- 1Department of Otolaryngology-Head and Neck Surgery, Graduate School of Medicine, Kyoto University, Kyoto, Japan
- 2Department of Otolaryngology-Head and Neck Surgery, Tazuke Kofukai Medical Research Institute Kitano Hospital, Osaka, Japan
Macrophages play important roles in tissue homeostasis and inflammation. Recent studies have revealed that macrophages are dispersed in the inner ear and may play essential roles in eliciting an immune response. Autoinflammatory diseases comprise a family of immune-mediated diseases, some of which involve sensorineural hearing loss, indicating that similar mechanisms may underlie the pathogenesis of immune-mediated hearing loss. Autoimmune inner ear disease (AIED) is an idiopathic disorder characterized by unexpected hearing loss. Tissue macrophages in the inner ear represent a potential target for modulation of the local immune response in patients with AIED/autoinflammatory diseases. In this review, we describe the relationship between cochlear macrophages and the pathophysiology of AIED/autoinflammatory disease.
Introduction
The functional mechanisms of resident macrophages (Mφs) in the inner ear remain largely unknown, in both the context of normal tissue physiology and disease development. Several recent reviews have discussed the importance of tissue-resident Mφs in the systemic and local regulation of inner ear homeostasis and disease pathology that improve our understanding of inner ear-resident Mφs (1–6).
In the United States, over 30 million adults, or approximately 15% of the entire population, are affected by hearing loss (7, 8). Research has indicated an expansion of the populations affected by sensorineural hearing loss, which impacts the conversion of mechanical sound to the neuroelectric indicators in the inner ear that relay signals to the auditory nerve (7, 8). Sensorineural hearing loss exerts a life-changing impact on affected patients; moreover, the current treatment modalities for sensorineural hearing loss are limited to the use of either cochlear implants or hearing aids. Cochlear implants directly stimulate the cochlear nerve by bypassing the damaged organ of Corti, which is most frequently affected by sensorineural hearing loss (9). Cochlear implants are among the artificial organs with the highest success rates; however, there is a social demand for more fundamental therapeutic options for sensorineural hearing loss.
Autoimmune inner ear disease (AIED) represents much fewer than 1% of all cases of sudden sensorineural hearing loss (10, 11), generally presenting as subacute, swiftly progressing, frequently fluctuating, bilateral, and asymmetrical sensorineural hearing loss (9–14). AIED can be labeled as primary AIED, in which the inner ear is the most affected organ, or secondary AIED (15–30% of cases), which develops in association with a systemic autoimmune disease (12), such as autoimmune hepatitis (15), systemic lupus erythematosus (SLE) (16), multiple sclerosis (MS) (17), rheumatoid arthritis (18), inflammatory bowel disease (IBD) (19), or antiphospholipid syndrome (14, 20).
Collectively, current evidence indicates that aberrant events in the early innate immune response play critical roles in the development and manifestation of autoimmune hearing loss (10, 11). As with most autoimmune diseases, it has been postulated that a misdirected assault on the self-organs, mainly inner ear proteins in AIED, activates the pro-inflammatory T-cell response and autoantibody formation; this represents the basic pathophysiology of AIED and other autoimmune diseases. Autoinflammatory diseases fall within the family of immune-mediated diseases, many of which induce sensorineural hearing loss, suggesting that similar mechanisms are involved in the pathogenesis of AIED (21). In the autoinflammatory disorder-related hearing loss, genetic mutations or polymorphisms inherited in an autosomal dominant manner result in a gain-of-function mutation within the gene for nod-like receptor (NLR) family pyrin domain 3 (NLRP3), leading to excessive interleukin (IL)-1β release, sensorineural hearing loss, systemic amyloidosis, and/or transient skin rashes. Muckle–Wells syndrome (MWS) and neonatal-onset multisystem inflammatory sickness belong to a family of autoinflammatory diseases known as a cryopyrin-related periodic syndrome, which also involves sensorineural hearing loss (22, 23). However, the role of Mφs in AIED and autoinflammatory diseases has not been well-documented thus far.
Although 70% of patients with AIED initially respond to corticosteroids (11), understanding the role of Mφs in the pathophysiology of progressive hearing loss is critical for the development of improved therapies. In this review, we discuss recent findings related to the development of immune-competent cells within the inner ear (24, 25), thereby clarifying the role of inner ear-resident Mφs in inner ear homeostasis and pathological processes. We also cover a wide range of research areas involving tissue-resident Mφs that include those related to recent advances in antigen differentiation, gene expression patterns, and the clinical features and pathology of AIED/autoinflammatory diseases.
Origins and the Diversification of Tissue-Resident Macrophages—Identification Through Differentiation of Antigens, Fate-Mapping, and Gene Expression Patterns
Macrophages are present in all vertebrate tissues, emerging earlier than any other blood cell type from mid-gestation and are distributed in almost every organ and tissue in the body throughout life (26, 27). In addition to their role in regulating tissue development and regeneration, Mφs aid in maintaining local homeostasis by responding to internal and external stimuli, appearing as phagocytes that protect against microbes. Furthermore, they participate in the clearance of useless and senescent cells and act as sentinels with trophic, regulatory, and repair functions. Heterogeneous Mφ phenotypes are observed in different tissue environments that highlight their organ-specific capabilities in developmental processes and normal physiology (28–30) (Figure 1). In addition, Mφs exhibit diverse tissue-specific functions, integrating cues from the external surroundings and their microenvironment. Hence, tissue-resident Mφs represent an appealing target for therapeutics given their implication in various pathological processes that include those related to atherosclerosis, autoimmune diseases, neurodegenerative and metabolic disorders, and tumor growth (13). Elucidating the developmental pathways and characteristics of Mφs may aid in the design of novel interventional strategies, which focused on the tissue-specific microenvironment.
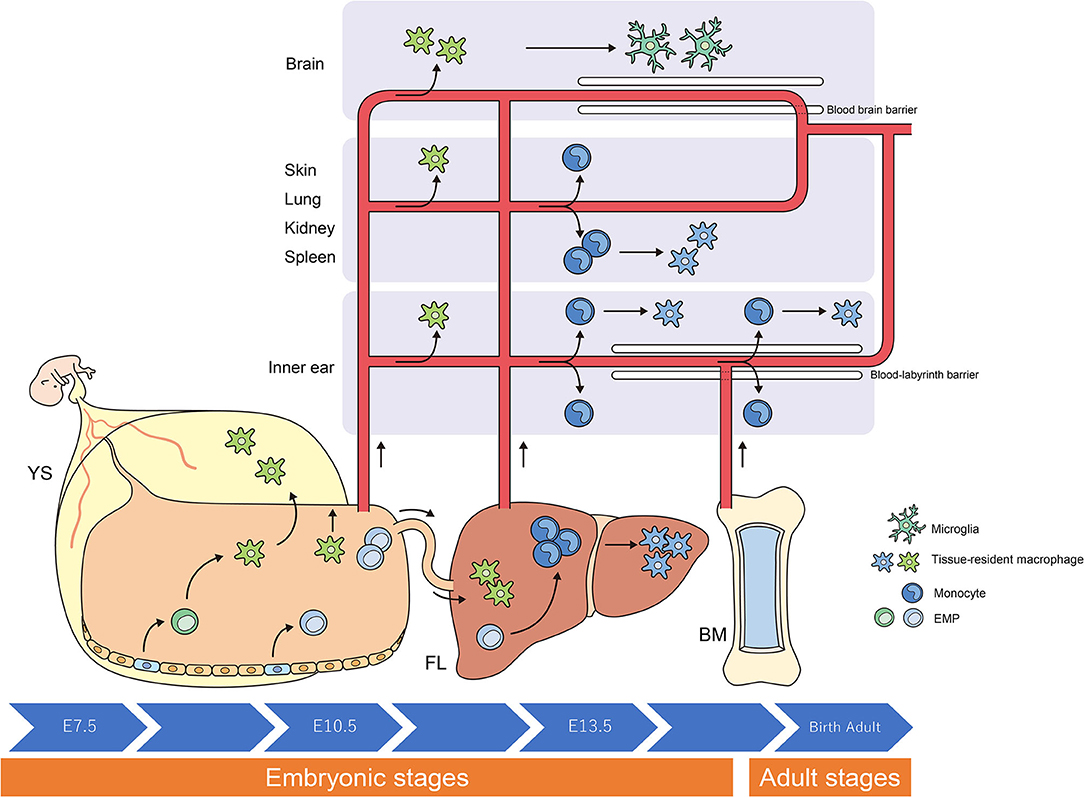
Figure 1. Origins and renewal of tissue-resident macrophages. The latest destiny-mapping studies in mice imply that Mφs derived from the YS during early embryogenesis contribute to pools of mature tissue Mφs that include Langerhans cells and microglia. Further seeding in different tissues occurs following definitive hematopoiesis in the FL or BM. Proliferative local expansion of tissue Mφs within the neonatal period, followed by low-level self-renewal throughout adulthood, appears sufficient for maintaining many tissue-resident Mφ populations. By helping to resolve acute inflammation, local proliferation may contribute to restoring homeostatic tissue-resident Mφ populations. Although the exact contribution of BM-derived inflammatory Mφs to these tissue-resident pools remains unclear, it nonetheless appears to take place, perhaps in a tissue-specific manner. YS, yolk sac; FL, fetal liver; BM, bone marrow; EMP, erythro-myeloid progenitor; Mφ, macrophage.
Researchers have debated whether resident Mφs are constantly and predominantly repopulated via the delivery of blood-circulating monocytes, which derive from progenitors inside the bone marrow (BM). However, recent studies have demonstrated that specific Mφ populations are independent of circulating monocytes and even adult BM hematopoiesis (1, 2, 24, 31). These tissue-resident Mφs are derived from the sequential seeding of tissues by means of various precursors during embryonic development. Primitive Mφs are generated from early erythro-myeloid progenitors (EMPs) inside the yolk sac (YS) that bypass monocytic intermediates and give rise to microglia via the transcription element c-Myb. Ultimately, fetal monocytes are generated from c-Myb+ EMPs that begin to seed the fetal liver (FL), giving rise to various types of mature Mφs. Hence, hematopoietic stem cell-impartial embryonic precursors transiently are present in the YS and FL represents the origin of long-lasting, self-renewing Mφ populations with organ-specific functions (1, 26) (Figure 1).
The percentage of resident Mφs varies according to their origin, the developmental stage of the organism, and the tissue type. For instance, most of the microglia in the brain originate from YS-derived Mφs, whereas Mφs from the FL and BM provide a negligible contribution to microglia during all stages of life (6, 10). In contrast, resident Mφs in the gut are derived from the YS at some point during early embryonic development. Monocytes derived from the FL give rise to most of the resident Mφs in the intestine at birth; however, throughout adulthood, most resident Mφs originate from the BM (5, 27).
When compared with other tissue-resident populations of Mφs, the cellular expression profiles of cochlear Mφs and the markers that can be used to visualize these cells have been poorly documented. The dynamics of Mφ populations in the developing cochlea have been characterized most appreciably in mice and are summarized in Figure 2 (24, 31, 32). Colony-stimulating factor 1 (Csf1) signaling controls the seeding of the larger Mφ population within the cochlea throughout development (24, 25). A second populace derived from the FL, which is Csf1 receptor-independent, is observed in the modiolus and the intraluminal surface of the perilymphatic area inside the embryonic cochlea (24). Cochlear Mφs and perivascular macrophage-like melanocytes (PVM/Ms) lie close to blood vessels in the adult cochlea (33)—such as in the cochlear modiolus, supporting cells, spiral ganglion neurons (SGNs), stria vascularis (SV), and spiral ligament (SLi)—under normal conditions (4, 24, 25, 31, 32, 34–36) (Figure 3).
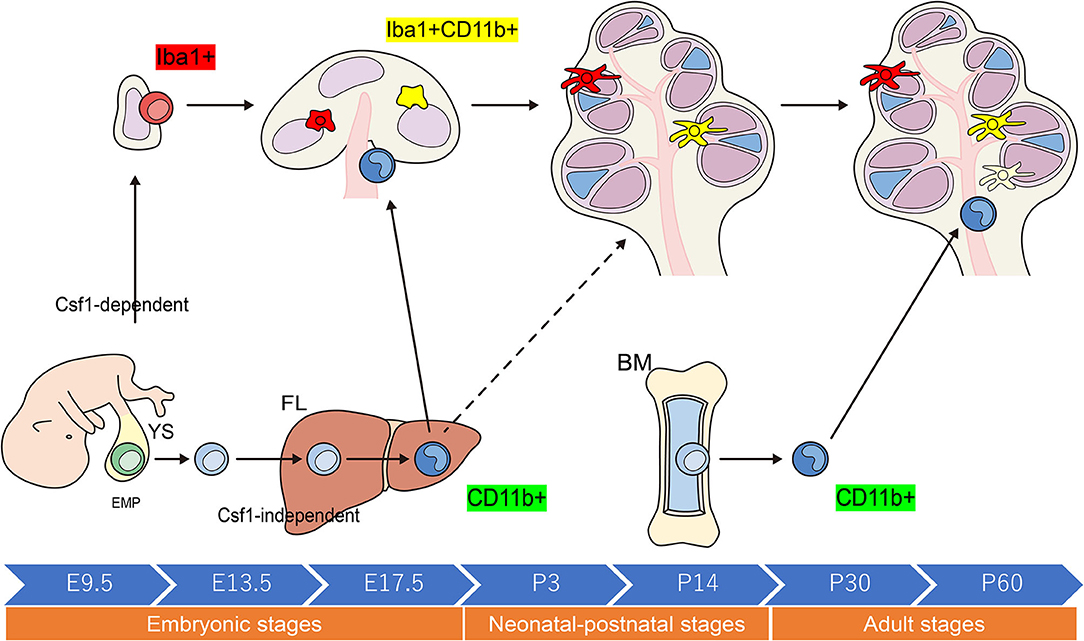
Figure 2. Schematic representation of the origins and distribution of resident macrophages in embryonic and adult cochleae. Two subtypes of resident Mφs are present within the embryonic cochlea: Csf1r-dependent Mφs that originate from the YS and Csf1r-independent Mφs that migrate from the FL via systemic circulation. A large proportion of the cochlear-resident Mφ population is derived from the YS given that Mφs expressing Iba1 reside in the mesenchyme surrounding the otocyst as early as E10.5. These Mφs are distributed within the SGN and SLi at E17.5. However, Csf1r-independent Mφs expressing CD11b migrate as early as E14.5 and reside only in specific components of the cochlea, consisting of the mesenchyme of the cochlear modiolus or the intraluminal floor of the perilymphatic area at E17.5. In the adult cochlea, the density of Mφs expressing Iba1 regularly decreases, whereas that of Mφs expressing CD11b increases, suggesting that the FL and BM contribute to the repopulation of cochlear-resident Mφs. YS, yolk sac; FL, fetal liver; BM, bone marrow; Mφ, macrophage; Csf1r, Colony-stimulating factor-1 receptor; SGN, spiral ganglia neurons; SLi, spiral ligament; EMP, erythro-myeloid progenitor.
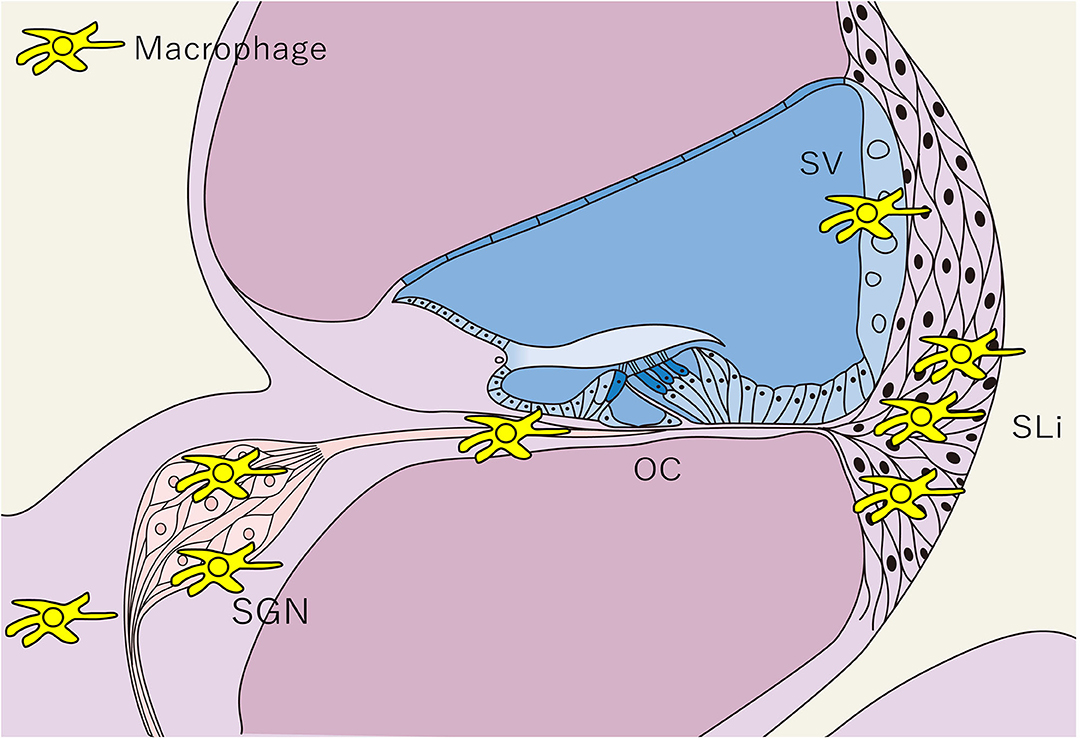
Figure 3. Distribution of tissue macrophages in the cochlea. The distribution of tissue Mφs is shown in a schematic cross-sectional view of the cochlea. Tissue Mφ are distributed in the SGN, SLi, SV, and cochlear modiolus. Cochlear Mφs also exhibit spindle-shaped cell bodies and dendritic cell processes under steady-state conditions, as observed in microglia in the central nervous system. SGN, spiral ganglion neurons; SLi, spiral ligament; SV, stria vascularis; OC, Organ of Corti; Mφ, macrophage.
The inner ear is responsible for auditory sensation and the perception of acceleration/rotation, and it is difficult to restore the population of sensory cells in the inner ear after degeneration due to acute or chronic inner ear injuries, such as those related to Meniere's disease and sudden sensorineural hearing loss. This is partly because, in humans, hair cells in the inner ear are terminally differentiated, losing their potential for self-renewal following significant damage after birth (33). SGNs, which mediate synaptic connections among the hair cells and the neurons of the cochlear nucleus, also undergo damage and degeneration, while damage or atrophy of the SV and SLi disrupts cochlear function. Repeated exposure of the auditory system to insults causes harm to these structures, resulting in functional impairments that lead to progressive hearing loss (7).
Cochlear Mφs persist from the early post-natal stages and renew or preserve their populace via the infiltration of circulating monocytes (34, 37, 38). PVM/Ms are found adjacent to the blood vessels in the SV and support cells in the cochlea (35, 39). PVM/Ms have a turnover time of several months in mice and are maintained via the migration of monocytes that originate from the BM into the cochlea (36). Functionally, PVM/Ms contribute to restoring the endocochlear potential, which is crucial for the activation of auditory hair cells (40, 41). Interestingly, Mφ heterogeneity results in distinct phenotypes and, more importantly, completely exceptional organic functions in other tissues (28). Therefore, future studies should aim to elucidate the roles of tissue-resident and BM-derived Mφs in the initiation, progression, and termination of inner ear diseases.
Macrophages and Autoimmune Diseases
Monocytes and Mφs can secrete a wealth of cytokines and chemokines, which further stimulate other forms of immune cells, thereby leading to inflammation (33, 42). The presence of autoantibodies and autoreactive B and T cells in most autoimmune diseases indicates that the adaptive immune system is essential for their pathogenesis; however, this cannot completely account for the resolution and development of those diseases, and studies have indicated that the innate immune response may also play necessary and irreplaceable roles in the pathogenesis of the autoimmune disease (33, 43, 44).
A monocyte or Mφ infiltration is typically observed in most autoimmune diseases (33). The regulatory mechanisms that involve monocytes and/or Mφs in the development of the autoimmune disease have not been fully elucidated; nevertheless, the consensus appears to signify that their atypical activation plays a key role in the abovementioned mechanisms. Mφs exhibit shifts in polarization primarily based on diverse stimuli produced with the aid of cytokines, microbes, microbial products, and other modulators in vitro (45). However, in an in vivo study, alterations in arginine metabolism following lipopolysaccharide (LPS) injection were found to elicit different phenotypes of Mφs in C57BL/6J and Balb/c mice (46). C57BL/6J peritoneal Mφs promoted inducible nitric oxide synthase (iNOS) activity, resulting in nitric oxide expression and a T-helper 1 (Th1) CD4+ T cell response. In contrast, Mφs from Balb/c mice triggered arginase activity, resulting in an ornithine and Th2 response. Analogous to Th1 and Th2, these Mφs have been termed M1 and M2 (46). Generally, M1-polarized Mφs are pro-inflammatory and secrete IL-12 and tumor necrosis factor (TNF)-α, which contribute to local irritation, while M2-polarized Mφs produce IL-4 and IL-10, which play roles in immunomodulation, wound repair, and tissue remodeling (43, 47).
Within tissues of the central nervous system (CNS), particularly in patients with progressive MS, infection is characterized by the massive activation of mononuclear phagocytes that include both monocyte-derived Mφs and resident microglia (48). The staging of MS lesions can be determined based totally on the presence of CD68-nice Mφs and human leukocyte antigens, together with the extent of myelin loss (49). Findings obtained using the experimental autoimmune encephalomyelitis (EAE) model, an animal model of MS, have indicated that Mφs play crucial roles in triggering adaptive immune responses. For example, the Mφ NLRP3 inflammasome plays key role in inducing autoreactive T cell migration into the CNS in EAE (50). Mφs also produce several key cytokines (IL-1β, IL-6, and IL-23) that promote the generation and maintenance of Th17 cells, an important cell subset mediating CNS autoimmunity in EAE (51). For this reason, accumulating evidence suggests that Mφs play divergent roles in the pathogenesis of MS, exacerbating tissue damage despite their outstanding growth-promoting and neuroprotective effects (52). As predicted, this dual role of Mφs in MS may be defined by their polarization state. Indeed, both M1 and M2 subsets are found in MS lesions. The unexpected pro-inflammatory M1 reaction is maintained at sites of CNS damage, while the immunoregulatory M2 response is comparatively weaker and more transient (52). Yamasaki et al. demonstrated that resident macroglia are associated with particle clearance. In the context of MS, these cells exhibit global suppression of metabolism throughout disease initiation, whereas monocyte-derived Mφs become exceptionally phagocytic and inflammatory, actively participating in the initiation of demyelination (53).
Monocytes/Mφs contribute to the pathogenesis of SLE by modulating the adaptive immune response in the kidney. Defective Mφ phagocytosis has also been thought to contribute to autoimmunity in SLE. The phagocytic potential of Mφs is vital for the clearance of dead cells and debris, which otherwise may be critical sources of autoantigens. Accumulating evidence from in vitro studies and murine models illustrates that ineffective clearance of apoptotic cells by Mφs may represent a critical trigger of the autoimmune response in SLE (33). In lupus-inclined NZB/W and NZW/BXSB mice, resident nephritic Mφs exhibit decreases in arginase and iNOS production despite treatment with M1- or M2 Mφ-inducing cytokines, regardless of their health status (54). Instead, these Mφs exhibit a combined pro- and anti-inflammatory phenotype throughout the course of lupus-related nephritis. The authors argued that monocyte-derived Mφs in these mice are poorly responsive to the cytokine stimulation that enables the transition to the corresponding M1 or M2 type (54). In addition to these phenotypic differences, functional analysis has confirmed that resident kidney Mφs exhibit greater antigen-producing and phagocytotic effects than monocyte-derived kidney Mφs (33, 54).
Autoimmune uveitis, which occurs in several diseases that include Behçet's disease, sarcoidosis, and Vogt–Koyanagi–Harada disease, is a sight-threatening ocular inflammatory disorder (55). Immunization with interphotoreceptor retinoid-binding protein and extra adjuvants leads to the priming of autoreactive CD4+ T cells in peripheral lymphoid organs and their polarization into pathogenic Th1 and Th17 cells. Once activated, Th cells in the eye induce the breakdown of the blood–retinal barrier, an immune barrier that protects the eyes from unfavorable inflammation through tight junctions between endothelial cells in blood vessels; these tight junctions block circulating leukocyte extravasation into the retina (56). Okunuki et al. suggested that retinal microglia constitute the essential cellular populace within the retina that enables entry of the autoreactive cells required for the initiation of autoimmune uveitis; however, systemic exposure to an autoantigen is in all likelihood the cause of autoimmunity in this disorder (55).
Taken together, these findings highlight the proposed relationship between monocytes/Mφs and the development of autoimmune diseases in the CNS, kidneys, and eyes. It is well-known that monocytes/Mφs are the key components of the innate immune system that underlie the amplification and suppression of inflammation (42). Increasing evidence indicates that these cells participate in the pathogenesis of autoimmune diseases mainly via their remarkably pro-inflammatory or fibrogenic functions (42, 57). As discussed above, the heterogeneity of monocyte/Mφ subpopulations varies dramatically in different autoimmune diseases, and their polarization profiles generally play key roles in diseases progression. However, for several autoimmune diseases, the phenotypic and functional characteristics of monocytes/Mφs remain poorly categorized, as many pro-inflammatory M1-polarized monocytes/Mφs concurrently express M2-associated markers or showcase immunomodulatory features (33).
The Role of Inner Ear Macrophages in AIED
The inner ear is fully able to mount an immune response following the invasion of outside antigens. Consequently, numerous mechanisms have been proposed to underlie cochlear damage that include antibody–antigen reactions with autoantibody enhancement (type II immune responses), complement machine activation, immune-complicated depletion (type III immune responses), direct damage mediated via cytotoxic T-cells crossing the blood–labyrinth barrier and reaching the endolymphatic sac, vasculitis, micro-thrombosis, and electrochemical reactions (type IV immune responses) (10, 12, 14, 20, 58). Antigen recognition by the innate immune cells of the inner ear (neutrophils, Mφs, and dendritic cells) stimulates the release of IL-1β, which in turn triggers a series of adaptive immune responses. The recruitment of immunocompetent cells and the promotion of an adaptive immune response occur in the presence of immune mediators, such as IL-1β, IL-2, and TNFα (13, 20). Studies have suggested an association between sudden sensorineural hearing loss and the presence of vestibulocochlear antibodies against inner ear antigens, such as heat shock protein 70, cochlin, β-tectorin, and types II and IX collagen (12, 20, 59–61).
Although the relationship between AIED/autoinflammatory diseases and cochlear monocytes/Mφs remains largely unknown, previous studies have indicated that cochlear Mφs play crucial roles in the onset and progression of infection after insults to the cochlear sensory epithelium. Such insults include noise or drug exposure and mechanical or surgical injury to the cochlea, such as that occurring during cochlear implantation (5, 31, 34, 62–72). These signals activate resident cochlear Mφs, switching them to a pro-inflammatory state and causing them to release cytokines that recruit monocytes into the cochlea (34, 62). Infiltrating monocytes then differentiate into macrophages within the cochlea, following which they exert phagocytotic functions (69, 73), produce inflammatory mediators, and play roles in antigen presentation (34). In some patients with Ménière's disease, high levels of IL-1β and TNF-α suggested that it was a chronic inflammatory disorder (74). In addition, several reports suggested an association between the immune responses caused by Mφs and Ménière's disease (75–80).
As described above, monocytes/Mφs are key components of the innate immune system in the pathogenesis of systemic autoimmune diseases and are involved in amplifying and suppressing inflammation (33, 42), mainly through their remarkably pro-inflammatory or fibrogenic properties (33, 42, 57). Nakanishi et al. suggested that monocytes are the primary regulators of IL-1 release in MWS, which is caused by a mutation in the NLRP3 gene that leads to excessive IL-1β production (81). Gattorneo et al. also reported that monocytes from patients with MWS released a minute amount of IL-1 when compared with control monocytes, but that patients were extremely responsive to anakinra use (IL-1 inhibition) (82). Additionally, Nakanishi et al. indicated that LPS stimulation can activate the NLRP3 inflammasome in monocyte/Mφ-like cells (CX3CR1-superb cells) in wild-type C57BL/6J mouse cochleae (81). These findings support the notion that local cochlear activation of the NLRP3 inflammasome in monocytes/Mφs can result in cochlear autoinflammation and sensorineural hearing loss (83). Vambutas et al. demonstrated that patients with steroid-resistant AIED are clinically sensitive to IL-1 inhibition; however, relative to those in individuals with steroid-sensitive AIED and controls, these monocytes synthesize greater but release much less IL-1 (84).
Previous studies have revealed that 46–57% of adult patients with IBD present with sensorineural hearing loss as an extraintestinal manifestation (85, 86); however, few reports have mentioned the roles of monocytes/Mφs. Dettmer et al. reported that the temporal bones of patients with IBD exhibited mild chronic inflammation, poorly defined granulomas, and CD68-positive Mφ infiltration (87). IBD may also be associated with Cogan's syndrome, a rare disorder characterized by eye and inner ear inflammation manifesting as interstitial keratitis and audiovestibular dysfunction, respectively (88). The mechanisms associated with eye and inner ear disorders in Cogan's syndrome are unknown; however, the authors of one autopsy case reported histopathologic evidence of vasculitis and an infiltration of CD45-positive inflammatory cells that include Mφs in both the cochlear and peripheral vestibular systems (89). Moreover, a few studies have suggested that the disease is a result of inner ear autoimmunity (90, 91). Aberrantly activated intestinal Mφs in patients with IBD produce diverse cytokines (IL-1β, IL-6, IL-23, TNF-α, and TNF-like protein 1A) required for T-cell differentiation, especially those related to the generation of Th1 and Th17 cells (92). Furthermore, in those with IBD, intestinal Mφs cause an abnormally fast breakdown of pro-inflammatory cytokines due to faster lysosomal degradation, whereas cytokine mRNA expression remains stable and within the normal range (93). This has been shown to elicit an impaired neutrophil response, leading to dysfunction in bacterial clearance and thereby boosting the formation of granulomas. Within the pathology of IBD, Mφs are hyperpolarized toward the M2 profile, as demonstrated in numerous studies. In various mouse models of IBD, inhibition of the pro-inflammatory activities of M1 Mφs or induction of tissue-repairing/immunomodulatory M2 Mφs results in attenuated experimental IBD (94, 95). Similar mechanisms are speculated to occur in the inner ear in patients with IBD.
In summary, cumulative and progressive sensory cell degeneration and death, caused by chronic inflammation, end in the activation of resident Mφs, with little infiltration of circulating monocytes (62), which parallels the innate immune response observed in chronic diseases. The activated resident Mφs adopt either a pro- or anti-inflammatory profile. Pro-inflammatory Mφs produce and release pro-inflammatory mediators—such as IL-1β, TNF-α, and IL-6—which send signals to nearby cells, leading to further inflammation and cellular damage/apoptosis (34, 96, 97) (Figure 4). However, to date, no study has clarified which cells in the inner ear are targeted by Mφs, and the precise differences in phenotype and activity among newly recruited monocytes/Mφs and resident Mφs remain to be determined. Across autoimmune disorders, the heterogeneity of monocyte/Mφ subpopulations varies dramatically; furthermore, their polarization profile usually plays a key role in disease development (33). Further research is required to elucidate the specific roles of cochlear monocytes/Mφs in the pathophysiology of autoimmune-mediated hearing loss.
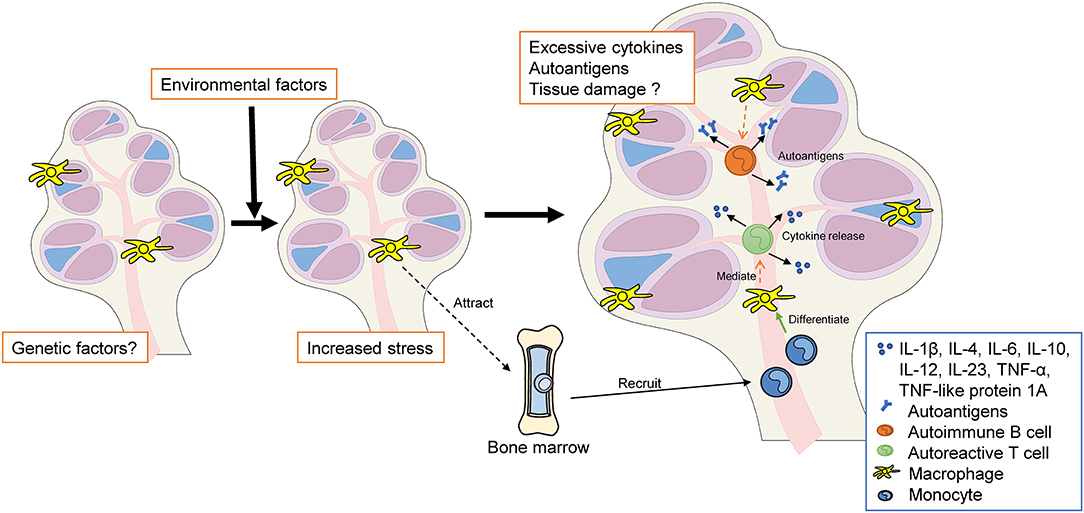
Figure 4. The hypothesis of autoimmune and autoinflammatory diseases in the inner ear. Genetic and/or environmental factors increase both systemic stress and local stress in the inner ear (e.g., MS, SLE, autoimmune uveitis, MWS, and IBD). Resident cochlear Mφs may be activated to a pro-inflammatory state. Pro-inflammatory macrophages release cytokines that recruit monocytes into the cochlea. These infiltrating monocytes differentiate into macrophages within the cochlea, following which they participate in phagocytosis, inflammatory mediator production, and antigen presentation. Excessive autoantigen and cytokine release may cause cochlear autoinflammation and sensorineural hearing loss. MS, multiple sclerosis; SLE, systemic lupus erythematosus; MWS, Muckle–Wells syndrome; IBD, inflammatory bowel disease; Mφ, macrophage, BM, bone marrow; TNF, tumor necrosis factor; IL, interleukin.
Future Directions
Numerous research groups have proposed Mφ-targeted treatment options for inflammatory diseases. Among the most investigated strategies is the facilitation of Mφ phagocytosis via nanoparticle targeting, which then passively targets the inflammatory site due to the mounting immune response (98, 99). The modulation and reprogramming of Mφs are also considered promising anti-tumor strategies (69–71). Using genetically modified monocytes or Mφs as vectors may aid in the development of therapeutic strategies that promote regeneration or regrowth of particular structures inside the inner ear (6, 61, 100). This idea is especially well-suited for secreted paracrine or endocrine factors, such as hormones or growth factors. Because the inner ear includes three fluid-filled compartments, secreted elements derived from genetically modified Mφs may reach the central cells via diffusion through the inner ear without the involvement of the blood or lymphatic circulation. The transplantation of genetically engineered cells that can secrete particular metabolic or humoral cues may help to augment pharmacologic immune modulation inside the inner ear; however, the delivery of genetically modified cells into the inner ear may be challenging due to its anatomical characteristics. Given that monocytes and Mφs can migrate into the inner ear in both pathological and physiological states (6, 32, 61, 69), the human monocyte lineage can be extracted, cultured ex vivo, genetically manipulated, and reimplanted locally or systemically. Intravenous management of genetically modified monocytes should permit them to reach the inner ear despite challenges related to tissue or organ specificity.
Extending studies to identify and establish the set of markers expressed by cochlear Mφs to include multiplex and comparative transcriptomic studies may improve our ability to identify sub-populations and roles and to compare the profiles of resident Mφs in different organs. To better understand the roles of these cells, numerous processes that permit source- and area- (cochlear sub-structure) specific Mφ analysis are required. Such techniques can also be used to identify remote cells or tissue indicators in situ. For example, in the brain, infiltrating monocytes have been shown to exhibit functional variations and contribute to disease pathology via multiple mechanisms following ischemic stroke and MS (53, 101). To develop therapeutic strategies for AIED and autoinflammation, it is first necessary to understand the effects of targeting cochlear Mφs. Furthermore, as hearing loss fluctuates in patients with AIED and autoinflammation, the timing of intravenous administration of genetically modified monocytes may represent a potential problem in clinical applications.
Conclusion
In this review, we discussed the latest advances and evidence regarding the connection between tissue-resident Mφs and AIED/autoinflammatory diseases. Three subtypes of cochlear-resident Mφs are found in exclusive components of the cochlea, and each subtype may play distinct roles in the abovementioned diseases. Furthermore, the dynamics and molecules expressed in tissue Mφs in the inner ear are gradually being elucidated. However, several aspects remain unclear. In particular, the role of tissue Mφs in the pathology of AIED is not well-understood, in part due to restrictions on the collection of human inner ear tissue. Moreover, additional research is required to compare the pathophysiology of such diseases in mouse models and humans. Despite the need for extensive research, the future of inner ear immunology and treatment for sensorineural hearing loss remains promising. Developing a greater understanding of monocyte/Mφ-associated activities within the cochlea may be essential for developing new biological and therapeutic strategies for related diseases.
Author Contributions
TM designed and conducted the study and reported the findings. TO validated the study design, reviewed the manuscript, and supervised the study. Both authors contributed to the article and approved the submitted version.
Funding
This work was supported by a JSPS KAKENHI grant (no. 19K09908) to TO. The funder had no role in the study design, data collection and analysis, publication decision, or manuscript preparation.
Conflict of Interest
The authors declare that the research was conducted in the absence of any commercial or financial relationships that could be construed as a potential conflict of interest.
Publisher's Note
All claims expressed in this article are solely those of the authors and do not necessarily represent those of their affiliated organizations, or those of the publisher, the editors and the reviewers. Any product that may be evaluated in this article, or claim that may be made by its manufacturer, is not guaranteed or endorsed by the publisher.
Acknowledgments
The authors wish to thank Edanz and Editage for English language editing and manuscript formatting.
References
1. Hoeffel G, Ginhoux F. Fetal monocytes and the origins of tissue-resident macrophages. Cell Immunol. (2018) 330:5–15. doi: 10.1016/j.cellimm.2018.01.001
2. Sreejit G, Fleetwood AJ, Murphy AJ, Nagareddy PR. Origins and diversity of macrophages in health and disease. Clin Transl Immunol. (2020) 9:e1222. doi: 10.1002/cti2.1222
3. Jenkins SJ, Allen JE. The expanding world of tissue-resident macrophages. Eur J Immunol. (2021) 51:1882–96. doi: 10.1002/eji.202048881
4. Liu W, Molnar M, Garnham C, Benav H, Rask-Andersen H. Macrophages in the human cochlea: saviors or predators-a study using super-resolution immunohistochemistry. Front Immunol. (2018) 9:223. doi: 10.3389/fimmu.2018.00223
5. Warchol ME. Interactions between macrophages and the sensory cells of the inner ear. Cold Spring Harb Perspect Med. (2019) 9:a033555. doi: 10.1101/cshperspect.a033555
6. Okano T. Immune system of the inner ear as a novel therapeutic target for sensorineural hearing loss. Front Pharmacol. (2014) 5:205. doi: 10.3389/fphar.2014.00205
7. Michels TC, Duffy MT, Rogers DJ. Hearing loss in adults: differential diagnosis and treatment. Am Fam Physician. (2019) 100:98–108.
8. Young YH. Contemporary review of the causes and differential diagnosis of sudden sensorineural hearing loss. Int J Audiol. (2020) 59:243–53. doi: 10.1080/14992027.2019.1689432
9. Carlson ML. Cochlear implantation in adults. N Engl J Med. (2020) 382:1531–42. doi: 10.1056/NEJMra1904407
10. Rossini BAA, De Oliveira Penido N, Munhoz MSL, Bogaz EA, Curi RS. Sudden sensorioneural hearing loss and autoimmune systemic diseases. Int Arch Otorhinolaryngol. (2017) 21:213–23. doi: 10.1055/s-0036-1586162
11. Breslin NK, Varadarajan VV, Sobel ES, Haberman RS. Autoimmune inner ear disease: a systematic review of management. Laryngoscope Investig Otolaryngol. (2020) 5:1217–26. doi: 10.1002/lio2.508
12. Ribeiro R, Serôdio JF, Amaral MC, Duarte JA, Durão C, Mendes N, et al. Sensorineural hearing loss and systemic autoimmune disease: the experience of a systemic immune-mediated diseases unit. Cureus. (2021) 13:e14075. doi: 10.7759/cureus.14075
13. Das S, Bakshi SS, Seepana R. Demystifying autoimmune inner ear disease. Eur Arch Oto-Rhino-Laryngology. (2019) 276:3267–74. doi: 10.1007/s00405-019-05681-5
14. Ciorba A, Corazzi V, Bianchini C, Aimoni C, Pelucchi S, Skarzyński PH, et al. Autoimmune inner ear disease (AIED): a diagnostic challenge. Int J Immunopathol Pharmacol. (2018) 32. doi: 10.1177/2058738418808680
15. Parizad EG, Matin HG, Parizad EG, Khosravi A. The prevalence of hearing loss in patients with hepatitis B infection compared with healthy volunteers. Iran J Otorhinolaryngol. (2017) 29:127.
16. Chawki S, Aouizerate J, Trad S, Prinseau J, Hanslik T. Bilateral sudden sensorineural hearing loss as a presenting feature of systemic lupus erythematosus Case report and brief review of other published cases. Med. (2016) 95:e4345. doi: 10.1097/MD.0000000000004345
17. Tanaka M, Tanaka K. Sudden hearing loss as the initial symptom in Japanese patients with multiple sclerosis and seropositive neuromyelitis optica spectrum disorders. J Neuroimmunol. (2016) 298:16–8. doi: 10.1016/j.jneuroim.2016.06.004
18. Li H, Zhang M, Wang M, Zhang S, Ma S, Wang X. Clinical feature and prognosis of sudden sensorineural hearing loss with rheumatoid arthritis. Otol Neurotol. (2021) 42:e267–71. doi: 10.1097/MAO.0000000000002962
19. Tirelli G, Tomietto P, Quatela E, Perrino F, Nicastro L, Cattin L, et al. Sudden hearing loss and Crohn disease: when Cogan syndrome must be suspected. Am J Otolaryngol - Head Neck Med Surg. (2015) 36:590–7. doi: 10.1016/j.amjoto.2015.02.013
20. Strum D, Kim S, Shim T, Monfared A. An update on autoimmune inner ear disease: a systematic review of pharmacotherapy. Am J Otolaryngol Head Neck Med Surg. (2020) 41:102310. doi: 10.1016/j.amjoto.2019.102310
21. Dinarello CA, Simon A, Van Der Meer JWM. Treating inflammation by blocking interleukin-1 in a broad spectrum of diseases. Nat Rev Drug Discov. (2012) 11:633–52. doi: 10.1038/nrd3800
22. Vambutas A, Pathak S. Autoimmune and autoinflammatory (disease) in otology: what is new in immune-mediated hearing loss. Laryngoscope Investig Otolaryngol. (2016) 1:110–5. doi: 10.1002/lio2.28
23. Vambutas A, Pathak S. Monocytes, macrophages, and microglia and the role of IL-1 in autoimmune inner ear disease (AIED). Curr Otorhinolaryngol Rep. (2018) 6:203–8. doi: 10.1007/s40136-018-0191-7
24. Kishimoto I, Okano T, Nishimura K, Motohashi T, Omori K. Early development of resident macrophages in the mouse cochlea depends on yolk sac hematopoiesis. Front Neurol. (2019) 10:1115. doi: 10.3389/fneur.2019.01115
25. Okano T, Kishimoto I. Csf1 signaling regulates maintenance of resident macrophages and bone formation in the mouse cochlea. Front Neurol. (2019) 10:1244. doi: 10.3389/fneur.2019.01244
26. Hoeffel G, Ginhoux F. Ontogeny of tissue-resident macrophages. Front Immunol. (2015) 6:486. doi: 10.3389/fimmu.2015.00486
27. Ginhoux F, Guilliams M. Tissue-resident macrophage ontogeny and homeostasis. Immunity. (2016) 44:439–49. doi: 10.1016/j.immuni.2016.02.024
28. Gordon S, Plüddemann A. Tissue macrophages: heterogeneity and functions. BMC Biol. (2017) 15:1–8. doi: 10.1186/s12915-017-0392-4
29. Wynn TA, Chawla A, Pollard JW. Macrophage biology in development, homeostasis and disease. Nature. (2013) 496:445–55. doi: 10.1038/nature12034
30. Schneider C, Kopf M. TEMPting fate MaYBe the solution. Immunity. (2015) 42:597–99. doi: 10.1016/j.immuni.2015.04.001
31. Okano T, Nakagawa T, Kita T, Kada S, Yoshimoto M, Nakahata T, et al. Bone marrow-derived cells expressing Iba1 are constitutively present as resident tissue macrophages in the mouse cochlea. J Neurosci Res. (2008) 86:1758–67. doi: 10.1002/jnr.21625
32. Okano T. Immune system and resident macrophages in the inner ear. J Japan Soc Immunol Allergol Otolaryngol. (2018) 36:233–8. doi: 10.5648/jjiao.36.233
33. Ma WT, Gao F, Gu K, Chen DK. The role of monocytes and macrophages in autoimmune diseases: a comprehensive review. Front Immunol. (2019) 10:1140. doi: 10.3389/fimmu.2019.01140
34. Hough K, Verschuur CA, Cunningham C, Newman TA. Macrophages in the cochlea; an immunological link between risk factors and progressive hearing loss. Glia. (2021) 70:219–38. doi: 10.1002/glia.24095
35. Shi X. Pathophysiology of the cochlear intrastrial fluid-blood barrier (review). Hear Res. (2016) 338:52–63. doi: 10.1016/j.heares.2016.01.010
36. Shi X. Resident macrophages in the cochlear blood-labyrinth barrier and their renewal via migration of bone-marrow-derived cells. Cell Tissue Res. (2010) 342:21–30. doi: 10.1007/s00441-010-1040-2
37. Hirose K, Li SZ. The role of monocytes and macrophages in the dynamic permeability of the blood-perilymph barrier. Hear Res. (2019) 374:49–57. doi: 10.1016/j.heares.2019.01.006
38. Hashimoto D, Chow A, Noizat C, Teo P, Beasley MB, Leboeuf M, et al. Tissue-resident macrophages self-maintain locally throughout adult life with minimal contribution from circulating monocytes. Immunity. (2013) 38:792–804. doi: 10.1016/j.immuni.2013.04.004
39. Hayashi Y, Suzuki H, Nakajima W, Uehara I, Tanimura A, Himeda T, et al. Cochlear supporting cells function as macrophage-like cells and protect audiosensory receptor hair cells from pathogens. Sci Rep. (2020) 10:1–13. doi: 10.1038/s41598-020-63654-9
40. Zhang W, Dai M, Fridberger A, Hassan A, DeGagne J, Neng L, et al. Perivascular-resident macrophage-like melanocytes in the inner ear are essential for the integrity of the intrastrial fluid-blood barrier. Proc Natl Acad Sci U S A. (2012) 109:10388–93. doi: 10.1073/pnas.1205210109
41. Neng L, Zhang F, Kachelmeier A, Shi X. Endothelial cell, pericyte, and perivascular resident macrophage-type melanocyte interactions regulate cochlear intrastrial fluid-blood barrier permeability. JARO J Assoc Res Otolaryngol. (2013) 14:175–85. doi: 10.1007/s10162-012-0365-9
42. Navegantes KC, Souza Gomes R, Pereira PAT, Czaikoski PG, Azevedo CHM, Monteiro MC. Immune modulation of some autoimmune diseases: the critical role of macrophages and neutrophils in the innate and adaptive immunity. J Transl Med. (2017) 15:1–21. doi: 10.1186/s12967-017-1141-8
43. Moore KJ, Sheedy FJ, Fisher EA. Macrophages in atherosclerosis: a dynamic balance. Nat Rev Immunol. (2013) 13:709–21. doi: 10.1038/nri3520
44. Ma WT, Chang C, Gershwin ME, Lian ZX. Development of autoantibodies precedes clinical manifestations of autoimmune diseases: a comprehensive review. J Autoimmun. (2017) 83:95–112. doi: 10.1016/j.jaut.2017.07.003
45. Murray PJ, Allen JE, Biswas SK, Fisher EA, Gilroy DW, Goerdt S, et al. Macrophage activation and polarization: nomenclature and experimental guidelines. Immunity. (2014) 41:14–20. doi: 10.1016/j.immuni.2014.06.008
46. Mills CD, Kincaid K, Alt JM, Heilman MJ, Hill AM. M-1/M-2 macrophages and the Th1/Th2 paradigm. J Immunol. (2000) 164:6166–73. doi: 10.4049/jimmunol.164.12.6166
47. Funes SC, Rios M, Escobar-Vera J, Kalergis AM. Implications of macrophage polarization in autoimmunity. Immunology. (2018) 154:186–95. doi: 10.1111/imm.12910
48. Mallucci G, Peruzzotti-Jametti L, Bernstock JD, Pluchino S. The role of immune cells, glia and neurons in white and gray matter pathology in multiple sclerosis. Prog Neurobiol. (2015) 127–8:1–22. doi: 10.1016/j.pneurobio.2015.02.003
49. Van Der Valk P, De Groot CJA. Staging of multiple sclerosis (MS) lesions: pathology of the time frame of MS. Neuropathol Appl Neurobiol. (2000) 26:2–10. doi: 10.1046/j.1365-2990.2000.00217.x
50. Inoue M, Williams KL, Gunn MD, Shinohara ML. NLRP3 inflammasome induces chemotactic immune cell migration to the CNS in experimental autoimmune encephalomyelitis. Proc Natl Acad Sci U S A. (2012) 109:10480–5. doi: 10.1073/pnas.1201836109
51. Holz K, Prinz M, Brendecke SM, Hölscher A, Deng F, Mitrücker HW, et al. Differing outcome of experimental autoimmune encephalitis in macrophage/neutrophil- and T cell-specific gp130-deficient mice. Front Immunol. (2018) 9:836. doi: 10.3389/fimmu.2018.00836
52. Kigerl KA, Gensel JC, Ankeny DP, Alexander JK, Donnelly DJ, Popovich PG. Identification of two distinct macrophage subsets with divergent effects causing either neurotoxicity or regeneration in the injured mouse spinal cord. J Neurosci. (2009) 29:13435–44. doi: 10.1523/JNEUROSCI.3257-09.2009
53. Yamasaki R, Lu H, Butovsky O, Ohno N, Rietsch AM, Cialic R, et al. Differential roles of microglia and monocytes in the inflamed central nervous system. J Exp Med. (2014) 211:1533–49. doi: 10.1084/jem.20132477
54. Sahu R, Bethunaickan R, Singh S, Davidson A. Structure and function of renal macrophages and dendritic cells from lupus-prone mice. Arthritis Rheumatol. (2014) 66:1596–607. doi: 10.1002/art.38410
55. Okunuki Y, Mukai R, Nakao T, Tabor SJ, Butovsky O, Dana R, et al. Retinal microglia initiate neuroinflammation in ocular autoimmunity. Proc Natl Acad Sci U S A. (2019) 116:9989–98. doi: 10.1073/pnas.1820387116
56. Crane IJ, Liversidge J. Mechanisms of leukocyte migration across the blood-retina barrier. Semin Immunopathol. (2008) 30:165–77. doi: 10.1007/s00281-008-0106-7
57. Laria A, Lurati A, Marrazza M, Mazzocchi D, Re KA, Scarpellini M. The macrophages in rheumatic diseases. J Inflamm Res. (2016) 9:1–11. doi: 10.2147/JIR.S82320
58. Goodall AF, Siddiq MA. Current understanding of the pathogenesis of autoimmune inner ear disease: A review. Clin Otolaryngol. (2015) 40:412–9. doi: 10.1111/coa.12432
59. Bloch DB, San Martin JE, Rauch SD, Moscicki RA, Bloch KJ. Serum antibodies to heat shock protein 70 in sensorineural hearing loss. Arch Otolaryngol Neck Surg. (1995) 121:1167–71. doi: 10.1001/archotol.1995.01890100075013
60. Li G, You D, Ma J, Li W, Li H, Sun S. The role of autoimmunity in the pathogenesis of sudden sensorineural hearing loss. Neural Plast. (2018) 2018:7691473. doi: 10.1155/2018/7691473
61. Fujioka M, Okano H, Ogawa K. Inflammatory and immune responses in the cochlea: Potential therapeutic targets for sensorineural hearing loss. Front Pharmacol. (2014) 5:287. doi: 10.3389/fphar.2014.00287
62. Frye MD, Yang W, Zhang C, Xiong B, Hu BH. Dynamic activation of basilar membrane macrophages in response to chronic sensory cell degeneration in aging mouse cochleae. Hear Res. (2017) 344:125–34. doi: 10.1016/j.heares.2016.11.003
63. Bank LM, Bianchi LM, Ebisu F, Lerman-Sinkoff D, Smiley EC, Shen YC, et al. Macrophage migration inhibitory factor acts as a neurotrophin in the developing inner ear. Development. (2012) 139:4666–74. doi: 10.1242/dev.066647
64. Kämpfe Nordström C, Danckwardt-Lillieström N, Laurell G, Liu W, Rask-Andersen H. The human endolymphatic sac and inner ear immunity: macrophage interaction and molecular expression. Front Immunol. (2018) 9:3181. doi: 10.3389/fimmu.2018.03181
65. Noonan KY, Lopez IA, Ishiyama G, Ishiyama A. Immune response of macrophage population to cochlear implantation: cochlea immune cells. Otol Neurotol. (2020) 41:1288–95. doi: 10.1097/MAO.0000000000002764
66. He W, Yu J, Sun Y, Kong W. Macrophages in noise-exposed cochlea: changes, regulation and the potential role. Aging Dis. (2020) 11:191–9. doi: 10.14336/AD.2019.0723
67. Zhang Y, Li Y, Fu X, Wang P, Wang Q, Meng W, et al. The detrimental and beneficial functions of macrophages after cochlear injury. Front Cell Dev Biol. (2021) 9:2122. doi: 10.3389/fcell.2021.631904
68. Wood MB, Zuo J. The contribution of immune infiltrates to ototoxicity and cochlear hair cell loss. Front Cell Neurosci. (2017) 11:106. doi: 10.3389/fncel.2017.00106
69. Hirose K, Discolo CM, Keasler JR, Ransohoff R. Mononuclear phagocytes migrate into the murine cochlea after acoustic trauma. J Comp Neurol. (2005) 489:180–94. doi: 10.1002/cne.20619
70. Zhang N, Cai J, Xu L, Wang H, Liu W. Cisplatin-induced stria vascularis damage is associated with inflammation and fibrosis. Neural Plast. (2020) 2020:8851525. doi: 10.1155/2020/8851525
71. Warchol ME, Schrader A, Sheets L. Macrophages respond rapidly to ototoxic injury of lateral line hair cells but are not required for hair cell regeneration. Front Cell Neurosci. (2021) 14:454. doi: 10.3389/fncel.2020.613246
72. Bermúdez-Muñoz JM, Celaya AM, Hijazo-Pechero S, Wang J, Serrano M, Varela-Nieto I. G6PD overexpression protects from oxidative stress and age-related hearing loss. Aging Cell. (2020) 19:e13275. doi: 10.1111/acel.13275
73. Kaur T, Zamani D, Tong L, Rube EW, Ohlemiller KK, Hirose K, et al. Fractalkine signaling regulates macrophage recruitment into the cochlea and promotes the survival of spiral ganglion neurons after selective hair cell lesion. J Neurosci. (2015) 35:15050–61. doi: 10.1523/JNEUROSCI.2325-15.2015
74. Frejo L, Lopez-Escamez JA. Cytokines and inflammation in Meniere disease. Clin Exp Otorhinolaryngol. (2022) 15:49–59. doi: 10.21053/ceo.2021.00920
75. Frejo L, Gallego-Martinez A, Requena T, Martin-Sanz E, Amor-Dorado JC, Soto-Varela A, et al. Proinflammatory cytokines and response to molds in mononuclear cells of patients with Meniere disease. Sci Rep. (2018) 8:1–11. doi: 10.1038/s41598-018-23911-4
76. Flook M, Frejo L, Gallego-Martinez A, Martin-Sanz E, Rossi-Izquierdo M, Amor-Dorado JC, et al. Differential proinflammatory signature in vestibular migraine and meniere disease. Front Immunol. (2019) 10:1229. doi: 10.3389/fimmu.2019.01229
77. Moleon MDC, Martinez-Gomez E, Flook M, Peralta-Leal A, Gallego JA, Sanchez-Gomez H, et al. Clinical and cytokine profile in patients with early and late onset meniere disease. J Clin Med. (2021) 10:4052. doi: 10.3390/jcm10184052
78. Frejo L, Requena T, Okawa S, Gallego-Martinez A, Martinez-Bueno M, Aran I, et al. Regulation of Fn14 receptor and NF-κB underlies inflammation in Meniere's disease. Front Immunol. (2017) 8:1739. doi: 10.3389/fimmu.2017.01739
79. Lopez-Escamez JA, Batuecas-Caletrio A, Bisdorff A. Towards personalized medicine in Ménière's disease. F1000Res. (2018) 7:F1000 Faculty Rev-1295. doi: 10.12688/f1000research.14417.1
80. Iinuma R, Okuda H, Obara N, Matsubara Y, Aoki M, Ogawa T. Increased monocyte chemotactic protein-1 accompanying pro-inflammatory processes are associated with progressive hearing impairment and bilateral disability of Meniere's disease. Audiol Neurotol. (2021) 1–9. doi: 10.1159/000518839
81. Nakanishi H, Kawashima Y, Kurima K, Chae JJ, Ross AM, Pinto-Patarroyo G, et al. NLRP3 mutation and cochlear autoinflammation cause syndromic and nonsyndromic hearing loss DFNA34 responsive to anakinra therapy. Proc Natl Acad Sci U S A. (2017) 114:E7766–75. doi: 10.1073/pnas.1702946114
82. Gattorno M, Tassi S, Carta S, Delfino L, Ferlito F, Pelagatti MA, et al. Pattern of interleukin-1β secretion in response to lipopolysaccharide and ATP before and after interleukin-1 blockade in patients with CIAS1 mutations. Arthritis Rheum. (2007) 56:3138–48. doi: 10.1002/art.22842
83. Nakanishi H, Prakash P, Ito T, Kim HJ, Brewer CC, Harrow D, et al. Genetic hearing loss associated with autoinflammation. Front Neurol. (2020) 11:141. doi: 10.3389/fneur.2020.00141
84. Vambutas A, Lesser M, Mullooly V, Pathak S, Zahtz G, Rosen L, et al. Early efficacy trial of anakinra in corticosteroid-resistant autoimmune inner ear disease. J Clin Invest. (2014) 124:4115–22. doi: 10.1172/JCI76503
85. Polat E, Çinar Z, Keskindemirci G, Yigit Ö, Kutluk G, Türe M, et al. Assessment of hearing function in children with inflammatory bowel disease. J Int Adv Otol. (2020) 16:362–6. doi: 10.5152/iao.2020.6035
86. Fousekis FS, Saridi M, Albani E, Daniel F, Katsanos KH, Kastanioudakis IG, et al. Ear involvement in inflammatory bowel disease: a review of the literature. J Clin Med Res. (2018) 10:609–14. doi: 10.14740/jocmr3465w
87. Dettmer M, Hegemann I, Hegemann SCA. Extraintestinal Crohn's disease mimicking autoimmune inner ear disease: a histopathological approach. Audiol Neurotol. (2010) 16:36–40. doi: 10.1159/000315063
88. Kessel A, Vadasz Z, Toubi E. Cogan syndrome - pathogenesis, clinical variants and treatment approaches. Autoimmun Rev. (2014) 13:351–4. doi: 10.1016/j.autrev.2014.01.002
89. Jung DH, Nadol JB, Folkerth RD, Merola JF. Histopathology of the inner ear in a case with recent onset of Cogan's syndrome: evidence for vasculitis. Ann Otol Rhinol Laryngol. (2016) 125:20–4. doi: 10.1177/0003489415595426
90. Lunardi C, Bason C, Leandri M, Navone R, Lestani M, Millo E, et al. Autoantibodies to inner ear and endothelial antigens in Cogan's syndrome. Lancet. (2002) 360:915–21. doi: 10.1016/S0140-6736(02)11028-2
91. Shamriz O, Tal Y, Gross M. Autoimmune inner ear disease: Immune biomarkers, audiovestibular aspects, and therapeutic modalities of cogan's syndrome. J Immunol Res. (2018) 2018:1498640. doi: 10.1155/2018/1498640
92. Ogino T, Nishimura J, Barman S, Kayama H, Uematsu S, Okuzaki D, et al. Increased Th17-inducing activity of CD14+ CD163low myeloid cells in intestinal lamina propria of patients with Crohn's disease. Gastroenterology. (2013) 145:1380–91. doi: 10.1053/j.gastro.2013.08.049
93. Smith AM, Rahman FZ, Hayee B, Graham SJ, Marks DJB, Sewell GW, et al. Disordered macrophage cytokine secretion underlies impaired acute inflammation and bacterial clearance in Crohn's disease. J Exp Med. (2009) 206:1883–97. doi: 10.1084/jem.20091233
94. Seo DH, Che X, Kwak MS, Kim S, Kim JH, Ma HW, et al. Interleukin-33 regulates intestinal inflammation by modulating macrophages in inflammatory bowel disease. Sci Rep. (2017) 7:1–3. doi: 10.1038/s41598-017-00840-2
95. Zhu Y, Li X, Chen J, Chen T, Shi Z, Lei M, et al. The pentacyclic triterpene Lupeol switches M1 macrophages to M2 and ameliorates experimental inflammatory bowel disease. Int Immunopharmacol. (2016) 30:74–84. doi: 10.1016/j.intimp.2015.11.031
96. Fujioka M, Kanzaki S, Okano HJ, Masuda M, Ogawa K, Okano H. Proinflammatory cytokines expression in noise-induced damaged cochlea. J Neurosci Res. (2006) 83:575–83. doi: 10.1002/jnr.20764
97. Tan WJT, Thorne PR, Vlajkovic SM. Characterisation of cochlear inflammation in mice following acute and chronic noise exposure. Histochem Cell Biol. (2016) 146:219–30. doi: 10.1007/s00418-016-1436-5
98. Miao X, Leng X, Zhang Q. The current state of nanoparticle-induced macrophage polarization and reprogramming research. Int J Mol Sci. (2017) 18:336. doi: 10.3390/ijms18020336
99. Gaspar N, Zambito G, Löwik CMWG, Mezzanotte L. Active nano-targeting of macrophages. Curr Pharm Des. (2019) 25:1951–61. doi: 10.2174/1381612825666190710114108
100. Ardura JA, Rackov G, Izquierdo E, Alonso V, Gortazar AR, Escribese MM. Targeting macrophages: Friends or foes in disease? Front Pharmacol. (2019) 10:1255. doi: 10.3389/fphar.2019.01255
Keywords: macrophages, monocytes, heterogeneity, autoimmune inner ear diseases, autoinflammatory diseases, colony-stimulating factor 1
Citation: Miwa T and Okano T (2022) Role of Inner Ear Macrophages and Autoimmune/Autoinflammatory Mechanisms in the Pathophysiology of Inner Ear Disease. Front. Neurol. 13:861992. doi: 10.3389/fneur.2022.861992
Received: 25 January 2022; Accepted: 02 March 2022;
Published: 06 April 2022.
Edited by:
Jose Antonio Lopez-Escamez, Universidad de Granada, SpainReviewed by:
Katharina Stölzel, University Medical Center Hamburg-Eppendorf, GermanyIvan a Lopez, University of California, Los Angeles, United States
Copyright © 2022 Miwa and Okano. This is an open-access article distributed under the terms of the Creative Commons Attribution License (CC BY). The use, distribution or reproduction in other forums is permitted, provided the original author(s) and the copyright owner(s) are credited and that the original publication in this journal is cited, in accordance with accepted academic practice. No use, distribution or reproduction is permitted which does not comply with these terms.
*Correspondence: Toru Miwa, dC1taXdhQGtpdGFuby1ocC5vci5qcA==