- 1Department of Neurology, Christian-Doppler Medical Centre, Paracelsus Medical University, Centre for Cognitive Neuroscience Salzburg, Member of the European Reference Network, Epicare, Salzburg, Austria
- 2Neuroscience Institute, Christian-Doppler Medical Centre, Paracelsus Medical University, Centre for Cognitive Neuroscience, Salzburg, Austria
- 3Department of Psychiatry, Psychotherapy & Psychosomatics, Christian-Doppler Medical Centre, Paracelsus Medical University, Salzburg, Austria
- 4Department of Psychology, Naturwissenschaftliche Fakultaet, Centre for Cognitive Neuroscience, Paris-Lodron University, Salzburg, Austria
- 5Department of Public Health, Health Services Research and Health Technology Assessment, UMIT–University for Health Sciences, Medical Informatics and Technology, Hall in Tirol, Austria
- 6Karl-Landsteiner Institute for Neurorehabilitation and Space Neurology, Salzburg, Austria
- 7Department of Mathematics, Paris-Lodron University, Naturwissenschaftliche Fakultaet, Salzburg, Austria
- 8Team Biostatistics and Big Medical Data, IDA Lab Salzburg, Paracelsus Medical University, Salzburg, Austria
- 9Research and Innovation Management, Paracelsus Medical University, Salzburg, Austria
Objective: According to Panksepp's hierarchical emotion model, emotion processing relies on three functionally and neuroanatomically distinct levels. These levels comprise subcortical networks (primary level), the limbic system (secondary level), and the neocortex (tertiary level) and are suggested to serve differential emotional processing. We aimed to validate and extend previous evidence of discrete and dimensional emotion processing in patient with juvenile myoclonic epilepsy (JME).
Methods: We recorded brain activity of patients with JME and healthy controls in response to lexical decisions to words reflecting the discrete emotion fear and the affective dimension negativity previously suggested to rely on different brain regions and to reflect different levels of processing. In all study participants, we tested verbal cognitive functions, as well as the relationship of psychiatric conditions, seizure types and duration of epilepsy and emotional word processing.
Results: In support of the hierarchical emotion model, we found an interaction of discrete emotion and affective dimensional processing in the right amygdala likely to reflect secondary level processing. Brain activity related to affective dimensional processing was found in the right inferior frontal gyrus and is suggested to reflect tertiary level processing. Psychiatric conditions, type of seizure nor mono- vs. polytherapy and duration of epilepsy within patients did not have any effect on the processing of emotional words. In addition, no differences in brain activity or response times between patients and controls were observed, despite neuropsychological testing revealed slightly decreased verbal intelligence, verbal fluency and reading speed in patients with JME.
Significance: These results were interpreted to be in line with the hierarchical emotion model and to highlight the amygdala's role in processing biologically relevant stimuli, as well as to suggest a semantic foundation of affective dimensional processing in prefrontal cortex. A lack of differences in brain activity of patients with JME and healthy controls in response to the emotional content of words could point to unaffected implicit emotion processing in patients with JME.
Introduction
A multitude of neuroimaging and electrophysiological evidence has been collected in recent years on emotional processing in the human brain (1). However, it is still not entirely clear which brain regions reflect emotional processing and what is the time course of such processing (2). Two main views provide evidence on emotional processing in humans. Discrete emotion theories (3–5) suggest that a limited number of discrete emotions (e.g., anger, fear, sadness) are evolutionary ingrained (3) and culturally universal (6). In contrast, dimensional emotion theories (7, 8) propose that a limited number of affective dimensions (e.g., valence and arousal) constitute the basis of emotional processing (9). Initially seen as opposing views, recent evidence is in support of the idea that discrete emotions and affective dimensions could both reflect emotional processing in humans (10, 11). A framework which is in support of this way of processing is provided by the hierarchical emotion model of Panksepp (5, 12, 13). According to this model, discrete emotions and affective dimensions are not seen as opposing views, but rather as describing different processes operating on different, neuroanatomically distinguishable levels (14). At a primary process level, discrete emotions are thought to originate in subcortical circuits, such as the periaqueductal gray (PAG). At a secondary process level, primary process level emotions are transformed into conditioned responses by classical and instrumental conditioning, which is served by limbic structures including the amygdala (15, 16). At the tertiary process level, neocortical structures such as the prefrontal cortex are thought to interact with the lower process levels by cognitive processes and to be shaped by socio-cultural demands clustering primary emotion information further into constellations of positive and negative affect (17, 18). Reading single words with emotional content has been reported to activate brain areas such as the hippocampus, parahippocampal gyrus, amygdala, anterior and posterior cingulate cortex, and orbitofrontal cortex (19–23). Briesemeister et al. (10, 11) reported electrophysiological and neuroimaging evidence for the processing of discrete emotion words reflecting high and low happiness but also for the processing of words reflecting the affective dimension of positivity in line with Panksepps hierarchical emotion model. The electrophysiological results indicated sequential processing of emotion information, with the discrete emotion happiness, affecting the early visual N1 component, and the affective dimension positivity, reflected in an N400-like component and the late positive complex. (10). Neuroimaging revealed limbic activity in the right amygdala for words reflecting the discrete emotion happiness, and activity in the prefrontal cortex such as the left and right inferior frontal gyri and left medial frontal gyrus for the affective dimension of positivity. These and other results strongly suggest that emotion processing relies on extended networks and might be altered if structures like the limbic system or the prefrontal cortex are impaired. This could be observed in certain neurological conditions, such as epilepsy in which patients have impaired emotion recognition (24–30). Juvenile myoclonic epilepsy (JME) comprises 5–10% of all epilepsies (31) and is one of the most common age-related idiopathic generalized epilepsies with a high reported genetic pre-disposition (32). JME-onset peaks between 14 and 16 years, usually presents massive myoclonic, generalized tonic-clonic and absence seizures (33–36). Executive functions are reported to be impaired in JME, comprising mental flexibility, inhibition of automated reactions, abstraction and categorization, planning and verbal fluency (37–40). Behavioral problems in patients with JME, such as poor social adjustment and impulsive personality traits, resembling patients with frontal lobe damage, are often observed (41, 42). The prevalence of psychiatric disorders of patients with JME varies between 35 and 49% and studies demonstrated increased mood, anxiety, and cluster B personality disorders (41, 43). Neuroimaging revealed subtle structural and functional alterations in thalamus and frontal cortex associated with cognitive, behavioral and emotional disturbances (44–47). Furthermore, recent studies reported morphological and functional alteration involving thalamo-cortical circuits, cerebellum, bilateral hippocampi, and cingulate, insular and occipital cortices (48–50). Thus, the clinical picture of patients with JME shows cognitive as well as emotional impairments.
In this prospective fMRI study on patients with JME and healthy controls we aimed to extend and to validate the previous reported dissociation of brain regions involved in discrete and dimensional emotion processing (11), by presenting words reflecting the negative emotion fear and the affective dimension of negativity. In line with the results of Briesemeister et al. (47) we hypothesized that the processing of words with high fear values differs from those with low fear values in the right amygdala and that the inferior frontal cortex shows increasing activity with increasing negativity of words. Furthermore, we expected general faster response times for words with high fear values compared to words with low fear values. In addition, we expected patients with JME and healthy controls to differ in the processing of words reflecting the affective dimension of negativity and that this differential processing is revealed by differences in brain activity in the inferior frontal cortex, since neuropsychological and neuroimaging evidence showed altered executive functioning in patients with JME. Concerning the neuropsychological testing, we expected patients with JME to show deficits in several verbal and cognitive measures related to executive functions and to be at higher risk of experiencing psychiatric disorders.
Materials and Methods
Participants
A total of 47 patients with JME were recruited in the Epilepsy Outpatient Clinic, Clinical Department of Neurology, Paracelsus Medical University, Salzburg, and were compared to 62 gender-, education- and age-matched healthy controls. Inclusion criteria for patients comprised the diagnosis of JME based on criteria of the International League against Epilepsy (51), above 14 years of age with willingness to participate in the project as well as informed consent obtained from patients or parents. Exclusion criteria for patients comprised the occurrence of any epileptic seizure <72 h prior to fMRI, neurological illnesses other than JME, structural lesions (if known from previous preliminary examinations), incompatibility with MRI investigation (e.g., metal implants, claustrophobia), pregnancy, and acute intake of Benzodiazepines. Exclusion criteria for healthy controls comprised individuals <18 years of age, previously known psychiatric and neurological illnesses or known structural lesions of the brain, incompatibility with MRI investigation, and pregnancy. Mean onset of epilepsy was at 14.23 years of age, mean epilepsy duration was 12.85 years. Three patients (6.2%) did not receive any antiseizure medication, 28 patients (58.3%) received monotherapy and 17 patients (35.4%) polytherapy. For a detailed sample description see Table 2. All participants gave written informed consent in accordance with the guidelines set by the local Ethics Committee.
Methods
Procedure
Psychiatric and neuropsychological assessments and functional magnet-resonance-imaging (fMRI) were carried out after all participants gave written informed consent. The test battery comprised a structured clinical interview on psychiatric and personality disorders, verbal intelligence, verbal fluency, verbal memory and reading speed. As the entire battery took about 2 h to complete, participants who were not able to complete it at once and were allowed to take part in two sessions. Additionally, participants completed structural and functional MRI. fMRI lasted about 1 h. fMRI was performed either before or after neuropsychological evaluation, or if participants wished to do so, on one of the following days. Healthy controls were compensated €100 for their participation, patients with JME did not receive financial compensation.
Neuropsychological and Clinical Tests
SCID I + II
The Structured Clinical Interview for Axis I disorders (SCID I) (52) and for Axis II disorders (SCID II) (53), based on the Diagnostic and Statistical Manual of Mental Disorders-IV, are considered to be the gold standard for a semi-structured assessment of clinical disorders and personality disorders, respectively. German versions (54) were used to assess DSM-IV Axis I and Axis II pathology.
Multiple-Choice Vocabulary Test
The Mehrfach-Wortschatz-Intelligenzttest (MWT-B) is a multiple-choice vocabulary test which serves as an estimate of crystalline verbal intelligence (55, 56). In this test the participants are asked to identify a known colloquial or scientific word in-between four non-words. The 37 items are arranged by increasing difficulty.
Verbal Fluency Test
The Regensburger Wortflüssigkeitstest (RWT) (57) assesses verbal fluency. Specifically, we measured semantic fluency by counting the number of animals and the number of - alternating – fruits and sports, as well as lexical fluency of words starting with the letter “S” and, again alternating, words starting with “G” and “R.” For each condition, the participant was given 2 min time.
Auditory Verbal Learning Test
The Verbaler Lern- und Merkfähigkeitstest (VLMT) (58, 59) is a German version of Rey's (60) Auditory Verbal Learning Test (61). The VLMT consists of fifteen words, which are spoken by the examiner on five successive trials. Following each trial, the individual recalls all memorized words. After the five trials, a second group of words (distraction) is read aloud and recalled by the individual. After the distraction list, the individual recalls the words from list one. Following approximately 20 to 30 min, the individual again recalls all words from list one. Finally, the individual is instructed to recognize words from list one from a list of forty-five words. The test measures verbal learning ability, verbal memory consolidation and verbal recognition.
Sentence Reading Test
The sentence reading test, developed by Bergmann and Wimmer (62) requests participants to read short sentences and judge their semantic content. The sentences are of simple content so that erroneous markings are infrequent and the number of sentences marked within 3 min largely reflects reading speed and semantic comprehension.
fMRI Paradigm
Lexical Decision Task (LDT)
When reading single words in the fMRI-scanner, participants completed a lexical decision task (LDT) (63) in which subjects were shown German nouns (targets; e.g., Henker [hangman]) or German non-words (non-targets; e.g. Luicke). Participants had to decide whether the presented stimulus was a correctly spelled German word or not. Non-words were created changing two letters of an existing German noun. The LDT is the most used task to measure lexical access to the visual word form (63–66).
Stimuli
Following the design of Briesemeister et al. (10, 11), we used 120 German four- to eight-letter nouns and 60 non-words in a 2 (discrete emotion) x 2 (affective dimension) within subjects design with 30 items per cell. Contrary to Briesemeister et al. who used happiness and positivity, we tried to extend previous results by using words reflecting the emotion fear and words reflecting the affective dimension of negativity. Stimuli were chosen from the Berlin Affective Word List-Reloaded (67) (BAWL-R) and its discrete-emotion extension, the Discrete Emotion Norms for Nouns BAWL (68) (DENN-BAWL). The BAWL-R is a rating-based word list with norms for affective valence (7-point Likert scale, ranging from negative [-3] to positive [3]) and arousal (5-point Likert scale, ranging from low [1] to high arousing [5]). Given that negativity judgments and BAWL-R's valence ratings are highly correlated (69), words with BAWL-R scores between −0.7 and 0.7 were defined as being of neutral valence (NEU), and words with valence scores below 1 were defined as being of negative valence (NEG). The DENN-BAWL norms were used to classify words as being either strongly or not strongly related to fear, with low-fear words (lowFEAR) having DENN-BAWL scores below 2.0 and high-FEAR words (highFEAR) having scores above 2.0. DENN-BAWL norms indicate the extent to which a single word is related to one of five discrete-emotion categories, with high scores indicating a strong relation. The resulting four orthogonal conditions (lowFEAR + NEU: e.g., “AGENT,” Engl. “AGENT”; lowFEAR + NEG: e.g., “MIETE,” ENGL “RENT”; highFEAR + NEU: e.g., “MESSER,” ENGL. “KNIFE”; highFEAR + NEG “TEUFEL,” ENGL. “DEVIL”) were uncorrelated for fear and valence scores, indicating that lowFEAR + NEG words are perceived as being negative but not relate to the discrete emotion fear, and vice versa. For statistical details about the stimulus set, see Table 1. Mean levels of arousal, imageability, orthographic neighborhood size, frequency of orthographic neighbors, frequency of higher-frequent orthographic neighbors, and the mean number of letters, syllables, bigram frequency, and higher-frequency orthographic neighbors were controlled using analyses of variance (ANOVAs, all Fs >1; see Table 1). The psycholinguistic variables were matched for the highFEAR-versus-lowFEAR and NEU-versus-NEG contrasts and tested with pairwise t-tests (all ts <1). A list containing all 120 words and 60 non-words is provided in the Supplementary Materials. In addition, 30 null-events, serving as baseline, in the form of a fixation cross (“+”) were included, which was meant to increase the signal-to-noise ratio of the fMRI paradigm.
fMRI Procedure
Before entering the scanner, participants received oral and written instructions to decide as quickly and accurately as possible via button press whether the presented letter string was a correct German word (left button) or a non-word (right button) of a button box. They were instructed not to press any button when presented with fillers. Eighteen practice trials (twelve words, six non-words, three null-events) that were not part of the stimulus set were used to familiarize the participants with the task. The stimuli were presented in an event-related design on a 32-inch LCD screen, specifically designed for use in an MRI environment (70). The screen resolution was 1,920 × 1,080 pixels, with a screen size of 69.8 × 39.3 cm, a refresh rate of 120 Hz, and a built-in linear luminance look-up table. The display was positioned at the far end of the bore and was viewed via a mirror positioned in the head coil of the MRI scanner. The total viewing distance was 220 cm. The total visible vertical extent of the screen subtended 10.2 degrees visual angle (deg). All stimuli were generated in MATLAB R2013a (Mathworks Inc., Natick, MA, USA) (71) using the Psychophysics toolbox (72). Stimuli were presented using Presentation software (73), which also recorded response times and accuracy of responses. Each trial began with the presentation of a fixation cross (+) in the center of the screen, which was presented for 2,148 ms on average (jitter: 1,401–4,098 ms), followed by the stimulus (800 ms) at the exact same position. The presentation of the words was randomized. The words were presented in black uppercase letters (Arial, font size 50) on a white background. Responses were given through a button box held in the right hand, the right button (blue) for a correct German word and the left button (green) for a non-word. An external pulse from the scanner controlled the start of the first trial.
fMRI Data Acquisition
fMRI data were acquired with a Siemens Magnetom Prisma-fit 3T MRI scanner with a 64-channel head/neck coil. The fMRI run for the lexical decision task consisted of 620 images and lasted about 10 min, including six dummy scans at the beginning. For distortion correction of the functional images an EPI fieldmap sequence based on the Siemens product fieldmapping sequence (TR 623 ms, TE1 4.92 ms, TE2 7.38 ms) was acquired. Structural imaging included a high-resolution T1w (TR 2400 ms, TE 2.24 ms) and T2w sequence (TR 3200 ms, TE 56 ms,), both with 0.8 mm isotropic resolution with sequences from the Human Connectome Project (HCP) Lifespan protocol (74). For preprocessing and statistical analysis, SPM12 software (75), running in a MATLAB R2013a environment (Mathworks Inc., Natick, MA, USA), and additional functions from AFNI (76) were used. Functional images were realigned, de-spiked (with the AFNI 3ddespike function), unwarped, and corrected for geometric distortions using the fieldmap of each participant and slice time corrected. The high resolution structural T1-weighted image of each participant was processed and normalized with the CAT12 toolbox (77) using default settings, each structural image was segmented into gray matter, white matter and CSF and denoised, then each image was warped into MNI space by registering it to the DARTEL template provided by the CAT12 toolbox via the high-dimensional DARTEL (60) registration algorithm. Based on these steps, a skull stripped version of each image in native space was created. To normalize functional images into MNI space, the functional images were co-registered to the skull stripped structural image and the parameters from the DARTEL registration were used to warp the functional images, which were resampled to 3 × 3 × 3 mm voxels and smoothed with a 6 mm FWHM Gaussian kernel. Statistical analysis was performed with a general linear model (GLM) two-staged mixed effects model. In the subject-specific first level model, each condition (discrete emotion: FEAR high/low, emotions dimension: Negativity yes/no) was modeled by convolving stick functions at its onsets with SPM12's canonical hemodynamic response function. Parameter estimates for each condition were calculated via these first-level GLMs, using a temporal high-pass filter (cutoff 128 s) to remove low-frequency drifts and modeling temporal autocorrelation across scans with an AR (1) process (78). For voxel-based group analyses, contrast images for effects of interest were calculated at the first level. These contrast images were used in second level analyses for a words vs. baseline contrast. All results from whole brain analyses are reported at a voxel-level threshold of p < 0.001 (uncorrected) with a FWE cluster-level correction of p < 0.05.
ROI Analysis
We performed three region of interest (ROI) analyses. The functionally ROI analysis was performed for our a priori regions (i.e., right amygdala, left and right inferior frontal gyrus) using the coordinates used by Briesemeister et al. (11). The amygdala and inferior frontal gyri were selected based on their suggested role in Panksepp's hierarchical emotion theory, representing secondary and tertiary level processes, respectively. The ROIs were created with a sphere of 6 mm in the right amygdala (x = 21, y = 2, z = −11) and in the left (x = −45, y = 35, z = 26) and right inferior frontal gyrus (x = 42, y = 26, z = −8). ROIs were built with the MarsBar toolbox implemented in SPM12. ROI extraction was performed with REX (79), based on the average contrast estimates of our four word conditions, for further statistical analysis.
Statistical Methods
Statistical analyses were performed in SPSS (version 18) (80) and R (version 4.0.5) (81). First, we defined primary, secondary and tertiary outcomes. Primary outcomes were any effects of activation in a 2 (group: JMEs/HCs) x 2 (discrete emotion: FEAR high/low) x 2 (emotions dimension: Negativity: yes/no) repeated measures ANOVA of our fMRI-data. Secondary outcomes were behavioral responses in the fMRI-paradigm (response times and error rates), again tested in a 2x2x2 design using a non-parametric ANOVA type test for repeated measure designs provided by the R package nparLD (82). Post-hoc contrasts in differences for the relative treatment effect were computed using a normal approximation with a Fisher transformation and the delta method, as described in Gunawardana and Konietschke (83). Mean lexical decision response times (LDRTs) were calculated for each participant and condition after the exclusion of non-responders, behavioral errors, and outliers, which were defined as responses faster than 300 ms or slower than 1,500 ms. In total 3.38 and 3.19% of responses were filtered for healthy controls and patients with JME, respectively. Error rates (ERRs) were calculated as the summed errors per condition and participant. Additionally, neuropsychological measures were defined as secondary outcomes. Group differences in neuropsychology variables were computed with univariate comparisons between groups, done via a non-parametric t-test (Brunner-Munzel Test) using the R package rankFD (84). Tertiary outcomes used the same model for behavioral responses as in the secondary outcomes but with an additional between subject factor for psychiatric comorbidities and the same model computed for patients only with type of seizure as a between subject factor (generalized tonic clonic seizures vs. absences and myoclonic seizures only vs. seizurefree). Further, we repeated the analysis of the secondary outcomes replacing group with type of antiseizure medication (mono- vs. polytherapy) only for patients with JME. We also conducted a repeated measures ANCOVA with 2 (discrete) x 2 (dimension) as within subject factors and duration of epilepsy as covariable only in patients, using the R-package nlme (85). In order to maintain control of the type I error and yet ensure adequate statistical power we employed the following scheme for p-value adjustment in our neuropsychological comparisons. For the primary outcomes, we used the Bonferroni procedure to control the FWER for each spherical regions of interest (i.e., multiplying the p-values by three). For secondary outcomes we used the Benjamini-Yekutieli (86) procedure to control the FDR at a level of 0.05. For tertiary outcomes, we did not conduct any correction for multiplicity as we considered them to be auxiliary analyses. For p-values we provide the unadjusted and adjusted version. Confidence intervals are unadjusted.
The effect measure used for primary outcomes was η2 and for non-parametric analyses (secondary outcomes) the RTE. For comparisons between two groups RTE is the probability that a random subject from one group has a higher value in the outcome variable than a random subject from the other group. It is identical to the area under the curve when using the outcome variable to classify subjects into the two groups. For more than two groups it is the probability that a random subject from one group has a higher value in the outcome variable than a random subject from the total sample.
Results
Clinical Features
Twenty-eight patients (60.9%) and 12 controls (19.7%) presented a psychiatric disorder. 15.2% of patients had Axis I, 23.9% Axis II and 21.7% Axis I & II disorders (see Table 2). Considering multiple diagnoses in one patient, affective (15.2%), anxiety (13.0%), substance-related disorders (13.0%) and obsessive-compulsive personality disorders (OCD; 21.7%) represented the majority of psychiatric comorbidities. For a detailed description of psychiatric diagnoses see Supplementary Table 1. Due to multiple sessions, incompliance and tiredness of study participants, not all neuropsychological subtests have been performed in all patients (Supplementary Table 2).
Neuropsychology
In our non-parametric ANOVA type test (82) patients had significantly lower scores in the verbal intelligence test than healthy controls (p = 0.01). Patients with JME performed worse in single phonematic fluency (p = 0.01) as well as single (p = 0.06) and alternating semantic fluency (p < 0.001). Reading speed was decreased in patients with JME compared to healthy controls (p < 0.001). Despite having significantly lower scores than healthy controls, patients performed, for the most part, on average in all subtests. There were no differences in verbal memory functions: total learning score, delayed recall and recognition. All reported results were significant after correction for multiple comparisons. For a detailed description see Supplementary Table 2.
Behavioral Results: Lexical Decision Response Times and Error Rates
A 2 (group: JMEs/HCs) x2 (discrete emotion: FEAR high/low) x2 (emotion dimension: Negativity: yes/no) non-parametric repeated measures ANOVA revealed a significant main effect of fear (p = 0.006) that was driven by faster responses of highFEAR words compared to lowFEAR words (detailed descriptive statistics are presented in Table 1, inferential statistics in Table 4, and in Supplementary Figure 1). Moreover, a FEAR x Negativity interaction approached significance (p < 0.001). Planned pairwise comparisons between all conditions revealed faster response times of highFEAR+NEU compared to lowFEAR+NEU words (p < 0.001). Error rates showed a significant main effect of group with healthy controls making significantly less errors (p < 0.001). A significant main effect of Negativity (p < 0.001) that was driven by an increased number of errors in response to words of negative valence (NEG) compared to words with neutral valence (NEU). Further, a FEAR x Negativity interaction (p < 0.001) was driven by more errors for highFEAR+NEG than highFEAR+NEU words. Post-hoc sensitivity analysis adding psychiatric comorbidity or type of seizure (generalized tonic-clonic seizures x myoclonus and/or absences x seizure free) did not change the significance of the aforementioned effects regarding response times and error rates. Further, no group or interaction with group was significant when comparing antiseizure medication (mono- vs. polytherapy) in patients only. Duration of epilepsy did not correlate with either, response time or error rate in the repeated measures ANCOVA.
Neuroimaging Results
Whole Brain Analysis
The voxel-based whole-brain analysis across both groups revealed a main effect for words compared to fixation-baseline. Higher activity for words was found in a number of regions commonly involved in word processing, including an extended cluster in the left and right occipital lobe including inferior occipitotemporal, parietal and frontal areas, a left medial temporal cluster, a right occipitoparietal cluster, a right parahippocampal cluster and a cluster located in the brainstem. Please see Figure 1 and Table 3 for details. We did not observe any statistically significant differences between patients with JME and controls in the words vs. fixation-baseline contrast.
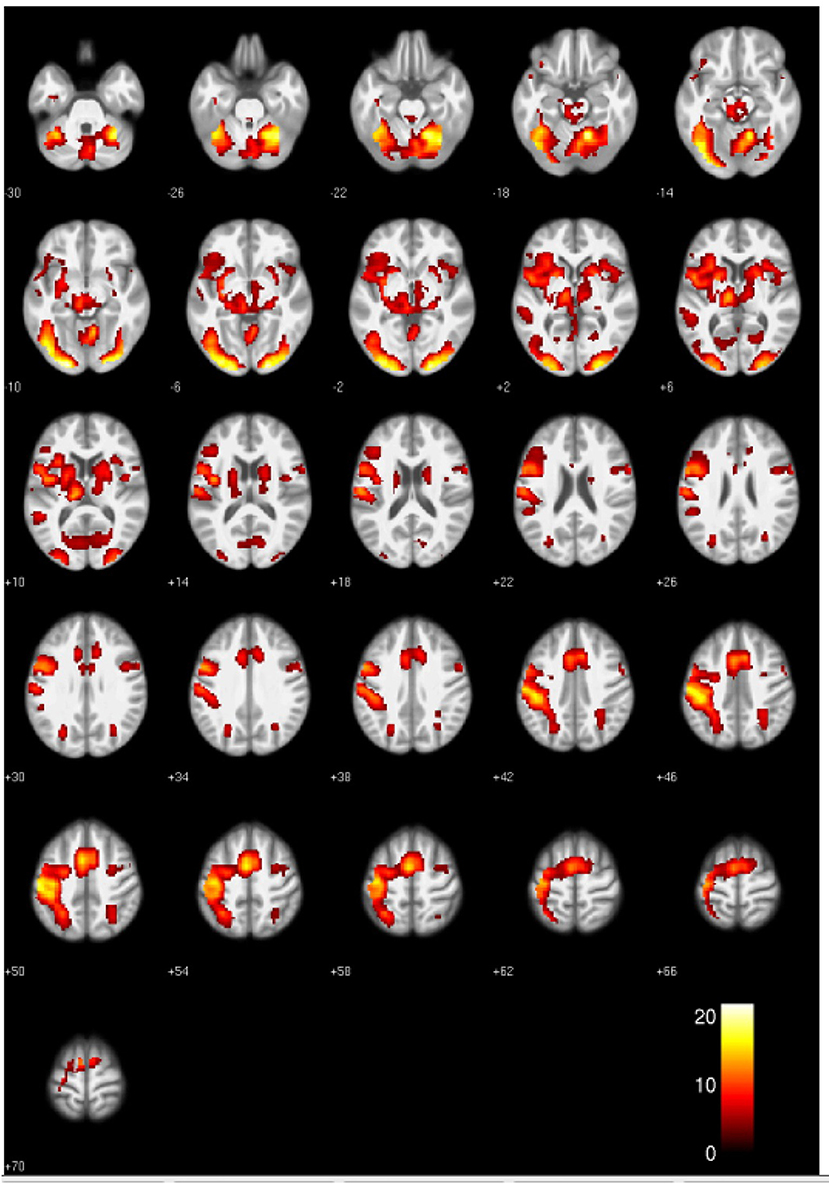
Figure 1. Activation clusters revealed by the whole brain analyses. Regions that elicited increased activation for words compared to baseline (irrespective of group) are shown in yellow. All clusters were extracted at a threshold of p < 0.001 [uncorrected, with a FWE cluster-level-correction (p < 0.05)].
ROI Analyses
A 2 (group: JMEs/HCs) x 2 (discrete emotion: FEAR high/low) x 2 (affective dimension: Negativity yes/no) repeated measures ANOVA revealed a main effect of affective dimension in the right inferior frontal gyrus (p = 0.001), which was driven by higher activity in response to words with negative valence compared to words with neutral valence. The same repeated measures ANOVA in the right amygdala revealed a significant interaction of fear and negativity (p = 0.018), driven by higher activity in response to words with high fear values and negative valence compared to words with low fear values and negative valence. No differences were found in the left inferior frontal gyrus. A main effect of fear in the right amygdala (p = 0.06) driven by higher activity for words with high fear compared to low fear, as well as a main effect of group in the right inferior frontal gyrus (p = 0.06), driven by higher activity in patients with JME compared to healthy controls, did not reach significance after Bonferroni p-value correction. Please see Table 4 and Figure 2 for details.
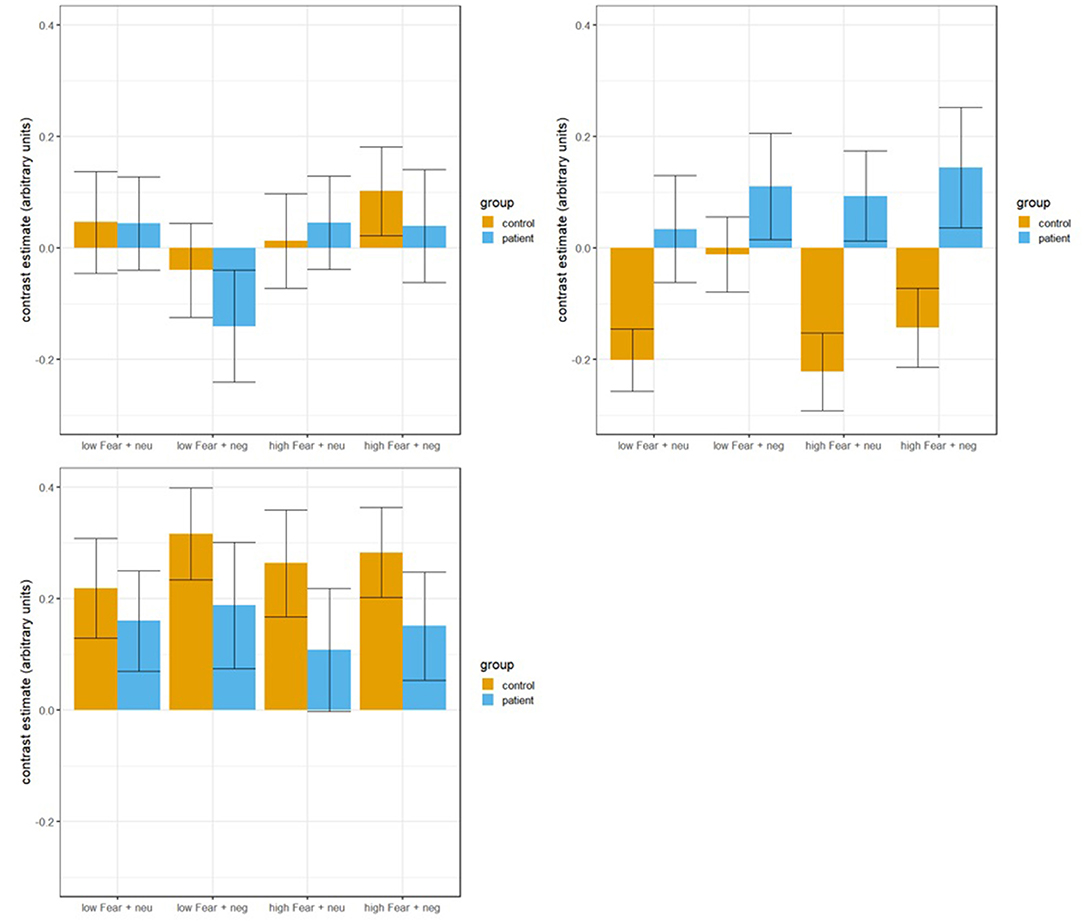
Figure 2. fMRI results for our conditions in the right amygdala (top left), right inferior frontal cortex (top right), and left inferior frontal gyrus (bottom left). Errror bars indicate standard error.
Discussion
With this study we aimed to validate and to extend previous findings suggesting hierarchical processing of emotion (10, 11, 13). In support of our hypothesis, we found an interaction of discrete emotion and affective dimensional processing in the right amygdala, likely to reflect secondary level processing. Further, brain activation in the right inferior frontal gyrus points to affective dimensional processing, suggested to reflect tertiary level processing. The processing of emotional words was not influenced by psychiatric conditions, type of seizure or duration of epilepsy, as well as mono- vs. polytherapy in JMEs only. Patients and healthy controls did not show any differences in brain activity or response times, despite higher incidences of psychiatric conditions and slightly decreased verbal intelligence, verbal fluency and reading speed in patients with JME.
Based on the results of Briesemeister et al. (10, 11) and the idea that affectively conditioned stimuli like words access secondary and tertiary process levels within Pankespp's hierarchical emotion model (13), we expected the amygdala and the inferior frontal gyrus, to be involved in implicit emotional word processing (19, 22, 23, 87). Therefore, we tested healthy controls and patients with JME which are suggested to have cognitive, behavioral and emotional disturbances, related to subtle structural and functional alterations in frontal brain regions (44–47). Furthermore, we were interested in verbal and neuropsychological functions of patients with JME, and if psychiatric conditions, type of seizure, mono- vs. polytherapy, and duration of epilepsy were related to discrete emotion and affective dimensional processing. The whole brain analysis revealed brain regions typically activated in reading emotional words (22), involving occipital, temporal, parietal and frontal regions. Activity in the right amygdala in response to the emotional content of words, confirmed the results of Briesemeister et al. (11) and Nakic et al. (19), supporting the idea of an amygdala involvement in implicit emotion processing. According to Nakic et al. (19), amygdala activity indicates the processing of emotional salience, and regions relevant for behavioral responses such as the medial orbito-frontal gyrus and the anterior cingulate cortex receive input from the amygdala, thereby facilitating behavioral lexical decision responses. In support of this proposal, we observed an interaction of discrete emotion and affective dimension driven by higher activity in response to high fear words with negative valence compared to low fear words with negative valence in the right amygdala. In line with this pattern of activity, response times revealed a significant main effect of fear, driven by faster response times for high fear words compared to low fear words, and an interaction of discrete emotion and affective dimensional processing, driven by faster response times for low fear words with negative valence compared to low fear words with neutral valence. These behavioral interactions support Nakic et al. (19) findings, of a correlation between amygdala and anterior cingulate cortex activity in conditions showing enhanced word processing speed for negative words. Activity in the right inferior frontal gyrus revealed a main effect of negativity, driven by higher activity for negative compared to neutral words, which confirms Briesemeister et al's. results, and are line with the suggestion of the hierarchical emotion model of explicit evaluation of emotional salient stimuli on tertiary levels lending further support to Panksepp's proposal, that tertiary level processes “require expansive neocortical tissues that permit linguistic-symbolic transformations” (88). Thus, activity in the inferior frontal gyrus could reflect increased computational demands within the so-called reading network underlying semantic evaluation and integration processes of emotional information (20, 89–91).
However, the interaction of discrete emotion and affective dimensional processing in the amygdala in the current study is in contrast with Briesemeister's double dissociation of discrete emotion and affective dimension. Thus, it further challenges the amygdala's role in emotion processing. Briesemeister et al. (10, 11) suggested that activity in the inferior frontal gyrus in response to the affective dimension of positivity and the activity in the amygdala in response to the discrete emotion happiness reflect the dissociation of discrete emotion and affective dimensional processing in the brain. However, as the hierarchical emotion model focuses on primary process level emotions, the amygdala's role – as a potential secondary level process – is not yet clear, and the current results do not necessarily contradict the hierarchical emotion model. The observed activity in the amygdala could be based on the processing of biological relevant information and could reflect the interaction of top-down affective dimensional and bottom-up discrete emotion information carried by conditioned affective stimuli such as words (20, 92, 93).
The absence of significant differences in brain activity and response times between patients with JME and healthy controls during implicit emotion processing could be interpreted in favor of the notion that emotion processing in reading operates rather independently of other cognitive domains, as previously reported in emotional face recognition (94). However, these results were somewhat unexpected, as patients with JME were reported to present structural and functional differences in frontal regions, as well as neuropsychological deficits, related to executive functions (47). These results might be attributable to the implicit nature of the lexical decision paradigm and could point to independent processing of emotional information in reading, despite known altered explicit executive functioning in JME (37, 39, 40, 47). In addition, this result could suggest rather unaffected implicit emotion processing in patients with JME and could emphasize the importance of a differentiation of the implicit and explicit nature of tasks used in studying emotion processing, considering that most tasks used are of explicit nature (25–27, 29, 95–98). Error rates revealed a significant main effect of negativity, driven by higher error rates in response to negative as compared to neutral words, and an interaction, showing higher error rates in response to words with high fear values and with negative valence compared to words with high fear values and neutral valence. Further, we found a statistically significant difference in error rates between healthy controls and patients with JME. However, as the total number of errors was 3.19% (healthy controls) and 5.99% (patients with JME), respectively, we interpret this to be in a normal range and carefully interpret this difference to be due to impaired cognitive functions, as revealed by the results of the neuropsychological testing.
Neuropsychology revealed slower reading-speed, lower semantic and phonematic verbal fluency, and reduced verbal crystalline intelligence in patients with JME. Slower reading speed and comprehension extends on previous results found in patients with temporal lobe epilepsy and idiopathic epilepsies in children (99–101). Deficits in verbal fluency in our patients confirm existing results in patients with JME, pointing to disturbed executive processing supporting epilepsy-specific patterns of neuropsychological dysfunctions (99, 102). Reduced verbal intelligence might be interpreted as a constitute of reading ability and verbal executive functioning, as overall intelligence level is usually normal in this patient group (103, 104). Additionally, we found no significant association between psychiatric conditions, type of seizure disorder or epilepsy duration and the outcome of emotional word processing in the lexical decision task.
This study has some limitations related to the interpretation of neuropsychological data and brain activity in response to the emotional content of words. First, as psychiatric disorders in JME patients are reported to be increased, a special focus on the type of psychiatric disorder and a direct comparison between those may unveil additional information (105). For simplicity, we only controlled statistically for psychiatric disorders (yes or no) within our groups and their effect on affective processing. Second, as argued by Hamann (106), our fMRI-results might reveal deeper insights into brain mechanisms of implicit affective processing if we were to have presented more types of emotions than only fear/negativity in our paradigm. There are, however, some strengths that we like to highlight. This study validates and replicates the rationale of a previous study (11), using a different set of emotions. Due to our implicit task design and with the inclusion of patients with JME, we were able to unveil unaffected implicit affective processing in this patient group. To our knowledge, this is the first study to report results of implicit affective processing in this patient group, as most studies use paradigms which focus on explicit emotion processing (26, 29, 97, 98) (e.g., emotion recognition).
In this study, we showed an interaction of discrete emotion and affective dimensional processing in the right amygdala. This interaction was driven by higher activity in response to words with high fear values and negative valence, compared to words with low fear values and negative valence. We interpreted this activity to reflect the interaction of top-down and bottom-up circuits of primary and tertiary process levels in the amygdala, which is suggested to be involved in the conditioning of emotional stimuli in general. Higher activity in the right inferior frontal gyrus for negative words is in line with the results of Briesemeister et al. and was interpreted to signal explicit semantic evaluation and emotional memory integration on the tertiary process level. The results of the neuropsychological testing revealed subtle deficits in reading speed, phonematic and semantic fluency, and verbal intelligence in patients with JME compared to healthy controls. However, this did not result in any behavioral differences concerning discrete emotion and affective dimensional processing of patients with JME and healthy controls. Thus, the results of the current study could point to unimpaired implicit emotion processing of patients with JME during lexical decisions to words carrying emotional information.
Data Availability Statement
The datasets presented in this article are not readily available because The decision of the Ethics Committee of the state of Salzburg does not include the sharing of the data. Requests to access the datasets should be directed to LR, bHVjYXMucmFpbmVyQHN0dWQuc2JnLmFjLmF0.
Ethics Statement
The studies involving human participants were reviewed and approved by Salzburg Ethics Committee. Written informed consent to participate in this study was provided by the participants' legal guardian/next of kin.
Author Contributions
LR, JH, GK, and MB contributed significantly to conception and design of the presented paper, acquisition, analysis and interpretation of the data as well as drafting of the paper. ES, PL, LK, SS-Y, MKi, MKr, GZ, and ET contributed to acquisition and analysis of data and revising the paper for intellectual content. GK, JH, MB, and ET contributed significantly to conception of the study, interpretation of the results and gave final approval of the submitted version of the manuscript. All authors contributed to the article and approved the submitted version.
Funding
The presented research was funded by the FWF, Austrian Science Fund (Project Number: KLI 543 B-27).
Conflict of Interest
ET reports personal fees from EVER Pharma, Marinus, Argenix, Arvelle/Angelini, Medtronic, Bial – Portela, S.A., NewBridge, GL Pharma, GlaxoSmithKline, Hikma, Boehringer Ingelheim, LivaNova, Eisai, UCB, Biogen, Genzyme Sanofi, GW Pharmaceuticals, and Actavis outside the submitted work; his institution received grants from Biogen, UCB Pharma, Eisai, Red Bull, Merck, Bayer, the European Union, FWF Osterreichischer Fond zur Wissenschaftsforderung, Bundesministerium fr Wissenschaft und Forschung, and Jubilaumsfond der sterreichischen Nationalbank outside the submitted work. GZ gratefully acknowledges the support of the WISS 2025 project; IDA-Lab Salzburg (20204-WISS/225/197-2019 and 20102-F1901166-KZP).
The remaining authors declare that the research was conducted in the absence of any commercial or financial relationships that could be construed as a potential conflict of interest.
Publisher's Note
All claims expressed in this article are solely those of the authors and do not necessarily represent those of their affiliated organizations, or those of the publisher, the editors and the reviewers. Any product that may be evaluated in this article, or claim that may be made by its manufacturer, is not guaranteed or endorsed by the publisher.
Acknowledgments
This work is generated within the European Reference Network for Rare and Complex Diseases EpiCARE.
Supplementary Material
The Supplementary Material for this article can be found online at: https://www.frontiersin.org/articles/10.3389/fneur.2022.875950/full#supplementary-material
References
1. Fritsch N, Kuchinke L. Acquired affective associations induce emotion effects in word recognition: an ERP study. Brain Lang. (2013) 124:75–83. doi: 10.1016/j.bandl.2012.12.001
2. Lindquist KA, Wager TD, Kober H, Bliss-Moreau E, Barrett LF. The brain basis of emotion: a meta-analytic review. Behav Brain Sci. (2012) 35:121. doi: 10.1017/S0140525X11000446
3. Darwin C. The Expression of the Emotions in Man and Animals. Chicago, IL: University of Chicago Press. (2015).
4. Ekman P. An argument for basic emotions. Cogn Emot. (1992) 6:169–200. doi: 10.1080/02699939208411068
5. Panksepp J. Affective Neuroscience: the Foundations of Human and Animal Emotions. Oxford: Oxford University Press. (2004).
6. Elfenbein HA. Non-verbal dialects and accents in facial expressions of emotion. Emotion Review. (2013) 5:90–6. doi: 10.1177/1754073912451332
8. Russell JA. Core affect and the psychological construction of emotion. Psychol Rev. (2003) 110:145. doi: 10.1037/0033-295X.110.1.145
9. Barrett LF. Are emotions natural kinds? Perspect Psychol Sci. (2006) 1:28–58. doi: 10.1111/j.1745-6916.2006.00003.x
10. Briesemeister BB, Kuchinke L, Jacobs AM. Emotion word recognition: discrete information effects first, continuous later? Brain Res. (2014) 1564:62–71. doi: 10.1016/j.brainres.2014.03.045
11. Briesemeister BB, Kuchinke L, Jacobs AM, Braun M. Emotions in reading: dissociation of happiness and positivity. Cog, Affect, Behavioral Neurosci. (2015) 15:287–98. doi: 10.3758/s13415-014-0327-2
12. Panksepp J, Biven L. The Archaeology of Mind: Neuroevolutionary Origins of Human Emotions (Norton Series on Interpersonal Neurobiology). New York, NY: WW Norton & Company. (2012).
13. Panksepp J. What is an emotional feeling? Lessons about affective origins from cross-species neuroscience. Moti Emo. (2012) 36:4–15. doi: 10.1007/s11031-011-9232-y
14. Panksepp J. Neurologizing the psychology of affects: how appraisal-based constructivism and basic emotion theory can coexist. Perspect Psychol Science. (2007) 2:281–96. doi: 10.1111/j.1745-6916.2007.00045.x
15. LeDoux JE. Emotion circuits in the brain. Annu Rev Neurosci. (2000) 23:155–84. doi: 10.1146/annurev.neuro.23.1.155
16. Maren S, Phan KL, Liberzon I. The contextual brain: implications for fear conditioning, extinction and psychopathology. Nature Rev Neurosci. (2013) 14:417–28. doi: 10.1038/nrn3492
17. Panskepp J. The Core Emotional Systems of the Mammalian Brain: the Fundamental Substrates of Human Conditions. About a Body: Working With the Embodied Mind in Psychotherapy. London: Routledge. (2006).
18. Panksepp J, Watt D. What is basic about basic emotions? Lasting lessons from affective neuroscience. Emotion review. (2011) 3:387–96. doi: 10.1177/1754073911410741
19. Nakic M, Smith BW, Busis S, Vythilingam M, Blair RJR. The impact of affect and frequency on lexical decision: the role of the amygdala and inferior frontal cortex. Neuroimage. (2006) 31:1752–61. doi: 10.1016/j.neuroimage.2006.02.022
20. Kuchinke L, Jacobs AM, Grubich C, Vo ML-H, Conrad M, Herrmann M. Incidental effects of emotional valence in single word processing: an fMRI study. Neuroimage. (2005) 28:1022–32. doi: 10.1016/j.neuroimage.2005.06.050
21. Herbert C, Ethofer T, Anders S, Junghofer M, Wildgruber D, Grodd W. Amygdala activation during reading of emotional adjectives Grodd W. alence in single word pr. Soc Cog Affect Neurosci. (2009) 4:35–49. doi: 10.1093/scan/nsn027
22. Citron FM. Neural correlates of written emotion word processing: a review of recent electrophysiological and hemodynamic neuroimaging studies. Brain Lang. (2012) 122:211–26. doi: 10.1016/j.bandl.2011.12.007
23. Schlochtermeier LH, Kuchinke L, Pehrs C, Urton K, Kappelhoff H, Jacobs AM. Emotional picture and word processing: an fMRI study on effects of stimulus complexity. PLoS ONE. (2013) 8:e55619. doi: 10.1371/journal.pone.0055619
24. Meletti S, Benuzzi F, Rubboli G, Cantalupo G, Maserati MS, Nichelli P. Impaired facial emotion recognition in early-onset right mesial temporal lobe epilepsy. Neurology. (2003) 60:426–31. doi: 10.1212/WNL.60.3.426
25. Meletti S, Benuzzi F, Cantalupo G, Rubboli G, Tassinari CA, Nichelli P. Facial emotion recognition impairment in chronic temporal lobe epilepsy. Epilepsia. (2009) 50:1547–59. doi: 10.1111/j.1528-1167.2008.01978.x
26. Monti G, Meletti S. Emotion recognition in temporal lobe epilepsy: a systematic review. Neurosci Biobehav Rev. (2015) 55:280–93. doi: 10.1016/j.neubiorev.2015.05.009
27. Broicher SD, Kuchukhidze G, Grunwald T, Kr temporal loben M, Jokeit H. Tell me how do I feel–ell me how do I feeldze G, Grunwald T, Kr temporal loben M, Jokmporal lobe epilepsy. Neuropsychologia. (2012) 50:118–28. doi: 10.1016/j.neuropsychologia.2011.11.005
28. Tanaka A, Akamatsu N, Yamano M, Nakagawa M, Kawamura M, Tsuji S, et al. more realistic approach, using dynamic stimuli, to test facial emotion recognition impairment in temporal lobe epilepsy. Epilepsy Behav. (2013) 28:12–6. doi: 10.1016/j.yebeh.2013.03.022
29. Kuchukhidze G, Höfler J, Kronbichler M, Schmid E, Kirschner M, Rainer L. Emotion recognition and social cognition in juvenile myoclonic epilepsy. Zeitschrift fnitioileptologie. (2019) 32:177–82. doi: 10.1007/s10309-019-0261-y
30. Szaflarski JP, Allendorfer JB, Heyse H, Mendoza L, Szaflarski BA, Cohen N. Functional MRI of facial emotion processing in left temporal lobe epilepsy. Epilepsy & Behavior. (2014) 32:92–9. doi: 10.1016/j.yebeh.2014.01.012
31. MacAllister WS, Schaffer SG. Neuropsychological deficits in childhood epilepsy syndromes. Neuropsychol Rev. (2007) 17:427–44. doi: 10.1007/s11065-007-9048-4
32. Zifkin B, Andermann E, Andermann F. Mechanisms, genetics, and pathogenesis of juvenile myoclonic epilepsy. Curr Opin Neurol. (2005) 18:147–53. doi: 10.1097/01.wco.0000162856.75391.b1
33. Janz D. Epilepsy with impulsive petit mal (juvenile myoclonic epilepsy). Acta Neurol Scand. (1985) 72:449–59. doi: 10.1111/j.1600-0404.1985.tb00900.x
34. Larch J, Unterberger I, Bauer G, Reichsoellner J, Kuchukhidze G, Trinka E. Myoclonic status epilepticus in juvenile myoclonic epilepsy. Epileptic Disorders. (2009) 11:309–14. doi: 10.1684/epd.2009.0284
35. Höfler J, Unterberger I, Dobesberger J, Kuchukhidze G, Walser G, Trinka E. Seizure outcome in 175 patients with juvenile myoclonic epilepsy-a long-term observational study. Epilepsy Res. (2014) 108:1817–24.
36. Reichsoellner J, Larch J, Unterberger I, Dobesberger J, Kuchukhidze G, Luef G. Idiopathic generalised epilepsy of late onset: a separate nosological entity? J Neurol Neurosurg Psych. (2010) 81:1218–22. doi: 10.1136/jnnp.2009.176651
37. Motamedi M, Nasergivehch S, Karamghadiri N, Noroozian M. Juvenile myoclonic epilepsy (JME): neuropsychological profile and related factors with cognitive dysfunction. Iran J Psychiatry. (2014) 9:14.
38. Pascalicchio TF, de Araujo Filho GM, da Silva Noffs MH, Lin K, Caboclo LOS, Vidal-Dourado M. Neuropsychological profile of patients with juvenile myoclonic epilepsy: a controlled study of 50 patients. Epilepsy Behav. (2007) 10:263–7. doi: 10.1016/j.yebeh.2006.11.012
39. Iqbal N, Caswell H, Muir R, Cadden A, Ferguson S, Mackenzie H. Neuropsychological profiles of patients with juvenile myoclonic epilepsy and their siblings: an extended study. Epilepsia. (2015) 56:1301–8. doi: 10.1111/epi.13061
40. Zamarian L, Höfler J, Kuchukhidze G, Delazer M, Bonatti E, Kemmler G. Decision making in juvenile myoclonic epilepsy. J Neurol. (2013) 260:839–46. doi: 10.1007/s00415-012-6715-z
41. Trinka E, Kienpointner G, Unterberger I, Luef G, Bauer G, Doering LB. Psychiatric comorbidity in juvenile myoclonic epilepsy. Epilepsia. (2006) 47:2086–91. doi: 10.1111/j.1528-1167.2006.00828.x
42. Moschetta S, Fiore LA, Fuentes D, Gois J, Valente KD. Personality traits in patients with juvenile myoclonic epilepsy. Epilepsy Behav. (2011) 21:473–7. doi: 10.1016/j.yebeh.2011.03.036
43. de Araújo Filho GM, Pascalicchio TF, Lin K, Guilhoto LMF, Yacubian EMT. Psychiatric disorders in juvenile myoclonic epilepsy: a controlled study of 100 patients. Epilepsy Behav. (2007) 10:437–41. doi: 10.1016/j.yebeh.2007.01.016
44. de Araujo Filho GM, Lin K, Lin J, Peruchi MM, Caboclo LOS, Guaranha MS. Are personality traits of juvenile myoclonic epilepsy related to frontal lobe dysfunctions? A proton MRS study. Epilepsia. (2009) 50:1201–9. doi: 10.1111/j.1528-1167.2009.02021.x
45. de Ara-1167oton MRS study juvenile myoclonic epilepsy related to ha MS. 100 patPersonality traits related to juvenile myoclonic epilepsy: MRI reveals prefrontal abnormalities through a voxel-based morphometry study. Epilepsy Behav. (2009) 15:202–7. doi: 10.1016/j.yebeh.2009.03.011
46. de Ara1jo Filho GM, Jackowski AP, Lin K, Silva I, Guaranha MS, Guilhoto LM. The integrity of corpus callosum and cluster B personality disorders: a quantitative MRI study in juvenile myoclonic epilepsy. Prog Neuro-Psychopharmacol Biol Psychiatry. (2010) 34:516–21. doi: 10.1016/j.pnpbp.2010.02.009
47. Wandschneider B, Thompson PJ, Vollmar C, Koepp MJ. Frontal lobe function and structure in juvenile myoclonic epilepsy: a comprehensive review of neuropsychological and imaging data. Epilepsia. (2012) 53:2091–8. doi: 10.1111/epi.12003
48. Lin K, Carrete H Jr, Lin J, Peruchi MM, De Araújo Filho GM, Guaranha MSB. Magnetic resonance spectroscopy reveals an epileptic network in juvenile myoclonic epilepsy. Epilepsia. (2009) 50:1191–200. doi: 10.1111/j.1528-1167.2008.01948.x
49. Ronan L, Alhusaini S, Scanlon C, Doherty CP, Delanty N, Fitzsimons M. Widespread cortical morphologic changes in juvenile myoclonic epilepsy: evidence from structural MRI. Epilepsia. (2012) 53:651–8. doi: 10.1111/j.1528-1167.2012.03413.x
50. Wong M. Juvenile myoclonic epilepsy: is it an idiopathic epilepsy caused by a malformation of cortical development? Epilepsy currents. (2010) 10:69–71. doi: 10.1111/j.1535-7511.2010.01360.x
51. Martínez M, Masakazu S, Munari C, Porter R, Roger J, Wolf P. Commission on classification and terminology of the international league against epilepsy. Proposal for revised classification of epilepsies and epileptic syndromes. Epilepsia. (1989) 30:389–99.
52. First MB. Structured Clinical Interview for DSM-IV Axis I Disorders. New York, NY: Biometrics Research Department (1997).
53. First MB, Gibbon M, Spitzer RL, Williams JB, Benjamin LS. Structured Clinical Interview for DSM-IV®Axis II Personality Disorders SCID-II. New York, NY: American Psychiatric Pub (1997).
54. Wittchen HU, Zaudig M, Fydrich T, Skid. Strukturiertes klinisches Interview für DSM-IV. Achse I und II. Handanweisung. New York, NY: American Psychiactric Publication (1997).
55. Lehrl S, Triebig G, Fischer B. Multiple choice vocabulary test MWT as a valid and short test to estimate premorbid intelligence. Acta Neurol Scand. (1995) 91:335–45. doi: 10.1111/j.1600-0404.1995.tb07018.x
56. Lehrl S. Manual zum MWT-B: Mehrfachwahl-Wortschatz-Intelligenztest, 5., unveränd. Spitta: Aufl Spitta-Verl, Balingen. (2005).
57. Aschenbrenner S, Tucha O, Lange KW. Regensburger Wortflcha O, Lange KW: RWT. Hogrefe: Verlag fger Wortflcha. (2000).
58. Helmstaedter C, Durwen HF. VLMT: Verbaler Lern-und Merkfähigkeitstest: Ein praktikables und differenziertes Instrumentarium zur Prüfung der verbalen Gedächtnisleistungen. Schweizer Archiv für Neurologie, Neurochirurgie und Psychiatrie. Göttingen: Hogrefe (1990).
59. Helmstaedter C, Lendt M, Lux S. Verbaler Lern-und Merkf, Lux S. tes : VLMT; Manual. Weinheim: Beltz-test. (2001).
60. Schmidt M. Rey Auditory Verbal Learning Test: A Handbook, Vol. 17). Los Angeles, CA: Western Psychological Services (1996).
61. Ashburner J, A. fast diffeomorphic image registration algorithm. Neuroimage. (2007) 38:95–113. doi: 10.1016/j.neuroimage.2007.07.007
62. Bergmann J, Wimmer H, A. dual-route perspective on poor reading in a regular orthography: evidence from phonological and orthographic lexical decisions. Cogn Neuropsychol. (2008) 25:653–76. doi: 10.1080/02643290802221404
63. Coltheart M, Besner D, Jonasson JT, Davelaar E. Phonological encoding in the lexical decision task. Q J Exp Psychol. (1979) 31:489–507. doi: 10.1080/14640747908400741
64. Dehaene S, Cohen L. The unique role of the visual word form area in reading. Trends Cogn Sci. (2011) 15:254–62. doi: 10.1016/j.tics.2011.04.003
65. Kronbichler M, Bergmann J, Hutzler F, Staffen W, Mair A, Ladurner G. Taxi vs. taksi: on orthographic word recognition in the left ventral occipitotemporal cortex. J Cog Nurosci. (2007) 19:1584–94. doi: 10.1162/jocn.2007.19.10.1584
66. Kronbichler M, Hutzler F, Wimmer H, Mair A, Staffen W, Ladurner G. The visual word form area and the frequency with which words are encountered: evidence from a parametric fMRI study. Neuroimage. (2004) 21:946–53. doi: 10.1016/j.neuroimage.2003.10.021
67. Vo ML, Conrad M, Kuchinke L, Urton K, Hofmann MJ, Jacobs AM. The Berlin affective word list reloaded (BAWL-R). Behav Res Methods. (2009) 41:534–8. doi: 10.3758/BRM.41.2.534
68. Briesemeister BB, Kuchinke L, Jacobs AM. Discrete emotion norms for nouns: Berlin affective word list (DENNacobs AMBehav Res Methods. (2011) 43:441–8. doi: 10.3758/s13428-011-0059-y
69. Briesemeister BB, Kuchinke L, Jacobs AM. Emotional valence: a bipolar continuum or two independent dimensions? Sage Open. (2012) 2:2158244012466558. doi: 10.1177/2158244012466558
70. Cambridge Research Systems. BOLDscreen 32 LCD for fMRI [Internet] (2012). Verfügbar unter: Available online at: http://www.crsltd.com/tools-for-functional-imaging/mr-safe-displays/boldscreen-32-lcd-for-fmri/nest/boldscreen-32-technical-specification#npm
72. Brainard DH, Vision S. The psychophysics toolbox. Spat Vis. (1997) 10:433–6. doi: 10.1163/156856897X00357
73. Peirce JW. PsychoPy—psychophysics software in Python. J Neurosci Methods. (2007) 162:8–13. doi: 10.1016/j.jneumeth.2006.11.017
74. Harms MP, Somerville LH, Ances BM, Andersson J, Barch DM, Bastiani M. Extending the human connectome project across ages: imaging protocols for the lifespan development and aging projects. Neuroimage. (2018) 183:972–84. doi: 10.1016/j.neuroimage.2018.09.060
75. Ashburner J, Barnes G, Chen CC, Daunizeau J, Flandin G, Friston K. SPM12 manual. London: Wellcome Trust Centre for Neuroimaging. (2014).
76. Cox RW, AFNI. software for analysis and visualization of functional magnetic resonance neuroimages. Comp Biomed Res. (1996) 29:162–73. doi: 10.1006/cbmr.1996.0014
77. Gaser C, Dahnke R. CAT-a computational anatomy toolbox for the analysis of structural MRI data. Hbm. (2016) 2016:336–48.
78. Friston KJ, Glaser DE, Henson RN, Kiebel S, Phillips C, Ashburner J. Classical and Bayesian inference in neuroimaging: applications. Neuroimage. (2002) 16:484–512. doi: 10.1006/nimg.2002.1091
79. Duff EP, Cunnington R, Egan GF, REX. response exploration for neuroimaging datasets. Neuroinformatics. (2007) 5:223–34. doi: 10.1007/s12021-007-9001-y
82. Noguchi K, Gel YR, Brunner E, Konietschke F. nparLD: an R software package for the nonparametric analysis of longitudinal data in factorial experiments. J Stat Software. (2012) 50:2. doi: 10.18637/jss.v050.i12
83. Gunawardana A, Konietschke F. Nonparametric multiple contrast tests for general multivariate factorial designs. J Multivar Anal. (2019) 173:165–80. doi: 10.1016/j.jmva.2019.02.016
85. Pinheiro J, Bates D, DebRoy S, Sarkar DR. Core Team NLME: Linear and non-Linear Mixed Effects Models. R package version 3.1-153. Vienna: CRAN (2021).
86. Benjamini Y, Yekutieli D. The control of the false discovery rate in multiple testing under dependency. Annals Stat. (2001) 1165–88. doi: 10.1214/aos/1013699998
87. Ponz A, Montant M, Liegeois-Chauvel C, Silva C, Braun M, Jacobs AM. Emotion processing in words: a test of the neural re-use hypothesis using surface and intracranial EEG. Soc Cogn Affect Neurosci. (2014) 9:619–27. doi: 10.1093/scan/nst034
88. Panksepp J. Affective consciousness: core emotional feelings in animals and humans. Conscious Cogn. (2005) 14:30–80. doi: 10.1016/j.concog.2004.10.004
89. Binder JR, Desai RH, Graves WW, Conant LL. Where is the semantic system? A critical review and meta-analysis of 120 functional neuroimaging studies. Cereb Cort. (2009) 19:2767–96. doi: 10.1093/cercor/bhp055
90. Binder JR, Desai RH. The neurobiology of semantic memory. Trends Cogn Sci. (2011) 15:527–36. doi: 10.1016/j.tics.2011.10.001
91. Kutas M, Federmeier KD. 30 years and counting: finding meaning in the N400 component of the event-related brain potential (ERP). Annu Rev Psychol. (2011) 62:621–47. doi: 10.1146/annurev.psych.093008.131123
92. Pessoa L, Adolphs R. Emotion processing and the amygdala: from adiestem? surface and intracrependency. neuroimaging: applicatiNat Rev Neurosci. (2010) 11:773–82. doi: 10.1038/nrn2920
93. Anderson AK, Phelps EA. Lesions of the human amygdala impair enhanced perception of emotionally salient events. Nature. (2001) 411:305–9. doi: 10.1038/35077083
94. Tracy JL, Robins RW. The automaticity of emotion recognition. Emotion. (2008) 8:81. doi: 10.1037/1528-3542.8.1.81
95. Bauer J, Kegel LC, Steiger BK, Jokeit H. Assessment tools for social cognition in epilepsy. Zeitschrift fl LC, Steiger BK. (2019) 32:183–6. doi: 10.1007/s10309-019-0260-z
96. Jokeit H, Eicher M, Ives-Deliperi V. Toward social neuropsychology of epilepsy: a review on social cognition in epilepsy. Acta Epilepsy. (2018) 1:8–17.
97. Gomez-Ibañez A, Urrestarazu E, Viteri C. Recognition of facial emotions and identity in patients with mesial temporal lobe and idiopathic generalized epilepsy: an eye-tracking study. Seizure. (2014) 23:892–8.
98. Jiang Y, Hu Y, Wang Y, Zhou N, Zhu L, Wang K. Empathy and emotion recognition in patients with idiopathic generalized epilepsy. Epilepsy & Behavior. (2014) 37:139–44. doi: 10.1016/j.yebeh.2014.06.005
99. Chaix Y, Laguitton V, Lauwers-Cances V, Daquin G, Cances C, Démonet JF. Reading abilities and cognitive functions of children with epilepsy: influence of epileptic syndrome. Brain Develop. (2006) 28:122–30. doi: 10.1016/j.braindev.2005.06.004
100. German4 E, Gagliano A, Arena C, Cedro C, Vetri L, Operto FF. Readingliano A, Arena C, Cedro C, Vetri L, Operto FF. epilep. Epilepsy Behav. (2020) 111:107118. doi: 10.1016/j.yebeh.2020.107118
101. Lah S, Castles A, Smith ML. Reading in children with temporal lobe epilepsy: a systematic review. Epilepsy Behav. (2017) 68:84y Behavview. 1016/j.yebeh.2016.12.021
102. Chowdhury FA, Elwes RD, Koutroumanidis M, Morris RG, Nashef L, Richardson MP. Impaired cognitive function in idiopathic generalized epilepsy and unaffected family members: an epilepsy endophenotype. Epilepsia. (2014) 55:835amily members1111/epi.12604
103. Kim SY, Hwang YH, Lee HW, Suh CK, Kwon SH, Park SP. Cognitive impairment in juvenile myoclonic epilepsy. J Clin Neurol. (2007) 3:86Neuroljuvenil3988/jcn.2007.3.2.86
104. Piazzini A, Turner K, Vignoli A, Canger R, Canevini MP. Frontal cognitive dysfunction in juvenile myoclonic epilepsy. Epilepsia. (2008) 49:657aive dysfunct1111/j.1528-1167.2007.01482.x
105. Anderson IM, Shippen C, Juhasz G, Chase D, Thomas E, Downey D. State-dependent alteration in face emotion recognition in depression. Br J Psych. (2011) 198:302endent alter1192/bjp.bp.110.078139
Keywords: discrete emotion, dimensional emotion, neuropsychology, juvenile myoclonic epilepsy (JME), implicit emotion processing
Citation: Rainer LJ, Kronbichler M, Kuchukhidze G, Trinka E, Langthaler PB, Kronbichler L, Said-Yuerekli S, Kirschner M, Zimmermann G, Höfler J, Schmid E and Braun M (2022) Emotional Word Processing in Patients With Juvenile Myoclonic Epilepsy. Front. Neurol. 13:875950. doi: 10.3389/fneur.2022.875950
Received: 14 February 2022; Accepted: 10 May 2022;
Published: 01 June 2022.
Edited by:
Julia Jacobs, University of Freiburg Medical Center, GermanyReviewed by:
Kette D. Valente, University of São Paulo, BrazilPatricia Rzezak, University of São Paulo, Brazil
Copyright © 2022 Rainer, Kronbichler, Kuchukhidze, Trinka, Langthaler, Kronbichler, Said-Yuerekli, Kirschner, Zimmermann, Höfler, Schmid and Braun. This is an open-access article distributed under the terms of the Creative Commons Attribution License (CC BY). The use, distribution or reproduction in other forums is permitted, provided the original author(s) and the copyright owner(s) are credited and that the original publication in this journal is cited, in accordance with accepted academic practice. No use, distribution or reproduction is permitted which does not comply with these terms.
*Correspondence: Lucas Johannes Rainer, bHVjYXMucmFpbmVyQHN0dWQuc2JnLmFjLmF0; bC5yYWluZXJAc2Fsay5hdA==