- 1Clinical Neurology Unit, Santa Maria della Misericordia University Hospital, Udine, Italy
- 2Department of Medical Area (DAME), University of Udine, Udine, Italy
- 3Neurology Unit, Santa Maria della Misericordia University Hospital, Udine, Italy
Introduction: Migraine and sleep share a complex and unclear relationship. Poor sleep may trigger migraine attacks; migraine, in turn, is frequently associated with sleep disorders. Few previous studies used questionnaires to assess sleep changes in patients who were treated with migraine-preventive medications (MPMs). More extensive polysomnography (PSG)-based studies for this purpose were not available.
Objective: To investigate possible sleep changes in patients with migraine treated with erenumab, using validated sleep questionnaires and home-PSG.
Methods: This observational, prospective, open-label pilot study was conducted at the Clinical Neurology Unit Headache Center of Udine University Hospital from 2020 to 2021. Patients were treated with erenumab as monotherapy or add-on treatment for migraine prevention. Sleep changes were evaluated with questionnaires and polysomnographic recordings at baseline, after 3 and 12 months of treatment. Erenumab efficacy and safety in migraine prophylaxis were also investigated.
Results: Twenty-nine patients completed 3 months of follow-up, whereas 15 patients completed 12 months. We found a weak trend of improvement in daytime somnolence after 3 months of treatment, with stronger results after 12 months (median Epworth Sleepiness Scale (ESS) score from 6.0 to 4.0, p = 0.015); a significant improvement in subjective sleep quality (median Pittsburgh Sleep Quality Index (PSQI) total score from 7 to 5; p = 0.001) was also observed. Home-PSG showed a significant increase in objective sleep efficiency (SE), both after 3 (from 88.1 to 91.0, p = 0.006) and 12 months (from 87.1 to 91.0, p = 0.006) of treatment. In addition, our data confirmed erenumab effectiveness and safety in migraine prevention.
Conclusion: Our study demonstrated an improvement in both subjective and objective sleep quality in patients treated with a migraine-preventive therapy. Erenumab, in particular, does not cross the blood-brain barrier (BBB), thus a direct effect on sleep is unlikely. Future studies are needed to better understand the mutual influence between migraine and sleep disorders.
Introduction
Migraine is a common disabling primary headache disorder with high socio-economic burden and personal impact. It typically occurs with recurrent attacks of pulsating quality and unilateral location, associated with autonomic symptoms, such as nausea, photophobia, and phonophobia, as defined by the International Classification of Headache Disorders, 3rd edition (ICHD-III) (1). Migraine etiology and pathophysiological mechanisms are complex and not fully understood. It is well-known that various triggers may precipitate migraine attacks, such as changes in lifestyle, physical activity, diet, and hormones and sleep disturbances (1, 2).
Increasing evidence suggests that sleep and migraine share a multifaceted and bidirectional relationship. Patients with migraine frequently report poor sleep quality and excessive daytime sleepiness (EDS). On the other hand, sleep disruption can be a trigger of migraine attacks, which often benefit of sufficient restful sleep (3–6). In clinical settings, physicians ordinarily consider sleep as one aspect of migraine treatment, selecting medications with sedative side effects, in an attempt to improve sleep as a protective factor against migraine attacks.
Several polysomnographic studies identified a decreased sleep efficiency (SE) and alterations in sleep architecture in patients with migraine when compared to healthy subjects (7–9). Furthermore, among adult patients with migraine, a high prevalence of sleep disorders has been found and can range from insomnia to more specific disorders, such as Restless Legs Syndrome (RLS) and parasomnias (10–15). Together, these findings suggest to consider migraine and sleep problems as conditions with common pathophysiologic elements that still are partly obscure. Few previous studies evaluated sleep changes in patients treated with migraine-preventive treatments by means of questionnaires (16–19). For example, a recent study investigated possible sleep changes in patients with chronic migraine effectively treated with a greater occipital nerve block, showing a significant improvement in scores of different clinical questionnaires evaluating sleep after 3 months of treatment (19). However, more extensive clinical and polysomnographic trials for this purpose are not currently available.
In the last years, the development of new monoclonal antibodies toward calcitonin gene-related peptide (CGRP) or its receptor has opened an innovative therapeutic scenario. Erenumab, in particular, was the first anti-CGRP receptor antibody employed in the clinical practice for migraine prevention (20).
The aim of the present study was to investigate possible sleep changes in patients with episodic and chronic migraine treated with erenumab, assessed with validated sleep questionnaires and polysomnography (PSG) performed at baseline, after 3 and 12 months of treatment.
Methods
Study Design, Eligibility Criteria, and Ethical Approval
This observational, prospective, open-label pilot study was performed at the Clinical Neurology Unit Headache Center of Udine University Hospital (Udine, Italy) between 2020 and 2021. It included patients treated with erenumab for the first time, prescribed on clinical indication as monotherapy or add-on treatment to other migraine-preventive medications (MPMs). Enrolled patients fulfilled all the established inclusion criteria and none of the exclusion criteria is reported in Table 1. This study was evaluated and approved by the Regional Ethics Committee of Friuli Venezia Giulia, Italy (approval code: CEUR-2020-OS-230) and was conducted in accordance with the Declaration of Helsinki code of ethics and the Good Clinical Practice guidelines. All patients included in the study provided written informed consent. The authors confirm full access to all anonymized study data.
Data Collection
Interview and Clinical Examination
The following socio-demographic characteristics and relevant clinical data were collected: age, gender, body mass index, major comorbidities, current medications, age at onset of migraine, family history of migraine, number, and type of previous and ongoing MPMs. Enrolled patients underwent neurological examinations that were performed by an expert team of specialists in headache disorders, at the baseline visit (T0) and during the follow-up after 3 (T1) and 12 months (T2) of treatment with erenumab. At each visit, detailed clinical characteristics of migraine were collected, such as frequency and intensity of attacks, pain quality and localization, presence of aura, associated vegetative symptoms, migraine triggers, and classes and number per month of symptomatic treatments used. We also evaluated, at T1 and T2 follow-up visits, how many patients reported a subjective improvement in attack duration and response to symptomatic medications after taking erenumab, measured as a dichotomous variable (yes/no). In addition, we collected data about any side effects reported during the treatment.
Headache Diary and Questionnaires
Patients were requested to fill out a headache diary for 2 months before the enrollment and throughout the entire study period to evaluate changes in frequency and severity of migraine attacks during the treatment. Migraine frequency was expressed as monthly migraine days (MMDs), while the severity of pain was a 3-point score (1 = mild, 2 = moderate, and 3 = severe). For each patient, migraine frequency at baseline was defined as the mean of MMDs during the 2 months preceding the enrollment. After 3 and 12 months of treatment, it was defined as the mean of MMDs in the month preceding T1 and in the 2 months preceding T2, respectively. Patients were considered as responders if they reported ≥50% reduction in migraine frequency.
All patients also completed a series of questionnaires collected via face-to-face interviews at baseline, T1, and T2 follow-up visits. We used the Pittsburgh Sleep Quality Index (PSQI) and the Epworth Sleepiness Scale (ESS) (22, 23), aimed at exploring subjective sleep quality and daytime sleepiness, respectively. Disability and impact on daily living associated with migraine were evaluated using both the Migraine Impact and Disability Assessment Scale (MIDAS) and the Headache Impact Test, 6th edition (HIT-6) (24, 25). Additionally, we included the Beck Depression Inventory-II questionnaire (BDI-II) and the EuroQoL Visual Analogue Scale (EQ-VAS) in order to rate depressive symptoms and subjective quality of life, respectively (26, 27).
Home-PSG
Enrolled patients underwent three home-PSG recording sessions at times T0, T1, and T2. All recordings were performed for two subsequent nights. For our comparisons, we used all second-night recordings in order to limit the possible “first-night effect,” due to the discomfort caused by electrodes and other measurement devices (28, 29). Sleep recordings were performed with a battery-powered self-applicable device positioned on the forehead (Sleep ProfilerTM, Advanced Brain Monitoring, Carlsbad, CA, USA). PSG recordings were uploaded to the Sleep ProfilerTM software and were initially analyzed by the automated scoring system. Finally, two different sleep specialists visually inspected each analysis, minimally editing as appropriate, to confirm staging accuracy. Further detailed information on Sleep ProfilerTM hardware and software, automated scoring system, and reliability of results in comparison with laboratory-PSG has been previously published (see Supplementary Materials) (30–32).
Erenumab Treatment
All included patients met the eligibility criteria established by the European Headache Federation and the American Headache Society for the treatment with new monoclonal antibodies acting on the CGRP pathway (33, 34). Patients with migraine and coexistent medication overuse headache (MOH) were not detoxified prior to start erenumab treatment. All subjects received the first dose of erenumab 70 mg administered subcutaneously after completing a baseline PSG recording. All patients continued the treatment at a dose of 70 mg monthly for the first 3 months of the study, followed by a second home-PSG. During that period, any other ongoing MPM was not discontinued or modified. From the fourth month, a dose switch to 140 mg monthly was proposed according to treatment effectiveness and tolerability; modifying or withdrawing concurrent oral MPMs was also allowed according to the physician's judgment. The concomitant inoculation of botulinum neurotoxin type A (BoNT-A) was not permitted in any case. All patients received a monthly treatment with erenumab for 52 weeks, then completed the study with the third home-PSG.
Study Endpoints
We defined a primary composite endpoint, such as variations in SE (I) derived from PSG (SE, %), in subjective sleep quality (II) measured by the PSQI score, and in EDS (III) assessed with the ESS score. SE ≥ 85%, PSQI score ≤ 5, and ESS score ≤ 10 were considered as normal thresholds (22, 23, 35). We compared all parameters collected at times T0, T1, and T2.
As secondary endpoints, we established the following issues: (i) changes in other PSG parameters, such as total sleep time (TST), duration of wake (W) and N1, N2, N3, and Rapid Eye Movement (REM) sleep stages, wake after sleep onset (WASO), sleep latency (SL), REM latency (RL), arousal index (number of arousal per hour, Ar/h), and awakening index (number of awakenings per hour, Aw/h); (ii) effects on migraine-related disability, measured by MIDAS and HIT-6 scales (24, 25); (iii) effects on mood, tested with the BDI-II questionnaire (26); (iv) subjective changes in life quality, assessed with the EQ-VAS (27). Finally, as erenumab has been recently approved and is still subject to additional monitoring by the regulatory agencies, we evaluated treatment effectiveness (v) and tolerability (vi), by using information from clinical interviews, headache diaries, and collecting patient-reported adverse events (AEs).
Statistical Analysis
All data were collected in an ad hoc created database (Excel 2019, Microsoft Corp., Redmond, WA, USA). Data cleaning was performed before the data analysis. Continuous variables were summarized by descriptive statistics, expressed as median, interquartile interval, and range, and categorical variables by absolute frequencies and percentages. Migraine parameters, sleep parameters, and sleep scores were compared using Fisher's and McNemar's tests for categorical data and Wilcoxon signed-rank test for continuous data, with the threshold of significance of p < 0.05. All statistical analyses were performed using SPSS version 25.0 (IBM Corporation, Chicago, IL, USA).
Results
Patient Characteristics
A total of 29 patients met the study inclusion criteria and completed the follow-up after 3 months of treatment. Fourteen patients were lost at follow-up because of limited access to hospital services due to the Coronavirus Disease-19 (COVID-19) pandemic, whereas 15 patients (51.7%) completed a 12-month follow-up visit. For this reason, statistical analyses were conducted separately for data collected at 3 months on the whole sample (n = 29) and 3 and 12 months on a subgroup of patients (n = 15).
Patients included were predominantly women (86.2%), with a median age of 46.1 years (range 19–61) at baseline. All patients presented a long clinical history of the disease, mostly with chronic migraine (65.5%), refractory to different classes of MPMs (median 4.0, range 2–10). Thirteen patients (44.8%) with chronic migraine had also failed previous treatment with BoNT-A injection. In 17 of 29 patients (58.6%), erenumab was prescribed in an add-on to other ongoing oral MPMs. The main baseline demographic and clinical characteristics of patients are reported in detail in Table 2.
Migraine Severity, Disability, Mood Level, and Life Quality
Considering the entire study sample (n = 29), eight patients (27.6%) showed a significant ≥50% reduction of MMD (p = 0.001) and were considered responders at 3 months of follow-up. At baseline, 27.6% of patients reported the subjective severity of migraine attack as “severe,” while 69.0% of them as “moderate.” After 3 months of treatment, we found a significant improvement in subjective attack severity; in fact, only 17.2% of patients described the migraine attack as “severe,” whereas patients with “mild” attacks were increased from 3.4% up to 37.9%. Moreover, 69.0% of the patients reported a subjective reduction in the duration of the single migraine attack, and 65.5% an improvement in response to symptomatic drugs (see Table 3).
The subgroup of patients at 12 months of follow-up (n = 15) showed a significant reduction from baseline in MMD and in subjective severity of migraine attacks (p = 0.001 and p = 0.002, respectively). Moreover, the proportion of patients reporting a reduction in duration of attacks was increased up to 73.3%. During the 12-month treatment period, 12 of 15 patients (78.5%) escalated the erenumab dose from 70 to 140 mg, and 11 (73.3%) withdrew the prior oral MPM because of erenumab effectiveness (see Table 4).
In the whole sample (n = 29), the median MIDAS total score was 45.0 at baseline, which improved to 24.0 after 3 months of follow-up. A ≥50% reduction of MIDAS score was achieved by 41.4% of patients. Considering the subgroup of 15 patients, a clinically relevant improvement in MIDAS score was confirmed both at 3- and 12-month follow-up visits. HIT-6 score also improved significantly after 3 months, with a 3-point reduction in 29 patients (p = 0.0001). Among 15 patients, changes from baseline in HIT-6 scores were – 4.0 after 3 months (p = 0.001) and – 6.0 after 12 months (p = 0.001). Lastly, we found a significant change in the median of BDI and EQ-VAS scale scores (p = 0.003 and p = 0.001, respectively) at a 3-month follow-up on 29 patients. Among the subgroup of 15 patients, the median scores on BDI and EQ-VAS scale were confirmed significantly improved from baseline, both to 3 and 12 months of follow-up (see Tables 3, 4).
All patients showed an optimal compliance to the treatment. The most common AE was constipation, reported by six patients (20.7%); other AEs were observed in isolated cases, particularly asthenia (3.4%), irritability (3.4%), and Raynaud's phenomenon (3.4%). All AEs were reported as mild; none of the patients discontinued the treatment because of side effects.
Subjective and Objective Sleep Quality Parameters
Sleep Questionnaires
At baseline, six (20.7%) of all enrolled patients (n = 29) had pathological ESS scores (>10). After 3 months of treatment, we observed pathological scores in only three subjects. None of the patients with 1 year of follow-up (n = 15) showed abnormal ESS values (Figure 1). Median ESS values showed a weak trend of improvement in levels of daytime somnolence after 3 months of treatment, with stronger results in the sub-analysis on 15 patients at 12-month follow-up. In this subgroup, there was a significant reduction in the median ESS score (from 6.0 to 4.0, p = 0.015). The median baseline total PSQI score was 7.0 and 17 patients (58.6 %) had scores >6, indicating a global poor sleep quality. After 1 year, we found a significant change (from 7 to 5; p = 0.001) in the median PSQI total score (see Tables 5, 6).
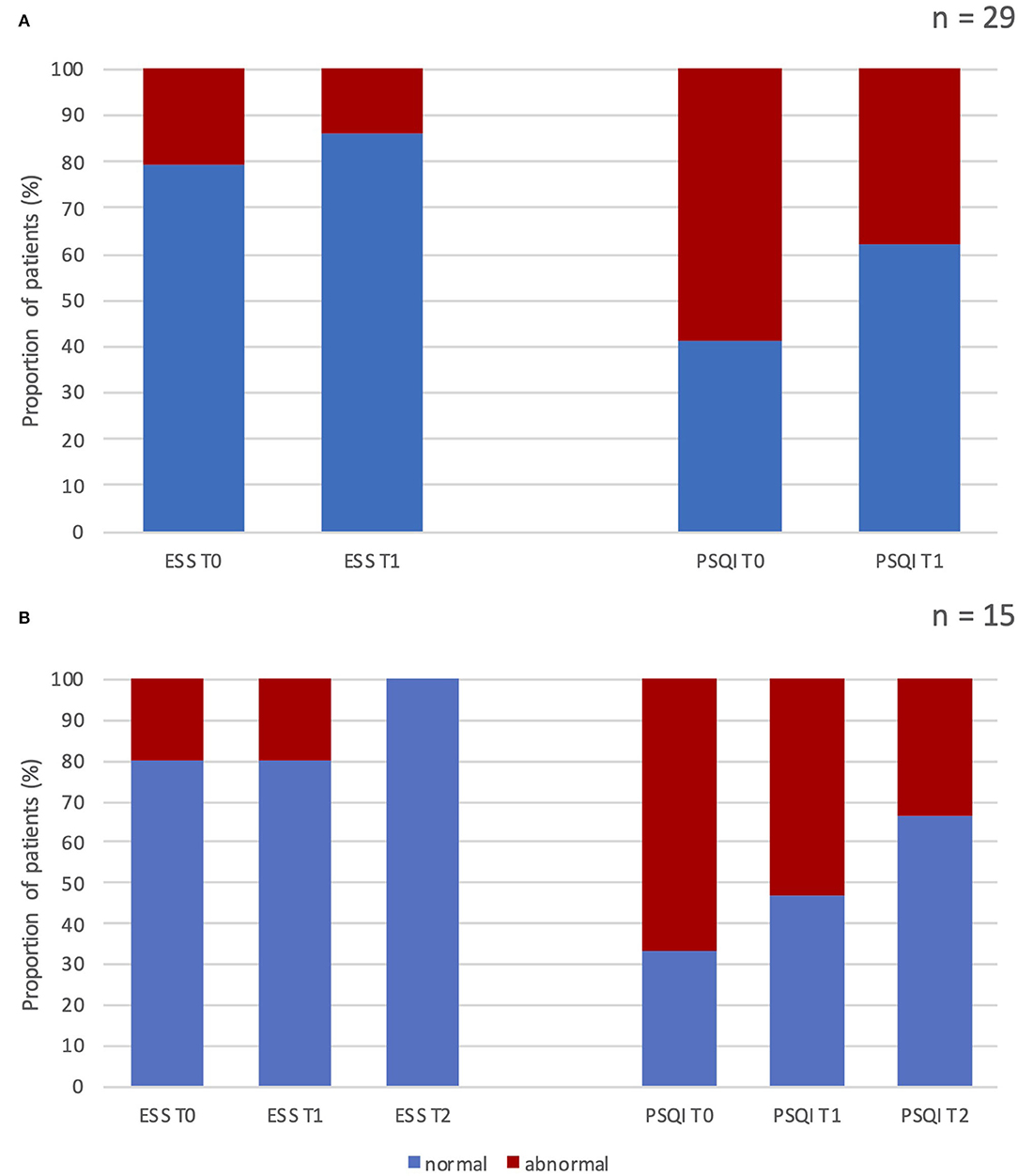
Figure 1. Percentage of patients with normal vs. abnormal scores at the Epworth Sleepiness Scale and Pittsburgh Sleep Quality Index before and after erenumab treatment. (A) Results after 3 months of treatment in all patients enrolled (n = 29). (B) Results after 3 and 12 months of treatment in patients who completed the 12-month follow-up (n = 15). ESS, Epworth Sleepiness Scale; PSQI, Pittsburgh Sleep Quality Index; T0, score at baseline; T1, score after 3 months of treatment; T2, score after 12 months of treatment; n, number of patients.
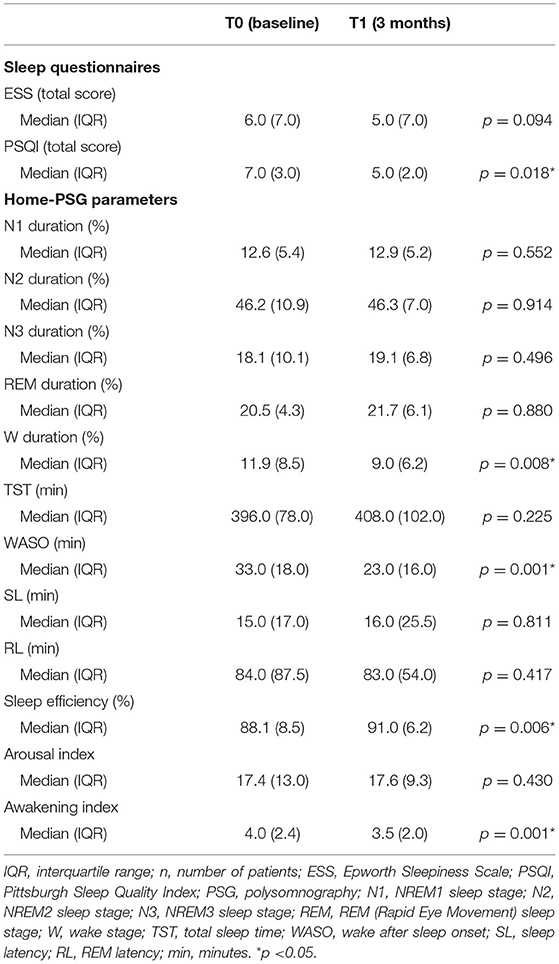
Table 5. Results of sleep questionnaires and home-polysomnography recordings at baseline and after 3 months of treatment with erenumab (n = 29).
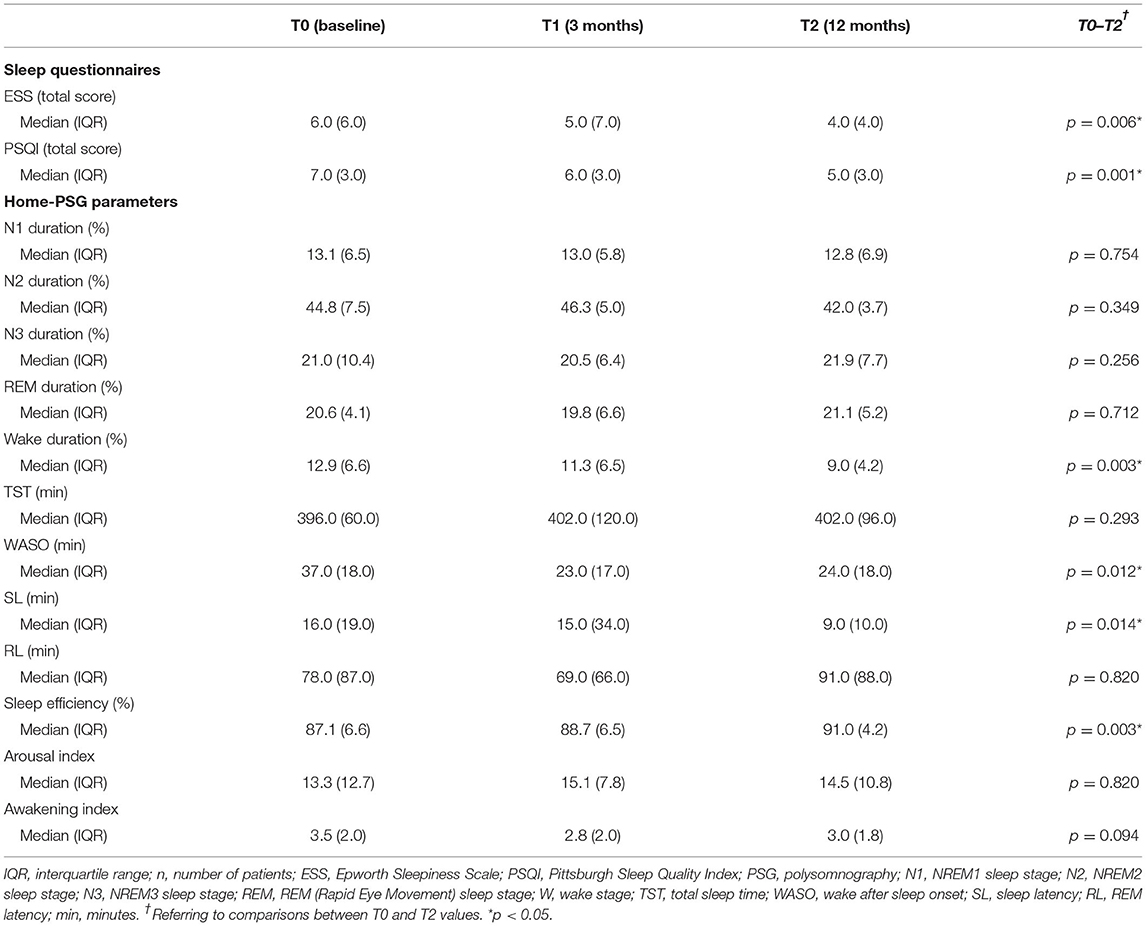
Table 6. Results of sleep questionnaires and home-polysomnography recordings at baseline and after 12 months of treatment with erenumab (n = 15).
Ten subjects (66.7%) had PSQI abnormal score at baseline, whereas the 53.3% and 33% of patients showed altered PSQI total score after 3 and 12 months of treatment, respectively (see Figure 1).
Each PSQI component (such as subjective sleep quality, SL, sleep duration, habitual SE, sleep disturbances, and daytime dysfunction) was also analyzed separately comparing the distribution of scores (from a minimum of 0 = normal to a maximum of 3 = severe alteration) among patients at baseline, 3 months, and 12 months of follow-up (see Figures 2, 3).
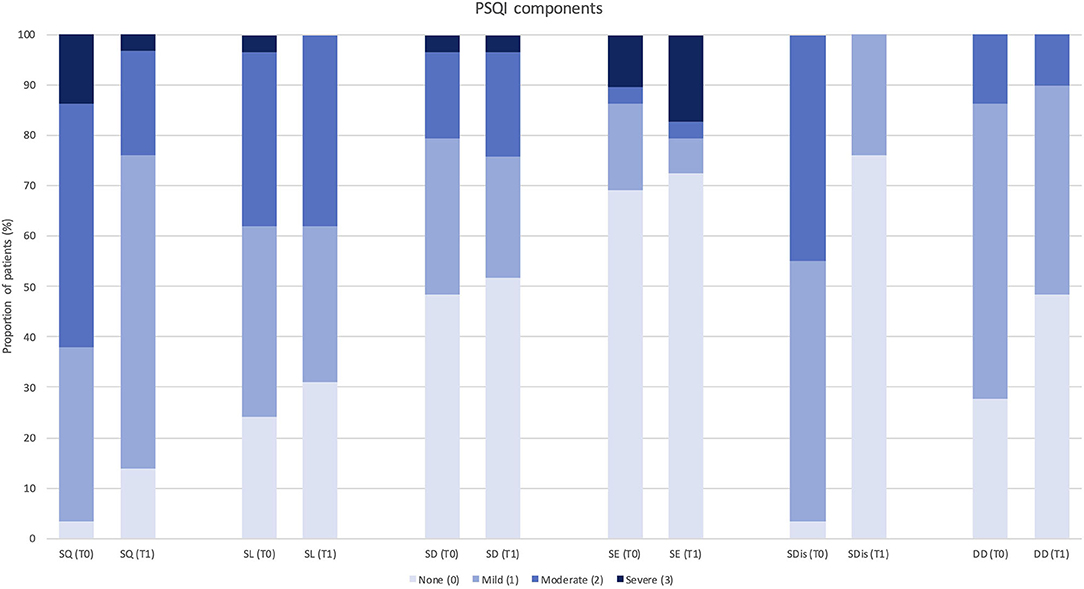
Figure 2. Change in Pittsburgh Sleep Quality Index component score after 3 months of treatment with erenumab (n = 29). PSQI, Pittsburgh Sleep Quality Index; SQ, subjective sleep quality; SL, sleep latency; SD, sleep duration; SE, habitual sleep efficiency; SDis, sleep disturbances; DD, daytime dysfunction; T0, score at baseline; T1, score after 3 months of treatment.
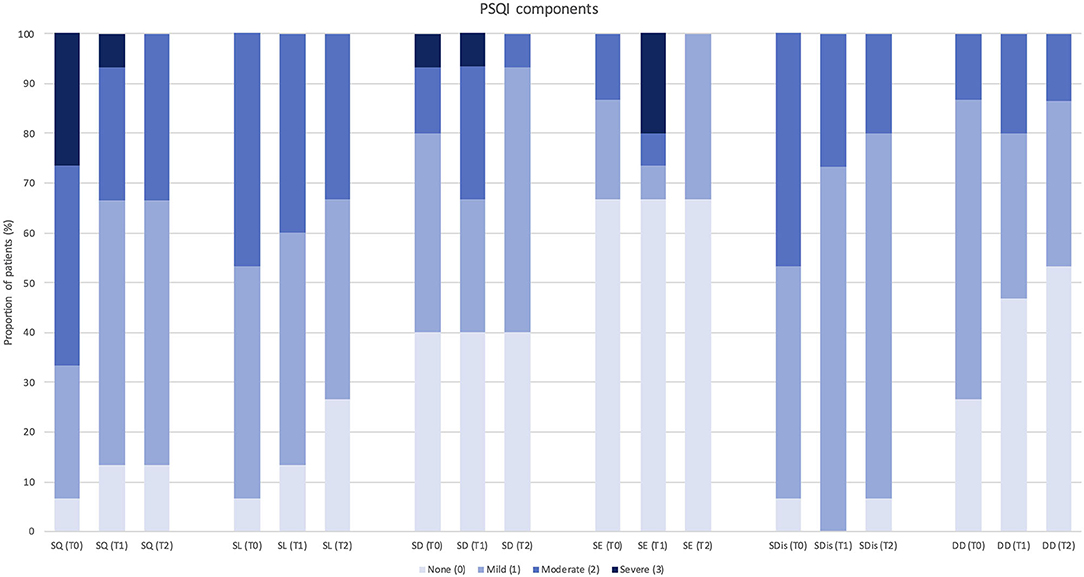
Figure 3. Change in each Pittsburgh Sleep Quality Index component score after 12 months of treatment with erenumab (n = 15). PSQI, Pittsburgh Sleep Quality Index; SQ, subjective sleep quality; SL, sleep latency; SD, sleep duration; SE, habitual sleep efficiency; SDis, sleep disturbances; DD, daytime dysfunction; T0, score at baseline; T1, score after 3 months of treatment; T2, score after 12 months of treatment.
Despite a global trend of improvement in single PSQI component scores, significant changes were observed in “subjective sleep quality” (p = 0.023) and “sleep disturbances” (p = 0.034) at 3-month follow-up (n = 29), and in “daytime dysfunction” (p = 0.046) at 12-month follow-up (n = 15). Only one PSQI component (i.e., use of sleeping medications) was not assessed, as none of the patients was using hypnotic drugs according to study exclusion criteria.
Home-PSG
Objective SE was significantly increased after erenumab treatment, both at 3-month (from 88.1 to 91.0, p = 0.006) and 12-month (from 87.1 to 91.0, p = 0.006) follow-up visits on 29 and 15 patients, respectively. This outcome was related to the improvement of some PSG parameters, specifically W (%), WASO, and Aw/h. After 3 months (n = 29), median W percentage was changed from 11.9 to 9.0 (p = 0.008), median WASO from 33.0 to 23.0 min (p = 0.001), and median Aw/h from 4.0 to 3.5 (p = 0.001) (see Table 5).
These results were confirmed at 12-month follow-up on 15 patients, particularly the reduction in median W percentage (p = 0.003) and WASO (p = 0.012); change in median Aw/h did not reach the statistical significance (p = 0.094), whereas median SL was significantly decreased from 16.0 to 9.0 min (p = 0.014) (see Table 6).
Interestingly, we found a weak positive correlation between several parameters related to migraine improvement (i.e., reduction in MMD, MIDAS score, severity, and duration of attacks) and the increase in objective SE, both after 3 and 12 months of treatment with erenumab (see Supplementary Table 2). This trend became statistically significant only for attacks severity at T1.
Sleep distribution in N1, N2, N3, and REM sleep stages was similar at baseline and at times T1 and T2. Similarly, we did not find significant changes in other PSG parameters, such as TST, RL, and Ar/h, during the follow-up (see Tables 5, 6).
Discussion
To our knowledge, this is the first polysomnographic study that evaluated objective sleep changes in patients who were treated with MPMs. A recent meta-analysis that includes trials based on PSQI and PSG demonstrated poorer sleep quality and a lower percentage of REM sleep in patients with migraine when compared to healthy controls (9). In our patients, we found a higher SE after the treatment with erenumab. This result was obtained through a significant reduction of wake time duration, WASO, and number of awakenings per hour, with unaltered TST and duration of different sleep stages, indicating a higher sleep stability after erenumab treatment. Particularly, we observed a weak positive relationship between the improvement in objective SE and a better response to the treatment with erenumab. This association, however, did not reach a clear statistical significance probably due to the limited sample size. Subjective measures of sleep quality (PSQI and ESS questionnaires) corroborated home-PSG data. Our patients reported to sleep better after only 3 months of treatment, reaching a significant decrease in EDS and daytime dysfunction after 12 months.
Duman et al. used for the first time the PSQI questionnaire and testing the subjective effects of migraine prophylaxis on sleep (16). In that study, patients were treated with propranolol or amitriptyline for 12 weeks, after which a significant improvement in sleep quality was observed in both groups, with greater results in those with initial PSQI scores ≥6 taking amitriptyline. These molecules exert central effects on sleep architecture by reducing REM sleep. In particular, it is well-known the sleep-promoting role of amitriptyline by modulating sleep-wake cycle neurotransmitters (36–38). Erenumab, in contrast, does not cross the blood-brain barrier (BBB) (39, 40), and thus a direct action on sleep central pathways is not expected. For this reason, the observed sleep changes can be attributed to migraine improvement itself. In fact, our PSG results occurred concurrently with erenumab effectiveness in reducing migraine frequency, severity, duration of attacks and improving the response to symptomatic medications and the disability related to migraine attacks. We also observed a significant improvement in patients' mood and self-reported quality of life. In this respect, our data are consistent with the increasing amount of literature demonstrating erenumab success in migraine prevention, with concurrent benefits on living standards and mood (17, 18, 20, 41–44). Real-life data support erenumab safety and tolerability. We detected infrequent and mild AEs, obtaining full adherence to the treatment. Compared to information in the Summary of Product Characteristics (45), we found a higher frequency of constipation (20.7%); however, none of the patients dropped out by reason of side effects, and constipation tended to dissipate over time, as described elsewhere (41, 42, 46).
Erenumab was the first monoclonal antibody approved by the U.S. Food Drug Administration and the European Medicines Agency in May 2018 for the treatment of chronic and episodic migraine (45, 47). Its efficacy is mainly attributed to the blockage of CGRP receptors expressed in the trigeminal system. CGRP is a vasoactive neuropeptide released from trigeminal ganglia cells at the level of intracranial cerebral and dural blood vessels, causing vasodilation and neurogenic inflammation and playing an important role in sustaining a state of central sensitization during migraine attacks (39, 40, 48).
Migraine and sleep share a complex bidirectional relationship, which still remains poorly understood. At least in part, they have common anatomic structures, neural pathways, signaling neurotransmitters, and neuropeptides. Both the brainstem and the hypothalamus play a central role in migraine and sleep-wake cycle regulation, particularly involving dopamine, serotonin, adenosine, melatonin, orexin, and pituitary adenylate cyclase-activating polypeptide (PACAP) as possible mediators of this relationship (49–51).
Patients with migraine were found with low serotonin levels during interictal periods and high mobilization of serotonin intracellular stores in the early phase of the attack, promoting wakefulness, disrupted sleep, and REM sleep reduction (52, 53). Instead, inhibition of serotoninergic neurons of the dorsal raphe nucleus in the brainstem is essential for the transition from NREM to REM sleep (54). Dopaminergic A11 hypothalamic nucleus, implicated in the prodromal symptoms of migraine, facilitates arousals and wakefulness and could explain the high prevalence of RLS in migraine patients (51). The dopamine neurons of the periaqueductal gray are also involved in wake promotion by inhibiting the hypothalamus, and in pain signaling between the trigeminocervical complex (TCC), the hypothalamus, and the thalamus. Noradrenergic neurons in the locus coeruleus work in concert with dopaminergic and serotoninergic systems of the brainstem as pain modulators and facilitate wakefulness (50, 55–57). Moreover, a possible association between the therapeutic effect of sleep in migraine and the glymphatic system (a little-known waste removal mechanism working during sleep in the central nervous system) has been hypothesized; its dysfunction has been also proposed as a possible mechanism in the development of chronic migraine (49, 58).
Finally, PACAP is emerging as an important molecular target in the pathophysiology of migraine. Similar to CGRP, it works on the nociceptive neurons of the TCC promoting vasodilation and increased cerebral blood flow during migraine attacks (59, 60). Recent evidence suggests that PACAP also plays an important role in the hypothalamus for sleep homeostasis. In animal models, it seems to act on sleep-related neuroendocrine changes, through the modulation of sleep-wake cycle pathway, clock genes expression, and melatonin synthesis (61–65). Despite current knowledge, the pathogenic correlation between regulation of sleep, migraine, and circadian rhythm remains largely unclear. PACAP and CGRP represent significant migraine triggers, but little is known about their circadian variability. Again, nothing is known about possible indirect central effects due to peripheral modulation of CGRP with erenumab or other anti-CGRP monoclonal antibodies.
This study has several limitations. The first one is represented by the small size of the sample, due to the strict enrollment criteria, and the even smaller size of the group that completed the 12-month follow-up. Sample reduction at 12 months was determined randomly, mainly because of limited hospital access during the COVID-19 pandemic and, rather, it was not influenced by factors such as patients' sleep quality or therapeutic response to erenumab. Despite some disadvantages when compared to laboratory recordings, home-PSG was a more cost-effective alternative, easy to perform during the COVID-19 pandemic, and enabled patients to sleep in their habitual setting. Another issue is the absence of a control group in the study design, although it has to be considered that since erenumab does not pass the BBB, we studied the impact of migraine improvement on sleep, rather than the direct effect of the drug on patients' sleep. Moreover, we are aware of the fact that a subgroup of patients continued to take other MPMs with a potential influence on sleep during the treatment with erenumab (i.e., antidepressants, antihistamines, and beta-blockers). In order to prevent a possible interference of these drugs on our results, however, other MPMs were not modified during the 2 months preceding the enrollment and for the following 3 months. Interestingly, during the second study period, we confirmed a global improvement in sleep quality although antidepressants had been discontinued in several cases. This gives more strength to the positive effect of erenumab on sleep, since this effect was obtained despite the withdrawal of other sleep-promoting drugs. One last point to consider is that most of the patients selected, due to the enrollment criteria, already had a quite normal SE at baseline. This precondition explains the mild but significant extent of results, since an impressive change in sleep quality was not expected.
Future studies on larger groups will be needed in order to perform further analyses, for instance, to evaluate whether there is a linear correlation between migraine improvement and sleep changes, or whether results are influenced by the treatment dose or the type of monoclonal antibody used. Explaining common pathophysiologic mechanisms between migraine, sleep homeostasis, and sleep disorders still remains very challenging.
In conclusion, erenumab is effective and safe in migraine prevention, demonstrating consequent benefits on both subjective sleep quality and sleep architecture. After starting the treatment, our patients significantly increased their SE, resulting in better life quality, improved daytime functioning, and reduced somnolence levels. Our findings support the growing body of evidence suggesting a common undelying pathophysiology for migraine and sleep disorders. Basic research into neuropeptides and their pathways appear to be crucial to improve our understanding of this fascinating relationship and developing new therapeutic solutions.
Data Availability Statement
The raw data supporting the conclusions of this article will be made available by the authors, without undue reservation.
Ethics Statement
The studies involving human participants were reviewed and approved by Comitato Etico Unico Regionale, Friuli Venezia Giulia (CEUR FVG), Italy. The patients/participants provided their written informed consent to participate in this study.
Author Contributions
GP, SP, and MV conceived the study and its design. GP, SP, and AS performed the acquisition and interpretation of data. GP and SP drafted the manuscript. AN contributed to the analysis and interpretation of data. AN, AS, GG, and MV substantively revised the manuscript. All authors made substantial contributions to conception, study design, data collection, and approved the final manuscript.
Conflict of Interest
GP and SP received travel grants from Novartis and Eli Lilly. AS received travel grants from Novartis. MV received travel grants, honoraria for scientific contributions or clinical investigation studies from Novartis, Teva Pharmaceuticals, and Eli Lilly.
The remaining authors declare that the research was conducted in the absence of any commercial or financial relationships that could be construed as a potential conflict of interest.
Publisher's Note
All claims expressed in this article are solely those of the authors and do not necessarily represent those of their affiliated organizations, or those of the publisher, the editors and the reviewers. Any product that may be evaluated in this article, or claim that may be made by its manufacturer, is not guaranteed or endorsed by the publisher.
Supplementary Material
The Supplementary Material for this article can be found online at: https://www.frontiersin.org/articles/10.3389/fneur.2022.869677/full#supplementary-material
Abbreviations
ICHD-III, International Classification of Headache Disorders, 3rd edition; EDS, excessive daytime sleepiness; SE, sleep efficiency; RLS, restless legs syndrome; MPM, migraine-preventive medication; CGRP, calcitonin gene-related peptide; PSG, polysomnography; MMD, monthly migraine days; PSQI, Pittsburgh Sleep Quality Index; ESS, Epworth Sleepiness Scale; MIDAS, Migraine Impact and Disability Assessment Scale; HIT-6, Headache Impact Test, 6th edition; BDI-II, Beck Depression Inventory-II; EQ-VAS, EuroQoL Visual Analogue Scale; MOH, medication overuse headache; BoNT-A, botulinum neurotoxin type A; TST, total sleep time; REM, rapid eye movement; WASO, wake after sleep onset; SL, sleep latency; RL, REM latency; AE, adverse event; BBB, blood-brain barrier; PACAP, pituitary adenylate cyclase-activating polypeptide; TCC, trigeminocervical complex.
References
1. Headache Classification Committee of the International Headache Society (IHS). The international classification of headache disorders, 3rd Edition. Cephalalgia. (2018) 38:1–211. doi: 10.1177/0333102417738202
3. Seidel S, Hartl T, Weber M, Matterey S, Paul A, Riederer F, et al. Quality of sleep, fatigue and daytime sleepiness in migraine—A controlled study. Cephalalgia. (2009) 29:662–9. doi: 10.1111/j.1468-2982.2008.01784.x
4. Karthik N, Kulkarni GB, Taly AB, Rao S, Sinha S. Sleep disturbances in ‘migraine without aura' — A questionnaire based study. J Neurol Sci. (2012) 321:73–6. doi: 10.1016/j.jns.2012.07.057
5. Sadeghniiat K, Rajabzadeh A, Ghajarzadeh M, Ghafarpour M. Sleep quality and depression among patients with migraine. Acta Med Iran. (2013) 51:784–8.
6. Waliszewska-Prosół M, Nowakowska-Kotas M, Chojdak-Łukasiewicz J, Budrewicz S. Migraine and sleep—An unexplained association? Int J Mol Sci. (2021) 22:5539. doi: 10.3390/ijms22115539
7. Nayak C, Sinha S, Nagappa M, Nagaraj K, Kulkarni GB, Thennarasu K, et al. Study of sleep microstructure in patients of migraine without aura. Sleep Breath. (2016) 20:263–9. doi: 10.1007/s11325-015-1207-x
8. Karthik N, Sinha S, Taly AB, Kulkarni GB, Ramachandraiah CT, Rao S. Alteration in polysomnographic profile in ‘migraine without aura' compared to healthy controls. Sleep Med. (2013) 14:211–4. doi: 10.1016/j.sleep.2012.10.019
9. Stanyer EC, Creeney H, Nesbitt AD, Robert Holland PR, Hoffmann J. Subjective sleep quality and sleep architecture in patients with migraine: a meta-analysis. Neurology. (2021) 97:e1620–e1631. doi: 10.1212/WNL.0000000000012701
10. Yang C-P, Wang S-J. Sleep in patients with chronic migraine. Curr Pain Headache Rep. (2017) 21:39. doi: 10.1007/s11916-017-0641-9
11. Acar BA, Acar T, Alagöz AN, Karacan A, Varim C, Uyanik MS, et al. Relationship between primary restless legs syndrome and migraine with aura. Kaohsiung J Med Sci. (2016) 32:420–6. doi: 10.1016/j.kjms.2016.06.003
12. Schürks M, Winter A, Berger K, Kurth T. Migraine and restless legs syndrome: a systematic review. Cephalalgia. (2014) 34:777–94. doi: 10.1177/0333102414537725
13. Guilleminault C, Palombini L, Pelayo R, Chervin RD. Sleepwalking and sleep terrors in prepubertal children: what triggers them? Pediatrics. (2003) 111:e17–e25. doi: 10.1542/peds.111.1.e17
14. Bruni O, Russo P, Violani C, Guidetti V. Sleep and migraine: an actigraphic study. Cephalalgia. (2004) 24:134–9. doi: 10.1111/j.1468-2982.2004.00657.x
15. Valente M, Janes F, Russo V, Fontana A, Travanut A, Sommaro M, et al. Prevalence of restless legs syndrome in migraine patients: a case-control study. Analysis of Risk Factors for Restless Legs Syndrome in Migraine Patients. Headache. (2017) 57:1088–95. doi: 10.1111/head.13124
16. Duman T, Dede OH, Uluduz D, Seydaoglu G, Okuyucu E, Melek I. Sleep changes during prophylactic treatment of migraine. Ann Indian Acad Neurol. (2015) 18:298–302. doi: 10.4103/0972-2327.160084
17. Russo A, Silvestro M, Scotto di Clemente F, Trojsi F, Bisecco A, Bonavita S, et al. Multidimensional assessment of the effects of erenumab in chronic migraine patients with previous unsuccessful preventive treatments: a comprehensive real-world experience. J Headache Pain. (2020) 21:69. doi: 10.1186/s10194-020-01143-0
18. de Luca C, Baldacci F, Mazzucchi S, Lombardo I, Curto L, Ulivi M, et al. CGRP Inhibitors and oxidative stress biomarkers in resistant migraine: a real-life study with Erenumab, Fremanezumab, and Galcanezumab. J Clin Med. (2021) 10:4586. doi: 10.3390/jcm10194586
19. Saçmaci H, Aktürk T, Tanik N, Inan LE. Clinical success of greater occipital nerve blockade in improving sleep quality of chronic migraineurs: a prospective observational study. Sleep Breath. (2021) 25:1003–10. doi: 10.1007/s11325-021-02309-z
20. Dodick DW. CGRP ligand and receptor monoclonal antibodies for migraine prevention: evidence review and clinical implications. Cephalalgia. (2019) 39:445–58. doi: 10.1177/0333102418821662
21. American Academy of Sleep Medicine. The International Classification of Sleep Disorders, Third Edition (ICSD-3). (2014).
22. Johns MW. A new method for measuring daytime sleepiness: the epworth sleepiness scale. Sleep. (1991) 14:540–5. doi: 10.1093/sleep/14.6.540
23. Buysse DJ, Reynolds CF III, Monk TH, Berman SR, Kupfer DJ. The Pittsburgh sleep quality index: a new instrument for psychiatric practice and research. Psychiatry Res. (1989) 28:193–213. doi: 10.1016/0165-1781(89)90047-4
24. Kosinski M, Bayliss MS, Bjorner JB, Ware JE Jr, Garber WH, Batenhorst A, et al. A six-item short-form survey for measuring headache impact: the HIT-6. Qual Life Res. (2003) 12:963–74. doi: 10.1023/A:1026119331193
25. Stewart WF, Lipton RB, Dowson AJ, Sawyer J. Development and testing of the Migraine Disability Assessment (MIDAS) questionnaire to assess headache-related disability. Neurology. (2001) 56:S20–S28. doi: 10.1212/WNL.56.suppl_1.S20
26. Beck A, Steer R, Brown GK. Manual for the Beck Depression Inventory-II. San Antonio, TX: The Psychological Corporation (1996). doi: 10.1037/t00742-000
27. EuroQol Group. EuroQol - a new facility for the measurement of health-related quality of life. Health Policy. (1990) 16:199–208. doi: 10.1016/0168-8510(90)90421-9
28. Miettinen T, Myllymaa K, Hukkanen T, Töyräs J, Sipilä K, Myllymaa S. Home polysomnography reveals a first-night effect in patients with low sleep bruxism activity. J Clin Sleep Med. (2018) 14:1377–86. doi: 10.5664/jcsm.7278
29. Veauthier C, Piper SK, Gaede G, Penzel T, Paul F. The first night effect in multiple sclerosis patients undergoing home-based polysomnography. Nat Sci Sleep. (2018) 10:337–44. doi: 10.2147/NSS.S176201
30. Stepnowsky C, Levendowski D, Popovic D, Ayappa I, Rapoport DM. Scoring accuracy of automated sleep staging from a bipolar electroocular recording compared to manual scoring by multiple raters. Sleep Med. (2013) 14:1199–207. doi: 10.1016/j.sleep.2013.04.022
31. Finan PH, Richards JM, Gamaldo CE, Han D, Leoutsakos JM, Salas R, et al. Validation of a wireless, self-application, ambulatory electroencephalographic sleep monitoring device in healthy volunteers. J Clin Sleep Med. (2016) 12:1443–51. doi: 10.5664/jcsm.6262
32. Levendowski DJ, Ferini-Strambi L, Gamaldo C, Cetel M, Rosenberg R, Westbrook PR. The accuracy, night-to-night variability, and stability of frontopolar sleep electroencephalography biomarkers. J Clin Sleep Med. (2017) 13:791–803. doi: 10.5664/jcsm.6618
33. Sacco S, Bendtsen L, Ashina M, Reuter U, Terwindt G, Mitsikostas D-D, et al. European headache federation guideline on the use of monoclonal antibodies acting on the calcitonin gene related peptide or its receptor for migraine prevention. J Headache Pain. (2019) 20:6. doi: 10.1186/s10194-018-0955-y
34. American Headache Society. The American Headache Society position statement on integrating new migraine treatments into clinical practice. Headache. (2019) 59:1–18. doi: 10.1111/head.13456
35. Chen X, Wang R, Zee P, Lutsey PL, Javaheri S, Alcántara C, et al. Racial/Ethnic differences in sleep disturbances: the Multi-Ethnic Study of Atherosclerosis (MESA). Sleep. (2015) 38:877–88. doi: 10.5665/sleep.4732
36. Gillin JC, Wyatt RJ, Fram D, Snyder F. The relationship between changes in REM sleep and clinical improvement in depressed patients treated with amitriptyline. Psychopharmacology. (1978) 59:267–72. doi: 10.1007/BF00426633
37. Betts TA, Alford C. Beta-Blockers and sleep: a controlled trial. Eur J Clin Pharmacol. (1985) 28:65–8. doi: 10.1007/BF00543712
38. Mertz H, Fass R, Kodner A, Yan-Go F, Fullerton S, Mayer EA. Effect of amitryptiline on symptoms, sleep, and visceral perception in patients with functional dyspepsia. Am J Gastroenterol. (1998) 93:160–5. doi: 10.1111/j.1572-0241.1998.00160.x
39. Edvinsson L. CGRP receptor antagonists and antibodies against CGRP and its receptor in migraine treatment. Br J Clin Pharmacol. (2015) 80:193–9. doi: 10.1111/bcp.12618
40. Edvinsson L, Warfvinge K. Recognizing the role of CGRP and CGRP receptors in migraine and its treatment. Cephalalgia. (2019) 39:366–73. doi: 10.1177/0333102417736900
41. Ashina M, Goadsby PJ, Reuter U, Silberstein S, Dodick D, Rippon GA, et al. Long-term safety and tolerability of erenumab: three-plus year results from a five-year open-label extension study in episodic migraine. Cephalalgia. (2019) 39:1455–64. doi: 10.1177/0333102419854082
42. Ashina M, Goadsby PJ, Reuter U, Silberstein S, Dodick DW, Xue F, et al. Long-term efficacy and safety of erenumab in migraine prevention: Results from a 5-year, open-label treatment phase of a randomized clinical trial. Eur J Neurol. (2021) 28:1716–25. doi: 10.1111/ene.14715
43. Zhu C, Guan J, Xiao H, Luo W, Tong R. Erenumab safety and efficacy in migraine: a systematic review and meta-analysis of randomized clinical trials. Medicine. (2019) 98:e18483. doi: 10.1097/MD.0000000000018483
44. Ornello R, Casalena A, Frattale I, Gabriele A, Affaitati G, Giamberardino MA, et al. Real-life data on the efficacy and safety of erenumab in the Abruzzo region, central Italy. J Headache Pain. (2020) 21:32. doi: 10.1186/s10194-020-01102-9
45. European Medicine Agency (EMA). AIMOVIGTM Summary of Product Characteristics. (2018). Available online at: https://www.ema.europa.eu/documents/product-information/aimovig-epar-product-information_en.pdf (accessed: November 8, 2021).
46. Tepper SJ, Ashina M, Reuter U, Brandes JL, DoleŽil D, Silberstein SD, et al. Long-term safety and efficacy of erenumab in patients with chronic migraine: results from a 52-week, open-label extension study. Cephalalgia. (2020) 40:543–53. doi: 10.1177/0333102420912726
47. U.S. Food and Drug Administration (FDA). AIMOVIGTM (erenumab-aooe) Injection, for Subcutaneous Use. Highlights of Prescribing Information (2018). Available online at: https://www.accessdata.fda.gov/drugsatfda_docs/label/2018/761077s000lbl.pdf (accessed: November 11, 2021).
48. Hansen JM, Hauge AW, Olesen J, Ashina M. Calcitonin gene-related peptide triggers migraine-like attacks in patients with migraine with aura. Cephalalgia. (2010) 30:1179–86. doi: 10.1177/0333102410368444
49. Vgontzas A, Pavlović JM. Sleep disorders and migraine: review of literature and potential pathophysiology mechanisms. Headache. (2018) 58:1030–9. doi: 10.1111/head.13358
50. Tiseo C, Vacca A, Felbush A, Filimonova T, Gai A, Glazyrina T, et al. Migraine and sleep disorders: a systematic review. J Headache Pain. (2020) 21:126. doi: 10.1186/s10194-020-01192-5
51. Clemens S, Rye D, Hochman S. Restless legs syndrome: revisiting the dopamine hypothesis from the spinal cord perspective. Neurology. (2006) 67:125–30. doi: 10.1212/01.wnl.0000223316.53428.c9
52. Schuh-Hofer S, Richter M, Geworski L, Villringer A, Israel H, Wenzel R, et al. Increased serotonin transporter availability in the brainstem of migraineurs. J Neurol. (2007) 254:789–96. doi: 10.1007/s00415-006-0444-0
53. Supornsilpchai W, Sanguanrangsirikul S, Maneesri S, Srikiatkhachorn A. Serotonin depletion, cortical spreading depression, and trigeminal nociception. Headache. (2006) 46:34–9. doi: 10.1111/j.1526-4610.2006.00310.x
54. Nitz D, Siegel J. GABA release in the dorsal raphe nucleus: role in the control of REM sleep. Am J Physiol. (1997) 273:R451–R455. doi: 10.1152/ajpregu.1997.273.1.R451
55. Akerman S, Goadsby P. Dopamine and migraine: biology and clinical implications. Cephalalgia. (2007) 27:1308–14. doi: 10.1111/j.1468-2982.2007.01478.x
56. Saper CB, Scammell TE, Lu J. Hypothalamic regulation of sleep and circadian rhythms. Nature. (2005) 437:1257–63. doi: 10.1038/nature04284
57. Dodick DW, Eross EJ, Parish JM. Clinical, anatomical, and physiologic relationship between sleep and headache. Headache. (2003) 43:282–92. doi: 10.1046/j.1526-4610.2003.03055.x
58. Iliff JJ, Wang M, Liao Y, Plogg BA, Peng W, Gundersen GA, et al. A paravascular pathway facilitates CSF flow through the brain parenchyma and the clearance of interstitial solutes, including amyloid β. Sci Transl Med. (2012) 4:147ra111. doi: 10.1126/scitranslmed.3003748
59. Schytz HW, Olesen J, Ashina M. The PACAP receptor: a novel target for migraine treatment. Neurotherapeutics. (2010) 7:191–6. doi: 10.1016/j.nurt.2010.02.003
60. Edvinsson L, Tajti J, Szalárdy L, Vécsei L. PACAP and its role in primary headaches. J Headache Pain. (2018) 19:21. doi: 10.1186/s10194-018-0852-4
61. Holland PR, Barloese M, Fahrenkrug J. PACAP in hypothalamic regulation of sleep and circadian rhythm: importance for headache. J Headache Pain. (2018) 19:20. doi: 10.1186/s10194-018-0844-4
62. Lindberg PT, Mitchell JW, Burgoon PW, Beaulé C, Weihe E, Schäfer MK-H, et al. Pituitary Adenylate Cyclase-Activating Peptide (PACAP)-Glutamate co-transmission drives circadian phase-advancing responses to intrinsically photosensitive retinal ganglion cell projections by suprachiasmatic nucleus. Front Neurosci. (2019) 13:1281. doi: 10.3389/fnins.2019.01281
63. Nielsen HS, Hannibal J, Knudsen SM, Fahrenkrug J. Pituitary adenylate cyclase-activating polypeptide induces period1 and period2 gene expression in the rat suprachiasmatic nucleus during late night. Neuroscience. (2001) 103:433–41. doi: 10.1016/S0306-4522(00)00563-7
64. Csernus V. The effect of PACAP on rhythmic melatonin release of avian pineals. Gen Comp Endocrinol. (2004) 135:62–9. doi: 10.1016/S0016-6480(03)00284-3
Keywords: migraine, PACAP, CGRP, erenumab, PSQI, sleep efficiency, polysomnography
Citation: Pellitteri G, Pez S, Nilo A, Surcinelli A, Gigli GL, Lettieri C and Valente M (2022) Erenumab Impact on Sleep Assessed With Questionnaires and Home-Polysomnography in Patients With Migraine: The ERESON Study. Front. Neurol. 13:869677. doi: 10.3389/fneur.2022.869677
Received: 04 February 2022; Accepted: 14 April 2022;
Published: 13 May 2022.
Edited by:
Aynur Özge, Mersin University, TurkeyReviewed by:
Catello Vollono, Agostino Gemelli University Polyclinic (IRCCS), ItalyLevent Ertugrul Inan, Health Science University Ankara SUAM, Turkey
Raffaele Ornello, University of L'Aquila, Italy
Copyright © 2022 Pellitteri, Pez, Nilo, Surcinelli, Gigli, Lettieri and Valente. This is an open-access article distributed under the terms of the Creative Commons Attribution License (CC BY). The use, distribution or reproduction in other forums is permitted, provided the original author(s) and the copyright owner(s) are credited and that the original publication in this journal is cited, in accordance with accepted academic practice. No use, distribution or reproduction is permitted which does not comply with these terms.
*Correspondence: Gaia Pellitteri, cGVsbGl0dGVyaS5nYWlhQGdtYWlsLmNvbQ==; Sara Pez, c2FyYS5wZXo5MUBnbWFpbC5jb20=
†These authors have contributed equally to this work and share first authorship