- 1School of Medicine, University of California, Irvine, Irvine, CA, United States
- 2Department of Neurosciences, University of California, San Diego, La Jolla, CA, United States
- 3Department of Neurology, University of California, Irvine, Irvine, CA, United States
Intracranial artery calcifications (IAC), a common and easily identifiable finding on computed tomorgraphy angiography (CTA), has gained recognition as a possible risk factor for ischemic stroke. While atherosclerosis of intracranial arteries is believed to be a mechanism that commonly contributes to ischemic stroke, and coronary artery calcification is well-established as a predictor of both myocardial infarction (MI) and ischemic stroke risk, IAC is not currently used as a prognostic tool for stroke risk or recurrence. This review examines the pathophysiology and prevalence of IAC, and current evidence suggesting that IAC may be a useful tool for prediction of stroke incidence, recurrence, and response to acute ischemic stroke therapy.
Introduction
Intracranial artery calcifications (IAC) are a common finding on computed tomography (CT) or CT angiography. In recent years, IAC has been recognized as a possible risk factor for ischemic stroke (1–3). Arterial calcification generally occurs as a diffuse process involving the medial layer or as localized plaques involving the intimal layer, however, these patterns have not been well-characterized in the context of intracranial arteries (4). Intimal calcification is commonly observed on CTA and more frequently associated with plaque and luminal stenosis in intracranial arteries, suggesting a potential contribution to a heightened risk of ischemic stroke (5–7). Despite this potentially increased risk, currently, no widely accepted clinical or radiographic tools exist to investigate the influence of intracranial artery calcification on the risk of ischemic stroke. This review focuses on the current understanding of intracranial artery calcification, its implications as a risk factor for ischemic stroke as well as predictive tools for its stratification and management.
Methods
This is a narrative review of literature on intracranial calcifications and stroke. On March 2020, the National Library of Medicine's Pubmed database for searched with the following string: “intracranial” AND “calcification” AND “stroke”. Results were filtered by (1) English language, (2) availability of full text, (3) human study population, and (4) publication date within the last 20 years (i.e., from January 2000 onwards). The search yielded at total of 192 articles. Abstracts for all articles were independently reviewed by three different authors on the paper. Articles were selected for inclusion based on (1) relevance to the topic and (2) presentation of original clinical data. Articles that were irrelevant, had incomplete data or were theoretical/analytical without presentation of original clinical data were excluded. Articles that met inclusion criteria by any one reviewer, even if it did not by the other two reviewers, were still included in the analysis. It is of note that for the most part all three reviewers were in consensus as to the inclusion or exclusion of individual articles. A total of 26 articles were selected, and a comprehensive analysis is presented here. All research was conducted in accordance with the ethical and scientific guidelines put forth by the Institutional Review Board at the University of California Irvine.
Pathophysiology: Role of Structure and Function
Intracranial arteries have some important structural differences from their more well-studied coronary counterparts. These differences include a more dense internal elastic lamina, thinner media and adventitia with less elastic fibers, less vasa vasorum, and lack of an external elastic lamina in intracranial arteries. In a 2017 review, Yang et al. propose that the paucity of elastic fiber in intracranial arteries may constitute a protective feature, as elastic fibers are thought to be more prone to inflammation, fibroproliferative response, and atherosclerotic damage (4). On the other hand, it has also been suggested that a decreased expression of anti-inflammatory molecular mediators in the intracranial artery microenvironment may actually cause increased susceptibility to plaque instability and inflammatory changes in comparison to extracranial arteries (8). Thus, while a comprehensive comparison between intracranial and extracranial artery microstructure has yet to be conducted, it is likely that calcification and atherosclerosis look very different in intra- vs. extracranial settings.
Arterial Calcification Patterns
Calcification may occur in the muscular medial layer or the endothelium of the intimal layer of the artery wall (see Figure 1). Age, pulse pressure, and family history are risk factors for both types of calcifications, whereas smoking and hypertension are risk factors for intimal calcification and diabetes and chronic kidney disease are risk factors for medial calcification (9, 10). Intimal calcification is considered a marker of atherosclerosis. Atherosclerosis progresses from endothelial damage to the formation of fibrous plaques with a core of lipid, cholesterol, and cellular debris under a cap (11). For reasons that are still not well understood, atherosclerotic lesions become calcified via a molecular mechanism that is similar to bone formation (11). While some research findings have suggested that the process of calcification has a stabilizing effect on associated plaque, others suggest that this relative structural stability may trade off with a higher overall plaque burden, and potentially plaque instability, in heavily calcified arteries (12–19).
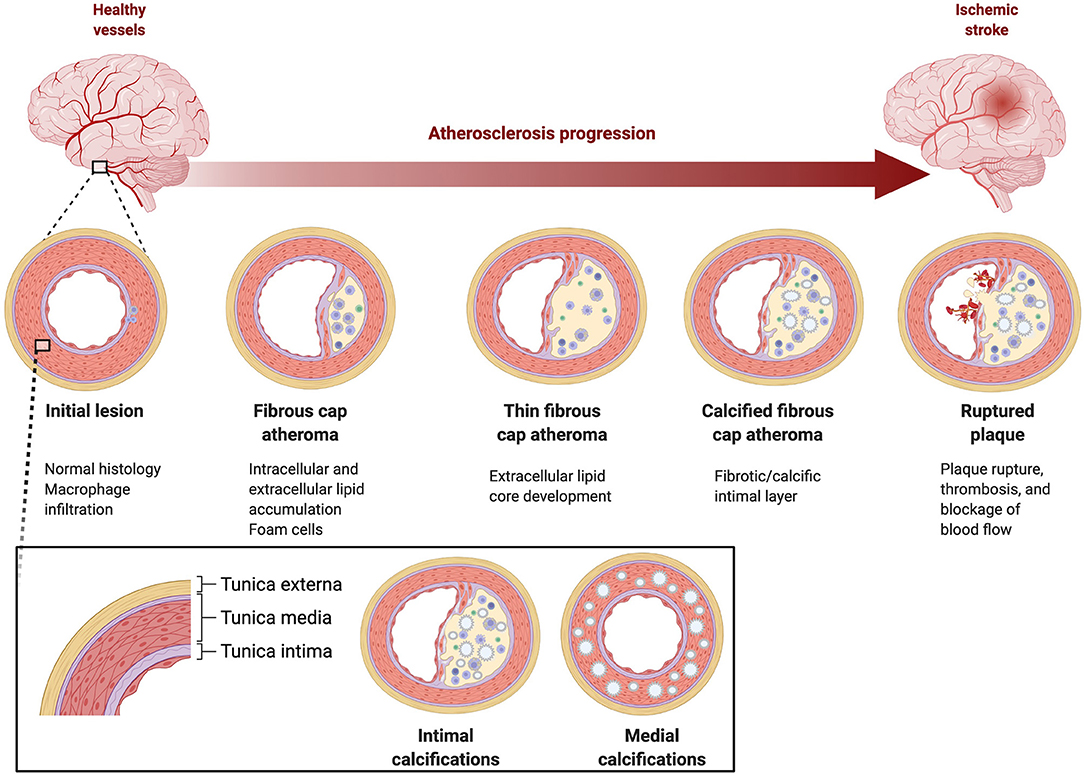
Figure 1. Pathophysiology of intracranial atherosclerosis. Large intracranial vessels are muscular arteries with three wall layers, tunica externa, tunica media, and tunica intima.
While atherosclerosis of the intima is presumed to contribute to stroke the clinical significance of medial calcification is unknown, thus, a challenge in scoring IAC lesions is differentiating between intimal and medial calcifications. Histological examination is still the most reliable way to differentiate between intimal and medial calcification, however a scoring system, Kockelkoren scoring, is based on CT imaging and incorporates circularity, thickness, and morphology to differentiate medial and intimal calcification in the intracranial internal carotid artery (20). Kockelkoren scoring has been used to evaluate differential effectiveness of stroke interventions (tPA or endovascular thrombectomy) (21, 22).
One cross-sectional study verified the validity of CT assessment of IAC by obtaining a CT scan prior to autopsy and histological evaluation (23). The calcification patterns seen on CT were correlated with the histological findings. Sixty seven percent of calcifications detected were in the intima, and the majority of calcification was observed in the vertebral artery (51%). All of the intimal calcifications were in progressive atherosclerotic lesions, and arteries with intimal calcification had more severe luminal stenosis than those without (23). In contrast, a large cross-sectional study of acute stroke patients by Vos et al. assessed localization of IAC (intimal vs. medial) by non-contrast CT (9), and found that dominant intimal calcification was present in 30.9% of subjects and dominant medial calcification in 46.9% of subjects, with no calcification in 10.5%. A histological study by Vos et al. found that calcification of the intracranial ICA was predominantly found in the internal elastic lamina, and was not related to the occurrence of intimal atherosclerotic lesions (24). The arterial wall layer where calcification depend on the specific intracranial artery being examined and the method by which calcification is assessed. Future studies are needed to determine whether the majority of vertebral artery calcifications are associated with atherosclerosis whereas the ICA calcifications are not, and whether this affects the risk of stroke for these vessels when other variables are controlled for.
Prevalence of IAC
Ethnicity and Prevalence of Intracranial Atherosclerosis
Among the limited available studies, some differences based on race and ethnicity have been observed. Although Japanese patients tend to have less atherosclerotic burden of the aorta, intracerebral atherosclerosis was more severe and occurred earlier in a study of Japanese patients than previously reported studies of Caucasians (25). Compared with studies of Caucasian and Japanese populations, Chinese patients have higher prevalence of cerebral atherosclerosis (26–28). In direct comparisons, Africans (29), African Americans (30), patients of African heritage in Europe (31), and a combined African American and Hispanic population (32) had more atherosclerotic lesions of intracranial arteries than Caucasians. Future studies directly comparing calcification of atherosclerotic lesions across populations of different ethnicities are needed.
Stroke patients have a high degree of IAC on imaging. Acute ischemic stroke patients in Nigeria and China were found to have high IAC burden (93 and 72% of subjects respectively) (29, 33). Calcification was mostly located in proximal inflow arteries in both studies.
Anatomical Distribution of IAC
The anatomical burden of IAC has been determined by a handful of X-Ray and CT studies to lie most prominently in the anterior circulation involving intracranial internal carotid arteries, followed by the vertebral arteries and the basilar artery, although there has been some variation across studies (Table 1) (23, 29, 33, 35, 37).
Ischemic Stroke Risk Prediction
Clinical Predictive Tools
The majority of strokes in the United States are ischemic (87%) and atherothrombotic disease is the leading cause of ischemic stroke (38). Modifiable risk factors contributing to development of atherothrombotic disease including hypertension, hypercholesterolemia, atrial fibrillation, tobacco use, diabetes mellitus, physical inactivity, and obstructive sleep apnea (33, 39, 40). The most commonly used clinical predictive tools are the atherosclerotic cardiovascular disease (ASCVD), the CHA2DS2-VASc score in the context of atrial fibrillation, and the ABCD2 score for stroke risk following TIA. Currently there are no widely used clinical tools or algorithms which incorporate atherosclerosis or IAC to predict occurrence of ischemic stroke. Most patients who undergo neuroimaging do so in the context of a suspected stroke or TIA. Therefore, the distinction of risk factors associated with IAC from those attributed to ischemic stroke may prove challenging. The major risk factors for IAC include age, male sex, hypertension, diabetes, hypercholesterolemia, ischemic stroke, and chronic kidney disease, all of which are also considered major risk factors for stroke (1, 29).
Scoring Methods for IAC and Ischemic Stroke Risk
The evaluation of IAC on head CT has largely followed principles established in the CAC literature, where coronary artery calcification (CAC) has been well-established as a predictor of both MI and ischemic stroke risk (39). Typically areas of high attenuation in arterial walls are interpreted as intra-arterial calcifications; in support of this methodology, a study by Denzel et al. showed good concordance between calcifications detected on CT and histologic analysis of carotid artery samples (41).
There are a variety of different techniques to calculate CAC, including both manual and automated techniques (42). The most widely used method is the Agatston method, whereby a specific set of serial transaxial cardiac CT images are read manually to generate a score based on the density and area of all coronary artery lesions identified throughout the series (43). CAC scoring by the traditional Agatston method has demonstrated consistent value in predicting cardiovascular events, including the ability to predict 10-year coronary heart disease risk with greater accuracy than diabetes, stroke, and other traditional risk factors (34, 44–59). Agatston scoring is currently used as a screen for clinically silent coronary ischemia (60). The Agatston scoring method was ubiquitous among the articles we reviewed (Table 2). However, the predictive value of this method for stroke outcomes is not clear: two of the included studies found no association of Agatston score with stroke risk, while three found some association with stroke incidence or recurrence. Limited emerging evidence suggests that new, alternative scoring methods may have increased predictive power for determining stroke outcomes over the Agatston method. The use of density and volume measurements, for example, showed improved prediction of CVD events in a subset of MESA (Multi-Ethnic Study of Atherosclerosis) participants when compared to traditional Agatston scoring (73). Modified methods similar to Agatston have been described with improved reproducibility (74).
Of the other scoring methods we encountered in our review, six studies used a simple binary yes/no to indicate whether calcification was observed. Six additional studies used semiquantitative methods involving categories with some degree of subjective interpretation. For example, in Power et al. (65), calicification of the carotid siphon was scored as Grade 0—absent, Grade 1—thin, discontinuous, Grade 2—thin, continuous or thick, discontinuous, and Grade 3—thick, continuous. While the subjectivity within each study may be addressed by using multiple image readers, these non-standardized methods of scoring render comparison across studies difficult.
Alternate imaging modalities such as ultrasound and MRI have also been explored (75). MRI, a more expensive imaging modality, has the additional benefit of more precisely characterizing different types of intravascular plaques (76). Although CT data is more widely available, the higher resolution afforded by MRI modalities may prove to have clinical importance in classifying plaque as being high or low risk, through the identification of intraplaque hemorrhage (IPH), a biomarker for future cerebrovascular events (15). One study found that the presence of IAC was associated with imaging markers of small vessel disease on MRI, suggesting that IAC could potentially be used as a marker for small vessel disease (33). Although preliminary studies have shown the feasibility of MRI in the accurate detection and quantification of intracranial calcification, to the best of our knowledge, currently there are no MRI-based scoring systems to quantify IAC (77, 78).
One challenge in scoring IAC lesions is differentiating between intimal and medial calcifications; while atherosclerosis of the intima is presumed to contribute to stroke the clinical significance of medial calcification is unknown. One promising new method, the Kockelkoren method, was developed specifically to differentiate intimal from medial calcification of the intracranial internal carotid artery. This method involves assigning points for circularity, thickness, and morphology (20), and was used in two of the studies we reviewed.
This method may be valuable for predicting both future stroke incidence and response to therapy, as intimal calcification is better correlated with progressive atherosclerotic lesions, and medial calcification may predict negative response to TPA but better outcomes after EVT. Although this method appears to provide clinically useful information about the histology of the lesion, it remains to be seen whether the Kockelkoren method can be used to reliably predict risk of future stroke. In summary, due to its prevalence in the clinical literature our group continues to actively use the Agatston scoring method, although we are following new literature validating the Kockelkoren method closely.
Association of IAC With Risk of Future Stroke
Several recent prospective studies have shown a significant association between IAC and stroke risk. Two studies that prospectively followed patients after stroke or TIA for multiple years found that IAC scores were associated with higher risk of recurrent TIA (69), stroke, post-stroke mortality, and small vessel occlusive disease (70). Similarly, a case-control study specifically examining vertebrobasilar artery calcification by CT found a higher risk of recurrent stroke and vascular events with higher calcification (79).
The value of IAC as a predictor of first-ever ischemic stroke was demonstrated in the Rotterdam study. Intracranial internal carotid artery calcification volume was shown to have similar or greater predictive power than extracranial artery atherosclerosis in the prediction of future stroke among 2,323 stroke-free subjects HR 1.43 [95% CI, 1.04-1.96]) (3).
One concern is the possibility that atherosclerosis may be a confounding factor that is independently associated with both IAC (1, 80, 81) and ischemic stroke (82). The SAMMPRIS trial (Stenting vs. Aggressive Medical Therapy of Intracranial Arterial Stenosis) found that, among stenotic arteries, calcified stenosis was actually associated with a decreased likelihood of recurrent ischemic stroke compared to non-calcified stenosis (83). In fact, it has been suggested that larger calcifications might contribute to plaque stability (84). Thus, it is critical that studies seeking to establish an association between IAC and stroke account for the influence of atherosclerosis.
One way of isolating the association between calcification and stroke from confounding risk factors is using the artery contralateral to the stroke in the same patient as a control. Two studies found a higher degree of calcification ipsilateral to stroke-associated lesions. One study found that there was significantly greater calcification in the ICA ipsilateral to infarction only for stroke of undetermined etiology, not cardioembolic. In the other study, microembolic signals were more prevalent ipsilateral to internal carotid artery calcification in the high-calcium group (42, 68).
Another possible application of IAC scores may lie in the prediction of cerebrovascular response to injury. Hussein et al. found a significant association between patients with extraordinarily high calcification scores and lower rates of vasospasm after aneurysmal subarachnoid hemorrhage, suggesting a possible protective effect of arterial calcification in the context of acute brain bleeds (66).
It is important to note that the association between calcification and stroke has not gone undisputed–Taoka and colleagues 2006 found that while calcium score was positively correlated with arteriosclerotic changes in the carotid siphon and carotid bifurcation, there was no correlation between the degree of calcium and the ability to predict future strokes within a 2 year time period (61). A more recent large prospective study found no association between internal carotid artery calcification and stroke. This study included 1,349 people followed over a median follow-up of 5.1 years (72). Some of this discrepancy may come from differences in the detection and quantification of IAC. Evaluation of IAC on head CT has largely followed principles established in the CAC literature, where areas of high attenuation in arterial walls are interpreted as intra-arterial calcifications. A study by Denzel et al. showed good concordance between calcifications detected on CT and histologic analysis of carotid artery samples (41). Nevertheless, there is still no universally agreed-upon cutoff value for identifying calcification in Hounsfield units, let alone a quantitative method for IAC scoring (1). It is therefore important to consider different IAC detection and scoring methods when interpreting the results of studies of IAC and ischemic stroke risk.
Taken together, this relatively small but promising group of results suggests that IAC captured on routine CT scan may hold value in predicting recurrent stroke risk, especially among individuals with multiple risk factors.
IAC as a Prognostic Marker for Acute Stroke Therapy
IAC may affect the outcome of therapeutic interventions for acute stroke, such as tPA or endovascular therapy (EVT). Three studies have examined the effect of IAC on tPA response. Gocmen et al. (22) conducted a retrospective study on 91 subjects who had acute anterior circulation strokes treated with IV tPA. Intracranial internal carotid artery calcification (IICAC) subtypes (medial vs. intimal) were compared to the response to IV tPA. In the study, IIIAC was diagnosed and classified according to Kockelkoren's methods. IV tPA was effective at 24 h in 48% of the subjects with no IICAC, 60% of the time in patients with intimal IICAC, and 43% of the time with medial IIAC. Medial IICAC was linked to negative early responses to IV TPA (p = 0.052) and increase of symptomatic intracerebral hemorrhage (21 vs. 4% intimal and no-IICAC p=0.052) (22). Similarly, in a prospective study of 982 patients, with acute ischemic stroke, IV tPA was associated with favorable clinical outcomes and recanalization in patients with medial but not intimal internal carotid artery calcification (71). Finally, Yu et al. (85) performed a retrospective study of 242 patients with acute non-cardiogenic stroke who received IV tPA and found that arterial calcification volume on the side of the lesion was associated with hemorrhagic transformation after thrombolysis. Additionally, the patients with poorer prognosis had more calcified vessels.
Compagne et al. (21) conducted a randomized prospective study on 344 subjects with acute ischemic strokes that were part of the MR CLEAN clinical trial of EVT. Intracranial internal carotid artery calcification from the horizontal part of the petrous segment to the circle of willis were measured using the Kockelkoren method. Patients with predominantly medial calcification had better functional outcome with EVT and there was no effect for EVT in patients with primarily intimal calcifications. However, there was no association between intracranial calcification volume and functional outcome (measured by 90 day modified Rankin scale) (21).
Therefore, the benefit of EVT or tPA in acute ischemic stroke patients is greater in patients with medial calcification or no calcification than in patients with intimal calcification. Compagne et al. offered the following possible explanations: either that EVT might cause greater risk of microemboli in patients with intimal calcification associated with atherosclerotic plaque, or that medial calcification may be associated with development of stronger collateral circulation pathways due to arterial stiffening.
Discussion
IAC research presents a promising new frontier in predicting ischemic stroke risk and outcomes. Given the ubiquity of CT imaging, the addition of IAC evaluation metrics to existing imaging data could potentially enhance the identification of patients at risk for first-ever or recurrent strokes which could ultimately guide management decisions to reduce these risks. However, given the uncertainties surrounding the pathologic correlation of IAC with cerebrovascular disease, further investigation is needed to characterize the direction and magnitude of these associations. Overall, the studies reviewed here suggests that IAC identified on CT imaging may help predict recurrent stroke risk. For those individuals at highest risk, consideration may eventually be warranted for screening CT or MRI, with recommendations analogous to those applied to CAC imaging in high cardiac risk patients. However, the SAMMPRIS trial and Taoka et al. found that calcification was not positively associated with risk of future stroke. These conflicting results necessitate the development of a standardized quantitative method for IAC measurement based on imaging data. Such a method should integrate the overall IAC density as well as the distribution of IAC across different vascular territories. One important asset in this regard could be the use of deep learning, which has been established as a promising tool for predicting final infarct lesions based on day 3 imaging data (86). Another direction for further research will be to examine the predictive value of IAC in ischemic stroke treatment outcomes after medical and/or endovascular therapy. Investigation of the role of IAC in treatment outcomes could lead to precision therapy. Finally, it is also possible that IAC may predict vascular cognitive decline and overall brain health (87).
Author contributions
GF, SR, KM, and AC are responsible for idea generation, literature search, data collection, and creating the first version of the manuscript. MS and WY are responsible for idea generation and review of the manuscript. All authors contributed to the article and approved the submitted version.
Conflict of Interest
The authors declare that the research was conducted in the absence of any commercial or financial relationships that could be construed as a potential conflict of interest.
Publisher's Note
All claims expressed in this article are solely those of the authors and do not necessarily represent those of their affiliated organizations, or those of the publisher, the editors and the reviewers. Any product that may be evaluated in this article, or claim that may be made by its manufacturer, is not guaranteed or endorsed by the publisher.
Acknowledgments
We wish to acknowledge the University of California, Irvine Department of Neurology.
References
1. Wu XH, Chen XY, Wang LJ, Wong KS. Intracranial artery calcification and its clinical significance. J Clin Neurol. (2016) 12:253–61. doi: 10.3988/jcn.2016.12.3.253
2. Chen XY, Lam WWM, Ng HK, Fan YH, Wong KS. Intracranial artery calcification: A newly identified risk factor of ischemic stroke. J Neuroimaging. (2007) 17:300–3. doi: 10.1111/j.1552-6569.2007.00158.x
3. Bos D, Portegies MLP, Van Der Lugt A, Bos MJ, Koudstaal PJ, Hofman A, et al. Intracranial carotid artery atherosclerosis and the risk of stroke in whites: the Rotterdam study. JAMA Neurol. (2014) 71:405–11. doi: 10.1001/jamaneurol.2013.6223
4. Yang WJ, Wong KS, Chen XY. Intracranial atherosclerosis: from microscopy to high-resolution magnetic resonance imaging. J stroke. (2017) 19:249–62. doi: 10.5853/jos.2016.01956
5. Sohn YH, Cheon HY, Jeon P, Kang SY. Clinical implication of cerebral artery calcification on brain CT. Circulation. (2004) 18:332–7. doi: 10.1159/000080772
6. Woodcock RJ, Goldstein JH, Kallmes DF, Cloft HJ, Phillips CD. Angiographic correlation of CT calcification in the carotid siphon. Am J Neuroradiol. (1999) 20:495–9.
7. Kassab MY, Gupta R, Majid A, Farooq MU, Giles BP, Johnson MD, et al. Extent of intra-arterial calcification on head CT is predictive of the degree of intracranial atherosclerosis on digital subtraction angiography. Cerebrovasc Dis. (2009) 28:45–8. doi: 10.1159/000219296
8. Qureshi AI, Caplan LR. Intracranial atherosclerosis. Lancet. (2014) 383:984–98. doi: 10.1016/S0140-6736(13)61088-0
9. Vos A, Kockelkoren R, de Vis JB, van der Schouw YT, van der Schaaf IC, Velthuis BK, et al. Risk factors for atherosclerotic and medial arterial calcification of the intracranial internal carotid artery. Atherosclerosis. (2018) 276:44–9. doi: 10.1016/j.atherosclerosis.2018.07.008
10. Yang WJ, Wasserman BA, Zheng L, Huang ZQ, Li J, Abrigo J, et al. Understanding the clinical implications of intracranial arterial calcification using brain ct and vessel wall imaging. Front Neurol. (2021) 12:1207. doi: 10.3389/fneur.2021.619233
11. Berliner J, Navab M, Fogelman A, Frank J, Demer L, Edwards P, et al. Atherosclerosis basic mechanisms oxidation, inflammation, and genetics. Circulation. (1995) 91:2488–96. doi: 10.1161/01.CIR.91.9.2488
12. Vengrenyuk Y, Carlier S, Xanthos S, Cardoso L, Ganatos P, Virmnani R, et al. hypothesis for vulnerable plaque rupture due to stress-induced debonding around cellular microcalcifications in thin fibrous caps. Proc Natl Acad Sci U S A. (2006) 103:14678–83. doi: 10.1073/pnas.0606310103
13. Van Den Bouwhuijsen QJA, Bos D, Ikram MA, Hofman A, Krestin GP, Franco OH, et al. Coexistence of calcification, intraplaque hemorrhage and lipid core within the asymptomatic atherosclerotic carotid plaque: The Rotterdam study. Cerebrovasc Dis. (2015) 39:319–24. doi: 10.1159/000381138
14. Teng Z, He J, Sadat U, Mercer JR, Wang X, Bahaei NS, et al. How does juxtaluminal calcium affect critical mechanical conditions in carotid atherosclerotic plaque? An exploratory study. IEEE Trans Biomed Eng. (2014) 61:35–40. doi: 10.1109/TBME.2013.2275078
15. Yang J, Pan X, Zhang B, Yan Y, Huang Y, Woolf AK, et al. Superficial and multiple calcifications and ulceration associate with intraplaque hemorrhage in the carotid atherosclerotic plaque. Eur Radiol. (2018) 28:4968–77. doi: 10.1007/s00330-018-5535-7
16. Liang M. The vulnerable plaque: the real villain in acute coronary syndromes. Open Cardiovasc Med J. (2011) 5:123–9. doi: 10.2174/1874192401105010123
17. Kwee RM. Systematic review on the association between calcification in carotid plaques and clinical ischemic symptoms. J Vasc Surg. (2010) 51:1015–25. doi: 10.1016/j.jvs.2009.08.072
18. Wahlgren CM, Zheng W, Shaalan W, Tang J, Bassiouny HS. Human carotid plaque calcification and vulnerability: relationship between degree of plaque calcification, fibrous cap inflammatory gene expression and symptomatology. Cerebrovasc Dis. (2009) 27:193–200. doi: 10.1159/000189204
19. Huang H, Virmani R, Younis H, Burke AP, Kamm RD, Lee RT. The impact of calcification on the biomechanical stability of atherosclerotic plaques. Circulation. (2001) 103:1051–6. doi: 10.1161/01.CIR.103.8.1051
20. Kockelkoren R, Vos A, Van HW, Vink A, Bleys RLAW, Verdoorn D, et al. Computed tomographic distinction of intimal and medial calcification in the intracranial internal carotid artery. PLoS ONE. (2017) 12. doi: 10.1371/journal.pone.0168360
21. Compagne K, Clephas P, Majoie C, Roos Y, Berkhemer O, Oostenbrugge R, et al. Intracranial carotid artery calcification and effect of endovascular stroke treatment. Stroke. (2018) 49:2961–8. doi: 10.1161/STROKEAHA.118.022400
22. Gocmen R, Arsava EM, Oguz KK, Topcuoglu MA. Atherosclerotic intracranial internal carotid artery calcification and intravenous thrombolytic therapy for acute ischemic stroke. Atherosclerosis. (2018) 270:89–94. doi: 10.1016/j.atherosclerosis.2018.01.035
23. Yang WJ, Zheng L, Wu XH, Huang ZQ, Niu CB, Zhao HL, et al. Postmortem study exploring distribution and patterns of intracranial artery calcification. Stroke. (2018) 49:2767–9. doi: 10.1161/STROKEAHA.118.022591
24. Vos A, Van Hecke W, Spliet WGM, Goldschmeding R, Isgum I, Kockelkoren R, et al. Predominance of nonatherosclerotic internal elastic lamina calcification in the intracranial internal carotid artery. Stroke. (2016) 47:221–3. doi: 10.1161/STROKEAHA.115.011196
25. Nakamura M, Yamamoto H, Kikuchi Y, Ishihara Y, Sata T, Yoshimura S. Cerebral atherosclerosis in Japanese. Stroke. (1971) 2:400–8. doi: 10.1161/01.STR.2.4.400
26. Chen XY, Wong KS, Lam WWM, Zhao HL, Ng HK. Middle cerebral artery atherosclerosis: Histological comparison between plaques associated with and not associated with infarct in a postmortem study. Cerebrovasc Dis. (2008) 25:74–80. doi: 10.1159/000111525
27. Denswil NP, van der Wal AC, Ritz K, de Boer OJ, Aronica E, Troost D, et al. Atherosclerosis in the circle of Willis: spatial differences in composition and in distribution of plaques. Atherosclerosis. (2016) 251:78–84. doi: 10.1016/j.atherosclerosis.2016.05.047
28. Leung SY, Ng THK, Yuen ST, Lauder IJ, Ho FCS. Pattern of cerebral atherosclerosis in Hong Kong Chinese. Severity in intracranial and extracranial vessels. Stroke. (1993) 24:779–86. doi: 10.1161/01.STR.24.6.779
29. Olatunji RB, Adekanmi AJ, Ogunseyinde AO. Intracranial arterial calcification in black africans with acute ischaemic stroke. Cerebrovasc Dis Extra. (2018) 8:26–38. doi: 10.1159/000485195
30. McGarry P, Solberg L, Guzman M, Strong J. Cerebral atherosclerosis in New Orleans. Comparisons of lesions by age, sex, and race. Lab Invest. (1985) 52:533–9.
31. Solberg L, McGarry P. Cerebral atherosclerosis in Negroes and Caucasians. Atherosclerosis. (1972) 16:141–54. doi: 10.1016/0021-9150(72)90048-2
32. Sacco RL, Kargman DE, Gu Q, Zamanillo Q. Race-ethnicity and determinants of intracranial atherosclerotic cerebral infarction. The Northern Manhattan Stroke Study. Stroke. (1995) 26:14–20. doi: 10.1161/01.STR.26.1.14
33. Chen YC, Wei XE, Lu J, Qiao RH, Shen XF, Li YH. Correlation between intracranial arterial calcification and imaging of cerebral small vessel disease. Front Neurol. (2019) 10. doi: 10.3389/fneur.2019.00426
34. Yang PS, Pak HN, Park DH, Yoo J, Kim TH, Uhm JS, et al. Non-cardioembolic risk factors in atrial fibrillation-associated ischemic stroke. PLoS ONE. (2018) 13. doi: 10.1371/journal.pone.0201062
35. Chen XY, Lam WWM, Ho KN, Fan YH, Wong KS. The frequency and determinants of calcification in intracranial arteries in Chinese patients who underwent computed tomography examinations. Cerebrovasc Dis. (2006) 21:91–7. doi: 10.1159/000090206
36. Bugnicourt JM, Chillon JM, Massy ZA, Canaple S, Lamy C, Deramond H, et al. High prevalence of intracranial artery calcification in stroke patients with CKD: a retrospective study. Clin J Am Soc Nephrol. (2009) 4:284–90. doi: 10.2215/CJN.02140508
37. Boström K, Hassler O. Radiological study of arterial calcification. Neurology. (1965) 15:1168–1168. doi: 10.1212/WNL.15.12.1168
38. Rothlisberger JM, Ovbiagele B. Antiplatelet therapies for secondary stroke prevention: An update on clinical and cost-effectiveness. J Comp Eff Res. (2015) 4:377–84. doi: 10.2217/cer.15.22
39. Benjamin EJ, Muntner P, Alonso A, Bittencourt MS, Callaway CW, Carson AP, et al. Heart Disease and Stroke Statistics-2019 Update: A Report From the American Heart Association. (2019). 56–528 p. doi: 10.1161/CIR.0000000000000659
40. Hill VA, Towfighi A. Modifiable risk factors for stroke and strategies for stroke prevention. Semin Neurol. (2017) 37:237–58. doi: 10.1055/s-0037-1603685
41. Denzel C, Lell M, Maak M, Höcki M, Balzer K, Müller KM, et al. Carotid artery calcium: Accuracy of a calcium score by computed tomography—an in vitro study with comparison to sonography and histology. Eur J Vasc Endovasc Surg. (2004) 28:214–20. doi: 10.1016/j.ejvs.2004.05.004
42. Wu XH, Chen XY, Fan YH, Leung TWH, Wong KS. High extent of intracranial carotid artery calcification is associated with downstream microemboli in stroke patients. J Stroke Cerebrovasc Dis. (2017) 26:442–7. doi: 10.1016/j.jstrokecerebrovasdis.2016.10.007
43. Agatston AS, Janowitz WR, Hildner FJ, Zusmer NR, Viamonte M, Detrano R. Quantification of coronary artery calcium using ultrafast computed tomography. J Am Coll Cardiol. (1990) 15:827–32. doi: 10.1016/0735-1097(90)90282-T
44. Detrano R, Guerci AD, Carr JJ, Bild DE, Burke G, Folsom AR, et al. Coronary calcium as a predictor of coronary events in four racial or ethnic groups. N Engl J Med. (2008) 358:1336–45. doi: 10.1056/NEJMoa072100
45. Erbel R, Mhlenkamp S, Moebus S, Schmermund A, Lehmann N, Stang A, et al. Coronary risk stratification, discrimination, and reclassification improvement based on quantification of Subclinical coronary atherosclerosis: The Heinz Nixdorf Recall study. J Am Coll Cardiol. (2010) 56:1397–406. doi: 10.1016/j.jacc.2010.06.030
46. Erbel R, Lehmann N, Möhlenkamp S, Churzidse S, Bauer M, Kälsch H, et al. Subclinical coronary atherosclerosis predicts cardiovascular risk in different stages of hypertension: Result of the heinz nixdorf recall study. Hypertension. (2012) 59:44–53. doi: 10.1161/HYPERTENSIONAHA.111.180489
47. McClelland RL, Jorgensen NW, Budoff M, Blaha MJ, Post WS, Kronmal RA, et al. 10-year coronary heart disease risk prediction using coronary artery calcium and traditional risk factors derivation in the MESA (Multi-Ethnic Study of Atherosclerosis) with validation in the HNR (Heinz Nixdorf Recall) Study and the DHS (Dallas Heart Stud. J Am Coll Cardiol. (2015) 66:1643–53. doi: 10.1016/j.jacc.2015.08.035
48. Khera A, Budoff MJ, O'Donnell CJ, Ayers CA, Locke J, De Lemos JA, et al. Astronaut cardiovascular health and risk modification (Astro-CHARM) coronary calcium atherosclerotic cardiovascular disease risk calculator. Circulation. (2018) 138:1819–27. doi: 10.1161/CIRCULATIONAHA.118.033505
49. Hecht HS. Coronary artery calcium scanning: past, present, and future. JACC Cardiovasc Imaging. (2015) 8:579–96. doi: 10.1016/j.jcmg.2015.02.006
50. Paixao ARM, Berry JD, Neeland IJ, Ayers CR, Rohatgi A, De Lemos JA, et al. Coronary artery calcification and family history of myocardial infarction in the dallas heart study. JACC Cardiovasc Imaging. (2014) 7:679–86. doi: 10.1016/j.jcmg.2014.04.004
51. Polonsky TS, McClelland RL, Jorgensen NW, Bild DE, Burke GL, Guerci AD, et al. Coronary artery calcium score and risk classification for coronary heart disease prediction. JAMA - J Am Med Assoc. (2010) 303:1610–6. doi: 10.1001/jama.2010.461
52. Yeboah J, McClelland RL, Polonsky TS, Burke GL, Sibley CT, O'Leary D, et al. Comparison of novel risk markers for improvement in cardiovascular risk assessment in intermediate-risk individuals. JAMA - J Am Med Assoc. (2012) 308:788–95. doi: 10.1001/jama.2012.9624
53. Jain A, McClelland RL, Polak JF, Shea S, Burke GL, Bild DE, et al. Cardiovascular imaging for assessing cardiovascular risk in asymptomatic men versus women the Multi-Ethnic Study of Atherosclerosis (MESA). Circ Cardiovasc Imaging. (2011) 4:8–15. doi: 10.1161/CIRCIMAGING.110.959403
54. Simon A, Megnien JL, Chironi G. The value of carotid intima-media thickness for predicting cardiovascular risk. Arterioscler Thromb Vasc Biol. (2010) 30:182–5. doi: 10.1161/ATVBAHA.109.196980
55. Peters SAE, Bakker M, Den Ruijter HM, Bots ML. Added value of CAC in risk stratification for cardiovascular events: a systematic review. Eur J Clin Invest. (2012) 42:110–6. doi: 10.1111/j.1365-2362.2011.02555.x
56. Peters SAE, Den Ruijter HM, Bots ML, Moons KGM. Improvements in risk stratification for the occurrence of cardiovascular disease by imaging subclinical atherosclerosis: A systematic review. Heart. (2012) 98:177–84. doi: 10.1136/heartjnl-2011-300747
57. Möhlenkamp S, Lehmann N, Moebus S, Schmermund A, Dragano N, Stang A, et al. Quantification of coronary atherosclerosis and inflammation to predict coronary events and all-cause mortality. J Am Coll Cardiol. (2011) 57:1455–64. doi: 10.1016/j.jacc.2010.10.043
58. Flueckiger P, Longstreth W, Herrington D, Yeboah J. Revised framingham stroke risk score, nontraditional risk markers, and incident stroke in a multiethnic cohort. Stroke. (2018) 49:363–9. doi: 10.1161/STROKEAHA.117.018928
59. Budoff MJ, Nasir K, McClelland RL, Detrano R, Wong N, Blumenthal RS, et al. Coronary calcium predicts events better with absolute calcium scores than age-sex-race/ethnicity percentiles: MESA (Multi-Ethnic Study of Atherosclerosis). J Am Coll Cardiol. (2009) 53:345–52. doi: 10.1016/j.jacc.2008.07.072
60. Greenland P, Alpert JS, Beller GA, Benjamin EJ, Budoff MJ, Fayad ZA, et al. 2010 ACCF/AHA guideline for assessment of cardiovascular risk in asymptomatic adults: Executive summary: a report of the American College of cardiology foundation/American Heart association task force on practice guidelines. Circulation. (2010) 122:2748–64. doi: 10.1161/CIR.0b013e3182051bab
61. Taoka T, Iwasaki S, Nakagawa H, Sakamoto M, Fukusumi A, Takayama K, et al. Evaluation of arteriosclerotic changes in the intracranial carotid artery using the calcium score obtained on plain cranial computed tomography scan: Correlation with angiographic changes and clinical outcome. J Comput Assist Tomogr. (2006) 30:624–8. doi: 10.1097/00004728-200607000-00012
62. Erbay S, Han R, Baccei S, Krakov W, Zou KH, Bhadelia R, et al. Intracranial carotid artery calcification on head CT and its association with ischemic changes on brain MRI in patients presenting with stroke-like symptoms: retrospective analysis. Neuroradiology. (2007) 49:27–33. doi: 10.1007/s00234-006-0159-z
63. de Weert TT, Cakir H, Rozie S, Cretier S, Meijering E, Dippel DW, et al. Intracranial internal carotid artery calcifications: association with vascular risk factors and ischemic cerebrovascular disease. AJNR Am J Neuroradiol. (2009) 30:177–84. doi: 10.3174/ajnr.A1301
64. Bugnicourt JM, Leclercq C, Chillon JM, Diouf M, Deramond H, Canaple S, et al. Presence of intracranial artery calcification is associated with mortality and vascular events in patients with ischemic stroke after hospital discharge: a cohort study. Stroke. (2011) 42:3447–53. doi: 10.1161/STROKEAHA.111.618652
65. Power A, Chan K, Haydar A, Hamady M, Cairns T, Taube D, et al. Intracranial arterial calcification is highly prevalent in hemodialysis patients but does not associate with acute ischemic stroke. Hemodial Int. (2011) 15:256–63. doi: 10.1111/j.1542-4758.2011.00543.x
66. Hussein HM, Zacharatos H, Cordina S, Lakshminarayan K, Ezzeddine MA. Intracranial vascular calcification is protective from vasospasm after aneurysmal subarachnoid hemorrhage. J Stroke Cerebrovasc Dis. (2014) 23:2687–93. doi: 10.1016/j.jstrokecerebrovasdis.2014.06.013
67. Lee JG, Lee KB, Roh H, Ahn MY, Bae HJ, Lee JS, et al. Intracranial arterial calcification can predict early vascular events after acute ischemic stroke. J Stroke Cerebrovasc Dis. (2014) 23:e331–7. doi: 10.1016/j.jstrokecerebrovasdis.2013.12.022
68. Kamel H, Gialdini G, Baradaran H, Giambrone AE, Navi BB, Lerario MP, et al. Cryptogenic stroke and nonstenosing intracranial calcified atherosclerosis. J Stroke Cerebrovasc Dis. (2017) 26:863–70. doi: 10.1016/j.jstrokecerebrovasdis.2016.10.035
69. Kong WY, Tan BY, Ellis ES, Ngiam NJ, Goh WG, Sharma VK, et al. Intracranial artery calcium burden predicts recurrent cerebrovascular events in transient ischaemic attack patients. J Stroke Cerebrovasc Dis. (2019) 28:2332–6. doi: 10.1016/j.jstrokecerebrovasdis.2019.05.027
70. Wu X, Bos D, Ren L, Leung TW, Chu WCW, Wong LKS, et al. Intracranial arterial calcification relates to long-term risk of recurrent stroke and post-stroke mortality. Front Neurol. (2020) 11. doi: 10.3389/fneur.2020.559158
71. Kauw F, de Jong PA, Takx RAP, de Jong HWAM, Kappelle LJ, Velthuis BK, et al. Effect of intravenous thrombolysis in stroke depends on pattern of intracranial internal carotid artery calcification. Atherosclerosis. (2021) 316:8–14. doi: 10.1016/j.atherosclerosis.2020.11.019
72. Bos D, Arshi B, van den Bouwhuijsen QJA, Ikram MK, Selwaness M, Vernooij MW, et al. Atherosclerotic carotid plaque composition and incident stroke and coronary events. J Am Coll Cardiol. (2021) 77:1426–35. doi: 10.1016/j.jacc.2021.01.038
73. Criqui MH, Knox JB, Denenberg JO, Forbang NI, McClelland RL, Novotny TE, et al. Coronary artery calcium volume and density: potential interactions and overall predictive value: the multi-ethnic study of atherosclerosis. JACC Cardiovasc Imaging. (2017) 10:845–54. doi: 10.1016/j.jcmg.2017.04.018
74. Callister TQ, Cooil B, Raya SP, Lippolis NJ, Russo DJ, Raggi P. Coronary artery disease: Improved reproducibility of calcium scoring with an electron-beam CT volumetric method. Radiology. (1998) 208:807–14. doi: 10.1148/radiology.208.3.9722864
75. Banchhor SK, Londhe ND, Araki T, Saba L, Radeva P, Khanna NN, et al. Calcium detection, its quantification, and grayscale morphology-based risk stratification using machine learning in multimodality big data coronary and carotid scans: a review. Comput Biol Med. (2018) 101:184–98. doi: 10.1016/j.compbiomed.2018.08.017
76. Xu X, Ju H, Cai J, Cai Y, Wang X, Wang Q. High-resolution MR study of the relationship between superficial calcification and the stability of carotid atherosclerotic plaque. Int J Cardiovasc Imaging. (2010) 26:143–50. doi: 10.1007/s10554-009-9578-3
77. Harteveld AA, Denswil NP, Siero JCW, Zwanenburg JJM, Vink A, Pouran B, et al. Quantitative intracranial atherosclerotic plaque characterization at 7T MRI: an ex vivo study with histologic validation. Am J Neuroradiol. (2016) 37:802–10. doi: 10.3174/ajnr.A4628
78. Turan TN, Rumboldt Z, Granholm AC, Columbo L, Welsh CT, Lopes-Virella MF, et al. Intracranial atherosclerosis: correlation between in-vivo 3T high resolution MRI and pathology. Atherosclerosis. (2014) 237:460–3. doi: 10.1016/j.atherosclerosis.2014.10.007
79. Magdič J, Cmor N, Kaube M, Fabjan TH, Hauer L, Sellner J, et al. Intracranial vertebrobasilar calcification in patients with ischemic stroke is a predictor of recurrent stroke, vascular disease, and death: a case-control study. Int J Environ Res Public Health. (2020) 17:2013. doi: 10.3390/ijerph17062013
80. Park KY, Kim YB, Moon HS, Suh BC, Chung PW. Association between cerebral arterial calcification and brachial-ankle pulse wave velocity in patients with acute ischemic stroke. Eur Neurol. (2009) 61:364–70. doi: 10.1159/000210549
81. Shapiro SD, Goldman J, Morgello S, Honig L, Elkind MSV, Marshall RS, et al. Pathological correlates of brain arterial calcifications. Cardiovasc Pathol. (2019) 38:7–13. doi: 10.1016/j.carpath.2018.09.003
82. Banerjee C, Chimowitz MI. Stroke caused by atherosclerosis of the major intracranial arteries. Circ Res. (2017) 120:502–13. doi: 10.1161/CIRCRESAHA.116.308441
83. Quiney B, Ying SM, Hippe DS, Balu N, Urdaneta-Moncada AR, Mossa-Basha M. The association of intracranial vascular calcification and stenosis with acute ischemic cerebrovascular events. J Comput Assist Tomogr. (2017) 41:849–53. doi: 10.1097/RCT.0000000000000629
84. Pugliese G, Iacobini C, Fantauzzi CB, Menini S. The dark and bright side of atherosclerotic calcification. Atherosclerosis. (2015) 238:220–30. doi: 10.1016/j.atherosclerosis.2014.12.011
85. Yu Y, Zhang FL, Qu YM, Zhang P, Zhou HW, Luo Y, et al. Intracranial calcification is predictive for hemorrhagic transformation and prognosis after intravenous thrombolysis in non-cardioembolic stroke patients. J Atheroscler Thromb. (2021) 28:356–64. doi: 10.5551/jat.55889
86. Yu Y, Xie Y, Thamm T, Gong E, Ouyang J, Huang C, et al. Use of deep learning to predict final ischemic stroke lesions from initial magnetic resonance imaging. JAMA Netw Open. (2020) 3:1–13. doi: 10.1001/jamanetworkopen.2020.0772
Keywords: stroke, calcification, atherosclerosis, imaging, biomarker
Citation: Fote GM, Raefsky S, Mock K, Chaudhari A, Shafie M and Yu W (2022) Intracranial Arterial Calcifications: Potential Biomarkers of Stroke Risk and Outcome. Front. Neurol. 13:900579. doi: 10.3389/fneur.2022.900579
Received: 20 March 2022; Accepted: 24 June 2022;
Published: 01 September 2022.
Edited by:
Gary Kui Kai Lau, The University of Hong Kong, Hong Kong SAR, ChinaReviewed by:
Wenjie Yang, University of Maryland, United StatesSlaven Pikija, University Hospital Salzburg, Austria
Xiangyan Chen, Hong Kong Polytechnic University, Hong Kong SAR, China
Copyright © 2022 Fote, Raefsky, Mock, Chaudhari, Shafie and Yu. This is an open-access article distributed under the terms of the Creative Commons Attribution License (CC BY). The use, distribution or reproduction in other forums is permitted, provided the original author(s) and the copyright owner(s) are credited and that the original publication in this journal is cited, in accordance with accepted academic practice. No use, distribution or reproduction is permitted which does not comply with these terms.
*Correspondence: Amit Chaudhari, YW1pdGNoYXVkaGFyaW1kQGdtYWlsLmNvbQ==