- 1Department of Neurology, General Hospital of Northern Theatre Command, Shenyang, China
- 2Department of Neurology, Haicheng Hospital of Traditional Chinese Medicine, Haicheng, China
- 3Department of Neurology, Chinese People's Liberation Army 321 Hospital, Baicheng, China
- 4Department of Neurology, Jiamusi University First Affiliated Hospital, Jiamusi, China
- 5Department of Neurology, The Second Affiliated Hospital of Harbin Medical University, Harbin, China
Background: Symptomatic intracranial hemorrhage (sICH) is a terrible complication after intravenous alteplase in stroke, and numerous biomarkers have been investigated. However, the change of biomarkers to sICH has not been well determined.
Aim: To investigate the association between the change of biomarkers and sICH.
Methods: This is a prospective cohort study, and patients with sICH within 24 h after thrombolysis were enrolled, while patients without sICH were matched by propensity score matching with a ratio of 1:1. The blood samples were collected before and 24 h after intravenous thrombolysis (IVT), and preset 49 serum biomarkers were measured by microarray analysis. Protein function enrichment analyses were performed to detect the association between the change of biomarkers and sICH.
Results: Of consecutive 358 patients, 7 patients with sICH in 24 h were assigned to the sICH group, while 7 matched patients without any ICH were assigned to the non-sICH group. A total of 9 biomarkers were found to significantly change before vs. after thrombolysis between groups, including increased biomarkers, such as brain-derived neurotrophic factor, C-C motif chemokine ligand (CCL)-24, interleukin (IL)-6, IL-10, IL-18, and vascular endothelial growth factor, and decreased biomarkers, such as CCL-11, intercellular adhesion molecule-1, and IL-7.
Conclusions: This is the first study to identify changes in serum biomarkers in patients with sICH after IVT, and found that 6 neuroinflammatory and 3 neuroprotective biomarkers may be associated with brain injury following post-thrombolytic sICH.
Clinical Trial Registration: https://www.clinicaltrials.gov, identifier: NCT02854592.
Introduction
Intravenous alteplase is the main effective treatment for acute ischemic stroke (AIS) within 4.5 h after the onset of symptoms (1). Symptomatic intracranial hemorrhage (sICH) is a rare but severe complication after thrombolysis that is closely related to disability and death (2).
Mountains of studies have investigated predictors for post-thrombolytic sICH in stroke, such as clinical, radiological, and laboratory factors (3–5). Although several biomarkers were found to be associated with post-thrombolytic hemorrhagic transformation (6–11), these biomarkers were only detected at admission. To date, however, only one study investigated the change of serum biomarkers after post-thrombolytic sICH (12). Given that changes in biomarkers before and after sICH maybe reflect the secondary brain injury of sICH, the issue needed to be further investigated.
In the INtravenous Thrombolysis REgistry for Chinese Ischemic Stroke within 4.5 h of onset (INTRECIS) (13), 5 centers were pre-designed to consecutively collect blood samples before and 24 h after thrombolysis. In this study, we tried to identify what serum biomarkers change significantly before thrombolysis vs. after sICH, compared with patients without any ICH and investigated the potential interactions by microarray analysis on 49 preset biomarkers.
Methods
Study Population and Procedure
From August 2018 to July 2019, patients receiving intravenous thrombolysis (IVT) within 4.5 h after symptoms onset were consecutively screened to collect blood samples before thrombolysis at five pre-set stroke centers in the INTRECIS study. The INTRECIS is a prospective, nationwide, multi-center, and registry study of consecutive adult patients who were eligible for treatment with IVT within 4.5 h of the onset of symptoms, and has been published recently (13). Patients who received a standard dose of alteplase (0.9 mg/kg, maximum 90 mg; manufacturer: Boehringer Ingelheim) within 4.5 h after symptoms onset were included in the study. The exclusion criteria were as follows: (1) patients received urokinase or non-standard dose of alteplase, (2) patients received endovascular intervention, (3) patients lacked complete clinical data, and (4) blood samples were not collected before or 24 h after IVT. According to the occurrence of sICH 24 h after IVT, enrolled patients were divided into two groups: (1) sICH group: patients who had sICH within 24 h after IVT; (2) non-sICH group: patients who did not have any ICH in 24 h after IVT. Furthermore, as described in our previous study (14), propensity score matching was performed between groups with a ratio of 1:1, the caliper of 0.1, and a nearest-neighbor matching strategy, and operated with control factors, such as age, current smoking, alcohol consumption, gender, systolic blood pressure, diastolic blood pressure, symptom onset-to-treatment time, blood glucose, National Institutes of Health Stroke Scale (NIHSS) score at admission, previous use of antiplatelet, and medical history, and the Trial of Org 10172 in Acute Stroke Treatment (TOAST) classification.
The baseline characteristics and clinical data of recruited patients were obtained from the electronic database: age, current smoking, alcohol consumption, gender, systolic blood pressure, diastolic blood pressure, symptom onset-to-treatment time, blood glucose, National Institutes of Health Stroke Scale (NIHSS) score at admission, previous use of antiplatelet, and medical history, and the Trial of Org 10172 in Acute Stroke Treatment (TOAST) classification. All patients underwent a CT scan at admission and 24 h (or when neurological deterioration occurred within 24 h) after IVT, to evaluate the presence of ICH. According to European Cooperative Acute Stroke Study (ECASS)-II study, sICH was defined as an increase of ≥4 on the NIHSS scores caused by ICH within 36 h (15).
Laboratory Determinations
The methods of laboratory determinations were described in our previous study (14). Briefly, 4 ml of venous blood were collected for measurement before and 24 h after IVT, respectively. The blood samples were centrifuged at 4°C and 1,000 g for 10 min, and then transferred into a cryotube and stored at −80°C. The customized protein microarray analysis from Raybiotech Inc. was used to simultaneously quantify 49 biomarkers in the above blood samples, which were preset based on published data.
Statistical Analysis
Descriptive statistics were performed to compare variables between the two groups. Continuous variables with normal distribution were described as means and standard deviation (SD). Continuous variables included age, systolic blood pressure, diastolic blood pressure, blood glucose, NIHSS score, symptom onset to thrombolysis time, and detected circulating concentration of biomarkers. The t-tests were used to analyze continuous variables with normal distribution. Additionally, categorical variables were described as numbers and proportions. Categorical variables included gender, medical history, and TOAST classification. The Pearson χ2 tests were used to analyze categorical variables.
The empirical Bayes-based linear model method was used to analyze the differential expression of biomarkers between different stages of disease with the R package limma. The differential expression was evaluated by adjusting the value of p (BH method) based on moderated t statistics. In all analyses, differences were considered statistically significant with a p < 0.05. The free statistical language R (version 3.10.3) was used for the outcomes and graph in microarray analysis.
Results
As shown in Figure 1, 358 patients with thrombolysis were consecutively screened in the present study, and 234 patients were excluded for different reasons: 160 patients received urokinase or the non-standard dose of alteplase, 10 patients received the endovascular intervention, 3 patients with incomplete clinical data, and 61 patients without blood samples collection. Finally, 124 patients were recruited into the current study, including 2 patients with ICH after 24 h, 4 patients with asymptomatic ICH in 24 h, 7 patients with sICH in 24 h, and 111 patients without any ICH in 24 h after IVT. There was no significant difference in the baseline characteristics between included patients and excluded patients (Table 1). With a ratio of 1:1, 7 patients without any ICH and 7 patients with sICH were matched to the non-sICH group and sICH group for final analysis (Figure 1). There was no significant difference in the baseline characteristics between the two groups (Table 2).
Changes in Biomarkers After sICH
Compared with the non-sICH group, significant changes of 9 biomarkers before and after sICH were observed in the sICH group (p < 0.05), including a significant increase in brain-derived neurotrophic factor (BDNF), C-C motif chemokine ligand (CCL)-24, interleukin (IL)-6, IL-10, IL-18, and vascular endothelial growth factor (VEGF), and a significant decrease in IL-7, intercellular adhesion molecule (ICAM)-1, and CCL-11 (Table 3). The scatter and volcano plot showed results of all measured biomarkers (Figures 2A,B). The column plot and heatmap showed the results of the identified biomarkers (Figures 2C,D).
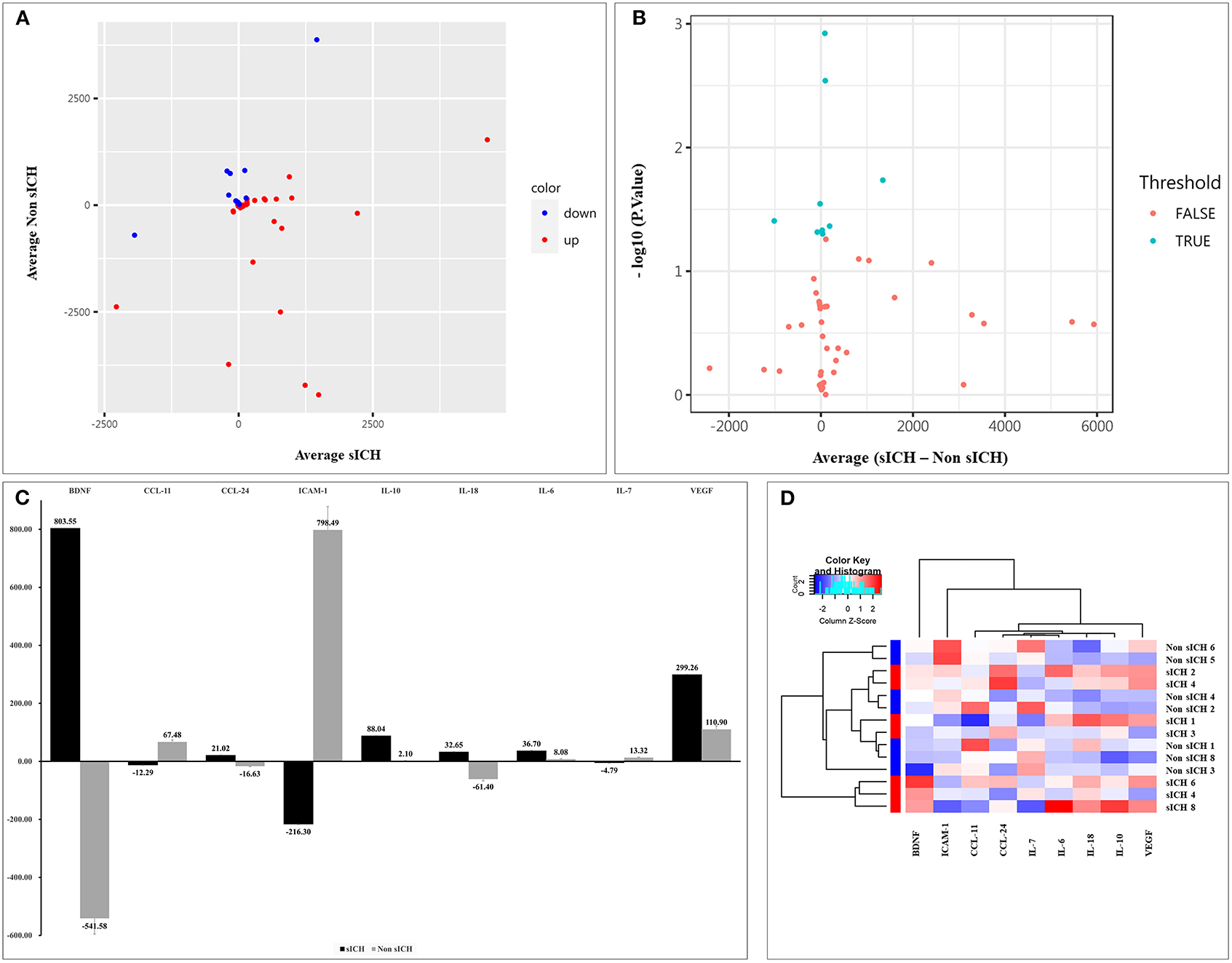
Figure 2. Results of detected biomarkers in the microarray analysis. (A) The scatter plot for detected biomarkers; the X-axis represents the average of serum levels in the sICH group, while the Y-axis represents the average of serum levels in the non-sICH group; compared with the non-sICH group, the blue point represents biomarkers with decrease in the sICH group, while the red point represents biomarkers with increase. sICH, symptomatic intracranial hemorrhage. (B) The volcano plot for detected biomarkers; the X-axis represents the average of change in serum levels between two groups while the Y-axis represents the –log10 p; the cyan point represents the biomarkers with a significant difference, while the red point represents the biomarkers without significant difference. sICH, symptomatic intracranial hemorrhage. (C) The column plot for identified biomarkers; the X-axis represents the identified biomarkers, while the Y-axis represents the average of serum levels in two groups; the deep color represents the sICH group, while the light color represents the non-sICH group. (D) The heatmap for identified biomarkers; the red color represents biomarkers with an increase, while the blue color represents the biomarkers with decrease; the darker the color, the more significant the difference of biomarkers. BDNF, brain-derived neurotrophic factor; CCL-11, C-C motif chemokine ligand (CCL)-11; CCL-24, C-C motif chemokine ligand (CCL)-24; ICAM-1, intercellular adhesion molecule-1; IL-10, interleukin-10; IL-18, interleukin-18; IL-6, interleukin-6; IL-7, interleukin-7; VEGF, vascular endothelial growth factor; and sICH, symptomatic intracranial hemorrhage.
Function Enrichment and Protein–Protein Interaction Network Analysis
A comprehensive gene ontology (GO) enrichment analysis was used to gain a deeper insight into the main functions of the identified biomarkers. The GO analysis consisted of biological processes, molecular functions, and cellular component analysis. The biological process analysis showed that ICAM-1, IL-6, IL-7, IL-10, IL-18, and VEGF were mostly included in the positive regulation of cell adhesion (Figure 3A). The molecular function analysis showed that CCL-11, CCL-24, IL-6, IL-7, IL-10, IL-18, and VEGF, were mostly included in the cytokine activity (Figure 3B). The cellular component analysis showed that IL-7, ICAM-A, and VEGF, were mostly included in the extracellular matrix (Figure 3C). The Kyoto Encyclopedia of Genes and Genomes (KEGG) enrichment analysis showed that CCL-11, CCL-24, IL-6, IL-7, IL-10, and IL-18, were included in cytokine–cytokine receptor interaction (Figure 3D).
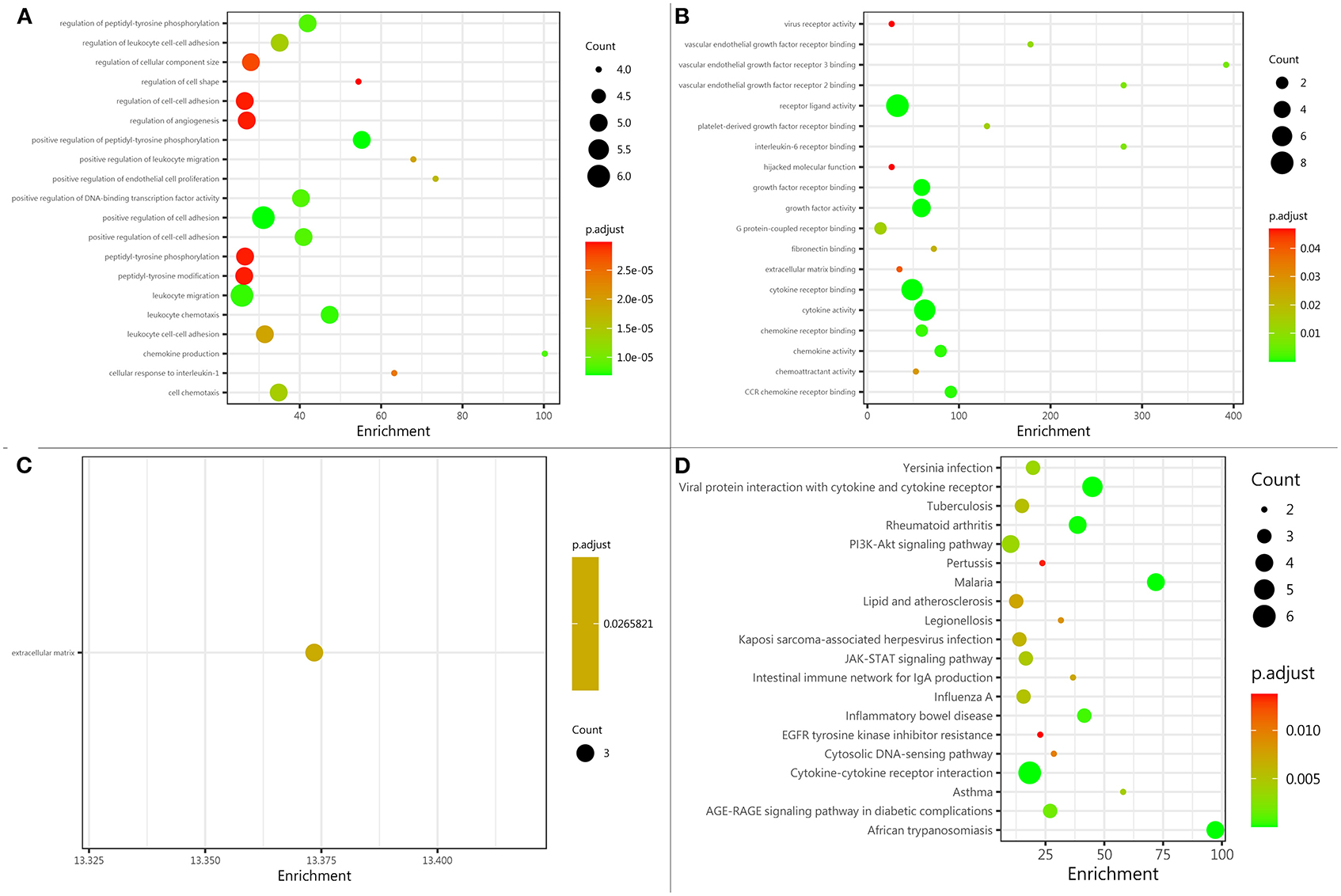
Figure 3. Protein function analysis of identified biomarkers. (A) Top 20 significantly enriched biological processes of identified biomarkers; the X-axis represents the enrichment, while the Y-axis represents the biological process. (B) The molecular function enriched by identified biomarkers; the X-axis represents the enrichment, while the Y-axis represents the molecular function. (C) The cellular component enriched by identified biomarkers; the X-axis represents the enrichment, while the Y-axis represents the cellular component. (D) The pathway enriched by identified biomarkers; the X-axis represents the enrichment, while the Y-axis represents the pathway. The deeper the color, the larger the value of p; the larger the circle, the bigger the counts.
Based on the information from the Search Tool for the Retrieval of Interacting Genes (STRING) database, the protein–protein interaction network constructed by the above 9 identified biomarkers was obtained (Figure 4). The results showed that IL-10 and IL-6 (degree = 8) could interact with the most biomarkers, followed by IL-18, IL-7, and VEGF (degree = 7), ICAM-1 and CCL-11 (degree = 6), BDNF (degree = 4), and CCL-24 (degree = 3).
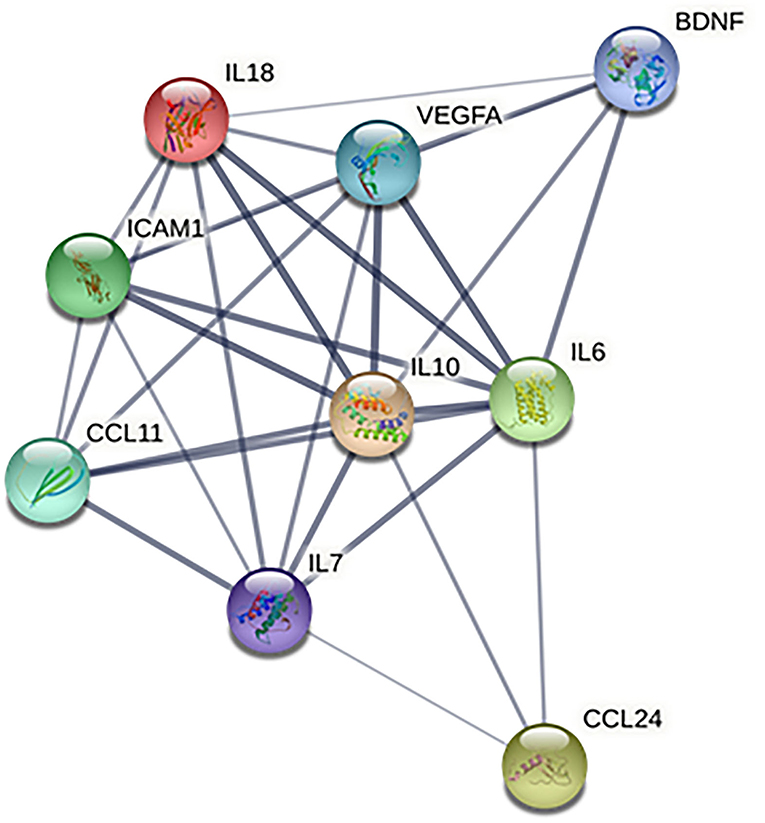
Figure 4. Protein–protein interaction network of identified biomarkers. BDNF, brain-derived neurotrophic factor; CCL-11, C-C motif chemokine ligand (CCL)-11; CCL-24, C-C motif chemokine ligand (CCL)-24; ICAM-1, intercellular adhesion molecule-1; IL-10, interleukin-10; IL-18, interleukin-18; IL-6, interleukin-6; IL-7, interleukin-7; and VEGF, vascular endothelial growth factor.
Discussion
To the best of our knowledge, this is the first report that identified significant changes in 9 serum biomarkers in a sICH group compared with those in a non-sICH group, including increased biomarkers, such as BDNF, CCL-24, IL-6, IL-10, IL-18, and VEGF, and decreased biomarkers, such as CCL-11, ICAM-1, and IL-7.
There have been a lot of studies to investigate the association of biomarkers with post-thrombolytic hemorrhagic transformation in stroke (6–11). However, these studies have not investigated changes in serum biomarkers before and after sICH in thrombolytic stroke. Our results showed that these significantly changed biomarkers mainly contributed to the inflammatory response. As a result of recruited leukocytes and released proinflammatory cytokines, inflammation contributed to brain injury following ICH (16). Our results indicate that neuroinflammatory responses play an important role in post-thrombolytic sICH.
In the present study, we found significantly decreased circulating levels of IL-7, ICAM-1, and CCL-11 in the sICH patients, compared with non-sICH patients, which is consistent with previous studies (17–19). After stroke injury, peripheral leukocytes migrate into injured brain tissue through adhesion molecules and chemokines (20). As an adhesive protein, ICAM-1 facilitates leukocyte adhesion to vascular endothelium and entry into brain tissue through the blood-brain barrier (21–24). The CCL-11 is a proinflammatory cytokine and induces chemotaxis of leukocytes into the injured brain region (24). IL-7 mainly activates recruited T lymphocytes and further produces proinflammatory factors (25–27). As ICAM-1, CCL-11, and IL-7 were widely involved in transmigration and activation of leukocytes, we hypothesized that a decrease in the three biomarkers could be due to their significant consumption of biomarkers by migrating into injured brain tissue along with leucocytes after sICH. Additionally, tPA was reported to induce a surge of circulating immune cells and mobilize peripheral neutrophils and T cells (28), which may also be involved in the neuroinflammatory response.
Our study also found that CCL-24, IL-6, and IL-18 significantly increased after sICH, compared with patients with non-sICH. These biomarkers act as proinflammatory factors and express in the recruited leukocytes and activated glia. For example, CCL-24 was found to act directly on chemokine receptor CCR-3 to recruit leukocytes and play a role in mediating the neuroinflammation set off by ischemic-reperfusion injury (29, 30). IL-6 was produced in activated microglia and caused the brain injury after intracerebral hemorrhage under inflamed conditions (31, 32). IL-18, produced by activated macrophages and involved in neuroinflammation (33), was correlated with poor outcomes in intracerebral hemorrhage (34). However, as shown in Table 2, the concentration of IL-6 significantly increased after IVT in both the sICH and non-sICH groups, which was not consistent with the other two biomarkers. These results suggested that IL-6 may be triggered to secrete by ischemic or hemorrhagic brain injury, while CCL-24 and IL-18 were specifically related to sICH.
In addition, BDNF, IL-10, and VEGF were significantly increased after sICH, which was consistent with previous studies (35–37). BDNF, secreted from microglia, was found to be involved in the endogenous neuroprotection after brain injury (35, 38–40). As an anti-inflammatory cytokine, IL-10 is secreted mainly by the lymphocytes and monocytes and it inhibits transcription and production of the proinflammatory factor IL-6 (41, 42), which was reported to be associated with better neurological prognosis (43). Additionally, VEGF was an important angiogenesis regulatory factor and mainly enhanced vascular permeability and promoted endothelial cell proliferation after ICH (44). As shown in Table 2, the concentrations of IL-10 and VEGF increased in two groups, which was not consistent with BDNF. These results suggest that as a self-defending mechanism, the secretion of IL-10 and VEGF may be over-released from the glia and leukocytes in response to ischemic or hemorrhagic brain injury, while BDNF may be specifically triggered by hemorrhagic insult.
Unexpectedly, we did not detect significant changes in other preset biomarkers, such as MMP-9 and ferritin, which were previously reported to be associated with post-thrombolytic hemorrhagic transformation in ischemic stroke (6). The negative results may be attributed to the following reason. Most previous studies mainly detected the levels of biomarkers at admission (45, 46), while the present study focused on investigating the change from admission to 24 h later.
Based on functional enrichment analysis, cell adhesion positive regulation, cytokine activity, extracellular matrix, and cytokine–cytokine receptor interaction were significantly enriched items. After a stroke, adhesion molecules (e.g., ICAM-1) mediated several leukocytes and cytokines (e.g., VEGF and IL-7) entered into the brain through the injured blood-brain barrier (20, 22). The chemokines (e.g., CCL-11 and CCL-24) recruited leukocytes to mediate activation and release of proinflammatory cytokines (e.g., IL-6 and IL-18) (47). In addition, the cytokine–cytokine receptor interaction between CCL-24 and CCR-3 mediated the recruitment of leukocytes (29). Therefore, we inferred that cell adhesion regulation, cytokine activity, and cytokine–cytokine receptor interaction may contribute to brain injury after ICH through mediating recruitment of leukocytes and releasing of proinflammatory cytokines.
We acknowledge that our study has several limitations. First, it is a small-sample study with only 7 AIS patients with post-thrombolytic sICH in 24 h and collecting blood samples. Additionally, the study only collected blood samples before and 24 h after IVT. A large cohort study with samples collected at more time points (e.g., 2, 6, and 12 h after IVT) is warranted. Second, only serum biomarkers were measured in the present study. Given the neuroinflammation-related or neuroprotection-related biomarkers, it may provide valuable information if these biomarkers are measured in the brain such as CSF. Third, the sensitivity and specificity of identified biomarkers for predicting sICH need to be validated in the future. Fourth, the change of biomarkers in patients with different etiologies should be investigated in the future. Finally, although baseline data were matched through propensity scores matching, some potential biases may be inevitable. For example, there may exist an imbalance in the baseline circulating levels of biomarkers.
Conclusion
For the first time, the present study prospectively investigated the changes of preset 49 serum biomarkers in patients with ischemic stroke receiving thrombolysis and found that 6 neuroinflammatory and 3 neuroprotective biomarkers were involved in the brain injury following post-thrombolytic sICH. The role of these biomarkers warrants further investigation given the potential therapeutic implications.
Data Availability Statement
The raw data supporting the conclusions of this article will be made available by the authors, without undue reservation.
Ethics Statement
The studies involving human participants were reviewed and approved by Institution Human Research Ethics Committees of General Hospital of Northern Theater Command. The patients/participants provided their written informed consent to participate in this study.
Author Contributions
H-SC designed and reviewed. YC conducted the analyses and drafted the manuscript. X-HW, YZ, S-YC, B-YS, and L-HW contributed to the implementation of blood sample collection. All authors contributed to the article and approved the submitted version.
Funding
The study was funded by grants from the National Key R&D Program of China (2017YFC1308203) and the Project on Research and Application of Effective Intervention Techniques for Chinese Stroke Guidelines from the National Health and Family Planning Commission in China (GN-2016R0008).
Conflict of Interest
The authors declare that the research was conducted in the absence of any commercial or financial relationships that could be construed as a potential conflict of interest.
Publisher's Note
All claims expressed in this article are solely those of the authors and do not necessarily represent those of their affiliated organizations, or those of the publisher, the editors and the reviewers. Any product that may be evaluated in this article, or claim that may be made by its manufacturer, is not guaranteed or endorsed by the publisher.
Acknowledgments
We thank all participating hospitals, relevant clinicians, and statisticians. We also thank all patients who participated in the present study.
References
1. Hacke W, Kaste M, Bluhmki E, Brozman M, Dávalos A, Guidetti D, et al. Thrombolysis with alteplase 3 to 4.5 hours after acute ischemic stroke. N Engl J Med. (2008) 359:1317–29. doi: 10.1056/NEJMoa0804656
2. Wardlaw JM, Murray V, Berge E, del Zoppo GJ. Thrombolysis for acute ischaemic stroke. Cochrane Database Syst Rev. (2014) 2014:CD000213. doi: 10.1002/14651858.CD000213.pub3
3. Uyttenboogaart M, Koch MW, Koopman K, Vroomen PC, De Keyser J, Luijckx GJ. Safety of antiplatelet therapy prior to intravenous thrombolysis in acute ischemic stroke. Arch Neurol. (2008) 65:607–11. doi: 10.1001/archneur.65.5.noc70077
4. Mundiyanapurath S, Hees K, Ahmed N, Wahlgren N, Uhlmann L, Kieser M, et al. Predictors of symptomatic intracranial haemorrhage in off-label thrombolysis: an analysis of the safe implementation of treatments in stroke registry. Eur J Neurol. (2018) 25:340–e11. doi: 10.1111/ene.13507
5. Larrue V, von Kummer R R, Müller A, Bluhmki E. Risk factors for severe hemorrhagic transformation in ischemic stroke patients treated with recombinant tissue plasminogen activator: a secondary analysis of the European-Australasian Acute Stroke Study (ECASS II). Stroke. (2001) 32:438–41. doi: 10.1161/01.STR.32.2.438
6. Wang W, Li M, Chen Q, Wang J. Hemorrhagic transformation after tissue plasminogen activator reperfusion therapy for ischemic stroke: mechanisms, models, and biomarkers. Mol Neurobiol. (2015) 52:1572–9. doi: 10.1007/s12035-014-8952-x
7. Maïer B, Desilles JP, Mazighi M. Intracranial hemorrhage after reperfusion therapies in acute ischemic stroke patients. Front Neurol. (2020) 11:599908. doi: 10.3389/fneur.2020.599908
8. Ouk T, Potey C, Maestrini I, Petrault M, Mendyk AM, Leys D, et al. Neutrophils in tPA-induced hemorrhagic transformations: main culprit, accomplice or innocent bystander? Pharmacol Ther. (2019) 194:73–83. doi: 10.1016/j.pharmthera.2018.09.005
9. Tanne D, Kasner SE, Demchuk AM, Koren-Morag N, Hanson S, Grond M, et al. Markers of increased risk of intracerebral hemorrhage after intravenous recombinant tissue plasminogen activator therapy for acute ischemic stroke in clinical practice: the multicenter rt-PA stroke survey. Circulation. (2002) 105:1679–85. doi: 10.1161/01.CIR.0000012747.53592.6A
10. Hsu PJ, Chen CH, Yeh SJ, Tsai LK, Tang SC, Jeng JS. High plasma D-dimer indicates unfavorable outcome of acute ischemic stroke patients receiving intravenous thrombolysis. Cerebrovasc Dis. (2016) 42:117–21. doi: 10.1159/000445037
11. Cocho D, Borrell M, Martí-Fàbregas J, Montaner J, Castellanos M, Bravo Y, et al. Pretreatment hemostatic markers of symptomatic intracerebral hemorrhage in patients treated with tissue plasminogen activator. Stroke. (2006) 37:996–9. doi: 10.1161/01.STR.0000206461.71624.50
12. Guo Z, Yu S, Xiao L, Chen X, Ye R, Zheng P, et al. Dynamic change of neutrophil to lymphocyte ratio and hemorrhagic transformation after thrombolysis in stroke. J Neuroinflammation. (2016) 13:199. doi: 10.1186/s12974-016-0680-x
13. Wang X, Li X, Xu Y, Li R, Yang Q, Zhao Y, et al. Effectiveness of intravenous r-tPA versus UK for acute ischaemic stroke: a nationwide prospective Chinese registry study. Stroke Vasc Neurol. (2021) 6:603–609. doi: 10.1136/svn-2020-000640
14. Cui Y, Zhao Y, Chen SY, Sheng BY, Wang LH, Meng WH, et al. Association of serum biomarkers with post-thrombolytic symptomatic intracranial hemorrhage in stroke: a comprehensive protein microarray analysis from INTRECIS study. Front Neurol. (2022) 13:751912. doi: 10.3389/fneur.2022.751912
15. Hacke W, Kaste M, Fieschi C, von Kummer R, Davalos A, Meier D, et al. Randomised double-blind placebo-controlled trial of thrombolytic therapy with intravenous alteplase in acute ischaemic stroke (ECASS II). Lancet. (1998) 352:1245–51. doi: 10.1016/S0140-6736(98)08020-9
16. Okada T, Suzuki H, Travis ZD, Zhang JH. The stroke-induced blood-brain barrier disruption: current progress of inspection technique, mechanism, and therapeutic target. Curr Neuropharmacol. (2020) 18:1187–212. doi: 10.2174/1570159X18666200528143301
17. Gris T, Laplante P, Thebault P, Cayrol R, Najjar A, Joannette-Pilon B, et al. Innate immunity activation in the early brain injury period following subarachnoid hemorrhage. J Neuroinflammation. (2019) 16:253. doi: 10.1186/s12974-019-1629-7
18. Kraus J, Gerriets T, Leis S, Stolz E, Oschmann P, Heckmann JG. Time course of VCAM-1 and ICAM-1 in CSF in patients with basal ganglia haemorrhage. Acta Neurol Scand. (2007) 116:49–55. doi: 10.1111/j.1600-0404.2006.00790.x
19. Lieschke S, Zechmeister B, Haupt M, Zheng X, Jin F, Hein K, et al. CCL11 differentially affects post-stroke brain injury and neuroregeneration in mice depending on age. Cells. (2019) 9:66. doi: 10.3390/cells9010066
20. Yang C, Hawkins KE, Doré S, Candelario-Jalil E. Neuroinflammatory mechanisms of blood-brain barrier damage in ischemic stroke. Am J Physiol Cell Physiol. (2019) 316:C135–53. doi: 10.1152/ajpcell.00136.2018
21. Rothlein R, Dustin ML, Marlin SD, Springer TA. A human intercellular adhesion molecule (ICAM-1) distinct from LFA-1. J Immunol. (1986) 137:1270–4.
22. Springer TA. Leucocyte adhesion to cells. Scand J Immunol. (1990) 32:211–6. doi: 10.1111/j.1365-3083.1990.tb02912.x
23. Hafler DA, Weiner HL. MS: a CNS and systemic autoimmune disease. Immunol Today. (1989) 10:104–7. doi: 10.1016/0167-5699(89)90236-3
24. Cannella B, Cross AH, Raine CS. Upregulation and coexpression of adhesion molecules correlate with relapsing autoimmune demyelination in the central nervous system. J Exp Med. (1990) 172:1521–4. doi: 10.1084/jem.172.5.1521
25. Swainson L, Kinet S, Mongellaz C, Sourisseau M, Henriques T, Taylor N. IL-7-induced proliferation of recent thymic emigrants requires activation of the PI3K pathway. Blood. (2007) 109:1034–42. doi: 10.1182/blood-2006-06-027912
26. Lawson BR, Gonzalez-Quintial R, Eleftheriadis T, Farrar MA, Miller SD, Sauer K, et al. Interleukin-7 is required for CD4(+) T cell activation and autoimmune neuroinflammation. Clin Immunol. (2015) 161:260–9. doi: 10.1016/j.clim.2015.08.007
27. Swardfager W, Winer DA, Herrmann N, Winer S, Lanctôt KL. Interleukin-17 in post-stroke neurodegeneration. Neurosci Biobehav Rev. (2013) 37:436–47. doi: 10.1016/j.neubiorev.2013.01.021
28. Shi K, Zou M, Jia DM, Shi S, Yang X, Liu Q, et al. tPA mobilizes immune cells that exacerbate hemorrhagic transformation in stroke. Circ Res. (2021) 128:62–75. doi: 10.1161/CIRCRESAHA.120.317596
29. Huber AK, Giles DA, Segal BM, Irani DN. An emerging role for eotaxins in neurodegenerative disease. Clin Immunol. (2018) 189:29–33. doi: 10.1016/j.clim.2016.09.010
30. Haines BA, Mehta SL, Pratt SM, Warden CH, Li PA. Deletion of mitochondrial uncoupling protein-2 increases ischemic brain damage after transient focal ischemia by altering gene expression patterns and enhancing inflammatory cytokines. J Cereb Blood Flow Metab. (2010) 30:1825–33. doi: 10.1038/jcbfm.2010.52
31. Leasure AC, Kuohn LR, Vanent KN, Bevers MB, Kimberly WT, Steiner T, et al. Association of serum IL-6 (interleukin 6) with functional outcome after intracerebral hemorrhage. Stroke. (2021) 52:1733–40. doi: 10.1161/STROKEAHA.120.032888
32. Ren H, Kong Y, Liu Z, Zang D, Yang X, Wood K, et al. Selective NLRP3 (pyrin domain-containing protein 3) inflammasome inhibitor reduces brain injury after intracerebral hemorrhage. Stroke. (2018) 49:184–92. doi: 10.1161/STROKEAHA.117.018904
33. Feng L, Chen Y, Ding R, Fu Z, Yang S, Deng X, et al. P2X7R blockade prevents NLRP3 inflammasome activation and brain injury in a rat model of intracerebral hemorrhage: involvement of peroxynitrite. J Neuroinflammation. (2015) 12:190. doi: 10.1186/s12974-015-0409-2
34. Fang HY, Ko WJ, Lin CY. Inducible heat shock protein 70, interleukin-18, and tumor necrosis factor alpha correlate with outcomes in spontaneous intracerebral hemorrhage. J Clin Neurosci. (2007) 14:435–41. doi: 10.1016/j.jocn.2005.12.022
35. Lee WD, Wang KC, Tsai YF, Chou PC, Tsai LK, Chien CL. Subarachnoid hemorrhage promotes proliferation, differentiation, and migration of neural stem cells via BDNF upregulation. PLoS ONE. (2016) 11:e0165460. doi: 10.1371/journal.pone.0165460
36. Yang G, Shao GF. Elevated serum IL-11, TNF α, and VEGF expressions contribute to the pathophysiology of hypertensive intracerebral hemorrhage (HICH). Neurol Sci. (2016) 37:1253–9. doi: 10.1007/s10072-016-2576-z
37. Dziedzic T, Bartus S, Klimkowicz A, Motyl M, Slowik A, Szczudlik A. Intracerebral hemorrhage triggers interleukin-6 and interleukin-10 release in blood. Stroke. (2002) 33:2334–5. doi: 10.1161/01.STR.0000027211.73567.FA
38. Imamura N, Hida H, Aihara N, Ishida K, Kanda Y, Nishino H, et al. Neurodegeneration of substantia nigra accompanied with macrophage/microglia infiltration after intrastriatal hemorrhage. Neurosci Res. (2003) 46:289–98. doi: 10.1016/S0168-0102(03)00065-8
39. Miller FD, Kaplan DR. Neurotrophin signalling pathways regulating neuronal apoptosis. Cell Mol Life Sci. (2001) 58:1045–53. doi: 10.1007/PL00000919
40. Ploughman M, Windle V, MacLellan CL, White N, Doré JJ, Corbett D. Brain-derived neurotrophic factor contributes to recovery of skilled reaching after focal ischemia in rats. Stroke. (2009) 40:1490–5. doi: 10.1161/STROKEAHA.108.531806
41. Zhou K, Zhong Q, Wang YC, Xiong XY, Meng ZY, Zhao T, et al. Regulatory T cells ameliorate intracerebral hemorrhage-induced inflammatory injury by modulating microglia/macrophage polarization through the IL-10/GSK3β/PTEN axis. J Cereb Blood Flow Metab. (2017) 37:967–79. doi: 10.1177/0271678X16648712
42. Tedgui A, Mallat Z. Anti-inflammatory mechanisms in the vascular wall. Circ Res. (2001) 88:877–87. doi: 10.1161/hh0901.090440
43. Vila N, Castillo J, Dávalos A, Esteve A, Planas AM, Chamorro A. Levels of anti-inflammatory cytokines and neurological worsening in acute ischemic stroke. Stroke. (2003) 34:671–5. doi: 10.1161/01.STR.0000057976.53301.69
44. Chu H, Tang Y, Dong Q. Protection of vascular endothelial growth factor to brain edema following intracerebral hemorrhage and its involved mechanisms: effect of aquaporin-4. PLoS ONE. (2013) 8:e66051. doi: 10.1371/journal.pone.0066051
45. Zhang M, Li W, Wang T, Zhang Q. Association between baseline serum ferritin and short-term outcome of intracerebral hemorrhage: a meta-analysis. J Stroke Cerebrovasc Dis. (2019) 28:1799–805. doi: 10.1016/j.jstrokecerebrovasdis.2019.03.037
46. Montaner J, Molina CA, Monasterio J, Abilleira S, Arenillas JF, Ribó M, et al. Matrix metalloproteinase-9 pretreatment level predicts intracranial hemorrhagic complications after thrombolysis in human stroke. Circulation. (2003) 107:598–603. doi: 10.1161/01.CIR.0000046451.38849.90
47. Kitaura M, Nakajima T, Imai T, Harada S, Combadiere C, Tiffany HL, et al. Molecular cloning of human eotaxin, an eosinophil-selective CC chemokine, and identification of a specific eosinophil eotaxin receptor, CC chemokine receptor 3. J Biol Chem. (1996) 271:7725–30. doi: 10.1074/jbc.271.13.7725
Keywords: alteplase, intravenous thrombolysis, symptomatic intracranial hemorrhage, biomarkers, microarray analysis
Citation: Cui Y, Wang X-H, Zhao Y, Chen S-Y, Sheng B-Y, Wang L-H and Chen H-S (2022) Change of Serum Biomarkers to Post-Thrombolytic Symptomatic Intracranial Hemorrhage in Stroke. Front. Neurol. 13:889746. doi: 10.3389/fneur.2022.889746
Received: 04 March 2022; Accepted: 21 April 2022;
Published: 02 June 2022.
Edited by:
Jean-charles Sanchez, Université de Genève, SwitzerlandReviewed by:
Pedro J. Modrego, Hospital Universitario Miguel Servet, SpainBo Song, First Affiliated Hospital of Zhengzhou University, China
Copyright © 2022 Cui, Wang, Zhao, Chen, Sheng, Wang and Chen. This is an open-access article distributed under the terms of the Creative Commons Attribution License (CC BY). The use, distribution or reproduction in other forums is permitted, provided the original author(s) and the copyright owner(s) are credited and that the original publication in this journal is cited, in accordance with accepted academic practice. No use, distribution or reproduction is permitted which does not comply with these terms.
*Correspondence: Hui-Sheng Chen, chszh@aliyun.com