- 1Telethon Kids Institute, University of Western Australia, Nedlands, WA, Australia
- 2Centre for Neuromuscular and Neurological Disorders, Perron Institute for Neurological and Translational Science, University of Western Australia, Perth, WA, Australia
The immunological mechanisms that contribute to multiple sclerosis (MS) differ between males and females. Females are 2–3 times more likely to develop MS compared to males, however the reason for this discrepancy is unknown. Once MS is established, there is a more inflammatory yet milder form of disease in females whereas males generally suffer from more severe disease and faster progression, neural degradation, and disability. Some of these differences relate to genetics, including genetic control of immune regulatory genes on the X-chromosome, as well as immune modulatory properties of sex hormones. Differences in MS development may also relate to how sex interacts with environmental risk factors. There are several environmental risk factors for MS including late-onset Epstein Barr virus infection, low serum vitamin D levels, low UV radiation exposure, smoking, obesity, and lack of physical activity. Most of these risk factors impact males and females differently, either due to biological or immunological processes or through behavioral differences. In this review, we explore these differences further and focus on how the interaction of environmental risk factors with sex hormones may contribute to significantly different prevalence and pathology of MS in males and females.
Introduction
The immunological impact of biological sex is highlighted by significantly different prevalences of immune mediated diseases between males and females. Males are more susceptible to infectious diseases, whereas females are more susceptible to autoimmune diseases such as multiple sclerosis (MS) and systemic lupus erythematosus (SLE) (1). The impact of sex on disease prevalence stems from both genetic and environmental factors as well as their interaction. Genetically, several immunologically important genes are expressed from the X-chromosome such as TLR7 and FoxP3 (2). Environmentally, sex hormones are potent immune modulators and exert their function through binding to extra- and intra-cellular receptors, widely expressed across the immune system. Sex differences in responses to environmental cues may influence the initiation and/or maintenance of autoimmune diseases. The genetic differences in how the immune system operates have been reviewed in detail elsewhere (1) as well as in the context of MS (3). This review will focus on the specific impact of sex hormones on the immune system and their interaction with environmental risk factors. The sex-specific impact of each environmental factor is summarized in (Figure 1).
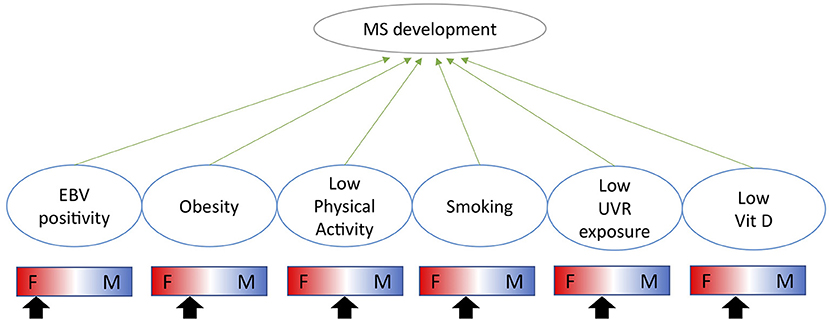
Figure 1. Indication of how each environmental risk factor impacts males and females differently and whether each factor is likely to increase the risk of MS more in females (F) or males (M) as indicated by the arrow.
Multiple Sclerosis
MS is a chronic inflammatory and neurodegenerative disease resulting in demyelination of neuron fibers, inefficient signal transmission and reduced muscular mobility. MS affects approximately 30 in 100,000 globally (4) and ~100 in 100,000 individuals in Australia (5). Prevalence of MS has increased over the last decades, particularly in females (6) who are now 2–3 times more likely to develop MS than males (6, 7). At the beginning of the century, prevalence was estimated to be proportional between males and females (8), suggesting that changes to environmental factors likely have impacted MS development during the last decades.
There are two forms of MS. The majority of individuals develop remittent relapsing MS (RRMS) where periods of disease activity (flare-ups) are followed by periods of low-symptomatic activity or absence of symptoms (remissions) (9). In 10–15% of patients, primary progressive MS is established and results in faster deterioration with no episodes of remission. RRMS is significantly more common in females compared to males, whereas there is less difference in incidence for primary progressive MS (10). In RRMS, females display elevated levels of inflammation in the CNS compared to males (11) whereas males display signs of more severe neurodegeneration and more disabling manifestations (11, 12). Over time RRMS converts into secondary progressive MS where episodes of remission cease; this appears to occur faster in males compared to females (12). As the disease progresses, MS leads to a debilitating lack of muscular mobility and cognitive deterioration. The main therapies involve disease modifying drugs including interferon (IFN)-β and biologicals such as B cell depleting monoclonals. Available treatments can slow disease progression, but no treatment is yet able to prevent or reverse disease progression.
There are several risk factors for MS, including genetic polymorphisms, relating mainly to variants in HLA-DR, where HLA-DRB1*15:01 has the strongest link with increased risk of MS (13). There are also several established environmental risk factors including Epstein-Barr virus (EBV) infection, low UV radiation (UVR) exposure, vitamin D deficiency, smoking, obesity and reduced physical exercise (14–16). How these impact MS risk and pathology in male and females will be discussed below.
Immune Mechanisms of MS
Traditionally, MS is viewed as a T cell dependent disease, particularly driven by activated and clonally expanded CD8+ T cells that are found in CNS lesions. These are likely activated in the periphery, potentially by an underlying viral infection such as Epstein-Barr virus (EBV). Target antigens in the CNS remain elusive but T cells specific for myelin-associated proteins are regularly observed (17). CD4+ T cells, mainly Th1, Th17 and Tregs, are also involved in MS pathology as recently reviewed (18). Their involvement likely explains the strong association of MS and HLA-DR haplotypes (19). MS lesions also contain a considerable proportion of B cells and monocytes. The role of the B cells in MS is still emerging (20). Increased IgGs are commonly observed in CSF from MS patients, however their contribution to MS pathology remain unclear (21). Instead, antibody-independent functions of B cells, such as antigen presentation or cytokine production may be more relevant (22). Evidence to support this stems from observations that MS patients benefit rapidly from anti-CD20 therapy that depletes several B cell subsets except antibody-producing plasma cells that lack CD20 expression (23).
Impact of Sex Hormones on the Immune System
The contribution of sex hormones during the development of MS remains unclear (24). Sex hormones have several distinct effects across the immune system that are relevant for MS pathology. Estrogen can promote either Th1 (25) or Th2 (26) differentiation at low or high concentrations, respectively. Estrogen may also expand FoxP3+ Tregs (27) while suppressing Th17 cells as demonstrated in an EAE model (28). Estrogen is essential for generation of inflammatory DC (29) and may interfere with B cell selection allowing for the escape of autoreactive B cells (30). Progesterone appears to prevent inflammation through reducing the activation of DC (31, 32), and inhibits Th1 and Th17 differentiation (33) whilst promoting Th2 immunity at high concentrations (34). This is likely important for fetus tolerance during pregnancy. Testosterone has general anti-inflammatory effects including dampening of macrophage and DC activation (35) and inhibition of Th1 immune differentiation (36, 37).
Impact by Sex Hormones on MS Pathology
In MS, the female sex hormones, estrogen and progesterone, appear to have protective effects; this is surprising given the increased prevalence of MS in females. However, this is supported by low risk of relapse during pregnancy (38) and the subsequent increased risk of relapse once pregnancy hormones return to normal (38). Decreased levels of estrogen are also associated with disease relapse in female patients (39). The impact of oral contraceptives remains unclear (40). Attempts to reduce MS symptoms through administration of estrogen derivates have shown some promise (41, 42), however further studies are required. Progesterone has been suggested to promote myelination in the CNS (43, 44) however does not appear to have an effect on relapse rate as reviewed (45). Not all female specific hormones may be beneficial in MS as exemplified by prolactin which is increased in female MS patients and promotes formation of autoreactive B cells (46). In male MS patients, up to 40% display decreased levels of testosterone compared to matched controls (47). Pilot studies further suggest that testosterone may ameliorate MS symptoms in males (48), however the mechanisms remain unclear but are likely mediated through the immune regulatory properties of testosterone as described above.
Given the importance of sex hormones in MS pathology, transgender individuals who take sex hormones to align their body with their gender may be subject to potential changes in risk of MS and disease pathology. Although this is a neglected area of research, a clinical records-based study identified an increased incidence of MS in trans individuals who were assigned as males at birth (49). These individuals are likely to be on estrogen supplementation or anti-testosterone treatment. No change in risk was observed for trans individuals who were assigned as females at birth and likely received testosterone treatment.
Together, these observations suggest that although the main female sex hormones promote immune activation and females are at increased risk of developing MS, female sex hormones may still have a beneficial impact on MS pathology. Whether the increased prevalence of MS in females instead relates to differences in hormone receptor expression, genetic differences related to the X-chromosome or sex-specific mechanisms in the CNS, such as increased ability of microglia in females to induce inflammation in response to cell damage, remains to be explored (3). How sex hormones interact with environmental risk factors are discussed below.
Impact of Environmental Risk Factors on MS Risk in Males and Females
Do Female-Specific Viral Responses Contribute to Skewed MS Prevalence?
Epstein-Barr virus (EBV) causes a chronic infection of B cells, that reprograms resting B cells into a memory-like phenotype. Over 95% of the adult population have serological evidence of past EBV infection (50). EBV is associated with development of several autoimmune diseases and B cell lymphomas (51). It is one of the strongest environmental risk factors for MS development. If EBV is acquired during childhood, the risk of subsequent MS development is low. However, if EBV is acquired during adolescence or early adulthood, there is a significantly increased risk of MS development, particularly if the individual experiences severe disease or hospitalization (52, 53). Severity and EBV-specific antibody titres correlate directly to risk of MS development (54). Modeling suggests that the difference in risk between childhood and adolescence is related to puberty onset, pointing toward a role for sex hormones (55). Females display elevated levels of EBV reactive antibodies compared to males also suggesting a more robust EBV response in females (56, 57). Plasmacytoid DC (pDC) from females typically produce more type I IFN following virus exposure, particularly through TLR7 (58), which together with TLR9 (59) recognizes EBV RNA and DNA in infected cells (60). Type I IFN production is also directly regulated by estrogen and associated with serum testosterone levels (61, 62). EBV-induced type I IFN production is thought to contribute to SLE development (60), whereas in MS, the ability of pDC to produce type I IFN is reduced (63, 64). Interferon-β treatment is successfully used to reduce relapse frequency and delay neurological disability in MS (65, 66). Whether reduced type I IFN responsiveness is an endogenous deficiency in MS that predisposes an individual to more severe EBV infection or if it relates to EBV's ability to inhibit type I IFN signaling as a mechanism for avoiding the immune system (67) remains to be elucidated.
The Impact of BMI and Physical Activity on MS Risk in Males and Females
Obesity during childhood/adolescence (68, 69) or adulthood (70) as well as reduced physical activity (71, 72) have been independently associated with an elevated risk of MS. There appears to be no difference between the sexes in association with MS and obesity in young adolescents (68). However, in adults, the association between obesity and MS appears stronger in females compared to males (73). Obesity leads to chronic inflammation which may contribute to MS pathology. Obesity has dramatically different consequences in males and females (74, 75); estrogen has been implicated as a central modulator in these pathways (76) whereas testosterone is likely beneficial in reducing adipose-induced inflammation (77). The relevance of these mechanisms for MS development remains unknown.
Vigorous exercise during adolescence is associated with a reduced risk of MS (71, 72). Long-term moderate physical activity reduces inflammation and risk of infection over time (78, 79) and its impact on the immune system may differ between male and females (80). However, how sex hormones contribute has been less investigated and there is little evidence to suggest that physical activity impacts the risk of MS differently in males and females (71).
The Impact of Smoking and Nicotine on MS in Males and Females
Smoking increases the risk of developing MS, likely due to its inflammatory properties in the airways that translate into systemic and chronic inflammation (81). Smoking increases the risk of MS although one of the main components, nicotine, likely has protective effects (82). Once MS is established, smoking continues to contribute to MS pathology by increasing the rate of relapses and decreasing the time to progressive MS (83). Only a handful of studies have assessed the risk in males and females, with trends suggesting that historical smoking may increase the risk for MS more in males compared to females (82, 84). However, levels of nicotine metabolites in serum was more strongly associated with increased risk for MS in females suggesting instead that females may be more sensitive to smoking (85). That smoking has differential effects on males and females is long established (86) and recent studies also suggest that sex may be important in how smoking induces inflammation (86–88). Smoking may also impact sex hormone production and signaling (89). Increased rates of female smoking during the last decades, and possibly increased sensitivity to smoking metabolites, have been discussed as one of the reasons why MS has increased significantly faster in females compared to males over the last decades (85, 90).
UVR and Vitamin D – Their Impact on MS in Males and Females
Decreased exposure to UV radiation (UVR) and vitamin D deficiency are independent risk factors for MS (91). Exposure to UVR modulates several aspects of the immune system (92, 93) and is essential for generation of active vitamin D. Vitamin D can also be obtained through diet and vitamin D itself has a range of immune modulatory properties that may be beneficial for reducing the risk of MS and other autoimmune diseases (94). The impacts of UVR and/or vitamin D on MS risk are interconnected and translate into a gradual increase in MS prevalence with increasing latitudes (95). However, the association between increased vitamin D levels and reduced risk of MS appears most prominent in fair-skinned individuals whereas increased UVR exposure is associated with reduced risk across several ethnic groups (96, 97). Furthermore, because vitamin D levels correlate with UVR exposure in fair-but not dark-skinned individuals, it has been argued that MS protection is mediated through UVR exposure (97). Nevertheless, vitamin D is protective in EAE models, with dependency on genetic background (98) and several studies suggest that intake of high-vitamin D foods reduces the risk of MS (99). Clinical trials with vitamin D supplementation have shown mixed results (100). Regarding sex-specific effects, vitamin D supplementation mainly protects female mice from EAE (101) and human in vitro studies suggest that vitamin D is more effective in reducing CD4+ T cell proliferation in female, compared to male MS patients. The ability of vitamin D to induce FoxP3+ Tregs may also be dependent on estrogen (102). How these findings translate into clinical efficacy remains unclear. Isolated studies have reported that vitamin D is only associated with reduced disease incidence and severity in females compared to males (103). However, this requires further investigation as other studies report no sex-specific effects of vitamin D (104), or indeed no clinical benefit at all (100).
The direct impact of UVR exposure on MS pathology through its immunomodulatory properties has been less investigated (105–107). In mice, UVR reduces the risk of EAE through a vitamin D-independent mechanism (108). The interaction of UVR exposure and sex is unclear. Isolated studies suggest that UVR exposure may decrease the risk of MS more in females compared to males (109), and that latitude may contribute to the increase in females with MS (7) whereas others have found no difference in sex ratios across latitudes (110). UVR exposure may influence the production of sex hormones (111, 112) which recently were associated with hormone-induced behavioral changes (112). Whether the impact of UVR exposure on reducing MS risk and pathology is mediated through sex hormones remains to be investigated. There are a range of mechanisms proposed for how UVR exposure impacts the immune system (113) including induction of regulatory T cells, modulation of skin resident DC and recruitment of anti-inflammatory monocytes. UVR-induced immunoregulatory molecules such as cis-urocanic acid and nitric oxide may also be involved (92, 93) as may RNA-release from damaged keratinocytes (114). Our lab has demonstrated that UVR impacts the abundance of circulating B cell subsets and activation of TNF-producing B cells in patients with early MS (106, 115), unfortunately these studies were not powered to investigate the impact of UVR in males and females separately. Although not uniformly found (116), UVR exposure may also increase systemic type I IFN signaling (107), which may be beneficial for reducing MS manifestations.
Discussion
The impact of sex hormones on autoimmune disease is complex. Not all female-dominated autoimmune diseases improve during pregnancy or by supplemental sex hormones. A classic example is SLE which is ~8 times more common in females compared to males (117). The risk of a SLE flare during pregnancy appears increased (118) and hormone replacement therapy increases the risk of mild flares (119). However, contraception does not significantly impact disease activity (120). Both SLE and MS are associated with previous EBV infection, reduced physical activity, obesity, smoking and reduced levels of vitamin D (121–124). However, the impact of sex hormones and UVR exposure differs substantially (125). Whereas, estrogen likely is beneficial in MS, the impact on SLE is less clear. UVR exposure is also beneficial in MS but in SLE, UVR exposure leads to a significant increase in manifestations, including skin rashes, and sun exposure can trigger disease flares (126). Whether this relates to an increase in dying cells from UVR exposure or UVR-induced type I IFN signaling (127), remains to be clarified. Notably, type I IFN signaling was upregulated in several immune cell subsets in MS patients treated with UVR (107) suggesting this may be a common response to UVR exposure. Avoidance of sun exposure is likely also responsible for the low levels of vitamin D observed in SLE patients.
With a change in environmental exposures, particularly in terms of increased pollution, airway irritants and reduced exposure to UVR through enhanced indoor lifestyles, the prevalence of MS may continue to increase. If women are more sensitive to these environmental exposures, we may continue to observe a disproportional increase of MS in females and possibly other autoimmune diseases.
Conclusion
The immunological impact of sex significantly contributes to an increased prevalence of autoimmune disease in females compared to males. However, the immunological mechanisms driving this development likely differ across diseases and may be related to both sex-specific genetic differences, immunological impact of sex hormones and sex-specific responses to environmental stimuli. The influence on disease initiation and progression may vary. Further investigations into the impact of genetic sex and sex hormones on disease mechanisms are central. Targeting sex hormones and sex-specific inflammatory pathways as a strategy to decrease inflammation in autoimmune and rheumatic disease is becoming more popular (128–130). As has been detailed in this review, sex-specific mechanisms likely contribute to autoimmune disease through several independent pathways, and mapping these will be essential to better treat autoimmune diseases in the future.
Author Contributions
JL drafted the manuscript. ST, SG, and PHH contributed to specific sections and edited the manuscript. All authors contributed to the article and approved the submitted version.
Funding
The authors would like to acknowledge funding support from Multiple Sclerosis WA.
Conflict of Interest
The authors declare that the research was conducted in the absence of any commercial or financial relationships that could be construed as a potential conflict of interest.
Publisher's Note
All claims expressed in this article are solely those of the authors and do not necessarily represent those of their affiliated organizations, or those of the publisher, the editors and the reviewers. Any product that may be evaluated in this article, or claim that may be made by its manufacturer, is not guaranteed or endorsed by the publisher.
References
1. Klein SL, Flanagan KL. Sex differences in immune responses. Nat Rev Immunol. (2016) 16:626–38. doi: 10.1038/nri.2016.90
2. Bianchi I, Lleo A, Gershwin ME, Invernizzi P. The X chromosome and immune associated genes. J Autoimmun. (2012) 38:J187–92. doi: 10.1016/j.jaut.2011.11.012
3. Gilli F, DiSano KD, Pachner AR. SeXX Matters in multiple sclerosis. Front Neurol. (2020) 11:616. doi: 10.3389/fneur.2020.00616
4. Feigin VL, Nichols E, Alam T, Bannick MS, Beghi E, N. Blake, et al. Global, regional, and national burden of neurological disorders, 1990–2016: a systematic analysis for the Global Burden of Disease Study 2016. Lancet Neurol. (2019) 18:459–480. doi: 10.1016/S1474-4422(18)30499-X
5. Campbell JA, Simpson S, Ahmad H, Taylor BV, van der Mei I, Palmer AJ. Change in multiple sclerosis prevalence over time in Australia 2010-2017 utilising disease-modifying therapy prescription data. Mult Scler. (2020) 26:1315–28. doi: 10.1177/1352458519861270
6. Orton S-M, Herrera BM, Yee IM, Valdar W, Ramagopalan SV, Sadovnick AD, et al. Sex ratio of multiple sclerosis in Canada: a longitudinal study. Lancet Neurol. (2006) 5:932–6. doi: 10.1016/S1474-4422(06)70581-6
7. Trojano M, Lucchese G, Graziano G, Taylor BV, Simpson S Jr, et al. Geographical variations in sex ratio trends over time in multiple sclerosis. PLoS ONE. (2012) 7:e48078. doi: 10.1371/journal.pone.0048078
8. Talley CL. The emergence of multiple sclerosis, 1870-1950: a puzzle of historical epidemiology. Perspect Biol Med. (2005) 48:383–95. doi: 10.1353/pbm.2005.0079
9. Sospedra M, Martin R. Antigen-specific therapies in multiple sclerosis. Int Rev Immunol. (2005) 24:393–413. doi: 10.1080/08830180500371256
10. Kalincik T, Vivek V, Jokubaitis V, Lechner-Scott J, Trojano M, Izquierdo G, et al. Sex as a determinant of relapse incidence and progressive course of multiple sclerosis. Brain. (2013) 136:3609–17. doi: 10.1093/brain/awt281
11. Bove R, Chitnis T. Sexual disparities in the incidence and course of MS. Clin Immunol. (2013) 149:201–10. doi: 10.1016/j.clim.2013.03.005
12. Aktas O, Ribbons KA, McElduff P, Boz C, Trojano M, Izquierdo G, et al. Male sex is independently associated with faster disability accumulation in relapse-onset MS but not in primary progressive MS. PLoS ONE. (2015) 10:e0122686. doi: 10.1371/journal.pone.0122686
13. Schmidt H, Williamson D, Ashley-Koch A. HLA-DR15 haplotype and multiple sclerosis: a HuGE review. Am J Epidemiol. (2007) 165:1097–109. doi: 10.1093/aje/kwk118
14. Ebers GC, Environmental factors and multiple sclerosis. Lancet Neurol. (2008) 7:268–77. doi: 10.1016/S1474-4422(08)70042-5
15. Yuan S, Xiong Y, Larsson SC. An atlas on risk factors for multiple sclerosis: a Mendelian randomization study. J Neurol. (2021) 268:114–24. doi: 10.1007/s00415-020-10119-8
16. Olsson T, Barcellos LF, Alfredsson L. Interactions between genetic, lifestyle and environmental risk factors for multiple sclerosis. Nat Rev Neurol. (2017) 13:25–36. doi: 10.1038/nrneurol.2016.187
17. Salou M, Nicol B, Garcia A, Laplaud DA. Involvement of CD8(+) T Cells in multiple sclerosis. Front Immunol. (2015) 6:604. doi: 10.3389/fimmu.2015.00604
18. Haase S, Linker RA. Inflammation in multiple sclerosis. Ther Adv Neurol Disord. (2021) 14:17562864211007687. doi: 10.1177/17562864211007687
19. Wagner CA, Goverman JM. Novel insights and therapeutics in multiple sclerosis. F1000Research. (2015) 4:517. doi: 10.12688/f1000research.6378.1
20. Li R, Patterson KR, Bar-Or A. Reassessing B cell contributions in multiple sclerosis. Nat Immunol. (2018) 19:696–707. doi: 10.1038/s41590-018-0135-x
21. Yu X, Zizzo Z, Kennedy PG. An appraisal of antigen identification and IgG effector functions driving host immune responses in multiple sclerosis. Mult Scler Relat Disord. (2021) 56:103328. doi: 10.1016/j.msard.2021.103328
22. van Langelaar J, Rijvers L, Smolders J, van Luijn MM. B and T Cells driving multiple sclerosis: identity, mechanisms and potential triggers. Front Immunol. (2020) 11:760. doi: 10.3389/fimmu.2020.00760
23. Hauser SL, Waubant E, Arnold DL, Vollmer T, Antel J, Fox RJ, et al. B-cell depletion with rituximab in relapsing-remitting multiple sclerosis. N Engl J Med. (2008) 358:676–88. doi: 10.1056/NEJMoa0706383
24. El-Etr M, Ghoumari A, Sitruk-Ware R, Schumacher M. Hormonal influences in multiple sclerosis: new therapeutic benefits for steroids. Maturitas. (2011) 68:47–51. doi: 10.1016/j.maturitas.2010.09.014
25. Karpuzoglu E, Phillips RA, Gogal RM Jr, Ansar Ahmed S. IFN-gamma-inducing transcription factor, T-bet is upregulated by estrogen in murine splenocytes: role of IL-27 but not IL-12. Mol Immunol. (2007) 44:1808–14. doi: 10.1016/j.molimm.2006.08.005
26. Navarro FC, Watkins SK. Estrogen stimulation differentially impacts human male and female antigen-specific T cell anti-tumor function and polyfunctionality. Gend Genome. (2017) 1:1–13. doi: 10.1089/gg.2017.0014
27. Polanczyk MJ, Carson BD, Subramanian S, Afentoulis M, Vandenbark AA, Ziegler SF, et al. Cutting edge: estrogen drives expansion of the CD4+CD25+ regulatory T cell compartment. J Immunol. (2004) 173:2227–30. doi: 10.4049/jimmunol.173.4.2227
28. Wang C, Dehghani B, Li Y, Kaler LJ, Vandenbark AA, Offner H. Oestrogen modulates experimental autoimmune encephalomyelitis and interleukin-17 production via programmed death 1. Immunology. (2009) 126:329–35. doi: 10.1111/j.1365-2567.2008.03051.x
29. Seillet C, Rouquie N, Foulon E, Douin-Echinard V, Krust A, Chambon P, et al. Estradiol promotes functional responses in inflammatory and steady-state dendritic cells through differential requirement for activation function-1 of estrogen receptor alpha. J Immunol. (2013) 190:5459–70. doi: 10.4049/jimmunol.1203312
30. Grimaldi CM, Jeganathan V, Diamond B. Hormonal regulation of B cell development: 17 beta-estradiol impairs negative selection of high-affinity DNA-reactive B cells at more than one developmental checkpoint. J Immunol. (2006) 176:2703–10. doi: 10.4049/jimmunol.176.5.2703
31. Butts CL, Shukair SA, Duncan KM, Bowers E, Horn C, Belyavskaya E, et al. Progesterone inhibits mature rat dendritic cells in a receptor-mediated fashion. Int Immunol. (2007) 19:287–96. doi: 10.1093/intimm/dxl145
32. Jones LA, Kreem S, Shweash M, Paul A, Alexander J, Roberts CW. Differential modulation of TLR3- and TLR4-mediated dendritic cell maturation and function by progesterone. J Immunol. (2010) 185:4525–34. doi: 10.4049/jimmunol.0901155
33. AbdulHussain G, Azizieh F, Makhseed M, Raghupathy R. Effects of progesterone, dydrogesterone and estrogen on the production of Th1/Th2/Th17 cytokines by lymphocytes from women with recurrent spontaneous miscarriage. J Reprod Immunol. (2020) 140:103132. doi: 10.1016/j.jri.2020.103132
34. Piccinni MP, Giudizi MG, Biagiotti R, Beloni L, Giannarini L, Sampognaro S, et al. Progesterone favors the development of human T helper cells producing Th2-type cytokines and promotes both IL-4 production and membrane CD30 expression in established Th1 cell clones. J Immunol. (1995) 155:128–33.
35. Drake CG, Doody AD, Mihalyo MA, Huang CT, Kelleher E, Ravi S, et al. Androgen ablation mitigates tolerance to a prostate/prostate cancer-restricted antigen. Cancer Cell. (2005) 7:239–49. doi: 10.1016/j.ccr.2005.01.027
36. Bebo BF Jr, Schuster JC, Vandenbark AA, Offner H. Androgens alter the cytokine profile and reduce encephalitogenicity of myelin-reactive T cells. J Immunol. (1999) 162:35–40.
37. Kissick HT, Sanda MG, Dunn LK, Pellegrini KL, On ST, Noel JK, et al. Androgens alter T-cell immunity by inhibiting T-helper 1 differentiation. Proc Natl Acad Sci U S A. (2014) 111:9887–92. doi: 10.1073/pnas.1402468111
38. Confavreux C, Hutchinson M, Hours MM, Cortinovis-Tourniaire P, Moreau T. Rate of pregnancy-related relapse in multiple sclerosis. Pregnancy in multiple sclerosis group. N Engl J Med. (1998) 339:285–91. doi: 10.1056/NEJM199807303390501
39. Trenova AG, Slavov GS, Manova MG, Kostadinova II, Vasileva TV. Female sex hormones and cytokine secretion in women with multiple sclerosis. Neurol Res. (2013) 35:95–9. doi: 10.1179/1743132812Y.0000000120
40. Krysko KM, Graves JS, Dobson R, Altintas A, Amato MP, Bernard J, et al. Sex effects across the lifespan in women with multiple sclerosis. Ther Adv Neurol Disord. (2020) 13:1756286420936166. doi: 10.1177/1756286420936166
41. Sicotte NL, Liva SM, Klutch R, Pfeiffer P, Bouvier S, Odesa S, et al. Treatment of multiple sclerosis with the pregnancy hormone estriol. Ann Neurol. (2002) 52:421–8. doi: 10.1002/ana.10301
42. Voskuhl RR, Wang H, Wu TCJ, Sicotte NL, Nakamura K, Kurth F, et al. Estriol combined with glatiramer acetate for women with relapsing-remitting multiple sclerosis: a randomised, placebo-controlled, phase 2 trial. Lancet Neurol. (2016) 15:35–46. doi: 10.1016/S1474-4422(15)00322-1
43. Schumacher M, Hussain R, Gago N, Oudinet JP, Mattern C, Ghoumari AM. Progesterone synthesis in the nervous system: implications for myelination and myelin repair. Front Neurosci. (2012) 6:10. doi: 10.3389/fnins.2012.00010
44. I Jure, De Nicola AF, Labombarda F. Progesterone effects on the oligodendrocyte linage: all roads lead to the progesterone receptor. Neural Regen Res. (2019) 14:2029–34. doi: 10.4103/1673-5374.262570
45. Voskuhl R, Momtazee C. Pregnancy: effect on multiple sclerosis, treatment considerations, and breastfeeding. Neurotherapeutics. (2017) 14:974–84. doi: 10.1007/s13311-017-0562-7
46. Correale J, Farez MF, Ysrraelit MC. Role of prolactin in B cell regulation in multiple sclerosis. J Neuroimmunol. (2014) 269:76–86. doi: 10.1016/j.jneuroim.2014.02.007
47. Chitnis T. The role of testosterone in MS risk and course. Mult Scler. (2018) 24:36–41. doi: 10.1177/1352458517737395
48. Sicotte NL, Giesser BS, Tandon V, Klutch R, Steiner B, Drain AE, et al. Testosterone treatment in multiple sclerosis: a pilot study. Arch Neurol. (2007) 64:683–8. doi: 10.1001/archneur.64.5.683
49. Pakpoor J, Wotton CJ, Schmierer K, Giovannoni G, Goldacre MJ. Gender identity disorders and multiple sclerosis risk: a national record-linkage study. Mult Scler. (2016) 22:1759–62. doi: 10.1177/1352458515627205
50. Kuri A, Jacobs BM, Vickaryous N, Pakpoor J, Middeldorp J, Giovannoni G, et al. Epidemiology of Epstein-Barr virus infection and infectious mononucleosis in the United Kingdom. BMC Public Health. (2020) 20:912. doi: 10.1186/s12889-020-09049-x
51. Jha HC, Pei Y, Robertson ES. Epstein-Barr virus: diseases linked to infection and transformation. Front Microbiol. (2016) 7:1602. doi: 10.3389/fmicb.2016.01602
52. Ascherio A, Munger KL. Environmental risk factors for multiple sclerosis. Part I: the role of infection. Ann Neurol. (2007) 61:288–99. doi: 10.1002/ana.21117
53. Xu Y, Hiyoshi A, Smith KA, Piehl F, Olsson T, Fall K, et al. Association of infectious mononucleosis in childhood and adolescence with risk for a subsequent multiple sclerosis diagnosis among siblings. JAMA Netw Open. (2021) 4:e2124932. doi: 10.1001/jamanetworkopen.2021.24932
54. Sundström P, Juto P, Wadell G, Hallmans G, Svenningsson A, Nyström L, et al. An altered immune response to Epstein-Barr virus in multiple sclerosis. Neurology. (2004) 62:2277. doi: 10.1212/01.WNL.0000130496.51156.D7
55. Rostgaard K, Balfour HH Jr, Jarrett R, Erikstrup C, Pedersen O, et al. Primary Epstein-Barr virus infection with and without infectious mononucleosis. PLoS ONE. (2019) 14:e0226436. doi: 10.1371/journal.pone.0226436
56. Ford JL, Stowe RP. Racial-ethnic differences in Epstein-Barr virus antibody titers among children US, and adolescents. Ann Epidemiol. (2013) 23:275–80. doi: 10.1016/j.annepidem.2013.02.008
57. Wagner H-J, Hornef M, Teichert H-M, Kirchner H. Sex difference in the serostatus of adults to the epstein-barr virus. Immunobiology. (1994) 190:424–9. doi: 10.1016/S0171-2985(11)80613-3
58. Laffont S, Rouquie N, Azar P, Seillet C, Plumas J, Aspord C, et al. X-Chromosome complement and estrogen receptor signaling independently contribute to the enhanced TLR7-mediated IFN-alpha production of plasmacytoid dendritic cells from women. J Immunol. (2014) 193:5444–52. doi: 10.4049/jimmunol.1303400
59. Fiola S, Gosselin D, Takada K, Gosselin J. TLR9 contributes to the recognition of EBV by primary monocytes and plasmacytoid dendritic cells. J Immunol. (2010) 185:3620–31. doi: 10.4049/jimmunol.0903736
60. Quan TE, Roman RM, Rudenga BJ, Holers VM, Craft JE. Epstein-Barr virus promotes interferon-alpha production by plasmacytoid dendritic cells. Arthritis Rheum. (2010) 62:1693–701. doi: 10.1002/art.27408
61. Seillet C, Laffont S, Tremollieres F, Rouquie N, Ribot C, Arnal JF, et al. The TLR-mediated response of plasmacytoid dendritic cells is positively regulated by estradiol in vivo through cell-intrinsic estrogen receptor alpha signaling. Blood. (2012) 119:454–64. doi: 10.1182/blood-2011-08-371831
62. Webb K, Peckham H, Radziszewska A, Menon M, Oliveri P, Simpson F, et al. Sex and pubertal differences in the type 1 interferon pathway associate with both X chromosome number and serum sex hormone concentration. Front Immunol. (2019) 9:3167. doi: 10.3389/fimmu.2018.03167
63. Mycko MP, Cwiklinska H, Cichalewska M, Matysiak M, Lewkowicz P, Sliwinska B, et al. Plasmocytoid dendritic cell deficit of early response to toll-like receptor 7 agonist stimulation in multiple sclerosis patients. Clin Immunol. (2014) 153:211–9. doi: 10.1016/j.clim.2014.04.016
64. Borden EC, Sen GC, Uze G, Silverman RH, Ransohoff RM, Foster GR, et al. Interferons at age 50: past, current and future impact on biomedicine. Nature Reviews Drug Discovery. (2007) 6:975–90. doi: 10.1038/nrd2422
65. Jacobs L, O'Malley J, Freeman A, Ekes R. Intrathecal interferon reduces exacerbations of multiple sclerosis. Science. (1981) 214:1026–8. doi: 10.1126/science.6171035
66. Madsen C. The innovative development in interferon beta treatments of relapsing-remitting multiple sclerosis. Brain Behav. (2017) 7:e00696. doi: 10.1002/brb3.696
67. Xia C, Anderson P, Hahm B. Viral dedication to vigorous destruction of interferon receptors. Virology. (2018) 522:19–26. doi: 10.1016/j.virol.2018.06.017
68. Huppke B, Ellenberger D, Hummel H, Stark W, Robl M, Gartner J, et al. Association of obesity with multiple sclerosis risk and response to first-line disease modifying drugs in children. JAMA Neurol. (2019) 76:1157–65. doi: 10.1001/jamaneurol.2019.1997
69. Hedstrom AK, Olsson T, Alfredsson L. High body mass index before age 20 is associated with increased risk for multiple sclerosis in both men and women. Mult Scler. (2012) 18:1334–6. doi: 10.1177/1352458512436596
70. Mokry LE, Ross S, Timpson NJ, Sawcer S, Davey Smith G, Richards JB. Obesity and multiple sclerosis: a mendelian randomization study. PLoS Med. (2016) 13:e1002053. doi: 10.1371/journal.pmed.1002053
71. Wesnes K, Myhr K-M, Riise T, Cortese M, Pugliatti M, Boström I, et al. Physical activity is associated with a decreased multiple sclerosis risk: the EnvIMS study. Mult Scler. (2017) 24:150–57. doi: 10.1177/1352458517694088
72. Gunnarsson M, Udumyan R, Bahmanyar S, Nilsagard Y, Montgomery S. Characteristics in childhood and adolescence associated with future multiple sclerosis risk in men: cohort study. Eur J Neurol. (2015) 22:1131–7. doi: 10.1111/ene.12718
73. Gianfrancesco MA, Acuna B, Shen L, Briggs FB, Quach H, Bellesis KH, et al. Obesity during childhood and adolescence increases susceptibility to multiple sclerosis after accounting for established genetic and environmental risk factors. Obes Res Clin Pract. (2014) 8:e435–47. doi: 10.1016/j.orcp.2014.01.002
74. Palmer BF, Clegg DJ. The sexual dimorphism of obesity. Mol Cell Endocrinol. (2015) 402:113–9. doi: 10.1016/j.mce.2014.11.029
75. Varghese M, Griffin C, McKernan K, Eter L, Lanzetta N, Agarwal D, et al. Sex differences in inflammatory responses to adipose tissue lipolysis in diet-induced obesity. Endocrinology. (2019) 160:293–312. doi: 10.1210/en.2018-00797
76. Monteiro R, Teixeira D, Calhau C. Estrogen signaling in metabolic inflammation. Mediators Inflamm. (2014) 2014:615917. doi: 10.1155/2014/615917
77. Bianchi VE. The anti-inflammatory effects of testosterone. J Endocr Soc. (2019) 3:91–107. doi: 10.1210/js.2018-00186
78. Kasapis C, Thompson PD. The effects of physical activity on serum C-reactive protein and inflammatory markers: a systematic review. J Am Coll Cardiol. (2005) 45:1563–9. doi: 10.1016/j.jacc.2004.12.077
79. Nieman DC, Wentz LM. The compelling link between physical activity and the body's defense system. J Sport Health Sci. (2019) 8:201–17. doi: 10.1016/j.jshs.2018.09.009
80. Brown AS, Davis JM, Murphy EA, Carmichael MD, Carson JA, Ghaffar A, et al. Gender differences in macrophage antiviral function following exercise stress. Med Sci Sports Exerc. (2006) 38:859–63. doi: 10.1249/01.mss.0000218125.21509.cc
81. Jafari N, Hintzen RQ. The association between cigarette smoking and multiple sclerosis. J Neurol Sci. (2011) 311:78–85. doi: 10.1016/j.jns.2011.09.008
82. Hedstrom AK, Baarnhielm M, Olsson T, Alfredsson L. Tobacco smoking, but not Swedish snuff use, increases the risk of multiple sclerosis. Neurology. (2009) 73:696–701. doi: 10.1212/WNL.0b013e3181b59c40
83. Healy BC, Ali EN, Guttmann CR, Chitnis T, Glanz BI, Buckle G, et al. Smoking and disease progression in multiple sclerosis. Arch Neurol. (2009) 66:858–64. doi: 10.1001/archneurol.2009.122
84. Riise T, Nortvedt MW, Ascherio A. Smoking is a risk factor for multiple sclerosis. Neurology. (2003) 61:1122–24. doi: 10.1212/01.WNL.0000081305.66687.D2
85. Sundstrom P, Nystrom L, Hallmans G. Smoke exposure increases the risk for multiple sclerosis. Eur J Neurol. (2008) 15:579–83. doi: 10.1111/j.1468-1331.2008.02122.x
86. Ashare RL, Wetherill RR. The intersection of sex differences, tobacco use, and inflammation: implications for psychiatric disorders. Curr Psychiatry Rep. (2018) 20:75. doi: 10.1007/s11920-018-0946-3
87. Faner R, Gonzalez N, Cruz T, Kalko SG, Agusti A. Systemic inflammatory response to smoking in chronic obstructive pulmonary disease: evidence of a gender effect. PLoS ONE. (2014) 9:e97491. doi: 10.1371/journal.pone.0097491
88. Moscovis SM, Cox A, Hall ST, Burns CJ, Scott RJ, Blackwell CC. Effects of gender, cytokine gene polymorphisms and environmental factors on inflammatory responses. Innate Immun. (2015) 21:523–30. doi: 10.1177/1753425914553645
89. Tweed JO, Hsia SH, Lutfy K, Friedman TC. The endocrine effects of nicotine and cigarette smoke. Trends Endocrinol Metab. (2012) 23:334–42. doi: 10.1016/j.tem.2012.03.006
90. Palacios N, Alonso A, Bronnum-Hansen H, Ascherio A. Smoking and increased risk of multiple sclerosis: parallel trends in the sex ratio reinforce the evidence. Ann Epidemiol. (2011) 21:536–42. doi: 10.1016/j.annepidem.2011.03.001
91. Lucas RM, Ponsonby AL, Dear K, Valery PC, Pender MP, Taylor BV, et al. Sun exposure and vitamin D are independent risk factors for CNS demyelination. Neurology. (2011) 76:540–8. doi: 10.1212/WNL.0b013e31820af93d
92. Hart PH, Gorman S, Finlay-Jones JJ. Modulation of the immune system by UV radiation: more than just the effects of vitamin D? Nat Rev Immunol. (2011) 11:584–96. doi: 10.1038/nri3045
93. Hart PH, Norval M, Byrne SN, Rhodes LE. Exposure to ultraviolet radiation in the modulation of human diseases. Annu Rev Pathol. (2019) 14:55–81. doi: 10.1146/annurev-pathmechdis-012418-012809
94. Dankers W, Colin EM, van Hamburg JP, Lubberts E. Vitamin D in autoimmunity: molecular mechanisms and therapeutic potential. Front Immunol. (2017) 7:697. doi: 10.3389/fimmu.2016.00697
95. Simpson S Jr, Wang W, Otahal P, Blizzard L, van der Mei IAF, Taylor BV. Latitude continues to be significantly associated with the prevalence of multiple sclerosis: an updated meta-analysis. J Neurol Neurosurg Psychiatry. (2019) 90:1193–200. doi: 10.1136/jnnp-2018-320189
96. Munger KL, Levin LI, Hollis BW, Howard NS, Ascherio A. Serum 25-hydroxyvitamin D levels and risk of multiple sclerosis. JAMA. (2006) 296:2832–8. doi: 10.1001/jama.296.23.2832
97. Langer-Gould A, Lucas R, Xiang AH, Chen LH, Wu J, Gonzalez E, et al. MS sunshine study: sun exposure but not vitamin d is associated with multiple sclerosis risk in blacks and hispanics. Nutrients. (2018) 10:268. doi: 10.3390/nu10030268
98. Krementsov DN, Asarian L, Fang Q, McGill MM, Teuscher C. Sex-specific gene-by-vitamin D interactions regulate susceptibility to central nervous system autoimmunity. Front Immunol. (2018) 9:1622. doi: 10.3389/fimmu.2018.01622
99. Baarnhielm M, Olsson T, Alfredsson L. Fatty fish intake is associated with decreased occurrence of multiple sclerosis. Mult Scler. (2014) 20:726–32. doi: 10.1177/1352458513509508
100. Feige J, Moser T, Bieler L, Schwenker K, Hauer L, Sellner J. Vitamin D supplementation in multiple sclerosis: a critical analysis of potentials and threats. Nutrients. (2020) 12:783. doi: 10.3390/nu12030783
101. Nashold FE, Spach KM, Spanier JA, Hayes CE. Estrogen controls vitamin D3-mediated resistance to experimental autoimmune encephalomyelitis by controlling vitamin D3 metabolism and receptor expression. J Immunol. (2009) 183:3672–81. doi: 10.4049/jimmunol.0901351
102. Correale J, Ysrraelit MC, Gaitán MI. Gender differences in 1,25 dihydroxyvitamin D3 immunomodulatory effects in multiple sclerosis patients and healthy subjects. J Immunol. (2010) 185:4948–58. doi: 10.4049/jimmunol.1000588
103. Kragt J, van Amerongen B, Killestein J, Dijkstra C, Uitdehaag B, Polman C, et al. Higher levels of 25-hydroxyvitamin D are associated with a lower incidence of multiple sclerosis only in women. Mult Scler. (2009) 15:9–15. doi: 10.1177/1352458508095920
104. Hupperts R, Smolders J, Vieth R, Holmoy T, Marhardt K, Schluep M, et al. Randomized trial of daily high-dose vitamin D3 in patients with RRMS receiving subcutaneous interferon beta-1a. Neurology. (2019) 93:e1906–16. doi: 10.1212/WNL.0000000000008445
105. Hart PH, Jones AP, Trend S, Cha L, Fabis-Pedrini MJ, Cooper MN, et al. A randomised, controlled clinical trial of narrowband UVB phototherapy for clinically isolated syndrome: the PhoCIS study. Mult Scler J Exp Transl Clin. (2018) 4:2055217318773112. doi: 10.1177/2055217318773112
106. Trend S, Leffler J, Cooper MN, Byrne SN, Kermode AG, French MA, et al. Narrowband UVB phototherapy reduces TNF production by B-cell subsets stimulated via TLR7 from individuals with early multiple sclerosis. Clin Transl Immunology. (2020) 9:e1197. doi: 10.1002/cti2.1197
107. Ostkamp P, Salmen A, Pignolet B, Gorlich D, Andlauer TFM, Schulte-Mecklenbeck A, et al. German competence network multiple, the BN. Sunlight exposure exerts immunomodulatory effects to reduce multiple sclerosis severity. Proc Natl Acad Sci U S A. (2021) 118:e2018457118. doi: 10.1073/pnas.2110306118
108. Irving AA, Marling SJ, Seeman J, Plum LA, DeLuca HF. UV light suppression of EAE (a mouse model of multiple sclerosis) is independent of vitamin D and its receptor. Proc Natl Acad Sci U S A. (2019) 116:22552–5. doi: 10.1073/pnas.1913294116
109. Orton SM, Wald L, Confavreux C, Vukusic S, Krohn JP, Ramagopalan SV, et al. Association of UV radiation with multiple sclerosis prevalence and sex ratio in France. Neurology. (2011) 76:425–31. doi: 10.1212/WNL.0b013e31820a0a9f
110. Kampman MT, Aarseth JH, Grytten N, Benjaminsen E, Celius EG, Dahl OP, et al. Sex ratio of multiple sclerosis in persons born from 1930 to 1979 and its relation to latitude in Norway. J Neurol. (2013) 260:1481–8. doi: 10.1007/s00415-012-6814-x
111. Myerson A, Neustads R. Influence of ultraviolet irradiation upon secretion of sex hormones in the male. Endocrinology. (1939) 25:7–12. doi: 10.1210/endo-25-1-7
112. Parikh R, Sorek E, Parikh S, Michael K, Bikovski L, Tshori S, et al. Skin exposure to UVB light induces a skin-brain-gonad axis and sexual behavior. Cell Rep. (2021) 36:109579. doi: 10.1016/j.celrep.2021.109579
113. Norval M, Halliday GM. The consequences of UV-induced immunosuppression for human health. Photochem Photobiol. (2011) 87:965–77. doi: 10.1111/j.1751-1097.2011.00969.x
114. Bernard JJ, Cowing-Zitron C, Nakatsuji T, Muehleisen B, Muto J, Borkowski AW, et al. Ultraviolet radiation damages self noncoding RNA and is detected by TLR3. Nat Med. (2012) 18:1286–90. doi: 10.1038/nm.2861
115. Trend S, Jones AP, Cha L, Cooper MN, Geldenhuys S, Fabis-Pedrini MJ, et al. Short-term changes in frequencies of circulating leukocytes associated with narrowband UVB phototherapy in people with clinically isolated syndrome. Sci Rep. (2019) 9:7980. doi: 10.1038/s41598-019-44488-6
116. Ponda MP, Liang Y, Kim J, Hutt R, Dowd K, Gilleaudeau P, et al. A randomized clinical trial in vitamin D-deficient adults comparing replenishment with oral vitamin D3 with narrow-band UV type B light: effects on cholesterol and the transcriptional profiles of skin and blood. Am J Clin Nutr. (2017) 105:1230–8. doi: 10.3945/ajcn.116.150367
117. Somers EC, Marder W, Cagnoli P, Lewis EE, DeGuire P, Gordon C, et al. Population-based incidence and prevalence of systemic lupus erythematosus: the Michigan Lupus Epidemiology and Surveillance program. Arthritis Rheumatol. (2014) 66:369–78. doi: 10.1002/art.38238
118. Lateef A, Petri M. Managing lupus patients during pregnancy. Best Pract Res Clin Rheumatol. (2013) 27:435–47. doi: 10.1016/j.berh.2013.07.005
119. Buyon JP, Petri MA, Kim MY, Kalunian KC, Grossman J, Hahn BH, et al. The effect of combined estrogen and progesterone hormone replacement therapy on disease activity in systemic lupus erythematosus: a randomized trial. Ann Intern Med. (2005) 142:953–62. doi: 10.7326/0003-4819-142-12_Part_1-200506210-00004
120. Petri M, Kim MY, Kalunian KC, Grossman J, Hahn BH, Sammaritano LR, et al. Combined oral contraceptives in women with systemic lupus erythematosus. N Engl J Med. (2005) 353:2550–8. doi: 10.1056/NEJMoa051135
121. Lossius A, Johansen JN, Torkildsen O, Vartdal F, Holmoy T. Epstein-Barr virus in systemic lupus erythematosus, rheumatoid arthritis and multiple sclerosis-association and causation. Viruses. (2012) 4:3701–30. doi: 10.3390/v4123701
122. Barbhaiya M, Costenbader KH. Environmental exposures and the development of systemic lupus erythematosus. Curr Opin Rheumatol. (2016) 28:497–505. doi: 10.1097/BOR.0000000000000318
123. Choi MY, Hahn J, Malspeis S, Stevens EF, Karlson EW, Sparks JA, et al. A combination of healthy lifestyle behaviors reduces risk of incident systemic lupus erythematosus. Arthritis Rheumatol. (2021). doi: 10.1002/art.41935
124. Tedeschi SK, Barbhaiya M, Malspeis S, Lu B, Sparks JA, Karlson EW, et al. Obesity and the risk of systemic lupus erythematosus among women in the nurses' health studies. Semin Arthritis Rheum. (2017) 47:376–83. doi: 10.1016/j.semarthrit.2017.05.011
125. Khan D, Ansar Ahmed S. The immune system is a natural target for estrogen action: opposing effects of estrogen in two prototypical autoimmune diseases. Front Immunol. (2015) 6:635. doi: 10.3389/fimmu.2015.00635
126. Foering K, Chang AY, Piette EW, Cucchiara A, Okawa J, Werth VP. Characterization of clinical photosensitivity in cutaneous lupus erythematosus. J Am Acad Dermatol. (2013) 69:205–13. doi: 10.1016/j.jaad.2013.03.015
127. Skopelja-Gardner S, An J, Tai J, Tanaka L, Sun X, Hermanson P, et al. The early local and systemic Type I interferon responses to ultraviolet B light exposure are cGAS dependent. Sci Rep. (2020) 10:7908. doi: 10.1038/s41598-020-64865-w
128. Avila M, Bansal A, Culberson J, Peiris AN. The role of sex hormones in multiple sclerosis. Eur Neurol. (2018) 80:93–9. doi: 10.1159/000494262
129. Gubbels Bupp MR, Jorgensen TN. Androgen-induced immunosuppression. Front Immunol. (2018) 9:794. doi: 10.3389/fimmu.2018.00794
Keywords: multiple sclerosis, sex hormones, environmental risk factors, immune regulation, Epstein-Barr virus (EBV), UV radiation, vitamin D
Citation: Leffler J, Trend S, Gorman S and Hart PH (2022) Sex-Specific Environmental Impacts on Initiation and Progression of Multiple Sclerosis. Front. Neurol. 13:835162. doi: 10.3389/fneur.2022.835162
Received: 14 December 2021; Accepted: 12 January 2022;
Published: 03 February 2022.
Edited by:
Andrew J. Steelman, University of Illinois at Urbana-Champaign, United StatesReviewed by:
Dimitry N. Krementsov, University of Vermont, United StatesCopyright © 2022 Leffler, Trend, Gorman and Hart. This is an open-access article distributed under the terms of the Creative Commons Attribution License (CC BY). The use, distribution or reproduction in other forums is permitted, provided the original author(s) and the copyright owner(s) are credited and that the original publication in this journal is cited, in accordance with accepted academic practice. No use, distribution or reproduction is permitted which does not comply with these terms.
*Correspondence: Jonatan Leffler, Jonatan.leffler@telethonkids.org.au