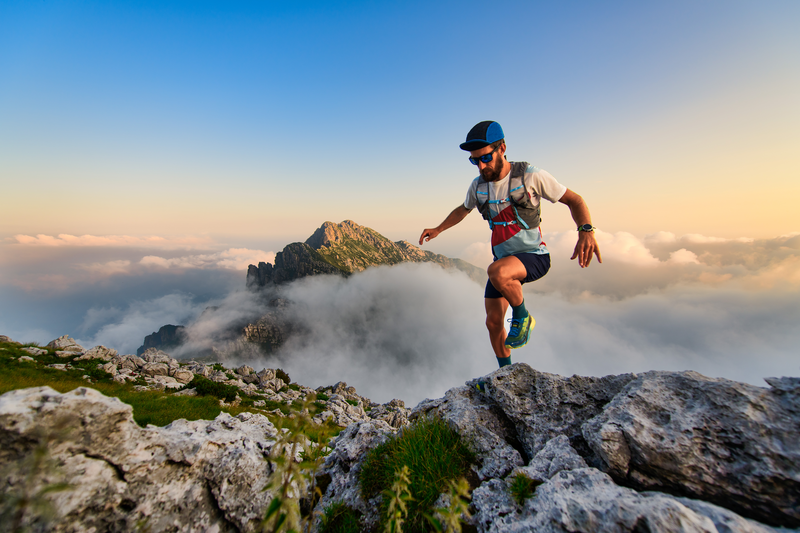
95% of researchers rate our articles as excellent or good
Learn more about the work of our research integrity team to safeguard the quality of each article we publish.
Find out more
SYSTEMATIC REVIEW article
Front. Neurol. , 01 July 2022
Sec. Experimental Therapeutics
Volume 13 - 2022 | https://doi.org/10.3389/fneur.2022.886603
This article is part of the Research Topic Hyperbaric Oxygen and the Brain View all 5 articles
Glioblastoma (GBM) is the most common and aggressive malignant brain tumor in adults. The mainstay of management for GBM is surgical resection, radiation (RT), and chemotherapy (CT). Even with optimized multimodal treatment, GBM has a high recurrence and poor survival rates ranging from 12 to 24 months in most patients. Recently, relevant advances in understanding GBM pathophysiology have opened new avenues for therapies for recurrent and newly diagnosed diseases. GBM's hypoxic microenvironment has been shown to be highly associated with aggressive biology and resistance to RT and CT. Hyperbaric oxygen therapy (HBOT) may increase anticancer therapy sensitivity by increasing oxygen tension within the hypoxic regions of the neoplastic tissue. Previous data have investigated HBOT in combination with cytostatic compounds, with an improvement of neoplastic tissue oxygenation, inhibition of HIF-1α activity, and a significant reduction in the proliferation of GBM cells. The biological effect of ionizing radiation has been reported to be higher when it is delivered under well-oxygenated rather than anoxic conditions. Several hypoxia-targeting strategies reported that HBOT showed the most significant effect that could potentially improve RT outcomes, with higher response rates and survival and no serious adverse events. However, further prospective and randomized studies are necessary to validate HBOT's effectiveness in the ‘real world' GBM clinical practice.
Glioblastoma (GBM) is the most common malignant primary brain tumor, representing more than 50% of all gliomas and ~20% of all primary malignant central nervous system (CNS) tumors (1–3). With an incidence rate of 3.19 cases per 100,000 individuals and a median age of 64 years, it is uncommon in children. The incidence is higher in men and Caucasians compared to Africans and Afro-Americans (4).
Currently, the standard of care consists of a multimodality treatment approach, including surgical resection, radiotherapy (RT), systemic therapy (chemotherapy—CT, targeted therapy), and supportive care. However, the overall prognosis remains unsatisfactory, long-term survival is rare, and the associated morbidity related to declining neurologic function and quality of life has a devastating impact on patients and their supportive social context (5, 6).
Blood vessels in GBM are dilated, tortuous, and excessively thin basement membrane. Subsequently, tumor vasculature is functionally abnormal with markedly increased interstitial fluid pressure, aggravating hypoxia and acidosis (7). Hence, under such conditions, conventional treatment is less effective because of the hypoxic microenvironment, contributing to a challenging scenario (8). Hypoxia emerges as an important factor involved in the tumor growth and aggressiveness, metabolic reprogramming, angiogenesis, resistance to cell death, immunosuppression, inflammation, and glial-to-mesenchymal transition of cancer cells. Thus, therapeutic approaches that target hypoxia-induced factors, such as a monoclonal antibody against VEGF (e.g., bevacizumab), have been used, but failed to increase survival (7, 9, 10).
A better understanding of hypoxia-mediated protection of GBM cells has led to other experimental types of treatment being tested in this setting, including hyperbaric oxygen therapy (HBOT). HBOT consists of breathing pure medical oxygen intermittently while inside a hyperbaric chamber pressurized to greater than sea level pressure (1 atmosphere absolute [ATA]). It is applied in several clinical conditions being also used for professional and military diving training. HBOT is considered therapeutic if the pressure used is 1.4 ATA or higher. Most series apply 2.0–2.5 ATA for 70 to 120 min (11, 12).
In 1996, Kohshi et al. published a pioneering report on the combination of HBOT with RT as a possible complementary treatment for patients with brain tumors (13). Several studies have substantiated the adjuvant use of HBOT with RT and CT from that moment on. In addition, HBOT has also proved to be useful in treating some cases of radiation-induced brain injury (14). Notwithstanding, scientific evidence is still needed to support these indications.
Given the lack of substantial data to incorporate HBOT as a complementary treatment in GBM, this review aims to comprise the existing literature and scientific evidence, analyze results from previous studies, and identify potential horizons that may merit further exploitation.
In order to include all available data on GBM and HBOT, we chose the scoping review as a methodology, as it could better map the existing literature and help to elucidate concepts.
Initially, our protocol did not contain any language or data limits. Our search strategies included case series, case reports, clinical practice recommendations, review articles, and supplemental files.
Queries were run on PUBMED, Google Scholar, and Cochrane. The first query employed “hyperbaric oxygenation” [MeSH] AND “brain” [MeSH] AND “neoplasms” [MeSH]. To ensure maximum inclusion, a second query was run on PUBMED with “glioma” [MeSH] AND “hyperbaric oxygenation” [MeSH].
This review followed the PRISMA criteria (15). Inclusion criteria consisted of (1) review articles of HBOT; (2) articles and expert meetings of clinical practice recommendations for GBM management; (3) articles regarding the physiological effects of oxygen on GBM; (4) case series of patients with GBM treated with HBOT; (5) case reports of patients with GBM treated with HBOT; (6) articles detailing preclinical data on the effects of oxygen on tumor cells; (7) articles/abstracts with available translations in Portuguese, English, Spanish, or French. The exclusion criteria comprised the following: (1) articles not mentioning GBM and/or HBOT at any point; (2) articles that only comprised normobaric oxygen use.
By the 27th of November 2021, our database search was completed.
In the previously mentioned identification phase (e.g., article searching), we obtained 512 results, which are detailed in Figure 1.
Figure 1. Adaptation of the PRISMA 2020 flow diagram for new systematic reviews [from (16)].
After excluding findings with no available abstract in English, duplicates and records that did not suit the purpose of this review, a total of 152 articles entered the subsequent stage. Articles not written or without available translation into Portuguese, English, Spanish, or French were excluded in the screening phase.
Two separate investigators (DAC and MS-A) screened all articles independently throughout this stage, ultimately including 89 articles. Therefore, we considered all research that pertained to GBM and HBOT eligible.
We have witnessed a gradual improvement in GBM's outcomes, presumably due to recent advances in multimodality therapy with surgery, new RT modalities, and anticancer agents (17). Although neuronavigation and intraoperative magnetic resonance (MRI) imaging are related to higher resection rates, they are not considered crucial prognostic factors in Neuro-Oncology (17).
Currently, the standard of care for these patients is maximal safe resection followed by postoperative RT with concomitant and adjuvant temozolomide (TMZ), as established by the phase III trial 22981/26981–EORTC/NCIC (1, 2). The median overall survival (OS) with this multimodal treatment is approximately 14 months (1, 2).
TMZ, an oral imidazotetrazinone methylation agent that inactivates the DNA repair enzyme O6-alkylguanine-DNA alkyltransferase, is the primary CT choice for glioma in clinical practice (9, 18). TMZ improves the patient's OS rate and extends progression-free survival (PFS) after surgery (1). However, the use of TMZ is limited due to its short plasma half-life, systemic toxicity, and limited access through the blood–brain barrier (9, 19). Moreover, the 5-year OS is still <8% under this treatment protocol (9).
The standard radiation dose is 60 Gy in 1.8–2.0 Gy per fraction administered over 6 weeks. Most dose-escalation studies were performed before the era of intensity-modulated RT (IMRT) (1, 2). IMRT allows for the selective delivery of high-dose per fraction radiation to the target volume while minimizing the dose to surrounding normal tissues (20). In addition, hypofractionation confers the benefits of shortening the RT course in patients with limited life expectancy, reducing costs and possibly increasing malignant cell death and decreasing accelerated repopulation (1, 20).
Panet-Raymond et al. (21) retrospectively studied the feasibility of delivering hypo-IMRT with concurrent and adjuvant TMZ in a cohort of 35 patients. The median OS was comparable to that after conventional fractionation, and the regimen was tolerable with no undue toxicity.
Helical tomotherapy (HT) can provide different modulation techniques and geometric flexibility compared to standard IMRT. In addition, superior control of dose distribution may allow for better dose uniformity within target and/or spare organs at risk (2).
Even though the optimal hypofractionation regimen using IMRT remains to be determined, the administration of hypo-IMRT with concomitant and adjuvant TMZ was found to be safe and feasible. The OS and PFS were comparable to those reported with conventional fractionation regimens (2).
Hypoxia has been suggested to contribute to glioma resistance to TMZ (9). Therefore, increasing the oxygen concentration in the tumor tissue may enhance CT effects (9). Likewise, the efficacy of ionizing radiation can be increased through the use of HBOT (22, 23) since the oxygen effect is caused by the reinforced formation of reactive oxygen species (ROS) and free radicals in the cell (23).
Reoxygenation during cancer treatment is one of the pinnacles of the four “Rs” of radiobiology, along with repair, repopulation, and reassortment (or redistribution) (24).
The presence or absence of molecular oxygen dramatically influences the biological effect of radiation in biological tissues. To generate an effect, molecular oxygen must be present during radiation exposure, or at least for the lifetime of the radiation-generated free radicals (24–26).
The primary mechanism of RT stems from the creation of ROS, consequently inducing cell death by apoptosis, necrosis, autophagy, and senescence. As oxygen is required for ROS generation, hypoxic tumors are resistant to the cytotoxic effects of RT. Hence, oxygen is responsible for the permanent damage induced by free radicals. Consequently, in its absence, these RT-induced lesions can be repaired.
For radiosensitization, only a small amount of oxygen is required. It is estimated that 0.5% oxygen (pO2 of about 3 mmHg) results in an intermediate radiosensitivity between hypoxia and total oxygenation. Several tumors implanted in animals have been shown to contain hypoxic cells that limit curability by single doses of X-rays. Hypoxic fractions range from 0 to 50%, with an average of about 15%.
There is strong evidence that human tumors contain hypoxic cells, including histological appearance, oxygen probe measurements, nitroimidazole binding, positron emission tomography (PET)/SPECT studies, and pretreatment hemoglobin levels. Oxygen probes with fast response times, implanted in a tumor and under computer control, may be used to obtain an oxygen profile. In addition, hypoxia in tumors can also be visualized with hypoxia markers such as pimonidazole or hypoxia-inducible factors (HIF—which play a role in radioresistance by upregulating downstream genes involved in apoptosis, metabolism, proliferation, and neovascularization) (27–32).
As a dynamic environment, an irradiated tissue can reoxygenate itself when hypoxic cells become, once more, oxygenated. Though the extent of reoxygenation and its rate varies widely for different experimental animal tumors, if it is rapid and complete, hypoxic cells will have little influence on the outcome of a fractionated radiation schedule.
While acutely hypoxic cells rapidly reoxygenate as the tumor's blood vessels open and close, chronically hypoxic cells respond slowly as the tumor shrinks. It has also been described that HBOT has a positive effect on radiosensitization in low-oxygenated tumor regions, as it helps to surpass the constraints of oxygen deficiency. Albeit reoxygenation cannot yet be measured in in vivo human tumors, it is presumed to occur.
Relying on anaerobic metabolism, gliomas thrive under hypoxic microenvironments, contributing to their poor prognosis. In fact, high lactic acid concentrations correlate with a worse prognosis (33).
Hypoxia plays a critical role in maintaining glioma malignancy and resistance, as it induces HIF pathways responsible for regulating important cell cycle genes (34). Consequently, hypoxia will also contribute to cell stemness maintenance by inhibiting cell cycle progression (blocking cells in the G1 phase).
Oxygen starvation mechanisms help explain GBM resistance to CT, promoting tumor growth, angiogenesis, and invasion. Angiogenesis is largely induced and driven by HIF-1α (35). However, the aberrant neovascularization that follows creates immature and flawed vessels that are unable to perfuse the rapidly growing tumor, leading to a hypoperfused neoplasm. The latter results in increasingly numerous necrotic areas (Figure 2).
Figure 2. Overview of the influence of hypoxia on glioblastoma: Oxygen deficiency is the main contributor to both chemo- and radioresistance (made with Canva® 2022, under a Pro license). HIF, hypoxia-inducible factors; HIF-1α, hypoxia-inducible factor 1α; HIF-2α, hypoxia-inducible factor 2α; pO2, partial pressure of oxygen; ROS, reactive oxygen species; SOX2, sex-determining region Y-box 2.
After demonstrating that glioma cells submitted to hyperbaric oxygenation grow, Wang et al. (36) also studied the effects of HBOT on the expression of the two main molecules that contribute to tumor progression: HIF-1α and HIF-2α. Both are also responsible for regulating sex-determining region Y-box 2 (SOX2—a stem cell marker responsible for blocking the cell cycle, inducing and maintaining cell stemness). In the same way, HIF-1α promotes the multidrug resistance mutation 1 (MDR1) transcription, thus enhancing multidrug resistance to cytotoxic agents. The authors hypothesize that a more oxygen-rich microenvironment not only promotes cell cycle progression but also decreases cell stemness (correlated with chemoresistance) by inhibiting HIF-1α, HIF-2α, and SOX2. In their experiment, mice submitted to HBOT alone had increased tumor volumes than control mice (e.g., normoxia). Yet, when HBOT was used adjunctively with TMZ, the mice had smaller tumors and prolonged survival than those treated with TMZ alone or given only HBOT. Therefore, despite promoting cell cycle progression (and inevitably, tumor growth), HBOT as an adjuvant therapy contributes to chemosensitisation (36). Even with this seemingly contradictory result, it is relevant to emphasize that the available literature does not support the hypothesis of HBOT as a promoter of malignant cell proliferation. Malignancy is not a contraindication for HBOT (37).
In 2004, Evans et al. had already established an association between hypoxia and tumor aggressiveness in 18 patients with supratentorial glial neoplasms. By intravenous administration of the agent 2-nitroimidazole EF5 [2-(2-nitro-1-H-imidazole-1-yl)-N-(2,2,3,3,3-pen-tafluoropropyl) acetamide] in the 24 to 48 h before the tumor biopsy, they measured hypoxia levels in vivo. Their findings support that severe hypoxia correlates with more aggressive clinical behaviors, with early recurrence being one of the consequences (38).
Hadanny and Efrati (39) also proposed an interesting concept: the “hyperoxic–hypoxic paradox.” This is based on the notion that the metabolic changes experienced at the cellular level triggered by hypoxic environments can also be induced by intermittent exposure to hyperoxia. In these conditions, tissue regeneration is activated by stimulating sirtuin 1 (SIRT1) and mitochondrial transfer. This results in a neuroprotective effect and the induction of neuro-recovery mechanisms (39).
The first documented use of hyperbaric medical therapy was in 1662 by Henshaw, a British physician who placed patients in a pressurized air container. Interestingly, it was carried out before the formulation of the Boyle–Mariotte Law, which described the relationship between pressure and volume of a gas, and before John Priestly discovered oxygen more than 100 years later.
Presently, the treatment of a patient resorting to HBOT occurs at elevated atmospheric pressures (higher than 1 ATA), with 100% oxygen. Thus, the tissue's oxygen partial pressure increases, resulting in a myriad of benefits, including improved oxygen supply, reduced inflammation and oedema, and even inhibition of infection.
HBOT employs several physiological principles of gas' diffusion and solubility, especially to predict the behavior of oxygen under pressure. Based on its solubility under pressure, when the oxygen concentration in a solution increases, the diffusion gradient for its delivery deeper into tissues also increases. Ultimately, the increase in dissolved oxygen generated by HBOT has several physiological effects capable of altering tissue responses to disease and injury. In a hyperbaric environment, it is possible to dissolve sufficient oxygen in the plasma to meet the body's usual needs. As physically dissolved oxygen will be more available than the one bound to hemoglobin, oxyhemoglobin will be able to pass unchanged from the arterial to the venous side (40).
The brain is the organ where HBOT has the most important metabolic effects. These are more pronounced in hypoxic/ischaemic states, where hyperbaric oxygenation can reduce cerebral oedema and improve the function of neurons rendered inactive by these lesion-inducing mechanisms. An enhanced electrical brain activity reflects this improvement in brain function. HBOT can also improve microcirculation, relieve cerebral hypoxia, partially preserve damaged tissue, and improve cerebral metabolism.
During treatment, the starting optimal pressure for patients with brain injury is 1.5 ATA, as the cerebral glucose metabolism is balanced at this pressure. Consequently, raising the pressure, even if only to 2 ATA, may have unfavorable effects. At high pressures, oxygen can have acute cytotoxic effects. These were first described in 1878 and usually manifest with seizures—the Paul-Bert effect—whose incidence ranges from 0.002 to 0.061% (41–43). Individual factors (e.g., previous seizures, carbon dioxide retention, alcohol, few drugs, fever, hypothermia, anxiety, cerebral trauma, metabolic acidosis, shock, increased pressure, and oxygen exposure) (44–46) can alter a person's sensibility and reduce their seizure threshold. The first-line approach for hyperoxic seizures is immediate treatment interruption.
Generally, HBOT therapy is safe and well-tolerated by humans at 1.5–2 ATA (11, 12).
Although rare and usually non-severe, HBOT has known potential side effects. In a retrospective analysis of 1.5 million treatments, only 0.68% were associated with an adverse event, with barotrauma and confinement anxiety being the most reported events (42). When patients are previously studied and the correct protocol is applied, side effects are scarce.
HBOT acts by increasing pressure (via pure oxygen administration) on the patient. The most common adverse effects will occur by disturbing air cavities, including ear, paranasal sinus, pathologic dental spaces, and emphysematous bulla (47).
Middle ear barotrauma (MEB) is this therapy's most common side effect. Its incidence varies according to the series and ranges from 0.37 to 84% in non-ventilated patients vs. 94% in ventilated patients (42, 48). Clinical presentation of MEB usually includes ear pain, hearing loss, tinnitus, and, more rarely, vertigo. Diagnosis is performed by otoscopy, and treatment will depend on its severity, generally consisting of decongestants, nasal and systemic steroids, and antibiotics.
To correctly equalize the air in the middle ear, the eustachian tube (which connects the middle ear to the nasopharynx) needs to be opened by the patient using Valsalva maneuvers (e.g., swallowing or chewing). Nevertheless, in patients who have difficulties equalizing air pressure, even medicated and trained, in some cases, a myringotomy with ventilation tubes may be necessary.
Sinus barotrauma (SB) is the second most frequent adverse effect, usually associated with upper airway infection. SB is rare with controlled pressurization and depressurization (49). The manifestations will depend on the affected sinus. Clinical presentation may include sensations of pressure, epistaxis, and pain. Treatment includes nasal decongestants, antihistamines, and/or steroid nasal spray, which should be prescribed prior to HBOT for SB prevention (50).
Despite being a highly vascularized tumor, GBM is characterized by extensive necrotic areas with surrounding hypoxia (6). Hypoxia has been associated with an increase in glioma stem-like cell properties, glioma growth, changes in cancer cell metabolism, and treatment resistance.
HBOT favorably improves oxygen transport to the hypoxic tumor tissues, thereby potentially increasing the sensitivity of tumor cells to antineoplastic treatment. However, while used alone, it does not inhibit tumor growth (33). In contrast, when used as an adjuvant treatment with RT and/or CT, it leads to a significant decrease in tumor size. Initial HBOT can modify the hypoxic microenvironment and impair the stemness-associated properties of cancer cells (51).
Regarding its benefit when used as an adjuvant treatment with TMZ, tumor tissue hypoxia tends to decrease with HBOT, and some hypoxic areas even return to regular oxygen supply. With an increase in oxygen concentration, the tumor tissue becomes more sensitive to CT drugs. In addition, hyperbaric oxygenation also amplifies the cell cycle arresting effect of CT, which might result in an increased affinity of tumor cells for TMZ (33), indicating that for the same drug dose, there is a higher rate of apoptosis. By combining nimustine and HBOT, the expression of inflammatory factors is reduced, and tumor growth is inhibited, indicating that inflammation plays a key role in tumor development (33).
HBOT has been used to treat late-onset RT-related injuries since 1975 (52), being CNS lesions a type 3 recommendation. In 1953, Gray et al. postulated that the primary source of radioresistance was oxygen deficiency (53). As RT's efficacy is based on the tumor tissue's oxygen content, which is why the combination with HBOT amplifies its therapeutic effect (functioning as a radiosensitizer), improving local tumor control and potentially extending survival time.
Using a mouse model of GBM, Wang et al. (54) investigated the effects of HBOT on ROS signaling from transplanted glioma cells, finding that under hyperbaric oxygenation, ROS signaling from glioma and brain cells was diminished. However, their findings raised a new question regarding the pro-oncogenic effects of HBOT on non-cancerous cells, as this therapy appears to produce systemic immunosuppression by inhibiting thymic T-cell maturation (54).
By transplanting gliomas into nude rats, Stuhr et al. (10) concluded that the tumor's hypoxic microenvironment could be altered through hyperoxia. Both normobaric and hyperbaric oxygenation (1.02 ATA vs. 2.04 ATA) inhibit tumor growth after only 4.5 h of treatment (approximately three exposures) (10). Nevertheless, HBOT significantly improves oxygen perfusion, damaging the tumor by inducing apoptosis and reducing its central vascular density.
The anticancer effects of hyperoxia and its action mechanisms are mostly identified in mouse lung cancer (55, 56). To detect the potential mechanism of HBOT in lung tumors, Chen et al. (57) selected, as an in vivo model, non-small cell human lung carcinoma A549-cell-transferred severe-combined immunodeficiency mice (SCID). Their results revealed that HBOT improves tissue angiogenesis and tumor hypoxia and increases tumor apoptosis by modifying the tumor's hypoxic microenvironment. The treatment also suppressed tumor growth in murine xenograft tumor models (57). This was the first study to demonstrate that HBOT immediately downregulated endogenous p53 protein, which rebounded to baseline after 20 h of treatment.
Zembrzuska et al. (58) studied the combination of isothiourea derivatives and HBOT, reporting that this regimen appears to be a promising therapeutic approach for malignant glioma, as it reduces the proliferation and viability of in vitro GBM cells compared to cells under normoxia and hypoxia.
Brizel et al. (59) also performed in vivo studies on rats with implanted mammary adenocarcinoma. These were divided into five groups, posteriorly treated with inhaled normobaric or hyperbaric oxygenation or normobaric or hyperbaric carbogen. Positive results were observed in the groups treated with high-pressure gases (59). Thews and Vaupel (60) conducted a similar study, which tested the influence of normal and hyperbaric oxygenation (pure oxygen vs. carbogen), local hypoxic regions occurrence, and spatial distribution of pO2, using the subcutaneously injected DS-sarcoma cell line in rats. Under the pressure of 2.07 ATA, the median pO2 was 5-fold higher. Following HBOT, the spatial distribution of pO2 profiles showed a nearly absolute elimination of hypoxic regions.
However, isolated HBOT has a limited curative effect and is typically not applied by itself (60).
Stuhr et al. (10) elucidated the effects of hyperbaric oxygenation alone on BT4C rat glioma xenografts in vivo. In his study, hyperoxia (both normal and hyperbaric) resulted in a 60% retardation of tumor growth compared to the control group. Although the proliferation rate of cells remained unchanged in the two groups, there were more necrotic areas, 20% more apoptotic cells in control tumors, and no damage to normal tissues (10). More inconsistent results were found with isolated HBOT, probably due to different HBOT procedures and intracranial measurement methods (61, 62).
Regarding the pO2 of normal brain tissue, in animal experiments, it has been noted to decrease quickly after HBOT, whereas the pO2 in high-grade gliomas falls more slowly after decompression due to the lower rate of oxygen consumption and the reduced blood flow to the tumor (63). Therefore, the pO2 within the tumor may remain elevated after decompression for a substantial time, suggesting that HBOT may increase the sensitivity of hypoxic tumor cells, even without increasing injury to the normal brain tissue (64).
Kunugita et al. (65) examined the effect of RT after HBOT on spontaneously arising murine squamous cell carcinomas (SCCVII) (with a radiobiological hypoxic fraction of ~10%) that were subcutaneously transplanted into C3H/He mice using a growth delay assay. They noted a significant SCCVII tumor growth delay in the treated animals within 30 min after HBOT, with the delay time being 1.61 times longer than that after RT alone (66).
Xie et al. (9), studied the combination of HBOT and nanotemozolomide in glioma using nude mice. Their findings confirm that HBOT is a suitable adjuvant treatment to CT, as the proliferation of tumor tissue was significantly lower when the two therapies were combined (9). Moreover, significant inhibition of tumor growth, weight, and size was also observed, thus supporting the pivotal role HBOT can have in enhancing antitumor agents' efficacy. A decrease in hypoxic fields after HBOT was also verified, adding up to 50% of hypoxic regions modified. Potentiation of the cell cycle arrest effect of the agent was also observed. The authors postulate that the increased tumor oxygen tension improves the therapeutical response to CT.
Accordingly, Lu et al. (67) found that pO2 in mice who underwent HBOT combined with nimustine therapy was significantly higher than standard, not only on the healthy brain tissue itself but also on the glioma tumor cells, which were approaching normal brain tissue pressures.
In a mouse model of aggressive metastatic cancer, Poff et al. (68) evaluated the efficacy of metabolic therapies, including the ketogenic diet, ketone supplementation, and hyperbaric oxygenation. In vitro, each therapy inhibited the proliferation and viability of tumor cells and decelerated disease progression. Nonetheless, when combined, they potentiated powerful antineoplastic effects by inhibiting metastasis proliferation and doubling the survival time of mice with systemic metastatic disease (69).
There are scarce data on prospective clinical studies evaluating HBOT in combination with RT or chemoradiation for GBM. Most of the evidence available consists of single-centered trials evaluating RT and HBOT. Nevertheless, findings have shown promising results in patients with high-grade glioma (69, 70).
In 1977, Chang et al. published their findings regarding whether HBOT during RT could improve results in glioma treatment (71). The median OS of the patients in the HBOT group was 38 weeks, compared with 31 weeks for patients in the control group, and survival rates after 18 months were 28 and 10%, respectively.
Kohshi et al. (72), conducted a non-randomized trial that evaluated the feasibility of associating RT with HBOT for 29 high-grade glioma patients. The median OS for patients with and without HBOT was 24 and 12 months, respectively (p < 0.05). All patients (n = 4) who received RT more than 30 min after decompression had worse outcomes. This constituted the early perception that the timing between modalities was critical to achieving the best outcomes. As no serious side effects were observed in HBOT patients, the authors concluded that irradiation after HBOT appeared to be a valuable treatment for high-grade gliomas. However, irradiation should be administered immediately after decompression (72).
Beppu et al. (69) published their phase II study integrating HBOT and beta-interferon, nimustine hydrochloride (ACNU), and RT (IAR, Interferon-ACNU-Radiotherapy) for the treatment of malignant supratentorial gliomas. Daily RT was completed within 15 min after HBOT. Of the 39 patients, 35 underwent a complete HBOT and IAR therapy schedule. Thirty patients (76.9%) either maintained or increased their Karnofsky Performance Status Scale (KPS) during HBOT/IAR, with a mean duration of 68 days. The combined complete and partial response rates for GBM, anaplastic astrocytoma, and overall general histologies were 50, 30, and 43%, respectively. Median time to progression (TTP) for GBM, anaplastic astrocytoma, and overall patients was 38, 56, and 43 weeks, respectively. The authors suggested that HBOT/IAR therapy could be applied to selected patients with poor prognostic factors due to its short treatment period, acceptable toxicity profile, and identical response when compared to patients with good prognostic factors (69).
Ogawa et al. reported the long-term results of a phase II trial for daily conventionally fractionated RT (60 Gy/30 fractions) 15 min after HBOT with multiagent CT (procarbazine, nimustine, and vincristine) in patients with high-grade gliomas. All patients were able to complete the RT immediately after HBOT. The median OS of 39 patients with GBM was 17.2 months. However, acute toxicity developed in almost 48 % of patients (70, 72).
Kohshi et al. (73) also investigated the combination of HBOT with fractionated stereotactic RT (FSRT) in a small cohort of previously irradiated patients with recurrent gliomas. The median OS of patients treated with this protocol was 19 and 11 months for anaplastic astrocytoma and GBM patients, respectively (73).
In another Japanese study, Yahara et al. (74) studied IMRT boosts after HBOT concurrent with TMZ-based CT. Of note, the authors estimated a biologically effective dose (BED) of 85.8 Gy10 for the high-dose clinical target volume (CTV). Previous studies have reported that dose escalation of RT for GBM above 60 Gy using conventional RT had limited success. The total BED of 85.8 Gy10 was relatively low compared to other trials. The first site of progression was locally in 58% of the patients with GBM, and grade ≥3 toxicity, such as brain radionecrosis, was not observed. The median OS was 22.1 months, which was promising considering a cohort of patients with GBM (75).
Arpa et al. (6) published their experience with HBOT and RT in a pilot study for recurrent high-grade glioma patients. In their small series of nine patients, RT was administered in daily 5-Gy fractions for 3–5 consecutive days. Each fraction was delivered within 1 h after HBOT. The disease control rate 3 months after HBOT-RT was 55.5% (5 patients), the median PFS for all patients was 5.2 months (95% CI: 1.34-NE), while 3- and 6-month PFS was 55.5% (95% CI: 20.4–80.4) and 27.7% (95% CI: 4.4–59.1), respectively. The median OS was 10.7 months (95% CI: 7.7-NE). No acute or late neurologic toxicity grade >2 was observed in 88.88% of patients, although one developed grade 3 radionecrosis. Despite the small cohort of selected patients, the paper poses a convenient short course alternative to systemic therapies for patients who cannot or refuse to undergo such treatments in this late stage of disease. To our knowledge, this is the only recruiting trial in this setting on ClinicalTrials.gov (6).
Altogether, the clinical studies enrolled over 200 patients with gliomas who received HBOT shortly before each RT fraction (Table 1).
Table 1. Summary of preclinical or clinical trials present in the literature, referring to the use of hyperbaric oxygen therapy in the treatment of gliomas and tumor cells, as well as in the carcinogenesis process.
The challenge of high-level evidence is multifactorial, considering the availability of HBOT and RT at the same institution, logistics to timely delivered RT, coordination between centers, and frailty of the targeted population to trial accrual candidates for a more robust trial. Another major obstacle to high-quality evidence is the simulation of a therapeutic session in a hyperbaric chamber. According to Lansdorp and van Hulst (75), the best approach to replicate the experience is to employ lower pressure and a gaseous mixture with 21% oxygen. Currently, this is considered the paramount approach to designing the sham-controlled arm (75).
Nevertheless, the findings from both the single- and double-arm studies indicated improvement in some outcomes (e.g., OS, PFS, TTP, and response rate) with HBOT and RT. Although reported toxicity included leukopenia, anemia, thrombocytopenia, fever, anorexia, and gastrointestinal symptoms like nausea, vomiting, or liver dysfunction, these reports cannot be attributed solely to HBOT. Patients with severe MEB were also uncommon (6, 70, 74, 76–78). So far, data favor further research on the clinical use of HBOT and RT for high-grade gliomas.
Thus, even though the available literature supports greater integration of HBOT into the treatment options, this should be preceded by precise planning and delivery in predetermined appropriate clinical settings.
Technological advances in Medical Physics, Machine Engineering, and Bioinformatics also bring new opportunities for enhancing RT's therapeutic index. A promising solution to fulfill the strict time interval between HBOT and RT could be the use of a device capable of performing both therapies, such as the one developed by HAUX-LIFE-SUPPORT GmbH for research at the University of Mainz (79).
Regarding radiation treatment, proton beam therapy (PBT) is a particle radiation therapy that uses protons instead of photons. Proton beams have the physical property (described as the Bragg's Peak) to deliver charged particles with a finite, energy-dependent range in tissue that can be adjusted to match the depth of the target. These results in a steep dose fall-off at the end of the particle path, allowing for better sparing of normal tissue.
Clinically, this offers an opportunity to improve RT for primary gliomas by reducing or eliminating the exposure of healthy tissues. This is of increasing interest, particularly in children.
Preventing irradiation of radiosensitive structures (e.g., hippocampus and cerebral cortex) and reducing the overall irradiated brain volume may improve quality of life endpoints, including neurocognitive dysfunctions and endocrine abnormalities. However, in spite of its dosimetric advantage and reported safety profile, PBT is not as widely available as photon-based RT, requiring greater investment.
A potential margin for dose escalation with PBT in GBM is an area of future research (80–85). In a National Cancer Data Base (NCDB) analysis, PBT was associated with improved outcomes in OS in adult patients either with low-grade or high-grade gliomas. Yet, its retrospective nature and inability to account for all potential confounding factors limit definitive conclusions. Other particle beams such as neutron and carbon ions are also of interest in glioma treatment investigation (85).
Currently, ongoing investigations are evaluating the use of PBT for gliomas. A Mayo Clinic trial studies the efficacy of 6-Fluoro-(18F)-l-3,4-dihydroxyphenylalanine-PET/MRI scan (18F-DOPA-PET/MRI) in the imaging of elderly patients with newly diagnosed GBM when planning for a short course of PBT. Using 18F-DOPA-PET scans and MRI scans may provide the radiation oncologist information on tumor vs. normal tissue, potentially contributing to a more accurate plan for radiation delivery.
FLASH RT uses ultrahigh-dose rates several times higher than any conventional dose rates used in RT or radiosurgery, delivering a RT session in nanoseconds. As a result, it generates a phenomenon known as the “FLASH effect.”
FLASH RT can overcome the limitations of nearby sensitivity of normal tissue and allow an increased radiation dose delivered to targets while keeping the toxicity of surrounding healthy tissues low (84–86). Oxygen plays a key role in the underlying biological mechanism resulting in the FLASH effect. Studies described ultrahigh-dose rate radiation could deplete local oxygen and induce a short-term protective hypoxic environment to the surrounding normal healthy tissues, increasing radioresistance. Although the oxygen depletion hypothesis is the most popular current explanation for the FLASH effect, other factors may play a role, including changes in ROS and redox chemistry between normal and tumor cells following FLASH dose rates, as well as immune responses and tumor microenvironment. The role played by this factor also requires further research. Remarkably, a first-in-human report has been published about a patient with CD30+T-cell cutaneous lymphoma treated using FLASH RT with electron beam therapy (84). FLASH RT still must overcome several technical limitations, but its property of inducing radioresistance to normal tissue can potentially change the future of clinical cancer treatment (86–89).
Lately, several radiosensitizers have been evaluated to reduce chronic and acute tumor hypoxia. For the past two decades, the DAHANCA group has been studying the integration of nimorazole in the standard therapeutic plan for head and neck cancers with positive results (90–94). To date, there is little knowledge of its use in high-grade glioma. One study evaluated the development of a bioresorbable layer for controlled TMZ and nimorazole release for palliative GBM treatment (95). However, the use of nimorazole (as an anti-hypoxia agent during RT) for high-grade gliomas remains to be clarified.
Survival of GBM patients depends on local disease control, as most will recur in proximity to the primary tumor site (6). Despite the endeavors and achievements made in treating GBM during the past decades, the prognosis remains poor, and long-term survival is rare.
Since the 1950s, researchers have assumed that oxygen is required to induce DNA damage and hypoxic adaptation is particularly restrictive to successful treatment of many solid tumors, including GBM. There are currently emerging data reporting that GBM patients undergoing combined RT and/or CT with HBOT are promising in terms of efficacy and safety. Presently, accurate quantification of the clinical efficacy of this therapeutical approach is still lacking. Further prospective and randomized studies are necessary to validate HBOT's effectiveness in standard clinical practice.
The original contributions presented in the study are included in the article/supplementary material, further inquiries can be directed to the corresponding author/s.
DAC conceived and designed this review. DAC and MS-A performed the acquisition, analysis, and interpretation of the data. Regarding the writing and detailed literature review, these were distributed as follows: EO—glioblastoma. EN and GF—hypoxia and radioresistance. DAC and MS-A—hypoxia and chemoresistance. AT, PD, GB, and CA—HBOT. DAC and MS-A—HBOT as a hypoxia reverser treatment. EN, GF, MS-A, and DAC—preclinical and clinical HBOT trials and the studies' limitations and future perspectives. Manuscript supervision was conducted by DAC and MS-A. All authors contributed to the article and approved the submitted version.
The authors declare that the research was conducted in the absence of any commercial or financial relationships that could be construed as a potential conflict of interest.
All claims expressed in this article are solely those of the authors and do not necessarily represent those of their affiliated organizations, or those of the publisher, the editors and the reviewers. Any product that may be evaluated in this article, or claim that may be made by its manufacturer, is not guaranteed or endorsed by the publisher.
The authors would like to acknowledge CUF Oncologia and the Portuguese Navy.
1. Stupp R, Mason WP, van den Bent MJ, Weller M, Fisher B, Taphoorn MJ, et al. Radiotherapy plus concomitant and adjuvant temozolomide for glioblastoma. N Engl J Med. (2005) 352:987–96. doi: 10.1056/NEJMoa043330
2. Jastaniyah N, Murtha A, Pervez N, Le D, Roa W, Patel S, et al. Phase I study of hypofractionated intensity modulated radiation therapy with concurrent and adjuvant temozolomide in patients with glioblastoma multiforme. Radiat Oncol. (2013) 8:38. doi: 10.1186/1748-717X-8-38
3. Stupp R, Toms SA, Kesari S. Treatment for patients with newly diagnosed glioblastoma–reply. JAMA. (2016) 315:2348–9. doi: 10.1001/jama.2016.1847
4. Bryukhovetskiy I, Ponomarenko A, Lyakhova I, Zaitsev S, Zayats Y, Korneyko M, et al. Personalized regulation of glioblastoma cancer stem cells based on biomedical technologies: from theory to experiment (review). Int J Mol Med. (2018) 42:691–702. doi: 10.3892/ijmm.2018.3668
5. Platten M, Wick W. Behandlung von hirntumorpatienten : hyperthermie, hyperbare oxygenierung, elektrische felder oder nanopartikel [Treatment of brain tumor patients: hyperthermia, hyperbaric oxygenation, electric fields or nanoparticles]. Nervenarzt. (2012) 83:982–7. doi: 10.1007/s00115-012-3569-7
6. Arpa D, Parisi E, Ghigi G, Cortesi A, Longobardi P, Cenni P, et al. Role of hyperbaric oxygenation plus hypofractionated stereotactic radiotherapy in recurrent high-grade glioma. Front Oncol. (2021) 11:643469. doi: 10.3389/fonc.2021.643469
7. Rosińska S, Gavard J. Tumor vessels fuel the fire in glioblastoma. Int J Mol Sci. (2021) 22:6514. doi: 10.3390/ijms22126514
8. Orukari IE, Siegel JS, Warrington NM, Baxter GA, Bauer AQ, Shimony JS, et al. Altered hemodynamics contribute to local but not remote functional connectivity disruption due to glioma growth. J Cereb Blood Flow Metab. (2020) 40:100–115. doi: 10.1177/0271678X18803948
9. Xie Y, Zeng X, Wu X, Hu J, Zhu Y, Yang X. Hyperbaric oxygen as an adjuvant to temozolomide nanoparticle inhibits glioma growth by inducing G2/M phase arrest. Nanomedicine. (2018) 13:887–98. doi: 10.2217/nnm-2017-0395
10. Stuhr LE, Raa A, Oyan AM, Kalland KH, Sakariassen PO, Petersen K, et al. Hyperoxia retards growth and induces apoptosis, changes in vascular density and gene expression in transplanted gliomas in nude rats. J Neurooncol. (2007) 85:191–202. doi: 10.1007/s11060-007-9407-2
11. Alpuim Costa D, Modas Daniel P, Vieira Branco J. The role of hyperbaric oxygen therapy in pneumatosis cystoides intestinalis-a scoping review. Front Med. (2021) 8:601872. doi: 10.3389/fmed.2021.601872
12. Alpuim Costa D, Amaro CE, Nunes A, Cardoso JS, Daniel PM, Rosa I, et al. Hyperbaric oxygen therapy as a complementary treatment for radiation proctitis: useless or useful? - A literature review. World J Gastroenterol. (2021) 27:4413–28. doi: 10.3748/wjg.v27.i27.4413
13. Jain KK. Role of HBO in enhancing cancer radiosensitivity. In: Sutorius G, editor. Textbook of Hyperbaric Medicine. Cham: Springer International Publishing (2017). p. 523–31.
14. Mathieu D, Marroni A, Kot J. Tenth European Consensus Conference on Hyperbaric Medicine: recommendations for accepted and non-accepted clinical indications and practice of hyperbaric oxygen treatment. Diving Hyperb Med. (2017) 47:24–32. doi: 10.28920/dhm47.2.131-132
15. Page MJ, Moher D, Bossuyt PM, Boutron I, Hoffmann TC, Mulrow CD, et al. PRISMA 2020 explanation and elaboration: updated guidance and exemplars for reporting systematic reviews. BMJ. (2021) 372:n160. doi: 10.1136/bmj.n160
16. Page MJ, McKenzie JE, Bossuyt PM, Boutron I, Hoffmann TC, Mulrow CD, et al. The PRISMA 2020 statement: an updated guideline for reporting systematic reviews. BMJ. (2021) 372:n71. doi: 10.1136/bmj.n71
17. Kawano H, Hirano H, Yonezawa H, Yunoue S, Yatsushiro K, Ogita M, et al. Improvement in treatment results of glioblastoma over the last three decades and beneficial factors. Br J Neurosurg. (2015) 29:206–12. doi: 10.3109/02688697.2014.967750
18. Tolcher AW, Gerson SL, Denis L, Geyer C, Hammond LA, Patnaik A, et al. Marked inactivation of O6-alkylguanine-DNA alkyltransferase activity with protracted temozolomide schedules. Br J Cancer. (2003) 88:1004–11. doi: 10.1038/sj.bjc.6600827
19. Patil R, Portilla-Arias J, Ding H, Inoue S, Konda B, Hu J, et al. Temozolomide delivery to tumor cells by a multifunctional nano vehicle based on poly(β-L-malic acid). Pharm Res. (2010) 27:2317–29. doi: 10.1007/s11095-010-0091-0
20. Floyd NS, Woo SY, Teh BS, Prado C, Mai WY, Trask T, et al. Hypofractionated intensity-modulated radiotherapy for primary glioblastoma multiforme. Int J Radiat Oncol Biol Phys. (2004) 58:721–6. doi: 10.1016/S0360-3016(03)01623-7
21. Panet-Raymond V, Souhami L, Roberge D, Kavan P, Shakibnia L, Muanza T, et al. Accelerated hypofractionated intensity-modulated radiotherapy with concurrent and adjuvant temozolomide for patients with glioblastoma multiforme: a safety and efficacy analysis. Int J Radiat Oncol Biol Phys. (2009) 73:473–8. doi: 10.1016/j.ijrobp.2008.04.030
22. Kohshi K, Beppu T, Tanaka K, Ogawa K, Inoue O, Kukita I, et al. Potential roles of hyperbaric oxygenation in the treatments of brain tumors. Undersea Hyperb Med. (2013) 40:351–62.
23. Bühler H, Strohm GL, Nguemgo-Kouam P, Lamm H, Fakhrian K, Adamietz IA. The therapeutic effect of photon irradiation on viable glioblastoma cells is reinforced by hyperbaric oxygen. Anticancer Res. (2015) 35:1977–83.
24. Hall EJ, Giaccia AJ. Radiobiology for the Radiologist. 8 ed. Philadelphia, PA: Lippincottt Williams & Wilkins (2018).
25. Akimoto T. [Radiobiological basis for hyperfractionated radiation therapy]. Gan To Kagaku Ryoho. (2008) 35:1820–2.
26. Shibayama C, Nakazawa M, Nakamura M, Akahane K, Takahashi S, Kashima E. [Accelerated fractionation]. Gan To Kagaku Ryoho. (2008) 35:1837–41.
27. Pan WL, Wong JH, Fang EF, Chan YS, Ng TB, Cheung RC. Preferential cytotoxicity of the type I ribosome inactivating protein alpha-momorcharin on human nasopharyngeal carcinoma cells under normoxia and hypoxia. Biochem Pharmacol. (2014) 89:329–39. doi: 10.1016/j.bcp.2014.03.004
28. Roh JL, Cho KJ, Kwon GY, Ryu CH, Chang HW, Choi SH, et al. The prognostic value of hypoxia markers in T2-staged oral tongue cancer. Oral Oncol. (2009) 45:63–8. doi: 10.1016/j.oraloncology.2008.03.017
29. Schrijvers ML, van der Laan BF, de Bock GH, Pattje WJ, Mastik MF, Menkema L, et al. Overexpression of intrinsic hypoxia markers HIF1alpha and CA-IX predict for local recurrence in stage T1-T2 glottic laryngeal carcinoma treated with radiotherapy. Int J Radiat Oncol Biol Phys. (2008) 72:161–9. doi: 10.1016/j.ijrobp.2008.05.025
30. Aga M, Bentz GL, Raffa S, Torrisi MR, Kondo S, Wakisaka N, et al. Exosomal HIF1α supports invasive potential of nasopharyngeal carcinoma-associated LMP1-positive exosomes. Oncogene. (2014) 33:4613–22. doi: 10.1038/onc.2014.66
31. Janssens GO, Rademakers SE, Terhaard CH, Doornaert PA, Bijl HP, van den Ende P, et al. Improved recurrence-free survival with ARCON for anemic patients with laryngeal cancer. Clin Cancer Res. (2014) 20:1345–54. doi: 10.1158/1078-0432.CCR-13-1730
32. Janssens GO, Rademakers SE, Terhaard CH, Doornaert PA, Bijl HP, van den Ende P, et al. Accelerated radiotherapy with carbogen and nicotinamide for laryngeal cancer: results of a phase III randomized trial. J Clin Oncol. (2012) 30:1777–83. doi: 10.1200/JCO.2011.35.9315
33. Xue T, Ding JS, Li B, Cao DM, Chen G. A narrative review of adjuvant therapy for glioma: hyperbaric oxygen therapy. Med Gas Res. (2021) 11:155–7. doi: 10.4103/2045-9912.318861
34. Dzhalilova DS, Makarova OV. HIF-dependent mechanisms of relationship between hypoxia tolerance and tumor development. Biochemistry. (2021) 86:1163–80. doi: 10.1134/S0006297921100011
35. Lum JJ, Bui T, Gruber M, Gordan JD, DeBerardinis RJ, Covello KL, et al. The transcription factor HIF-1alpha plays a critical role in the growth factor-dependent regulation of both aerobic and anaerobic glycolysis. Genes Dev. (2007) 21:1037–49. doi: 10.1101/gad.1529107
36. Wang P, Gong S, Pan J, Wang J, Zou D, Xiong S, et al. Hyperbaric oxygen promotes not only glioblastoma proliferation but also chemosensitization by inhibiting HIF1α/HIF2α-Sox2. Cell Death Discov. (2021) 7:103. doi: 10.1038/s41420-021-00486-0
37. Feldmeier J, Carl U, Hartmann K, Sminia P. Hyperbaric oxygen: does it promote growth or recurrence of malignancy? Undersea Hyperb Med. (2003) 30:1–18.
38. Evans SM, Judy KD, Dunphy I, Jenkins WT, Hwang WT, Nelson PT, et al. Hypoxia is important in the biology and aggression of human glial brain tumors. Clin Cancer Res. (2004) 10:8177–84. doi: 10.1158/1078-0432.CCR-04-1081
39. Hadanny A, Efrati S. The hyperoxic-hypoxic paradox. Biomolecules. (2020) 10:958. doi: 10.3390/biom10060958
40. Costa DA, Costa TP, Netto EC, Joaquim N, Ventura I, Pratas AC, et al. New perspectives on the conservative management of osteoradionecrosis of the mandible: a literature review. Head Neck. (2016) 38:1708–16. doi: 10.1002/hed.24495
41. Grim PS, Gottlieb LJ, Boddie A, Batson E. Hyperbaric oxygen therapy. JAMA. (1990) 263:2216–20. doi: 10.1001/jama.263.16.2216
42. Jokinen-Gordon H, Barry RC, Watson B, Covington DS. A retrospective analysis of adverse events in hyperbaric oxygen therapy (2012-2015): lessons learned from 1.5 million treatments. Adv Skin Wound Care. (2017) 30:125–9. doi: 10.1097/01.ASW.0000508712.86959.c9
43. Costa DA, Ganilha JS, Barata PC, Guerreiro FG. Seizure frequency in more than 180,000 treatment sessions with hyperbaric oxygen therapy - a single centre 20-year analysis. Diving Hyperb Med. (2019) 49:167–74. doi: 10.28920/dhm49.3.167-174
44. Ciarlone GE, Hinojo CM, Stavitzski NM, Dean JB. CNS function and dysfunction during exposure to hyperbaric oxygen in operational and clinical settings. Redox Biol. (2019) 27:101159. doi: 10.1016/j.redox.2019.101159
45. Lee YI, Ye BJ. Underwater and hyperbaric medicine as a branch of occupational and environmental medicine. Ann Occup Environ Med. (2013) 25:39. doi: 10.1186/2052-4374-25-39
46. Sanders RW, Katz KD, Suyama J, Akhtar J, O'Toole KS, Corll D, et al. Seizure during hyperbaric oxygen therapy for carbon monoxide toxicity: a case series and five-year experience. J Emerg Med. (2012) 42:e69–72. doi: 10.1016/j.jemermed.2008.12.017
47. Heyboer M 3rd, Sharma D, Santiago W, McCulloch N. Hyperbaric oxygen therapy: side effects defined and quantified. Adv Wound Care. (2017) 6:210–24. doi: 10.1089/wound.2016.0718
48. Miyazawa T, Ueda H, Yanagita N. Eustachian tube function and middle ear barotrauma associated with extremes in atmospheric pressure. Ann Otol Rhinol Laryngol. (1996) 105:887–92. doi: 10.1177/000348949610501109
49. Plafki C, Peters P, Almeling M, Welslau W, Busch R. Complications and side effects of hyperbaric oxygen therapy. Aviat Space Environ Med. (2000) 71:119–24.
51. Song K, Chen J, Ding J, Xu H, Xu H, Qin Z. Hyperbaric oxygen suppresses stemness-associated properties and Nanog and oncostatin M expression, but upregulates β-catenin in orthotopic glioma models. J Int Med Res. (2020) 48:300060519872898. doi: 10.1177/0300060519872898
52. Fernández E, Morillo V, Salvador M, Santafé A, Beato I, Rodríguez M, et al. Hyperbaric oxygen and radiation therapy: a review. Clin Transl Oncol. (2021) 23:1047–53. doi: 10.1007/s12094-020-02513-5
53. Gray LH, Conger AD, Ebert M, Hornsey S, Scott OC. The concentration of oxygen dissolved in tissues at the time of irradiation as a factor in radiotherapy. Br J Radiol. (1953) 26:638–48. doi: 10.1259/0007-1285-26-312-638
54. Wang YG, Long J, Shao DC, Song H. Hyperbaric oxygen inhibits production of CD3+ T cells in the thymus and facilitates malignant glioma cell growth. J Int Med Res. (2018) 46:2780–91. doi: 10.1177/0300060518767796
55. Lee HY, Kim IK, Lee HI, Lee HY, Kang HS, Yeo CD, et al. Combination of carboplatin and intermittent normobaric hyperoxia synergistically suppresses benzo[a]pyrene-induced lung cancer. Korean J Intern Med. (2018) 33:541–51. doi: 10.3904/kjim.2016.334
56. Kim SW, Kim IK, Ha JH, Yeo CD, Kang HH, Kim JW, et al. Normobaric hyperoxia inhibits the progression of lung cancer by inducing apoptosis. Exp Biol Med. (2018) 243:739–48. doi: 10.1177/1535370218774737
57. Chen SY, Tsuneyama K, Yen MH, Lee JT, Chen JL, Huang SM. Hyperbaric oxygen suppressed tumor progression through the improvement of tumor hypoxia and induction of tumor apoptosis in A549-cell-transferred lung cancer. Sci Rep. (2021) 11:12033. doi: 10.1038/s41598-021-91454-2
58. Zembrzuska K, Ostrowski RP, Matyja E. Hyperbaric oxygen increases glioma cell sensitivity to antitumor treatment with a novel isothiourea derivative in vitro. Oncol Rep. (2019) 41:2703–16. doi: 10.3892/or.2019.7064
59. Brizel DM, Lin S, Johnson JL, Brooks J, Dewhirst MW, Piantadosi CA. The mechanisms by which hyperbaric oxygen and carbogen improve tumor oxygenation. Br J Cancer. (1995) 72:1120–4. doi: 10.1038/bjc.1995.474
60. Thews O, Vaupel P. Spatial oxygenation profiles in tumors during normo- and hyperbaric hyperoxia. Strahlenther Onkol. (2015) 191:875–82. doi: 10.1007/s00066-015-0867-6
61. Wang YG, Zhan YP, Pan SY, Wang HD, Zhang DX, Gao K, et al. Hyperbaric oxygen promotes malignant glioma cell growth and inhibits cell apoptosis. Oncol Lett. (2015) 10:189–95. doi: 10.3892/ol.2015.3244
62. Ding JB, Chen JR, Xu HZ, Qin ZY. Effect of hyperbaric oxygen on the growth of intracranial glioma in rats. Chin Med J. (2015) 128:3197–203. doi: 10.4103/0366-6999.170278
63. Jamieson D, Van Den Brenk HA. Measurement of oxygen tensions in cerebral tissues of rats exposed to high pressures of oxygen. J Appl Physiol. (1963) 18:869–76. doi: 10.1152/jappl.1963.18.5.869
64. Sümen G Cimşit M Eroglu L. Hyperbaric oxygen treatment reduces carrageenan-induced acute inflammation in rats. Eur J Pharmacol. (2001) 431:265–8. doi: 10.1016/S0014-2999(01)01446-7
65. Kunugita N, Kohshi K, Kinoshita Y, Katoh T, Abe H, Tosaki T, et al. Radiotherapy after hyperbaric oxygenation improves radioresponse in experimental tumor models. Cancer Lett. (2001) 164:149–54. doi: 10.1016/S0304-3835(00)00721-7
66. Bennett MH, Feldmeier J, Smee R, Milross C. Hyperbaric oxygenation for tumor sensitisation to radiotherapy. Cochrane Database Syst Rev. (2018) 4:CD005007. doi: 10.1002/14651858.CD005007.pub4
67. Lu Z, Ma J, Liu B, Dai C, Xie T, Ma X, et al. Hyperbaric oxygen therapy sensitizes nimustine treatment for glioma in mice. Cancer Med. (2016) 5:3147–55. doi: 10.1002/cam4.851
68. Poff AM, Ward N, Seyfried TN, Arnold P, D'Agostino DP. Non-toxic metabolic management of metastatic cancer in VM mice: novel combination of ketogenic diet, ketone supplementation, and hyperbaric oxygen therapy. PLoS ONE. (2015) 10:e0127407. doi: 10.1371/journal.pone.0127407
69. Beppu T, Kamada K, Nakamura R, Oikawa H, Takeda M, Fukuda T, et al. A phase II study of radiotherapy after hyperbaric oxygenation combined with interferon-beta and nimustine hydrochloride to treat supratentorial malignant gliomas. J Neurooncol. (2003) 61:161–70. doi: 10.1023/A:1022169107872
70. Ogawa K, Ishiuchi S, Inoue O, Yoshii Y, Saito A, Watanabe T, et al. Phase II trial of radiotherapy after hyperbaric oxygenation with multiagent chemotherapy (procarbazine, nimustine, and vincristine) for high-grade gliomas: long-term results. Int J Radiat Oncol Biol Phys. (2012) 82:732–8. doi: 10.1016/j.ijrobp.2010.12.070
71. Chang CH. Hyperbaric oxygen and radiation therapy in the management of glioblastoma. Natl Cancer Inst Monogr. (1977) 46:163–9.
72. Kohshi K, Kinoshita Y, Imada H, Kunugita N, Abe H, Terashima H, et al. Effects of radiotherapy after hyperbaric oxygenation on malignant gliomas. Br J Cancer. (1999) 80:236–41. doi: 10.1038/sj.bjc.6690345
73. Kohshi K, Yamamoto H, Nakahara A, Katoh T, Takagi M. Fractionated stereotactic radiotherapy using gamma unit after hyperbaric oxygenation on recurrent high-grade gliomas. J Neurooncol. (2007) 82:297–303. doi: 10.1007/s11060-006-9283-1
74. Yahara K, Ohguri T, Udono H, Yamamoto J, Tomura K, Onoda T, et al. Radiotherapy using IMRT boosts after hyperbaric oxygen therapy with chemotherapy for glioblastoma. J Radiat Res. (2017) 58:351–6. doi: 10.1093/jrr/rrw105
75. Lansdorp CA, van Hulst RA. Double-blind trials in hyperbaric medicine: a narrative review on past experiences and considerations in designing sham hyperbaric treatment. Clin Trials. (2018) 15:462–76. doi: 10.1177/1740774518776952
76. Chen JR, Xu HZ, Ding JB, Qin ZY. Radiotherapy after hyperbaric oxygenation in malignant gliomas. Curr Med Res Opin. (2015) 31:1977–84. doi: 10.1185/03007995.2015.1082988
77. Ogawa K, Yoshii Y, Inoue O, Toita T, Saito A, Kakinohana Y, et al. Prospective trial of radiotherapy after hyperbaric oxygenation with chemotherapy for high-grade gliomas. Radiother Oncol. (2003) 67:63–7. doi: 10.1016/S0167-8140(02)00406-1
78. Ogawa K, Kohshi K, Ishiuchi S, Matsushita M, Yoshimi N, Murayama S. Old but new methods in radiation oncology: hyperbaric oxygen therapy. Int J Clin Oncol. (2013) 18:364–70. doi: 10.1007/s10147-013-0537-6
79. HAUX-LIFE-SUPPORT GmbH. HAUX-LIFE-SUPPORT GmbH: HAUX-LIFE-SUPPORT GmbH Special Hyperbaric Systems. (2022). Available online at: https://hauxlifesupport.de/en/products/special-systems/ (accessed May 10, 2022).
80. Chambrelant I, Eber J, Antoni D, Burckel H, Noël G, Auvergne R. Proton therapy and gliomas: a systematic review. Radiation. (2021) 1:218–33. doi: 10.3390/radiation1030019
81. Park Y, Yu ES, Ha B, Park HJ, Kim JH, Kim JY. Neurocognitive and psychological functioning of children with an intracranial germ cell tumor. Cancer Res Treat. (2017) 49:960–9. doi: 10.4143/crt.2016.204
82. Patel S, Kostaras X, Parliament M, Olivotto IA, Nordal R, Aronyk K, et al. Recommendations for the referral of patients for proton-beam therapy, an Alberta Health Services report: a model for Canada? Curr Oncol. (2014) 21:251–62. doi: 10.3747/co.21.2207
83. Thariat J, Sio T, Blanchard P, Patel S, Demizu Y, Ampil F, et al. Using proton beam therapy in the elderly population: a snapshot of current perception and practice. Int J Radiat Oncol Biol Phys. (2017) 98:840–2. doi: 10.1016/j.ijrobp.2017.01.007
84. Chapman TR, Ermoian RP. Proton therapy for pediatric cancer: are we ready for prime time? Future Oncol. (2017) 13:5–8. doi: 10.2217/fon-2016-0373
85. Jhaveri J, Cheng E, Tian S, Buchwald Z, Chowdhary M, Liu Y, et al. Proton vs. photon radiation therapy for primary gliomas: an analysis of the national cancer data base. Front Oncol. (2018) 8:440. doi: 10.3389/fonc.2018.00440
86. Hughes JR, Parsons JL. FLASH radiotherapy: current knowledge and future insights using proton-beam therapy. Int J Mol Sci. (2020) 21:6492. doi: 10.3390/ijms21186492
87. Esplen N, Mendonca MS, Bazalova-Carter M. Physics and biology of ultrahigh dose-rate (FLASH) radiotherapy: a topical review. Phys Med Biol. (2020) 65:23TR03. doi: 10.1088/1361-6560/abaa28
88. Zakaria AM, Colangelo NW, Meesungnoen J, Azzam EI, Plourde MÉ, Jay-Gerin JP. Ultra-High Dose-Rate, Pulsed (FLASH) radiotherapy with carbon ions: generation of early, transient, highly oxygenated conditions in the tumor environment. Radiat Res. (2020) 194:587–93. doi: 10.1667/RADE-19-00015.1
89. Bourhis J, Sozzi WJ, Jorge PG, Gaide O, Bailat C, Duclos F, et al. Treatment of a first patient with FLASH-radiotherapy. Radiother Oncol. (2019) 21 139:18–22. doi: 10.1016/j.radonc.2019.06.019
90. Toustrup K, Sørensen BS, Metwally MA, Tramm T, Mortensen LS, Overgaard J, et al. Validation of a 15-gene hypoxia classifier in head and neck cancer for prospective use in clinical trials. Acta Oncol. (2016) 55:1091–8. doi: 10.3109/0284186X.2016.1167959
91. Overgaard J, Hansen HS, Overgaard M, Bastholt L, Berthelsen A, Specht L, et al. A randomized double-blind phase III study of nimorazole as a hypoxic radiosensitizer of primary radiotherapy in supraglottic larynx and pharynx carcinoma. Results of the Danish Head and Neck Cancer Study (DAHANCA) Protocol 5-85. Radiother Oncol. (1998) 46:135–46. doi: 10.1016/S0167-8140(97)00220-X
92. Saksø M, Jensen K, Andersen M, Hansen CR, Eriksen JG, Overgaard J. DAHANCA 28: A phase I/II feasibility study of hyperfractionated, accelerated radiotherapy with concomitant cisplatin and nimorazole (HART-CN) for patients with locally advanced, HPV/p16-negative squamous cell carcinoma of the oropharynx, hypopharynx, larynx and oral cavity. Radiother Oncol. (2020) 148:65–72. doi: 10.1016/j.radonc.2020.03.025
93. Overgaard J, Sand Hansen H, Lindeløv B, Overgaard M, Jørgensen K, Rasmusson B, et al. Nimorazole as a hypoxic radiosensitizer in the treatment of supraglottic larynx and pharynx carcinoma. First report from the Danish Head and Neck Cancer Study (DAHANCA) protocol 5-85. Radiother Oncol. (1991) 20(Suppl. 1):143–9. doi: 10.1016/0167-8140(91)90202-R
94. Saksø M, Andersen E, Bentzen J, Andersen M, Johansen J, Primdahl H, et al. A prospective, multicenter DAHANCA study of hyperfractionated, accelerated radiotherapy for head and neck squamous cell carcinoma. Acta Oncol. (2019) 58:1495–501. doi: 10.1080/0284186X.2019.1658897
Keywords: glioblastoma, glioma, hypoxia, cancer, radiation, chemotherapy, hyperbaric oxygenation, hyperbaric oxygen
Citation: Alpuim Costa D, Sampaio-Alves M, Netto E, Fernandez G, Oliveira E, Teixeira A, Daniel PM, Bernardo GS and Amaro C (2022) Hyperbaric Oxygen Therapy as a Complementary Treatment in Glioblastoma—A Scoping Review. Front. Neurol. 13:886603. doi: 10.3389/fneur.2022.886603
Received: 28 February 2022; Accepted: 24 May 2022;
Published: 01 July 2022.
Edited by:
Amir Hadanny, Yitzhak Shamir Medical Center, IsraelReviewed by:
Enrico M. Camporesi, USF Health, United StatesCopyright © 2022 Alpuim Costa, Sampaio-Alves, Netto, Fernandez, Oliveira, Teixeira, Daniel, Bernardo and Amaro. This is an open-access article distributed under the terms of the Creative Commons Attribution License (CC BY). The use, distribution or reproduction in other forums is permitted, provided the original author(s) and the copyright owner(s) are credited and that the original publication in this journal is cited, in accordance with accepted academic practice. No use, distribution or reproduction is permitted which does not comply with these terms.
*Correspondence: Diogo Alpuim Costa, ZGlvZ28uY29zdGFAY3VmLnB0; ZGlvZ29hbHB1aW1jb3N0YUBnbWFpbC5jb20=
†These authors have contributed equally to this work
Disclaimer: All claims expressed in this article are solely those of the authors and do not necessarily represent those of their affiliated organizations, or those of the publisher, the editors and the reviewers. Any product that may be evaluated in this article or claim that may be made by its manufacturer is not guaranteed or endorsed by the publisher.
Research integrity at Frontiers
Learn more about the work of our research integrity team to safeguard the quality of each article we publish.