/v) PBS containing a protease inhibitor cocktail and homogenized for 30 s with 20-s intervals using an ultrasonic homogenizer (Hielscher, UP200H, Germany). The samples were centrifuged at 10,000 rpm for 10 min at 4°C (38), and supernatants were used to determine MDA, TNF levels, and protein contents.
Measurement of brain tissue MDA and TNF-α
The tissue level of MDA and TNF-α contents was measured in the brain homogenates of mice after a single seizure with commercial kits (Karmania Pars Gene Company, Kerman, Iran), as previously described (29, 39). The concentrations of MDA and TNF-α were measured in brain homogenates that were prepared immediately after MES seizure. Protein concentration in brain homogenates was determined using Bradford's method (Karmania Pars Gene Company, Kerman, Iran). MDA concentration is expressed as nmol/mg protein tissue, and brain TNF-α levels are expressed as pg/mg protein.
Statistical analysis
Results are expressed as HLE and percentage protection and mean ± SEM where applicable. The data were analyzed by one-way ANOVA and Tukey's post-hoc test (p ≤ 0.05) using GraphPad Prism 5.0. Results obtained with the chimney test are presented as the percentage of impaired mice in groups of 10 animals and were compared with Fisher's exact probability test. Statistical significance for biochemical assays was determined using two-way ANOVA for multigroup comparisons.
Results
Effect of methadone on MES-induced seizures in mice
Table 1 shows the significant effects of methadone (0.1, 0.3, 1, and 3 mg/kg i.p.), valproate sodium (300 mg/kg i.p.), and gabapentin (50 mg/kg i.p.) acute administration on MES-induced seizure (one-way ANOVA; F6, 63 = 0.8806, p < 0.0001). Tukey's multiple-comparisons post-hoc analysis showed that the duration of HLE was significantly (p < 0.001) reduced by administration of methadone (0.3 and 3 mg/kg i.p.) with protection percentage of 24 and 13.3, respectively. Treatment with sodium valproate (300 mg/kg) and gabapentin (50 mg/kg) alone decreased duration of HLE, and provided 53 and 45% protection against MES-induced seizure in mice.
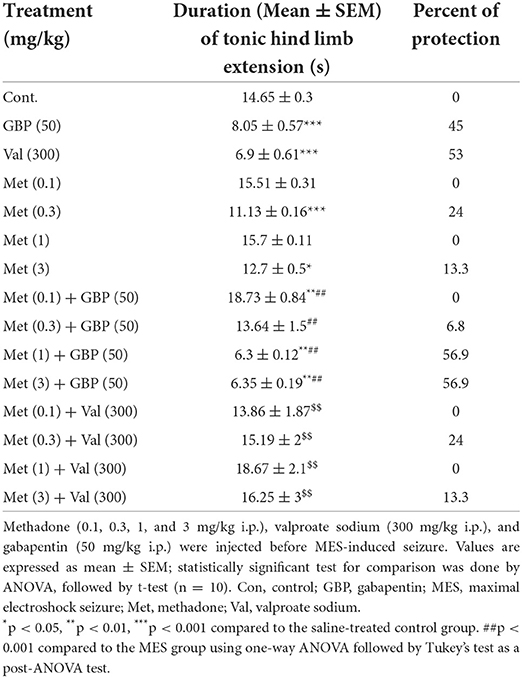
Table 1. Effect of methadone, gabapentin, and sodium valproate, and their combination pretreatment on MES-induced seizures in mice.
Effect of methadone in combination with gabapentin or valproate on MES-induced seizures
Effects of methadone on anticonvulsant effects of gabapentin are shown in Table 1. Two-way ANOVA, including methadone (0.1, 0.3, 1, and 3 mg/kg) and gabapentin (50 mg/kg), revealed a significant effect (factor methadone, F4, 90 =19.36, p < 0.001; factor gabapentin; F1, 90 = 31.91, p < 0.001; factor methadone × gabapentin, F4, 90 = 19.3, p = 0.0001. Post-hoc analyses showed that methadone at doses of 0.1 and 0.3 mg/kg significantly (p < 0.001) decreased the effect of gabapentin on HLE duration, while at doses of 1 and 3 mg/kg it caused increased anticonvulsant effect of the gabapentin significantly (p < 0.001) with protection percentage of 56.9 and 56.9% (Table 1).
Moreover, the results (Table 1) showed a significant interaction between methadone (0.1, 0.3, 1, and 3 mg/kg) and valproate sodium (300 mg/kg) on MES (two-way ANOVA; factor methadone, F4, 90 = 4.98, p < 0.001; factor valproate sodium, F1, 90 = 5.69, p < 0.001, factor methadone × valproate sodium, F4, 90 = 6.2, p = 0.0002). Further analyses showed that methadone at all doses significantly (p < 0.05) reduced the duration of the convulsions and protection percentage of valproate sodium (300 mg/kg) against the MES-induced convulsions.
Effects of different doses of methadone alone or in combination with valproate or gabapentin on motor coordination of mice after application of MES
The influence of the investigated compounds on motor coordination in mice is presented in Table 2. Our results showed that treatments of methadone both alone and combined with anticonvulsants had no impact on motor coordination in mice.
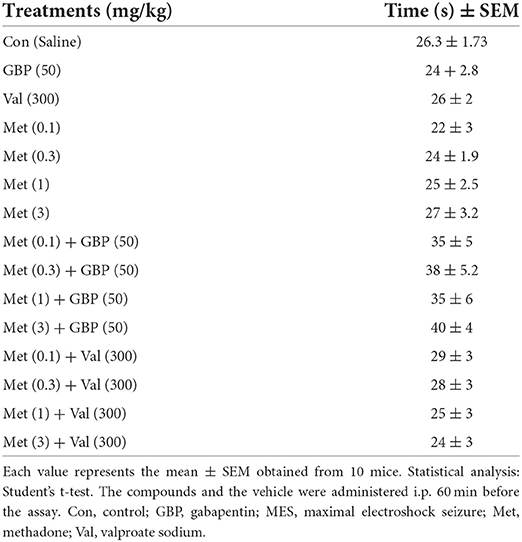
Table 2. Effect of methadone, gabapentin, and valproate sodium pretreatment per se, and combined therapy on motor coordination of mice in the chimney test.
Influence of pretreatment with different doses of methadone, gabapentin, and valproate per se on the brain MDA level in the mice after MES-induced seizure
There were significant differences in the mean brain MDA levels between different groups (p < 0.05). The results showed that brain MDA levels increased significantly (p < 0.001) after MES challenge compared to the control group. Pretreatment with gabapentin (50 mg/kg i.p.) or valproate sodium (300 mg/kg i.p.) per se significantly reduced brain level of MDA after MES administration (Figure 1A). Brain MDA content was significantly (p < 0.01) decreased following injection of all doses of methadone compared to vehicle-treated MES-induced seizure animals (Figure 1B).
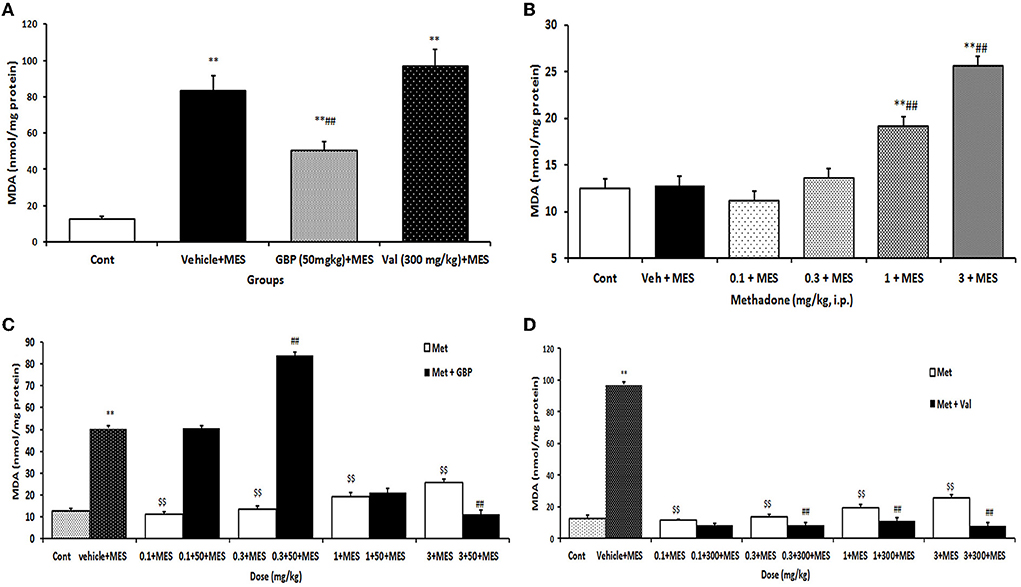
Figure 1. Changes of MDA (nmol/mg protein) in the brain of mice in different treatment groups: (A) changes of brain MDA level in the saline-treated control, 50 mg/kg i.p. gabapentin (GBP) and 300 mg/kg i.p. valproate (Val)-treated groups after MES-induced seizure; (B) changes of brain MDA level of mice after treatment with (0.1, 0.3, 1, and 3 mg/kg i.p.) Met and MES-induced seizure; (C) changes of brain MDA level treated with GBP (50 mg/kg i.p.) per se and different doses of Met plus GBP before MES; (D) changes of brain MDA level treated with valproate (Val) (300 mg/kg i.p.) per se and different doses of Met plus Val before MES. Data are expressed as mean ± SEM. N = 10. **p < 0.01compared with the normal group, $$p < 0.01 treatments vs. vehicle + MES, and ##p < 0.001 compared with the saline + MES group using one-way ANOVA followed by Tukey's test as a post-ANOVA test. Cont, control; GBP, gabapentin; Met, methadone; Val, valproate sodium; MES, maximal electroshock seizure.
Influence of combined therapy with methadone and gabapentin or valproate on the brain MDA level in the mice after MES-induced seizures
Methadone at doses of 0.1 and 0.3 mg/kg i.p. decreased the reducing effect of gabapentin on MES-induced increase of MDA levels. But at higher doses it increased the suppressive effect of gabapentin on the increase in MDA level induced by MES in the brain (Figure 1C). Methadone at doses of 0.3, 1, and 3 mg/kg i.p. significantly increased the suppression of brain MDA levels produced by valproate after MES-induced seizures (Figure 1D).
Influence of treatment with different doses of methadone, gabapentin, and valproate per se on the brain TNF-α level in MES-induced seizure in mice
MES-induced seizures increased TNF-α levels significantly (p < 0.001) in mice brains compared to the control group. Treatment with both gabapentin (50 mg/kg i.p.) and valproate sodium (300 mg/kg i.p.) per se significantly (p < 0.01) prevented MES-induced TNF-α increase in the brain (Figure 2A). Treatment with all doses of methadone before MES-induced seizures suppressed the increase in the TNF-α levels in brain tissue (Figure 2B).
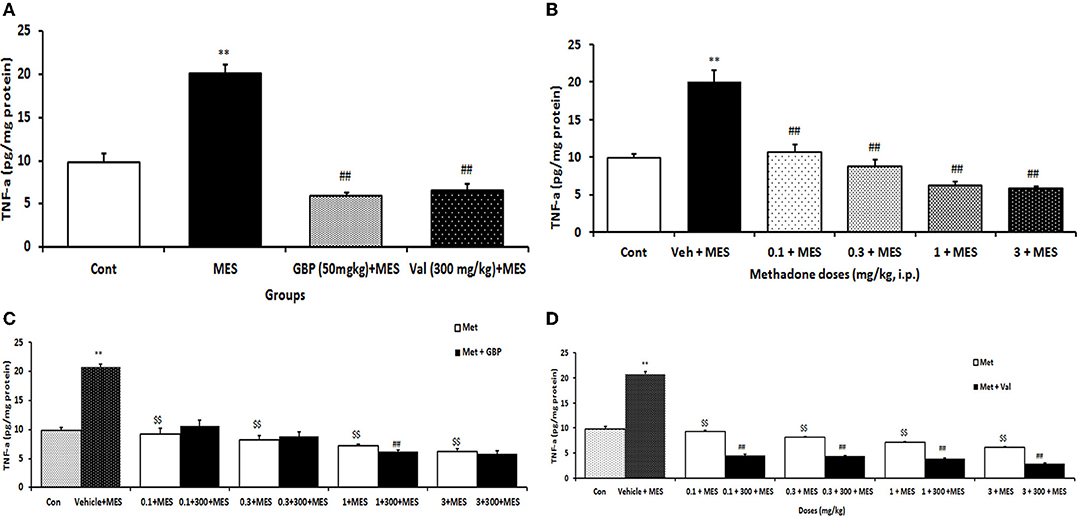
Figure 2. Changes of TNF-α (pg/mg protein) in the brain of mice in different treatment groups: (A) changes of brain TNF-α level in the saline-treated control, 50 mg/kg i.p. gabapentin (GBP) and 300 mg/kg i.p. valproate (Val)-treated groups after MES-induced seizure; (B) changes of brain TNF-α level of mice after treatment with (0.1, 0.3, 1, and 3 mg/k i.p.) Met and MES-induced seizure; (C) changes of brain TNF-α level treated with GBP (50 mg/kg i.p.) per se and different doses of Met plus GBP before MES; (D) changes of brain TNF-α level treated with valproate (Val) (300 mg/kg i.p.) per se and different doses of Met plus Val before MES. Data are expressed as mean ± SEM. N = 10. **p < 0.01 compared with the normal group, $$p < 0.01 treatments vs. vehicle + MES, and ##p < 0.001 compared with the saline + MES group using two-way ANOVA followed by Tukey's test as a post-ANOVA test. Cont, control; GBP, gabapentin; Met, methadone; Val, valproate sodium; MES, maximal electroshock seizure.
Influence of combination therapy with methadone and gabapentin or valproate sodium on brain TNF-α level in mice following MES-induced seizure
Treatment with methadone significantly (p < 0.01) increased the suppressive effect of gabapentin on TNF-α level in the brain only at the dose 1 mg/kg (Figure 2C), while methadone at all doses increased the suppressive effect of valproate on the TNF-α level in the brain of mice receiving MES (Figure 2D).
Discussion
Methadone is a drug used to treat morphine dependence that has been reported to show additional therapeutic action on inflammation and oxidative stress (40, 41). It is interesting that, in addition to opioid receptor agonist properties, methadone acts as a sodium and potassium channel blocker and NMDA receptor antagonist (24, 26). Both proconvulsant and anticonvulsant effects have been reported for NMDA receptor antagonists and potassium channels (42–44). Therefore, this study was designed to evaluate the potential impact of methadone alone or combined with two antiepileptic drugs on epilepsy and measure the amount of a lipid peroxidation product and cytokines in the brain.
In this study, we showed that pretreatment of mice with methadone resulted in a dual effect on the duration of the HLE in MES-induced seizures. Methadone at doses of 0.3 and 3 mg/kg significantly reduces this parameter compared to the control group. The percentage of seizure inhibition by methadone is 24 and 13.3% in 0.3 and 3 mg/kg dosage, respectively, compared to the control group. This effect is probably pharmacodynamic as there is no evidence that opioids affect the brain concentration of two antiepileptic drugs. This shows that methadone induced a U-shaped change in seizure suppression in comparison to the control group. These findings are consistent with a previous report that opioid agonists have a biphasic effect on seizure modulation (45). Furthermore, this study showed that the suppression of MES-induced seizures produced by gabapentin and valproate was affected by pretreatment with methadone. Therefore, we identified a new way to evaluate the relationship between opioids and anticonvulsants for either therapeutic purposes or drug interactions.
Similarly, a previous study has reported a dose-dependent effect of opioids in pentylenetetrazole (PTZ)-induced seizures, which supports the idea that methadone, as an opioid agonist, has a dual effect of inhibiting electrically induced seizures (46). Some publications are consistent with our results showing that opioid agonists have controversial dose-dependent effects on seizure activity in different animal seizure/epilepsy models (47–50). An earlier study has shown a reduction in the severity of PTZ-induced seizures after dynorphin treatment in mice, supporting our finding (51). Available experimental data indicated that opioid agonists, regardless of their action on the seizure threshold, showed potential for changing the effects of antiepileptic drugs (52).
In contrast, many experiments have shown that the interaction of opioid agonists with the agents has shown an anticonvulsant effect (53, 54). Therefore, the association between opioid receptor agonists and anticonvulsant drugs is balanced between increasing and decreasing effects on the seizure threshold. However, only a few studies examined the interaction between opioid agonists, anticonvulsant drugs, and possible molecular mechanisms of the disease. Therefore, we evaluated the effect of methadone treatment on the efficacy of two anticonvulsant drugs and the possible mechanisms involved in these conditions.
The induction of seizure by MES represents an experimental model of tonic–clonic seizures with similarities to human seizures (55, 56). The model has also shown high sensitivity to compounds affecting opioidergic mechanisms (57). Prolonged tonic phase of the seizure has been found to affect antioxidant enzyme activity and increase lipid peroxidation in the brain (58, 59).
In this study, methadone administration resulted in (1) reduced duration of HLE; (2) increased percentage of protection against electroshock-induced seizure; (3) different effects on the efficacy of gabapentin and valproate; (4) attenuation of the brain MDA and TNF-α levels; and (5) no adverse effects on motor coordination.
The findings of this study are in concordance with an earlier study in which combined treatment with a plant extract with anti-inflammatory and antioxidant activity and valproate suppressed the development of seizures more effectively in patients with epilepsy who were refractory to standard antiepileptic treatment (60). Similarly, it was reported that a plant extract containing antioxidants enhanced the antiseizure effect of carbamazepine in an experimental model of acute seizures (61).
To explain the observed alteration in the antiseizure effects of gabapentin and valproate by methadone, one should consider the molecular mechanisms of action of these drugs on brain neurotransmitters and neuronal ion channels under modulatory control of MDA and TNF-α. Many studies have shown that an increase in lipid peroxidation levels in the brain has a pivotal role in epilepsy that in turn ignites the neuroinflammation cascades in the brain (62, 63). Scientific evidence indicates that success in the treatment of seizure is associated with the suppression of neuroinflammation, excitotoxicity, and enhancement of inhibitory neurotransmission in the brain (64). Also, a reciprocal relationship exists between inhibitory neurotransmission, reactive oxygen species, and TNF-α levels in the brain during the seizure (65–67). In this study, the MES group exhibited significant induction in lipid peroxidation and TNF-α production in the mice brains. This is in line with the previously described association between biosynthesis of cytokines and acute seizures in rodents (68). Methadone administration was able to impede the elevation of MDA and TNF-α in the MES-induced seizure in mice brains, suggesting the probable antioxidative and anti-inflammatory effect of methadone that may contribute to its protective action toward seizure and antiepileptic medication. Previous investigations showed a correlation between plasma levels of cytokine and methadone maintenance therapy outcomes in patients with opioid use disorder (69).
Moreover, methadone has demonstrated anti-inflammatory activity by activating opioid receptors (16). Several publications have shown a relationship between increased lipid peroxidation and cytokines levels in the brain and change in the potency of anticonvulsant drugs (70, 71). Oxidative stress and inflammation are interrelated mechanisms involved in the regulation of neural activity, and increasing their levels in the brain may contribute to hyperexcitability and epilepsy (72). In addition to its direct effect on GABA receptors, gabapentin has been shown to have anti-inflammatory effects that may contribute to its neuroprotective effect against seizure severity (68, 73). Previous investigations using a neuropathic pain model showed a synergistic effect between anti-inflammatory agents and gabapentin (74).
However, valproate exerts its effects by increasing the concentrations of GABA via inhibiting its metabolism and increasing its synthesis (75). Therefore, there is still a significant and positive association between an increase in the oxidative stress markers and a decrease in the antioxidant levels with the number of seizures in valproate monotherapy (65).
In this study, we observed increased MDA and TNF-α levels in the brains of mice after MES, suggesting their role in epileptogenesis, which is in line with the previous studies where seizure induced excitotoxicity and increase in oxidative stress and cytokine levels in the brain (76). Our results also demonstrated that administration of methadone increased brain tissue content of MDA while decreasing TNF-α levels, suggesting their role in the development of MES-induced seizures. Furthermore, the administration of methadone and the anticonvulsant drugs enhanced their suppressive effect on the levels of MDA and TNF-α in the brains of mice at effective anticonvulsant doses. Another study revealed the improvement of mortality rate in kainic acid-induced epilepsy by inhibiting the inflammation and oxidant stress (77). Furthermore, recent evidence has documented that suppression of oxidative stress/cytokine production contributes to the antiseizure potency of anticonvulsant drugs (78).
MDA, as the by-product of the peroxidation of polyunsaturated fatty acids, is toxic to neurons and plays a crucial role in the induction of neuroinflammation. An increase in its brain levels leads to the progression of epilepsy through destabilization of the lipid membrane (79). There is a positive correlation between plasma MDA level and seizure severity in patients with epilepsy (80, 81). In the pilocarpine-induced seizure model, administration of antioxidants could protect the neuron's lipid peroxidation and nitrite formation-induced damages (82, 83). This is in line with a previous study that reported that MES-induced seizures increased oxidative stress, neurotoxicity, and cognitive impairment (84). The administration of methadone per se and in combination with antidepressants was able to suppress lipid peroxidation and inflammation in the brains of mice receiving MES, suggesting the potential anti-inflammatory and antioxidant activity of methadone. Therefore, methadone at specific doses is regarded as an anticonvulsant adjuvant and protects the brain from seizure damage.
TNF-α is an endogenous cytokine whose rise in the brains of rodents is caused by seizure activity and clinical subjects after seizure activity (85). In the central nervous system, TNF-α as a pro-inflammatory cytokine has a regulatory action on neuronal excitability and myelin stability under physiological conditions (86). TNF-α is both produced by neurons in physiological conditions (32) and overproduced by overactive microglia under pathological conditions (87). Recent studies have shown paradoxical effects of TNF-α on seizure (88, 89). This finding may partly explain why methadone administration alone and in adjunctive therapy with an anticonvulsant regimen failed to completely suppress seizure responses. Indeed, other factors, such as the regulation of GABA release, may mediate these effects (90, 91). Today, it is known that the narrow and different therapeutic dosage range of antiepileptic compounds can be caused by the differences in the mechanisms of epilepsy induction in acute and chronic models or the different methods of drug administration (92, 93).
The data in this study report the controversial effect of methadone per se and in combination therapy gabapentin and valproate effects on MES-induced seizures in mice. Accordingly, this study showed that administration of methadone with gabapentin and valproate in mice with MES-induced seizure affected brain MDA and TNF-α levels, which might be possible mechanisms of methadone effects on the modulation of anticonvulsant drugs. Further studies are required to establish the exact mechanism of action of the opioid agonists on the modulation of anticonvulsant drugs and warrant further investigations.
Conclusion and perspectives
The data in this study are the first report of biphasic anticonvulsant and proconvulsant effects of methadone on MES-induced seizures. In addition, the data show that methadone interacts with the anticonvulsive effects of valproate sodium and gabapentin, which were free from adverse effects on motor coordination. Specifically, the mix of methadone as an opioid receptor agonist and gabapentin and valproate as anticonvulsants display increased and decreased potency, respectively, in mice with MES-induced seizure. The diverse effects of methadone pretreatment shown here make it important for its potential application in human translation studies and aimed at preventing inappropriate drug therapeutic interactions in the treatment of epilepsy. Moreover, brain levels of MDA and TNF-α are shown to serve as viable candidates for drug interactions efficacy monitoring, hence facilitating the quest for antiepileptic drug therapies. The mechanisms responsible for these effects are unclear from this study but seem to involve changes in brain MDA and TNF-α levels.
Data availability statement
The raw data supporting the conclusions of this article will be made available by the authors, without undue reservation.
Ethics statement
The animal study was reviewed and approved by Ethical Committee of Shahed University with the code number: IR.SHAHED.REC.1400.072.
Author contributions
AM performed experiments, assessed, and prepared the data. MH co-performed animal experiments, assessed and analyzed data, designed and coordinated the study, interpreted data and wrote the article. All authors contributed to the article and approved the submitted version.
Acknowledgments
The authors thank the vice-chancellor of the Technology and Research of Shahed University and Mehdi Hosseini for their support and help.
Conflict of interest
The authors declare that the research was conducted in the absence of any commercial or financial relationships that could be construed as a potential conflict of interest.
Publisher's note
All claims expressed in this article are solely those of the authors and do not necessarily represent those of their affiliated organizations, or those of the publisher, the editors and the reviewers. Any product that may be evaluated in this article, or claim that may be made by its manufacturer, is not guaranteed or endorsed by the publisher.
References
1. Löscher W, Klein P. The pharmacology and clinical efficacy of antiseizure medications: From bromide salts to cenobamate and beyond. CNS Drugs. (2021) 35:935–63. doi: 10.1007/s40263-021-00827-8
2. Park KM, Kim SE, Lee BI. Antiepileptic drug therapy in patients with drug-resistant epilepsy. J Epilepsy Res. (2019) 9:14–26. doi: 10.14581/jer.19002
3. Tortella FC, DeCoster MA. Kappa opioids: therapeutic considerations in epilepsy and CNS injury. Clin Neuropharmacol. (1994) 17:403–16.
4. Bausch SB, Garland JP, Yamada J. The delta opioid receptor agonist, SNC80, has complex, dose-dependent effects on pilocarpine-induced seizures in Sprague-Dawley rats. Brain Res. (2005) 1045:38–44. doi: 10.1016/j.brainres.2005.03.008
5. Lesani A, Javadi-Paydar M, Khodadad TK, Asghari-Roodsari A, Shirkhodaei M, Norouzi A, et al. Involvement of the nitric oxide pathway in the anticonvulsant effect of tramadol on pentylenetetrazole-induced seizures in mice. Epilepsy Behav. (2010) 19:290–5. doi: 10.1016/j.yebeh.2010.08.006
6. Frey HH, Schicht S. Interaction of mu-opioid antagonistic drugs with antiepileptics. Pharmacol Toxicol. (1996) 78:264–8. doi: 10.1111/j.1600-0773.1996.tb00215.x
7. Marchi N, Granata T, Janigro D. Inflammatory pathways of seizure disorders. Trends Neurosci. (2014) 37:55–65. doi: 10.1016/j.tins.2013.11.002
8. Loscher W. Single versus combinatorial therapies in status epilepticus: novel data from preclinical models. Epilepsy Behav. (2015) 49:20–5. doi: 10.1016/j.yebeh.2015.02.027
9. Gomes T, Juurlink DN, Antoniou T, Mamdani MM, Paterson JM, van den Brink W. Gabapentin, opioids, and the risk of opioid-related death: A population-based nested case-control study. PLoS Med. (2017) 14:e1002396. doi: 10.1371/journal.pmed.1002396
10. Dobashi T, Tanabe S, Jin H, Nishino T, Aoe T. Valproate attenuates the development of morphine antinociceptive tolerance. Neurosci Lett. (2010) 485:125–8. doi: 10.1016/j.neulet.2010.08.084
11. Homayoun H, Khavandgar S, Namiranian K, Gaskari SA, Dehpour AR. The role of nitric oxide in anticonvulsant and proconvulsant effects of morphine in mice. Epilepsy Res. (2002) 48:33–41. doi: 10.1016/s0920–1211(01)00316–3
12. Lauretti GR, Ahmad I, Pleuvry BJ. The activity of opioid analgesics in seizure models utilizing N-methyl-d-aspartic acid, kainic acid, bicuculline and pentylenetetrazole. Neuropharmacol. (1994) 33:155–60. doi: 10.1016/0028–3908(94)90002–7
13. Garant DS, Gale K. Infusion of opiates into substantia nigra protects against maximal electroshock seizures in rats. J Pharmacol Exp Ther. (1985) 234:45–8.
14. Burtscher J, Schwarzer C. The opioid system in temporal lobe epilepsy: functional role and therapeutic potential. Front Mol Neurosci. (2017) 10:245. doi: 10.3389/fnmol.2017.00245
15. Michalska M, Katzenwadel A, Wolf P. Methadone as a “Tumor Theralgesic” against Cancer. Front Pharmacol. (2017) 8:733. doi: 10.3389/fphar.2017.00733
16. Fakhraei N, Javadian N, Rahimian R, Nili F, Rahimi N, Hashemizadeh S, et al. Involvement of central opioid receptors in protective effects of methadone on experimental colitis in rats. Inflammopharmacology. (2018) 26:1399–413. doi: 10.1007/s10787-018-0538-1
17. Gholami M, Saboory E, Ahmadi AA, Asouri M, Nasirikenari M, Rostamnezhad M. Long-time effects of prenatal morphine, tramadol, methadone, and buprenorphine exposure on seizure and anxiety in immature rats. Int J Neurosci. (2020) 130:898–905. doi: 10.1080/00207454.2019.1709841
18. Wu W, Yao H, Dwivedi I, Negraes PD, Zhao HW, Wang J, et al. Methadone suppresses neuronal function and maturation in human cortical organoids. Front Neurosci. (2020) 14:593248. doi: 10.3389/fnins.2020.593248
19. Lee CH, Berkowitz BA. Calcium antagonist activity of methadone, l-acetylmethadol and l-pentazocine in the rat aortic strip. J Pharmacol Exp Ther. (1977) 202:646–53.
20. Yang JC, Shan J, Ng KF., Pang P. Morphine and methadone have different effects on calcium channel currents in neuroblastoma cells. Brain Res. (2000) 870:199–203. doi: 10.1016/s0006-8993(00)02369-6
21. Lum JS, Bird KM, Wilkie J, Millard SJ, Pallimulla S, Newell KA, et al. Prenatal methadone exposure impairs adolescent cognition and GABAergic neurodevelopment in a novel rat model of maternal methadone treatment. Prog Neuropsychopharmacol Biol Psychiatry. (2021) 110:110281. doi: 10.1016/j.pnpbp.2021.110281
22. Kazemi Roodsari S, Bahramnejad E, Rahimi N, Aghaei I, Dehpour AR. Methadone's effects on pentylenetetrazole-induced seizure threshold in mice: NMDA/opioid receptors and nitric oxide signaling. Ann N Y Acad Sci. (2019) 1449:25–35. doi: 10.1111/nyas.14043
23. Mastronicola D, Arcuri E, Arese M, Bacchi A, Mercadante S, Cardelli P, et al. Morphine but not fentanyl and methadone affects mitochondrial membrane potential by inducing nitric oxide release in glioma cells. Cell Mol Life Sci. (2004) 61:2991–7. doi: 10.1007/s00018-004-4371-x
24. Matsui A, Williams JT. Activation of μ-opioid receptors and block of KIR 3 potassium channels and NMDA receptor conductance by l- and d-methadone in rat locus coeruleus. Br J Pharmacol. (2010) 161:1403–13. doi: 10.1111/j.1479–5381.2010.00967.x
25. Bobula B, Hess G. Effects of morphine and methadone treatments on glutamatergic transmission in rat frontal cortex. Pharmacol Rep. (2009) 61:1192–7. doi: 10.1016/s1734-1140(09)70183-5
26. Arancibia-Cárcamo IL, Kittler JT. Regulation of GABA(A) receptor membrane trafficking and synaptic localization. Pharmacol Ther. (2009) 123:17–31. doi: 10.1016/j.pharmthera.2009.03.012
27. Stoetzer C, Kistner K, Stüber T, Wirths M, Schulze V, Doll T, et al. Methadone is a local anaesthetic-like inhibitor of neuronal Na+ channels and blocks excitability of mouse peripheral nerves. Br J Anaesth. (2015) 114:110–20. doi: 10.1093/bja/aeu206
28. Traficante DC, Feibish G, Kashani J. Methadone-induced Torsades de Pointes Masquerading as Seizures. Clin Pract Cases Emerg Med. (2017) 1:40–3. doi: 10.5811/cpcem.2016.11.32664
29. Petrillo S, Pietrafusa N, Trivisano M, Calabrese C, Saura F, Gallo MG, et al. Imbalance of systemic redox biomarkers in children with epilepsy: role of ferroptosis. Antioxidants (Basel). (2021) 10:1267. doi: 10.3390/antiox10081267
30. Vezzani A, Friedman A. Brain inflammation as a biomarker in epilepsy. Biomark Med. (2011) 5:607–14. doi: 10.2217/bmm.11.61
31. Pribiag H, Stellwagen D. TNF-α downregulates inhibitory neurotransmission through protein phosphatase 1-dependent trafficking of GABA(A) receptors. J Neurosci. (2013) 33:15879–93. doi: 10.1523/JNEUROSCI.0530-13.2013
32. Probert L. TNF and its receptors in the CNS: the essential, the desirable and the deleterious effects. Neuroscience. (2015) 302:2–22. doi: 10.1016/j.neuroscience.2015.06.038
33. Pottoo FH, Salahuddin M, Khan FA, Al Dhamen MA, Alsaeed WJ, Gomaa MS, et al. Combinatorial regimen of carbamazepine and imipramine exhibits synergism against grandmal epilepsy in rats: inhibition of pro-inflammatory cytokines and pi3k/akt/mtor signaling pathway. Pharmaceuticals (Basel, Switzerland). (2021) 14:1204. doi: 10.3390/ph14111204
34. Dal-Pizzol F, Klamt F, Vianna MM, Schröder N, Quevedo J, Benfato MS, et al. Lipid peroxidation in hippocampus early and late after status epilepticus induced by pilocarpine or kainic acid in Wistar rats. Neurosci Lett. (2000) 291:179–82. doi: 10.1016/s0304-3940(00)01409-9
35. Li F, Yang Z, Lu Y, Wei Y, Wang J, Yin D, et al. Malondialdehyde suppresses cerebral function by breaking homeostasis between excitation and inhibition in turtle Trachemys scripta. PLoS ONE. (2010) 5:e15325. doi: 10.1371/journal.pone.0015325
36. Manocha A, Mediratta PK, Sharma KK. Studies on the anticonvulsant effect of U50488H on maximal electroshock seizure in mice. Pharmacol Biochem Behav. (2003) 76:111–7. doi: 10.1016/s0091-3057(03)00218-1
37. Fisseha N, Shibeshi W, Bisrat D. Evaluation of anticonvulsant activity of 80% methanolic root bark extract and solvent fractions of Pentas schimperiana (A. Rich) Vatke (Rubiaceae) in swiss albino mice. Adv Pharmacol Pharm Sci. (2021) 2021:6689879. doi: 10.1155/2021/6689879
38. Luszczki JJ, Wojda E, Andres-Mach M, Cisowski W, Glensk M, Glowniak K, et al. Anticonvulsant and acute neurotoxic effects of imperatorin, osthole and valproate in the maximal electroshock seizure and chimney tests in mice: a comparative study. Epilepsy Res. (2009) 85:293–9. doi: 10.1016/j.eplepsyres.2009.03.027
39. Luszczki JJ, Sawicka KM, Kozinska J, Dudra-Jastrzebska M, Czuczwar SJ. Amiloride enhances the anticonvulsant action of various antiepileptic drugs in the mouse maximal electroshock seizure model. J Neural Transm (Vienna). (2009) 116:57–66. doi: 10.1007/s00702-008-0152-2
40. Mao Z, Zheng YL, Zhang YQ. Behavioral impairment and oxidative damage induced by chronic application of nonylphenol. Int J Mol Sci. (2010) 12:114–27. doi: 10.3390/ijms12010114
41. Leventelis C, Goutzourelas N, Kortsinidou A, Spanidis Y, Toulia G, Kampitsi A, et al. Buprenorphine and methadone as opioid maintenance treatments for heroin-addicted patients induce oxidative stress in blood. Oxid Med Cell Longev. (2019) 2019:9417048. doi: 10.1155/2019/9417048
42. Hays L, Reid C, Doran M, Geary K. Use of methadone for the treatment of diabetic neuropathy. Diabetes Care. (2005) 28:485–7. doi: 10.2337/diacare.28.2.485
43. Wickenden AD. Potassium channels as anti-epileptic drug targets. Neuropharmacology. (2002) 43:1055–60. doi: 10.1016/s0028-3908(02)00237-x
44. Natchimuthu V, Bandaru S, Nayarisseri A, Ravi S. Design, synthesis and computational evaluation of a novel intermediate salt of N-cyclohexyl-N-(cyclohexylcarbamoyl)-4-(trifluoromethyl) benzamide as potential potassium channel blocker in epileptic paroxysmal seizures. Comput Biol Chem. (2016) 64:64–73. doi: 10.1016/j.compbiolchem.2016.05.003
45. Sotgiu ML, Valente M, Storchi R, Caramenti G, Biella GE. Cooperative N-methyl-D-aspartate (NMDA) receptor antagonism and mu-opioid receptor agonism mediate the methadone inhibition of the spinal neuron pain-related hyperactivity in a rat model of neuropathic pain. Pharmacol Res. (2009) 60:284–90. doi: 10.1016/j.phrs.2009.04.002
46. Potschka H, Friderichs E, Löscher W. Anticonvulsant and proconvulsant effects of tramadol, its enantiomers and its M1 metabolite in the rat kindling model of epilepsy. Br J Pharmacol. (2000) 131:203–12. doi: 10.1038/sj.bjp.0703562
47. Montaser-Kouhsari L, Payandemehr B, Gholipour T, Ziai P, Nabavizadeh P, Ghasemi A, et al. A role for opioid system in the proconvulsant effects of sildenafil on the pentylenetetrazole-induced clonic seizure in mice. Seizure. (2011) 20:409–13. doi: 10.1016/j.seizure.2011.01.014
48. Lee RJ, Bajorek JG, Lomax P. Similar anticonvulsant, but unique, behavioural effects of opioid agonists in the seizure-sensitive Mongolian gerbil. Neuropharmacology. (1984) 23:517–24. doi: 10.1016/0028-3908(84)90024-8
49. Shafaroodi H, Asadi S, Sadeghipour H, Ghasemi M, Ebrahimi F, Tavakoli S, et al. Role of ATP sensitive potassium channels in the biphasic effects of morphine on pentylenetetrazole-induced seizure threshold in mice. Epilepsy Res. (2007) 75:63–9. doi: 10.1016/j.eplepsyres.2007.04.005
50. Riazi K, Honar H, Homayoun H, Rashidi N, Kiani S, Ebrahimkhani MR, et al. The synergistic anticonvulsant effect of agmatine and morphine: possible role of alpha 2-adrenoceptors. Epilepsy Res. (2005) 65:33–40. doi: 10.1016/j.eplepsyres.2005.04.003
51. Honar H, Riazi K, Homayoun H, Sadeghipour H, Rashidi N, Ebrahimkhani MR, et al. Ultra-low dose naltrexone potentiates the anticonvulsant effect of low dose morphine on clonic seizures. Neurosci. (2004) 129:733–42. doi: 10.1016/j.neuroscience.2004.08.029
52. Loacker S, Sayyah M, Wittmann W, Herzog H, Schwarzer C. Endogenous dynorphin in epileptogenesis and epilepsy: anticonvulsant net effect via kappa opioid receptors. Brain. (2007) 130:1017–28. doi: 10.1093/brain/awl384
53. Jackson HC, Nutt DJ. Investigation of the involvement of opioid receptors in the action of anticonvulsants. Psychopharmacology (Berl). (1993) 111:486–90. doi: 10.1007/BF02253540
54. Yahyavi-Firouz-Abadi N, Tahsili-Fahadan P, Riazi K, Ghahremani MH, Dehpour AR. Melatonin enhances the anticonvulsant and proconvulsant effects of morphine in mice: role for nitric oxide signaling pathway. Epilepsy Res. (2007) 75:138–44. doi: 10.1016/j.eplepsyres.2007.05.002
55. Hassanipour M, Shirzadian A, Boojar MM, Abkhoo A, Abkhoo A, Delazar S, et al. Possible involvement of nitrergic and opioidergic systems in the modulatory effect of acute chloroquine treatment on pentylenetetrazol induced convulsions in mice. Brain Res Bull. (2016) 121:124–30. doi: 10.1016/j.brainresbull.2015.11.020
56. Loscher W, Nolting B. The role of technical, biological and pharmacological factors in the laboratory evaluation of anticonvulsant drugs. IV Protective indices. Epilepsy Res. (1991) 9:1–10. doi: 10.1016/0920–1211(91)90075–q
57. Loscher W, Fiedler M. The role of technical, biological and pharmacological factors in the laboratory evaluation of anticonvulsant drugs. VII Seasonal influences on anticonvulsant drug actions in mouse models of generalized seizures. Epilepsy Res. (2000) 38:231–48. doi: 10.1016/s0920–1211(99)00095–9
58. Tortella FC, Robles L, Echevarria E, Hunter JC, Hughes J. PD117302, a selective non-peptide opioid kappa agonist, protects against NMDA and maximal electroshock convulsions in rats. Life Sci. (1990) 46:PL1–7. doi: 10.1016/0024-3205(90)90501-h
59. Cardenas-Rodriguez N, Huerta-Gertrudis B, Rivera-Espinosa L, Montesinos-Correa H, Bandala C, Carmona-Aparicio L, et al. Role of oxidative stress in refractory epilepsy: evidence in patients and experimental models. Int J Mol Sci. (2013) 14:1455–76. doi: 10.3390/ijms14011455
60. Reddy AJ, Dubey AK, Handu SS, Sharma P, Mediratta PK, Ahmed QM, et al. Anticonvulsant and antioxidant effects of Musa sapientum stem extract on acute and chronic experimental models of epilepsy. Pharmacognosy Res. (2018) 10:49–54. doi: 10.4103/pr.pr_31_17
61. Zagaja M, Andres-Mach M, Skalicka-Wozniak K, Rekas AR, Kondrat-Wróbel MW, Gleńsk M, et al. Assessment of the combined treatment with umbelliferone and four classical antiepileptic drugs against maximal electroshock-induced seizures in mice. Pharmacology. (2015) 96:175–80. doi: 10.1159/000438704
62. Sarangi SC, Pattnaik SS, Joshi D, Chandra PP, Kaleekal T. Adjuvant role of Ocimum sanctum hydroalcoholic extract with carbamazepine and phenytoin in experimental model of acute seizures. Saudi Pharm J. (2020) 28:1440–50. doi: 10.1016/j.jsps.2020.09.010
63. Oliveira AA, Almeida JP, Freitas RM, Nascimento VS, Aguiar LM, Júnior HV, et al. Effects of levetiracetam in lipid peroxidation level, nitrite-nitrate formation and antioxidant enzymatic activity in mice brain after pilocarpine-induced seizures. Cell Mol Neurobiol. (2007) 27:395–406. doi: 10.1007/s10571-006-9132-y
64. Vasconcellos LRC, Martimiano L, Dantas DP, Fonseca FM, Mata-Santos H, Travassos L, et al. Intracerebral injection of heme induces lipid peroxidation, neuroinflammation, and sensorimotor deficits. Stroke. (2021) 52:1788–97. doi: 10.1161/STROKEAHA.120.031911
65. Olmos G, Lladó J. Tumor necrosis factor alpha: a link between neuroinflammation and excitotoxicity. Mediators Inflamm. (2014) 2014:861231. doi: 10.1155/2014/861231
66. Beltrán-Sarmiento E, Arregoitia-Sarabia CK, Floriano-Sánchez E, Sandoval-Pacheco R, Galván-Hernández DE, Coballase-Urrutia E, et al. Effects of valproate monotherapy on the oxidant-antioxidant status in mexican epileptic children: a longitudinal study. Oxid Med Cell Longev. (2018) 2018:7954371. doi: 10.1155/2018/7954371
67. Tejada S, Roca C, Sureda A, Rial RV, Gamundí A, Esteban S. Antioxidant response analysis in the brain after pilocarpine treatments. Brain Res Bull. (2006) 69:587–92. doi: 10.1016/j.brainresbull.2006.03.002
68. Ren S, Breuillaud L, Yao W, Yin T, Norris KA, Zehntner SP, et al. TNF-α-mediated reduction in inhibitory neurotransmission precedes sporadic Alzheimer's disease pathology in young Trem2R47H rats. J Biol Chem. (2021) 296:100089. doi: 10.1074/jbc.RA120.016395
69. Maroso M, Balosso S, Ravizza T, Iori V, Wright CI, French J, et al. Interleukin-1β biosynthesis inhibition reduces acute seizures and drug resistant chronic epileptic activity in mice. Neurotherapeutics. (2011) 8:304–15. doi: 10.1007/s13311-011-0039-z
70. Lu RB, Wang TY, Lee SY, Chen SL, Chang YH, See Chen P, et al. Correlation between interleukin-6 levels and methadone maintenance therapy outcomes. Drug Alcohol Depend. (2019) 204:107516. doi: 10.1016/j.drugalcdep.2019.06.018
71. Goel R, Goel A, Kumar Y. Influence of carvedilol on anticonvulsant effect of gabapentin. Acta Neurol Belg. (2011) 111:296–305.
72. Zagaja M, Bryda J, Szewczyk A, Szala-Rycaj J, Łuszczki JJ, Walczak M, et al. Xanthotoxin enhances the anticonvulsant potency of levetiracetam and valproate in the 6-Hz corneal stimulation model in mice. Fundam Clin Pharmacol. (2022) 36:133–42. doi: 10.1111/fcp.12713
73. Parsons ALM, Bucknor EMV, Castroflorio E, Soares TR, Oliver PL, Rial D. The interconnected mechanisms of oxidative stress and neuroinflammation in epilepsy. Antioxidants (Basel). (2022) 11:157. doi: 10.3390/antiox11010157
74. Dias JM, de Brito TV, de Aguiar Magalhães D, da Silva Santos PW, Batista JA., do Nascimento Dias EG, et al. Gabapentin, a synthetic analogue of gamma aminobutyric acid, reverses systemic acute inflammation and oxidative stress in mice. Inflammation. (2014) 37:1826–36. doi: 10.1007/s10753–014–9913–2
75. Fotio Y, Aboufares El Alaoui A, Borruto AM, Acciarini S, Giordano A, Ciccocioppo R. Efficacy of a combination of N-Palmitoylethanolamide, Beta-Caryophyllene, Carnosic Acid, and Myrrh extract on chronic neuropathic pain: a preclinical study. Front Pharmacol. (2019) 10:711. doi: 10.3389/fphar.2019.00711
76. Owens MJ, Nemeroff CB. Pharmacology of valproate. Psychopharmacol Bull. (2003) 37 (Suppl .2):17–24.
77. Ferriero DM. Protecting neurons. Epilepsia. (2005) 46(Suppl. 7):45–51. doi: 10.1111/j.1528–1167.2005.00302.x
78. Hsieh PF, Hou CW, Yao PW, Wu SP, Peng YF, Shen ML, et al. Sesamin ameliorates oxidative stress and mortality in kainic acid-induced status epilepticus by inhibition of MAPK and COX-2 activation. J Neuroinflammation. (2011) 8:57. doi: 10.1186/1742-2094-8-57
79. Taskiran AS, Ergul M. The modulator action of thiamine against pentylenetetrazole-induced seizures, apoptosis, nitric oxide, and oxidative stress in rats and SH-SY5Y neuronal cell line. Chem Biol Interact. (2021) 340:109447. doi: 10.1016/j.cbi.2021.109447
80. Shin EJ, Jeong JH, Chung YH, Kim WK, Ko KH, Bach JH, et al. Role of oxidative stress in epileptic seizures. Neurochem Int. (2011) 59:122–37. doi: 10.1016/j.neuint.2011.03.025
81. Deepa D, Jayakumari B, Thomas SV. Lipid peroxidation in women with epilepsy. Ann Indian Acad Neurol. (2008) 11:44–6. doi: 10.4103/0972-2327.40225
82. Das A, Sarwar MS, Hossain MS, Karmakar P, Islam MS, Hussain ME, et al. Elevated serum lipid peroxidation and reduced vitamin c and trace element concentrations are correlated with epilepsy. Clin EEG Neurosci. (2019) 50:63–72. doi: 10.1177/1550059418772755
83. Militão GC, Ferreira PM, de Freitas RM. Effects of lipoic acid on oxidative stress in rat striatum after pilocarpine-induced seizures. Neurochem Int. (2010) 56:16–20. doi: 10.1016/j.neuint.2009.08.009
84. Ercegovac M, Jovic N, Simic T, Beslac-Bumbasirevic L, Sokic D, Djukic T, et al. Byproducts of protein, lipid and DNA oxidative damage and antioxidant enzyme activities in seizure. Seizure. (2010) 19:205–10. doi: 10.1016/j.seizure.2010.02.002
85. Tchekalarova J, da Conceição Machado K, Gomes Júnior AL, de Carvalho Melo Cavalcante AA, Momchilova A, Tzoneva R. Pharmacological characterization of the cannabinoid receptor 2 agonist, β-caryophyllene on seizure models in mice. Seizure. (2018) 57:22–6. doi: 10.1016/j.seizure.2018.03.009
86. Li T-R, Jia Y-J, Wang Q, Shao X-Q, Zhang P, Lv R-J. Correlation between tumor necrosis factor alpha mRNA and microRNA-155 expression in rat models and patients with temporal lobe epilepsy. Brain Res. (2018) 1700:56–65. doi: 10.1016/j.brainres.2018.07.013
87. Breder CD, Tsujimoto M, Terano Y, Scott DW, Saper CB. Distribution and characterization of tumor necrosis factor-alpha-like immunoreactivity in the murine central nervous system. J Comp Neurol. (1993) 337:543–67. doi: 10.1002/cne.903370403
88. Riazi K, Galic MA, Kuzmiski JB, Ho W, Sharkey KA, Pittman QJ. Microglial activation and TNFα production mediate altered CNS excitability following peripheral inflammation. Proc Natl Acad Sci USA. (2008) 105:17151–6. doi: 10.1073/pnas.0806682105
89. Balosso S, Ravizza T, Perego C, Peschon J, Campbell IL, De Simoni MG, et al. Tumor necrosis factor-alpha inhibits seizures in mice via p75 receptors. Ann Neurol. (2005) 57:804–12. doi: 10.1002/ana.20480
90. Balosso S, Ravizza T, Aronica E, Vezzani A. The dual role of TNF-α and its receptors in seizures. Exp Neurol. (2013) 247:267–71. doi: 10.1016/j.expneurol.2013.05.010
91. Hahm ET, Kim Y, Lee JJ, Cho YW. GABAergic synaptic response and its opioidergic modulation in periaqueductal gray neurons of rats with neuropathic pain. BMC Neurosci. (2011) 12:41. doi: 10.1186/1471-2202-12-41
92. Jahani R, Khoramjouy M, Nasiri A, Sojoodi Moghaddam M, Asgharzadeh Salteh Y, Faizi M. Neuro-behavioral profile and toxicity of the essential oil of Dorema ammoniacum gum as an anti-seizure, anti-nociceptive, and hypnotic agent with memory-enhancing properties in D-galactose induced aging mice. Iran J Pharm Res. (2020) 19:110–21. doi: 10.22037/ijpr.2020.113738.14458
Keywords: methadone, valproate sodium, gabapentin, maximal electroshock seizures, malondialdehyde, tumor necrosis factor-alpha, mice
Citation: Moradi Jafari A and Hassanpourezatti M (2022) Influence of methadone on the anticonvulsant efficacy of valproate sodium gabapentin against maximal electroshock seizure in mice by regulation of brain MDA TNF-α. Front. Neurol. 13:920107. doi: 10.3389/fneur.2022.920107
Received: 14 April 2022; Accepted: 26 July 2022;
Published: 23 August 2022.
Edited by:
Fernando Cendes, State University of Campinas, BrazilReviewed by:
Victor Rodrigues Santos, Federal University of Minas Gerais, BrazilCameron S. Metcalf, The University of Utah, United States
Copyright © 2022 Moradi Jafari and Hassanpourezatti. This is an open-access article distributed under the terms of the Creative Commons Attribution License (CC BY). The use, distribution or reproduction in other forums is permitted, provided the original author(s) and the copyright owner(s) are credited and that the original publication in this journal is cited, in accordance with accepted academic practice. No use, distribution or reproduction is permitted which does not comply with these terms.
*Correspondence: Majid Hassanpourezatti, SGFzc2FucG91ciYjeDAwMDQwO3NoYWhlZC5hYy5pcg==; aGFzc2FucG91cmV6YXR0aSYjeDAwMDQwO2dtYWlsLmNvbQ==