- 1Department of Medicine, Faculty of Medicine, Chulalongkorn Centre of Excellence for Parkinson's Disease & Related Disorders, Chulalongkorn University and King Chulalongkorn Memorial Hospital, Thai Red Cross Society, Bangkok, Thailand
- 2The Academy of Science, The Royal Society of Thailand, Bangkok, Thailand
The capacity for voluntary control is seen as essential to human movements; the sense that one intended to move (willing) and those actions were self-generated (self-agency) gives the sense of voluntariness and of being in control. While the mechanisms underlying voluntary movement have long been unclear, recent neuroscientific tools have identified networks of different brain areas, namely, the prefrontal cortex, supplementary motor area, and parietal cortex, that underlie voluntary action. Dysfunction in these brain areas can result in different forms of semivoluntary movement as the borderland of voluntary and involuntary movement where a person may experience a disordered sense of will or agency, and thus the movement is experienced as unexpected and involuntary, for an otherwise voluntary-appearing movement. Tics, functional movement disorders, stereotypies, perseveration, compulsions, utilization behaviors, and motor mannerism have been described elsewhere in the context of psychoses, and are often mistaken for each other. Yet, they reflect an impairment of prefrontal cortices and related circuits rather than simple motor systems, which results in the absence of subjective recognition of the movements, in contrast to other neurological movement disorders where principal abnormalities are located within the basal ganglia and its connections. Therefore, their recognition is clinically important since they are usually associated with neurodevelopmental and neurodegenerative disorders. In this review, we first defined a conceptual framework, from both a neuroanatomical and a neurophysiological point of view, for the generation of voluntary movement. We then examined the evidence linking dysfunctions in different motor pathways to each type of movement disorder. We looked at common semivoluntary movement disorders providing an overview, where possible, of their phenomenology and brain network abnormalities for each condition. We also emphasized important clinical feature similarities and differences to increase recognition of each condition in practice.
Introduction
Human movements can be broadly divided into two types: those associated with intentional action (intentional or voluntary movement) and those without intention, which includes normal non-intentional movements, reflex (response to external signal) and involuntary movement (1). Voluntary movements are self-generated, willed actions performed as a result of cognitive processes (2). While non-intentional movements, on the other hand, refer to body movements outside of one's intention (3). It includes several kinds of movements. Non-intentional normal movements in which the movements are not done by one's intention, but often naturally occur without causing problems in daily life (e.g., associate movements, mirror movements, yawning, etc.) (4). Reflexes are another normal, non-intentional activity in response to stimuli (5). Several reflexes that affect movement can be classified as proprioceptive reflexes, which originate from receptors within muscles, tendons, and joints (e.g., stretch reflex and tendon reflex); and exteroceptive reflexes, which originate from afferent input from the skin and subcutaneous tissue (e.g., extensor-thrust reflex, flexor reflex, and crossed extensor reflex) (6). The last one is pathologic involuntary movements, in contrast, abnormal movements which are considered to be treated as a symptom of disorders (e.g., tremor, dystonia, chorea, etc.) (3). This subcategory also includes involuntary movements that appear during voluntary activity (e.g., mirror movements and synkinesias) (7). It can be noted that mirror movements have been considered either physiological, presenting in healthy children and can be elicited in adults under conditions of intense physical activity, movements involving large force generation, and proximal muscle use (8, 9); or pathological when mirror movements persist in the adult with age-related neurological diseases (e.g., stroke, amyotrophic lateral sclerosis, Parkinson's disease, etc.) (10–12).
Though the voluntary movement is a fundamental component of human functioning, what exactly happens in the brain when we decide to move remains unclear. This review will first highlight key mechanisms in the generation of voluntary movement, with insights into the nature of volition (How to define voluntary action), then explore neurological conditions in the borderland between voluntary and involuntary movements, i.e., semivoluntary movement (Figure 1). Finally, we look at some behaviors that, by clinical features, can mimic repetitive semivoluntary movement.
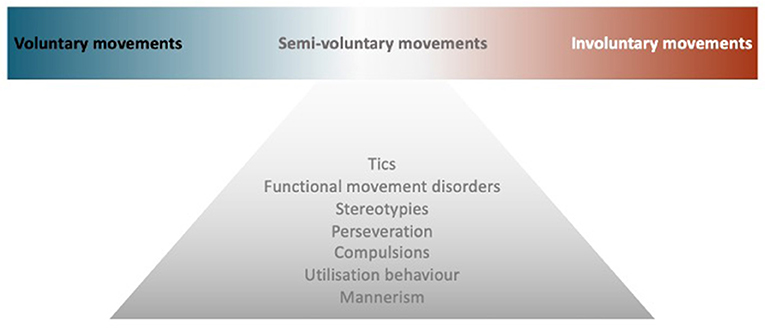
Figure 1. Model of semivoluntary movement. A diagram illustrates the concept of semivoluntary movement which is the neurological conditions in the borderland between voluntary and involuntary movements.
How Do We Move?
Neuroanatomical conceptions of motor pathways, initially described by (13), centered on “final common pathways” through the primary motor cortex to the lower motor neurons of the spinal cord which have long axons traveling along peripheral nerves to innervate skeletal muscles (13, 14). All the movements reflect the interaction of these supraspinal commands along with sensory inputs and spinal cord interneurons. Moreover, these final common pathways are greatly regulated or modulated by two feedback loops; the basal ganglia loop and the cerebellar loop, which play an important role in a variety of movements. Voluntary movement is, by definition, the intended execution of an action that is the result of cognitive processes (1). In other words, voluntary movement is a product of the coordinated operation of various neural systems and is essential for flexibly achieving a particular goal. The prefrontal cortex (PFC) and limbic area, thus, have been demonstrated to be important structures for executive functions (15, 16) in which they initiate an intention to move and determines what kind of movement is required (goal-directed action; drive to move), then the presupplementary or supplementary motor area (SMA) makes an actual plan for the movement including order, sequence, and timings (internal preparation for movement; the decision to move) (17, 18). On the other hand, the premotor cortex primarily selects movement based on external information from primary sensory cortices, with is, in turn, transferred to the parietal cortex and premotor cortex, respectively. The parietopremotor-primary motor (M1) circuit is involved when motor actions depend on external cues, i.e., when actions are driven by sensory stimuli (19). Pre-SMA-M1 and parietopremotor-M1 circuits are normally balanced allowing healthy humans to effortlessly perform daily movements, but without unintentionally touching or reacting to every object from their surroundings (7). This information is then sent down via the motor cortex to two side loops—basal ganglia and cerebellum—for checking and modulating motor control. Then, it needs to be conveyed to the motor cortex again passing through the thalamus. Finally, M1 sends the final messages for the actual movements (motor execution) to some subcortical structures, such as the reticular nucleus, vestibular nucleus, red nucleus, spinal motoneurons, and so on (20) (Figure 2).
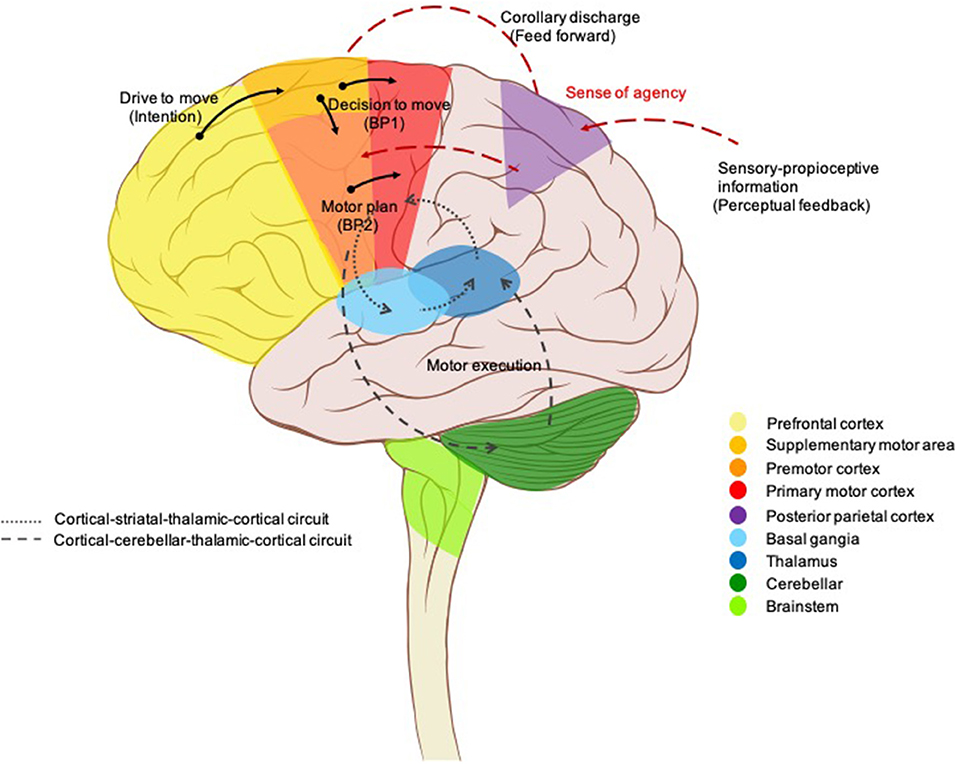
Figure 2. Brain circuits control voluntary movement. Voluntary movements are executed consciously under the control of the brain, starting with the intention to move created in the prefrontal cortex and limbic area. Next, presupplementary and supplementary motor areas are involved in programming the complex sequences of movements required. The premotor cortex primarily selects movements based on external information from the parietal cortex, it also contributes to some aspects of voluntary movement. The presupplementary and supplementary motor areas; together with the premotor area generate the readiness potentials (Bereitschaftspotential 1). This information is sent down via the motor cortex to two side loops—basal ganglia and cerebellum—for checking and modulating motor control. Then, it needs to be conveyed to the motor cortex again passing through the thalamus. A corollary discharge is also created in parallel (feedforward model) and then sent to the parietal cortex for comparison with the proprioceptive feedback, resulting in a sense of agency. Finally, the neural signal leaves the primary motor cortex (Bereitschaftspotential 2) for the spinal cord and contralateral muscles to trigger the actual movement.
Neurophysiological studies, to date, have generally focused on a theoretical framework of voluntary movement as a multilevel process that incorporates internal prediction (feedforward) with external cues (feedback) (21). Figure 2 illustrates this proposed model. A cortical process starts with a drive to move, as a result of limbic, homeostasis, and goal-directed processes. The goals or outcomes of the voluntary action are more varied and more complex in humans than in animals. In animal studies, goal-directed actions typically involve the search for biologically important goals (e.g., food, water, etc.). While voluntary actions in humans may be directed at more abstract goals, or higher needs (22). The SMA is involved in the process of planning for purposeful actions (e.g., throwing a ball, rising from a chair) (18, 23). The network of cortical areas responsible for the motor preparation process was further investigated by Cunnington et al. (24) who demonstrated that the SMA, pre-SMA, anterior cingulate cortex (ACC), and premotor cortex actively generate the early component of Bereitschaftspotential (BP) or readiness potential BP1 which linked to motivational, intentional, and timing properties (25–27). In addition, the late component (BP2) of the BP, arising from the M1, was found to be linked to motor execution and performance (27–29). Whenever a voluntary movement is made, an efferent signal is produced and sent to the motor system, a copy of the predicted sensory signal, known as an efference copy (corollary discharge), is also created in parallel, by providing the input to a feedforward model, and then sent to the brain regions that receive perceptual input for comparison with the proprioceptive feedback after completion of the actions (21, 30). This process has been localized to the temporoparietal junction (TPJ), PFC, and the cerebellum (31–33). The purpose is not only to rectify movement accuracy but also is the basis of our sense of agency (SoA), the feeling of control over one's own actions and their consequences in the external world (34) which is a fundamental aspect of voluntary movements, as it allows us to distinguish between those sensory consequences that we cause, and those are external generate (35). SoA is generated when the predicted effect of the action (feedforward) matches the actual effect (feedback) (36, 37). In contrast, if a mismatch is detected, the movement is generated without an associated SoA, and thus can be perceived as externally generated (38, 39), a phenomenon known as loss of sensory attenuation (40, 41), which may explain why patients report that they do not experience the self-pace movement as voluntary (42).
Disorders of the Motor System
Based on the above mechanisms for voluntary movement, any damage of the final common pathways usually causes paralysis or paresis (20). Dysfunction can be in the upper motor neurons, for example, primary motor cortex, subcortical structures, brainstem, and corticospinal tracts; or lower motor neurons, which are alpha motor neurons of the spinal cord, peripheral nerves, muscles, or neuromuscular junctions (43). Movement disorders caused by defects in the basal ganglia loop are called extrapyramidal disorders with specific localizations within the basal ganglia, classically associated with certain movement disorders: substantia nigra with bradykinesia and rest tremor; subthalamic nucleus with ballism; caudate nucleus with chorea; and putamen with dystonia. Pathology of the cerebellum loop, or its pathways, is typically characterized by impairment of coordination (asynergy and ataxia), misjudgment of distance (dysmetria), and intention tremor (3, 44).
However, there are some exceptions to this general rule, for example, myoclonus does not appear to be mainly related to basal ganglia pathology, and often arise elsewhere in the central nervous system, namely, the cerebral cortex (cortical reflex myoclonus), brainstem (reticular reflex myoclonus, hyperekplexia, and rhythmical brainstem myoclonus such as palatal myoclonus and ocular myoclonus), and spinal cord (segmental myoclonus and propriospinal myoclonus) (3). Finally, any damage at the final common pathways input (higher motor disorders), namely, the PFC and related regions, namely, premotor cortex, SMA, pre-SMA, ACC, and parietal areas, appear to involve self-initiation and conscious awareness of movement (7). When individuals perform the movement without experiencing the feeling of ownership, then there is no sense of voluntariness concerning the movement, thus they feel quite similar to physically involuntary movements (45, 46) (Figure 3).
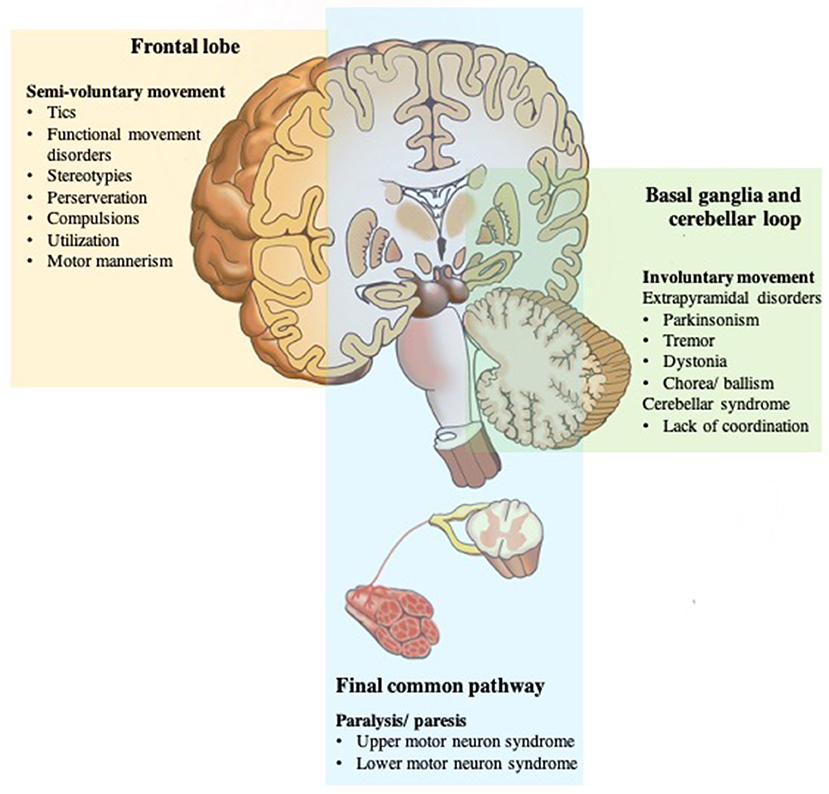
Figure 3. Disorders of the motor system. The motor system is deconstructed into component parts, but they work together to produce movement that we take for granted until there is a problem. Any damage of the final common pathways (shown in blue) can cause upper or lower motor neuron syndromes resulting in an inability to move due to paresis or paralysis. Dysfunction of the two parallel loops (shown in green) disturbs smooth movements resulting in pathologic involuntary movement disorders; basal ganglia loop dysfunction with extrapyramidal disorders; cerebellar loop dysfunction with a lack of coordination. While any damage of the frontal lobe or final common pathways input (shown in yellow) appears to involve self-initiation and conscious awareness of movement, and thus, results in no sense of voluntariness for an otherwise voluntary-appearing movement.
Capacity for voluntary action is gradually developed across childhood to reach a plateau where a well-functioning motor system permits well-controlled fluid movement. However, certain repetitive semivoluntary movements can be part of normal motor development suggesting an etiological basis of incomplete cortical control of endogenous patterning in maturing neuromuscular pathways (47). These movements appear to go through a normal progression in normal developing children, eventually disappearing and being replaced by more complex voluntary movements concerning daily activities and ordering of objects as a result of full frontal cortex development and maturation (48). On the flip side, increasing age accompanied by a progressive decline in the frontal cortex function, and certain neurological and developmental disorders, can cause these pathological semivoluntary movements to re-emerge (Figure 4). In the following section, we will cover common semivoluntary movement disorders with an overview, where possible, of the phenomenology and anatomy and neuropathophysiology of each condition. We also emphasize important clinical features similarities and differences to increase recognition of each condition in practice.
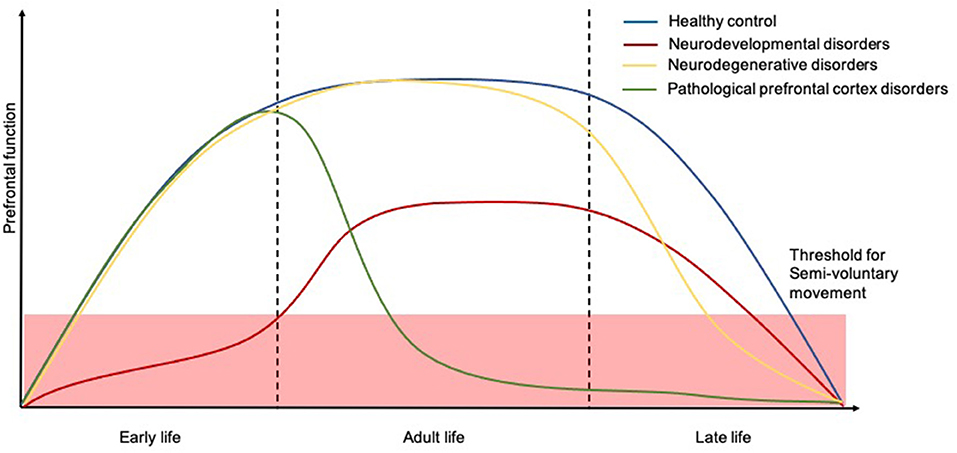
Figure 4. Temporal trajectories of the relationship between human prefrontal function and course of semivoluntary movement. The x-axis shows time and the y-axis shows the relative human prefrontal function. The schematic illustrates how prefrontal function in people with neurodevelopmental disorders (red), neurodegenerative disorders (yellow), and other pathological prefrontal cortex disorders (green) might decline, for which the semivoluntary movement (under the threshold) will begin to emerge compared to healthy population (blue).
Disorders of Semivoluntary Movements
Tics and Tourette Syndrome
Tics are hyperkinetic movement disorders characterized by sudden, brief, intermittent, and repetitive movements or vocalizations which appear seemingly uncontrollable, out of context, and exaggerated of normal movement (49, 50). Tics have intra- and interpersonal phenomenological variability which can be simple or complex movements, or even stereotyped, but look like movements that can be made voluntarily or can be mimicked easily by the patients. Simple tics are defined as movements involving a single muscle or muscle group and appear as a purposeless jerk (e.g., eye blinking, nose wrinkling, head jerking, and shoulder shrugging) or sound (e.g., grunting, throat clearing). Complex tics, on the other hand, are more coordinated and often resemble goal-directed movements (or sounds), but lack an obvious purpose and appear repetitively with an inappropriate intensity and frequency (51). Further typical features in complex tics were pali-, echo-, and coprophenomena. Paliphenomena indicates the repetition of words or phrases (palilalia) or motor acts (palipraxia) (52). Echophenomena indicates the imitation of movements (echopraxia) and vocalizations (echolalia) (53), while coprophenomena represent obscene, offensive, or other socially inappropriate behaviors (verbal—coprolalia and gestures—copropraxia) (54). When multiple motor and vocal tics are present in a patient, beginning before the age of 18 years and present for more than 12 months in the absence of secondary causes, the criteria are met for a diagnosis of Tourette syndrome (TS) (55). The majority of patients with TS present with comorbidities of attention deficit hyperactivity disorder (ADHD), obsessive-compulsive disorder (OCD), anxiety disorders, and depression (56).
Tics resemble voluntary actions in that they share many of their neurophysiological characteristics. First, conscious awareness of tics is facilitated in most cases by a premonitory urge, a sensory phenomenon (urge, itch, tingling, tension, feeling, or other sensation) that appears before the occurrence of the tic. The sensation often intensifies until the movement is performed, and is relieved following the completion of the movement (50, 57). Second, tics can be completely or partially inhibited on demand. Moreover, patients may report varying levels of voluntariness, they are often unsure whether the movements just happening or done intentionally. Tics are thus located in the borderland between voluntary and involuntary actions.
Neuroscientific tools to explore brain structure, excitability, and connectivity have come a long way over the last decade which has allowed new insights into brain function in patients with tics. Neuroimaging, including structural MRI, metabolic PET, and functional MRI (fMRI), demonstrated structural and functional abnormalities at different levels over and above the hyperactive corticostriatothalamocortical (CSTC) loops, a series of circuits that are involved in the generation of voluntary action, which were previously believed to be involved in the generation of tics. Recent studies have readjusted the pathophysiology from the subcortical, striatothalamic, to the primary sensorimotor cortical level including premotor, primary motor, and sensory areas (58–61). It is suggested that more recent generalized neuroanatomical dysfunction may account for heterogeneity across clinical manifestations, psychopathology, and associated behaviors (Figure 2) (56, 58).
Electrophysiological studies have been performed in patients with tics to determine brain activity. In the late 20th century, it was reported by Obeso et al. that there was no premovement electroencephalogram (EEG) potential or motor-related cortical potential (MRCP) before simple tics, while they were present in voluntary imitated tics (62). These findings suggested that tics differ from voluntary movements due to a lack of cortical preparatory activity. More recent work, on the other hand, demonstrated MRCPs before tics and the imitations, but early BP components (BP1) were not seen (63). Interestingly, the premotor potential in these patients, presented as brief negativity starting 100–200 ms before the onset of movement, resembled the late BP, or BP2, potential and is similar to MRCPs shown in voluntary movements made in response to a triggering stimulus (64). Therefore, it is proposed that tics might be an internally driven movement, performed as a result of internal stimuli, and thus by-passing area 6, the brain area involved in the generation of early BP (65).
Functional Movement Disorders
Functional movement disorders are under the umbrella term of functional neurologic symptom disorder (FNDs), which is defined by the Diagnostic and Statistical Manual of Mental Disorders, fifth edition (DSM-5) as neurological symptoms that are unexplained by other traditional neurological or medical conditions (55). FND is a preferential term to a wide variety of terms that have previously been used, namely, psychogenic, hysterical, conversion, and somatization, as it reflects a deeper understanding of the pathophysiologic aspect, shifting away from focusing highly on psychological precipitants as seen in Freudian conversion theories (66). Patients with FNDs can manifest a variety of movement types that often mimic neurological movement disorders such as tremor, myoclonus, dystonia, tics, and parkinsonism, but exhibit physiological characteristics that imply voluntary control, including variable or inconsistency (fluctuate in pattern, degree, and distribution); incongruous (difficult to explain by a known type of involuntary movement); distraction (decreases or disappears when the attention of the patient is drawn away from the movement); entrainment (movement characteristics cannot be maintained during contralateral competing movements); suggestibility (movement might start or stop or move to another body part as the suggestion of the examiner); or the presence of a cortical potential characteristic of self-paced voluntary action. Yet a hallmark of FNDs is that they are experienced, and subjectively reported by the patient as, completely involuntary (67, 68). While anxiety, depression, and personality disorders might be frequent co-occurrences, these features are not identifiable in every patient (69, 70). Moreover, these disorders are also seen in other neurological patients, so there is not a mandatory correlation and thus, psychological stressors and psychiatric comorbidity were removed from the diagnostic criteria in the DSM-5 (55, 71).
Advances in imaging techniques have provided new insights into differences in brain activity, functional connectivity, and brain structures in FNDs that are not detected on a structural MRI. For example, a voxel-based morphometry analysis showed abnormalities in the limbic area structure (72). fMRI and PET demonstrate abnormalities in regional brain activation and functional connectivity that mediate emotional regulation and awareness (ACC, vmPFC, insula, amygdala, and vermis) (73), executive and cognitive control including motor inhibition (ACC, dlPFC, and inferior frontal gyrus) (74, 75), motor planning (SMA), and perceptual awareness (PCC/TPJ) (31, 32).
Edwards et al. have proposed three key concepts in the neurobiology of FNDs which are attention, beliefs, and agency (76). First, FNDs look like a movement that has been consciously produced by the patient because attention is required to manifest the movements (77), and movements worsen when attention is drawn toward them, typically during physical examination (78). Electrophysiology in patients with FNDs has found evidence for a BP similar to that can be seen with voluntary movements, though, the presence of BP does not always indicate that a movement is voluntary (65). Indeed, prefrontal activation which represents movement-related attention has also been demonstrated in FNDs (79). Another concept is that of symptom-related beliefs and expectations which play an important role in altering sensory experiences. Patients with FNDs usually overestimated their symptoms according to Parees et al. who found that patients with functional tremor significantly overrated tremor duration in self-reported diaries compared with actigraphy (80). They have interpreted this finding within a Bayesian framework as an abnormally strong prior expectation, proposed to reside within SMA, over relevant bottom-up sensory data, and thus without appropriated and mismatch top-down control, this might result in the inappropriate selection of action in FNDs (65). The third key concept relates to SoA, which arises from the appropriate match of volition and movement feedback, likely centered on the parietal area. Mismatch in this process is misinterpreted by patients as without agency, and thus not self-generated which is a hallmark of FNDs.
Stereotypies
Stereotypies lack a clearly defined terminology, yet continue to be debatable. One current workable definition by Edwards et al. defines them as a non-goal-directed movement pattern or vocalization that is repeated continuously for some time in the same form and on multiple occasions, which is typically distractible (81). Further confusion is generated by the attempt to classify stereotypies as voluntary or involuntary. One commonly quoted definition by Jankovic names them as being involuntary movements (3), however, this raises further questions, as stereotypy can be suppressed by distraction meaning that stereotypies require attention to manifest. Furthermore, it is probably impossible to obtain accurate reports from affected individuals as they are often very young children or persons with impaired mentation or learning difficulties.
Stereotypies can be classified according to their phenomenology as simple (e.g., leg shaking, foot tapping, hair twirling, nail biting, teeth grinding, body rocking, etc.) or more complex (e.g., hand waving, repeatedly opening and closing hands, hand posturing, head nodding, headbanging, repeatedly sitting down and getting up from a chair, finger wagging, pacing, orofacial movements, self-biting, and other self-injurious behaviors) (81, 82). Occasionally, vocal or phonic stereotypies (e.g., moaning, humming, grunting, or repeating words and phrases) can also be presented (83). From an etiologic point of view, stereotypies are divided into 2 categories, either primary or secondary, depending on the presence or absence of additional neurological or psychiatric disorders (83). Primary stereotypies appear to be purely physiological, i.e., they occur in normally developing children, however, subtle developmental issues have been noted (84, 85). It has been estimated that the prevalence of stereotypies in normal children is around 20%, with a typical age of onset by 3 years of age (86). The natural course is variable, with some declining after age 4 years, some persisting for multiple years (remained stable, improved, or became worse), and some even presenting into adulthood when stressed (86, 87). Common physiological stereotypies in adults are leg shaking, i.e., leg stereotypy disorder (88), playing with pens or hair, face touching, nail biting, hand or foot tapping, and body rocking (82). Secondary stereotypies, on the other hand, most often occur in children with autistic spectrum disorders, intellectual disability, or other neurological problems whereas stereotypies in adults are associated with various conditions, namely, drug taking, cerebrovascular diseases, namely, the frontal lobe, neurodegenerative diseases, infection, autoimmune encephalitis, and psychiatric conditions (89).
The underlying pathophysiology of stereotypies remains unknown. Psychological hypotheses had been proposed, including disorders of arousal and motor control, learned behavior, or as a component of underlying psychiatric disorders (90, 91). However, there is objective evidence supporting the involvement of underlying neurobiological abnormalities, ranging from structural brain imaging to neurophysiological studies. Reductions in frontal white matter and caudate nucleus were reported in healthy children with complex stereotypies (92). Other studies reported focal basal ganglia lesions resulting in stereotypies (93, 94). Looking into cerebral activity, unlike voluntary movements and FNDs, stereotypies that were not preceded by MRCPs suggest their physiologically distinction from that of voluntary movements (95). These results imply that stereotypies arise without normal control from premotor areas and indicate dysfunction within prefrontocorticobasal ganglia circuits (see Figure 2). Instead, this motor activity probably originates from the basal ganglia, related to the striatal dopaminergic system in particular, given that stereotypies were induced in rodents administered with dopamine D1 receptor agonists (96–98), and improved with D1 receptor antagonists (96, 99). Similarly, they have been found as a side effect of dopaminergic drugs, such as amphetamine, cocaine, and levodopa in clinical observation (97).
Perseveration
Perseveration refers to the inappropriate continuation or repetition of response or activity (e.g., behavior, word, thought, strategy, or emotion). This term was first used by Neisser in 1895 and, since then, many clinical researchers have attempted to describe perseveration in several different forms and in association with a variety of neurological disorders and many underlying hypothetical mechanisms (100). However, there continues to be a lack of agreement in the literature as to how it should be classified. In this article, the authors have reviewed descriptions and classifications according to Liepmann (101) as this definition has been wildly used when describing perseveration in the form of movement disorders. He proposed three types of perseveration: (1) intentional perseveration, which is the repetition of previous response to a subsequent stimulus; (2) tonic perseveration, which is the inability to discontinue an action, e.g., unable to release another's hand after shaking it; and (3) clonic perseveration, which will be discussed in this section, a continuous repetition of an action that can be induced by passively moving a body part or by an external cue and its persistence occurs even after cessation of the external cue, e.g., continue to draw multiple loops when asked to draw once (101). The ability to induce the movement is a key to identifying and differentiating this phenomenology from stereotypies. Clonic perseveration is known to be a sequela of brain damage and typically occurs in a setting with a reduced level of consciousness, aspontaneity, usually with mutism and frontal releasing sign (102, 103). In those who relatively preserved language and speech, verbal perseveration, characterized by inappropriate repetition of phrases and words, was noted (103).
In considering neuroanatomical correlation, perseveration is commonly viewed as a cardinal feature of prefrontal pathology (102, 104, 105), however, it has been documented in thalamic (103, 106) or subthalamic infarction (107), and the fiber tracts connecting the limbic system to the frontal cortex (103). Therefore, it is suggested that perseveration occurs as a result of disconnection of the prefrontal corticobasal gangliathalamocortical loops that are important for the termination of motor plans (108–110).
Behaviors That Mimic Semivoluntary Movements
The clinical distinction between movement and behavior is usually obvious. Movement is defined by the act of moving, whereas behavior defines how one acts and especially one's actions toward others (111). However, from a phenomenological viewpoint, there are some borderline forms in which repetitive unintentional behavior can mimic repetitive semivoluntary movement. Included are (1) compulsions, (2) utilization behavior (UB), and (3) motor mannerism.
Compulsions are characterized by repetitive and excessive behaviors that one is compelled to perform in response to intrusive and uncontrollable thoughts, which are labeled obsessions. These obsessive thoughts and compulsive behaviors are key components of OCD. More recently, DSM-5 recognizes four major types of obsessions: (i) obsessions related to fear of contamination associated with washing rituals; (ii) obsessions linked to harming focused checking rituals; (iii) obsessions of symmetry or order associated with counting rituals; and (iv) obsessive ideas linked to concerns about sex, religion, aggression, and other matters, associated with checking behaviors and/or purification rituals (55). The phenomenology of compulsions is complex and varied performing to reassure or counteract obsessions, for example, excessive washing or cleaning to remove germs, praying to counter sacrilegious thoughts, checking for assurance that doors are locked or people are OK, putting things in order (arranging), repeating other behaviors to get rid of a thought, etc. (112). In clinical practice, however, the boundary between compulsions and tics particularly complex motor tics, such as repeating actions until it feels right, is not easily determined. In addition, the frequent comorbidity between OCD and tics or Tourette Syndrome have been reported in which 20 to 60% of Tourette Syndrome sufferers display OCD symptoms and studies of patients with OCD have found tics in more than 50% of cases and Tourette Syndrome in 15% of cases (113, 114). For the clinician, certain distinctions, namely the content of obsessions, the nature of compulsions, the functional relationship between obsessions and compulsions, and the response to treatment are potentially useful discriminators for decision making (115).
According to the recent neurobiological model, OCD is characterized by the aberrant activity of the CSTC pathway in terms of overall hyperactivity of the corticostriatal loop (direct loop), in consequence, the indirect loop is no longer able to regulate or inhibit impulsive behavior and actions that are no more relevant or adequate to the situation. This results in a cortical hyperactivation leading to the typical symptoms of OCD, e.g., impulsivity and impaired action inhibition (116, 117). Concerning brain activity, previous studies that recorded event-related potential component of BP in patients with OCD found that they presented with a greater BP1 slope gradient and amplitude over bilateral frontoparietal areas corresponding to the motor cortex, which support the hypothesis that stronger motor response preparation for external stimuli might characterize OCD (118, 119).
Utilization behavior refers to a disorder in which patients automatically use or manipulate objects presented in the field of vision in an “object-appropriate” manner which is inappropriate for a given context (120). For example, when shown a toothbrush, a patient is likely to pick it up and begin to brush his teeth correctly, but in a context in which brushing teeth would not normally be done, e.g., in an appointment with a doctor. Moreover, patients usually claim that they intended to do so, as they do not detect a mismatch between actions and intentions. UB has been being recognized as an abnormal behavior following dysfunction of frontal areas or system, such that the top-down control of the frontal lobe can no longer inhibit the dependency of the parietal lobe upon sensory or environmental input (120, 121). Therefore, UB is another type of environmental dependency, as a result of an imbalance between frontal and parietal lobes.
Motor mannerisms are another unusual repetitive, distinctive behavior in an exaggerated and bizarre fashion, for example, eccentric postures, gestures, facial expressions, peculiar speech, unusual appearance in clothing, or makeup, etc. (122). They are considered goal-directed actions carried out by individuals in an attempt to call attention to themselves (122). Although mannerisms do not usually interfere with life, or cause self-injury, they can lead to withdrawal from, and rejection by, society. Mannerisms occur frequently in schizophrenia, however, they can be found in normal subjects, especially during puberty and adolescence, abnormal personalities, and neurological disorders (123).
Like semivoluntary movements mentioned earlier, mannerisms are under voluntary control but are displayed unconsciously, but the main difference is that mannerisms appear overdrawn and are considered to serve a distinct purpose, which is primarily communicative (124).
The pathophysiological mechanisms underlying manneristic behaviors are far from being understood. Some neurophysiological results suggest abnormal behavior-physiology interaction, secondary to pathological alteration of the brain metabolism, and disturbed perceptivity of social signals may be involved (125).
Conclusion
The study of voluntary control is an integral part of the study of human movement. However, there is very limited information and tests available that allow distinctions between voluntary and involuntary movements to be made with confidence. A spectrum of semivoluntary movements exist in which patients may experience a disordered sense of will or agency, and thus a movement is experienced as unexpected and involuntary, for an otherwise voluntary-appearing movement.
The, as yet unclear, pathophysiological mechanisms underlying these conditions require further investigations, however, several functionally distinct areas within the frontal cortex before the primary motor area have been identified. These motor areas are differentially involved in movements made under different conditions. Table 1 summarizes different types of semivoluntary movements and associated behavior manifestation and several critical components, namely, semiology, common presentations, and neuroanatomical and neurophysiology pathologies to better distinguish between each condition and facilitate communication among clinicians and with patients.
Semivoluntary movement is much more common than currently thought; given that most patients do not seek medical attention because of lack of resulting disability or misinterpretation as psychogenic in origin. Arguably, their recognition is clinically relevant since they are usually associated with severe conditions such as neurodevelopmental and neurodegenerative disorders.
Furthermore, limited improvement for these conditions has been reported with existing pharmacological therapies, and more effective treatments are needed. We hope that this review lays the foundation for stimulating discussion that will lead to a more comprehensive study, as a prerequisite for the discovery of improved pharmacologic interventions is understanding the underlying pathophysiology, epidemiology, including biological mechanisms.
Author Contributions
SV and RB contributed to the conception and design of the study. SV organized the database and wrote the first draft of the manuscript. RB wrote sections of the manuscript. Both authors contributed to manuscript revision, read, and approved the submitted version of the manuscript.
Funding
RB is supported by Senior Research Scholar Grant (RTA6280016) of the Thailand Science Research and Innovation (TSRI), the International Research Network Grant of the Thailand Research Fund (IRN59W0005), the Chulalongkorn Academic Advancement Fund into its Second Century Project of Chulalongkorn University, and the Centre of Excellence Grant of Chulalongkorn University (GCE 6100930004-1), Bangkok, Thailand.
Conflict of Interest
The authors declare that the research was conducted in the absence of any commercial or financial relationships that could be construed as a potential conflict of interest.
Publisher's Note
All claims expressed in this article are solely those of the authors and do not necessarily represent those of their affiliated organizations, or those of the publisher, the editors and the reviewers. Any product that may be evaluated in this article, or claim that may be made by its manufacturer, is not guaranteed or endorsed by the publisher.
References
1. Schwartz AB. Movement: how the brain communicates with the world. Cell. (2016) 164:1122–35. doi: 10.1016/j.cell.2016.02.038
4. Ugawa Y. Voluntary and involuntary movements: a proposal from a clinician. Neurosci Res. (2020) 156:80–7. doi: 10.1016/j.neures.2019.10.001
5. Fisher MA. “Reflexes, spinal cord and blink,” In: Aminoff MJ, Daroff RB, editors. Encyclopedia of the Neurological Sciences (Second Edition). Oxford: Academic Press (2014), p. 3–5.
6. JE D. Human reflexes, pathophysiology of motor systems, methodology of human reflexes. Basel. (1973) 3:687–712.
7. Kojović M, Bhatia KP. Bringing order to higher order motor disorders. J Neurol. (2019) 266:797–805. doi: 10.1007/s00415-018-8974-9
8. Bodwell JA, Mahurin RK, Waddle S, Price R, Cramer SC. Age and features of movement influence motor overflow. J Am Geriatr Soc. (2003) 51:1735–9. doi: 10.1046/j.1532-5415.2003.51557.x
9. Mayston MJ, Harrison LM, Stephens JA. A neurophysiological study of mirror movements in adults and children. Ann Neurol. (1999) 45:583–94.
10. Nelles G, Cramer SC, Schaechter JD, Kaplan JD, Finklestein SP. Quantitative assessment of mirror movements after stroke. Stroke. (1998) 29:1182–7. doi: 10.1161/01.STR.29.6.1182
11. Krampfl K, Mohammadi B, Komissarow L, Dengler R, Bufler J. Mirror movements and ipsilateral motor evoked potentials in ALS. Amyotroph Lateral Scler Other Motor Neuron Disord. (2004) 5:154–63. doi: 10.1080/14660820410019657
12. Chatterjee P, Banerjee R, Choudhury S, Mondal B, Kulsum MU, Chatterjee K, et al. Mirror movements in Parkinson's disease: an under-appreciated clinical sign. J Neurol Sci. (2016) 366:171–6. doi: 10.1016/j.jns.2016.05.026
17. Picard N, Strick PL. Activation of the supplementary motor area (SMA) during performance of visually guided movements. Cereb Cortex. (2003) 13:977–86. doi: 10.1093/cercor/13.9.977
18. Roland PE, Larsen B, Lassen NA, Skinhøj E. Supplementary motor area and other cortical areas in organization of voluntary movements in man. J Neurophysiol. (1980) 43:118–36. doi: 10.1152/jn.1980.43.1.118
19. Passingham RE. Two cortical systems for directing movement. Ciba Found Symp. (1987) 132:151–64. doi: 10.1002/9780470513545.ch10
21. Grüsser O-J. Early concepts on efference copy and reafference. Behav. Brain Sci. (1994) 17:262–5. doi: 10.1017/S0140525X00034415
23. Halsband U, Matsuzaka Y, Tanji J. Neuronal activity in the primate supplementary, pre-supplementary and premotor cortex during externally and internally instructed sequential movements. Neurosci Res. (1994) 20:149–55. doi: 10.1016/0168-0102(94)90032-9
24. Cunnington R, Windischberger C, Deecke L, Moser E. The preparation and readiness for voluntary movement: a high-field event-related fMRI study of the Bereitschafts-BOLD response. NeuroImage. (2003) 20:404–12. doi: 10.1016/S1053-8119(03)00291-X
25. Deecke L, Kornhuber HH. An electrical sign of participation of the mesial ‘supplementary' motor cortex in human voluntary finger movement. Brain Res. (1978) 159:473–6. doi: 10.1016/0006-8993(78)90561-9
26. Nguyen VT, Breakspear M, Cunnington R. Reciprocal interactions of the sma and cingulate cortex sustain premovement activity for voluntary actions. J Neurosci. (2014) 34:16397–407. doi: 10.1523/JNEUROSCI.2571-14.2014
27. Colebatch JG. Bereitschaftspotential and movement-related potentials: origin, significance, and application in disorders of human movement. Move Disord. (2007) 22:601–10. doi: 10.1002/mds.21323
28. Shibasaki H, Hallett M. What is the Bereitschaftspotential? Clin Neurophysiol. (2006) 117:2341–56. doi: 10.1016/j.clinph.2006.04.025
29. Deecke L. Bereitschaftspotential as an indicator of movement preparation in supplementary motor area and motor cortex. Ciba Found Symp. (1987) 132:231–50. doi: 10.1002/9780470513545.ch14
30. Sperry RW. Neural basis of the spontaneous optokinetic response produced by visual inversion. J Comp Physiol Psychol. (1950) 43:482–9. doi: 10.1037/h0055479
31. Sirigu A, Daprati E, Pradat-Diehl P, Franck N, Jeannerod M. Perception of self-generated movement following left parietal lesion. Brain. (1999) 122:1867–74. doi: 10.1093/brain/122.10.1867
32. Voon V, Gallea C, Hattori N, Bruno M, Ekanayake V, Hallett M. The involuntary nature of conversion disorder. Neurology. (2010) 74:223–8. doi: 10.1212/WNL.0b013e3181ca00e9
33. Welniarz Q, Worbe Y, Gallea C. The forward model: a unifying theory for the role of the cerebellum in motor control and sense of agency. Front Syst Neurosci. (2021) 15:644059. doi: 10.3389/fnsys.2021.644059
34. Haggard P. Human volition: towards a neuroscience of will. Nat Rev Neurosci. (2008) 9:934–46. doi: 10.1038/nrn2497
35. Tapal A, Oren E, Dar R, Eitam B. The sense of agency scale: a measure of consciously perceived control over one's mind, body, and the immediate environment. Front Psychol. (2017) 8:1552. doi: 10.3389/fpsyg.2017.01552
36. Linser K, Goschke T. Unconscious modulation of the conscious experience of voluntary control. Cognition. (2007) 104:459–75. doi: 10.1016/j.cognition.2006.07.009
37. Sirigu A, Daprati E, Ciancia S, Giraux P, Nighoghossian N, Posada A, et al. Altered awareness of voluntary action after damage to the parietal cortex. Nat Neurosci. (2004) 7:80–4. doi: 10.1038/nn1160
38. Blakemore SJ, Wolpert DM, Frith CD. Abnormalities in the awareness of action. Trends Cogn Sci. (2002) 6:237–42. doi: 10.1016/S1364-6613(02)01907-1
39. Frith CD, Blakemore SJ, Wolpert DM. Abnormalities in the awareness and control of action. Philos Trans R Soc Lond B Biol Sci. (2000) 355:1771–88. doi: 10.1098/rstb.2000.0734
40. Chapman CE, Bushnell MC, Miron D, Duncan GH, Lund JP. Sensory perception during movement in man. Exp Brain Res. (1987) 68:516–24. doi: 10.1007/BF00249795
41. Blakemore SJ, Wolpert DM, Frith CD. Central cancellation of self-produced tickle sensation. Nat Neurosci. (1998) 1:635–40. doi: 10.1038/2870
42. Pareés I, Brown H, Nuruki A, Adams RA, Davare M, Bhatia KP, et al. Loss of sensory attenuation in patients with functional (psychogenic) movement disorders. Brain. (2014) 137:2916–21. doi: 10.1093/brain/awu237
43. Conor Fearon BM, Hiroshi Mitsumoto. Disorders of Upper and Lower Motor Neurons Bradley and Daroff's Neurology in Clinical Practice. New York, NY: Elsevier (2021), 1535–67.
44. Jankovic J. Parkinson Disease and Other Movement Disorders Bradley and Daroff's Neurology in Clinical Practice. (2021), p. 1498–534.
45. Gallagher S. The natural philosophy of agency. Philosophy Compass. (2007) 2:347–57. doi: 10.1111/j.1747-9991.2007.00067.x
46. Haggard P, Clark S, Kalogeras J. Voluntary action and conscious awareness. Nat Neurosci. (2002) 5:382–5. doi: 10.1038/nn827
47. Thelen E. Rhythmical stereotypies in normal human infants. Anim Behav. (1979) 27(Pt 3):699–715. doi: 10.1016/0003-3472(79)90006-X
48. Evans DW, Leckman JF, Carter A, Reznick JS, Henshaw D, King RA, et al. Ritual, habit, and perfectionism: the prevalence and development of compulsive-like behavior in normal young children. Child Dev. (1997) 68:58–68. doi: 10.1111/j.1467-8624.1997.tb01925.x
50. Leckman JF, Bloch MH, King RA, Scahill L. Phenomenology of tics and natural history of tic disorders. Adv Neurol. (2006) 99:1–16.
51. Jankovic J. Tourette syndrome. Phenomenology and classification of tics. Neurol Clin. (1997) 15:267–75. doi: 10.1016/S0733-8619(05)70311-X
52. Verdellen C, van de Griendt J, Hartmann A, Murphy T. European clinical guidelines for Tourette syndrome and other tic disorders. Part III: behavioural and psychosocial interventions. Eur Child Adolesc Psychiatry. (2011) 20:197–207. doi: 10.1007/s00787-011-0167-3
53. Ganos C, Ogrzal T, Schnitzler A, Münchau A. The pathophysiology of echopraxia/echolalia: relevance to Gilles de la Tourette syndrome. Mov Disord. (2012) 27:1222–9. doi: 10.1002/mds.25103
54. Freeman RD, Zinner SH, Müller-Vahl KR, Fast DK, Burd LJ, Kano Y, et al. Coprophenomena in tourette syndrome. Development Med Child Neurol. (2009). 51:218–27. doi: 10.1111/j.1469-8749.2008.03135.x
55. Association AP. Diagnostic and statistical manual of mental disorders (DSM-5®). Virginia: American Psychiatric Pub (2013).
56. Robertson MM. Tourette syndrome, associated conditions and the complexities of treatment. Brain. (2000) 123:425–62. doi: 10.1093/brain/123.3.425
57. Leckman JF, Walker DE, Cohen DJ. Premonitory urges in Tourette's syndrome. Am J Psychiatr. (1993) 150:98–102. doi: 10.1176/ajp.150.1.98
58. Ganos C, Roessner V, Münchau A. The functional anatomy of Gilles de la Tourette syndrome. Neurosci Biobehav Rev. (2013) 37:1050–62. doi: 10.1016/j.neubiorev.2012.11.004
59. Franzkowiak S, Pollok B, Biermann-Ruben K, Südmeyer M, Paszek J, Jonas M, et al. Altered pattern of motor cortical activation-inhibition during voluntary movements in Tourette syndrome. Mov Disord. (2010) 25:1960–6. doi: 10.1002/mds.23186
60. Franzkowiak S, Pollok B, Biermann-Ruben K, Südmeyer M, Paszek J, Thomalla G, et al. Motor-cortical interaction in Gilles de la Tourette syndrome. PLoS ONE. (2012) 7:e27850. doi: 10.1371/journal.pone.0027850
61. Polyanska L, Critchley HD, Rae CL. Centrality of prefrontal and motor preparation cortices to Tourette Syndrome revealed by meta-analysis of task-based neuroimaging studies. Neuroimage Clin. (2017) 16:257–67. doi: 10.1016/j.nicl.2017.08.004
62. Obeso JA, Rothwell JC, Marsden CD. Simple tics in Gilles de la Tourette's syndrome are not prefaced by a normal premovement EEG potential. J Neurol Neurosurg Psychiatry. (1981) 44:735–8. doi: 10.1136/jnnp.44.8.735
63. Karp BI, Porter S, Toro C, Hallett M. Simple motor tics may be preceded by a premotor potential. J Neurol Neurosurg Psychiatry. (1996) 61:103–6. doi: 10.1136/jnnp.61.1.103
64. Papa SM, Artieda J, Obeso JA. Cortical activity preceding self-initiated and externally triggered voluntary movement. Mov Disord. (1991) 6:217–24. doi: 10.1002/mds.870060305
65. Kranick SM, Hallett M. Neurology of volition. Exp Brain Res. (2013) 229:313–27. doi: 10.1007/s00221-013-3399-2
66. Kanaan RAA. Freud's hysteria and its legacy. Handb Clin Neurol. (2016) 139:37–44. doi: 10.1016/B978-0-12-801772-2.00004-7
67. Hallett M. Physiology of psychogenic movement disorders. J Clin Neurosci. (2010) 17:959–65. doi: 10.1016/j.jocn.2009.11.021
68. Hallett M. Volitional control of movement: the physiology of free will. Clin Neurophysiol. (2007) 118:1179–92. doi: 10.1016/j.clinph.2007.03.019
69. Kranick S, Ekanayake V, Martinez V, Ameli R, Hallett M, Voon V. Psychopathology and psychogenic movement disorders. Mov Disord. (2011) 26:1844–50. doi: 10.1002/mds.23830
70. Stone J, Edwards MJ. How “psychogenic” are psychogenic movement disorders? Mov Disord. (2011) 26:1787–8. doi: 10.1002/mds.23882
71. Edwards MJ, Stone J, Lang AE. From psychogenic movement disorder to functional movement disorder: it's time to change the name. Mov Disord. (2014) 29:849–52. doi: 10.1002/mds.25562
72. Labate A, Cerasa A, Mula M, Mumoli L, Gioia MC, Aguglia U, et al. Neuroanatomic correlates of psychogenic non-epileptic seizures: a cortical thickness and VBM study. Epilepsia. (2012) 53:377–85. doi: 10.1111/j.1528-1167.2011.03347.x
73. Voon V, Brezing C, Gallea C, Ameli R, Roelofs K, LaFrance WC, et al. Emotional stimuli and motor conversion disorder. Brain. (2010) 133:1526–36. doi: 10.1093/brain/awq054
74. Voon V, Brezing C, Gallea C, Hallett M. Aberrant supplementary motor complex and limbic activity during motor preparation in motor conversion disorder. Mov Disord: Offic J Mov Disord Soc. (2011) 26:2396–403. doi: 10.1002/mds.23890
75. Czarnecki K, Jones DT, Burnett MS, Mullan B, Matsumoto JY. SPECT perfusion patterns distinguish psychogenic from essential tremor. Parkinsonism Relat Disord. (2011) 17:328–32. doi: 10.1016/j.parkreldis.2011.01.012
76. Edwards MJ, Fotopoulou A, Pareés I. Neurobiology of functional (psychogenic) movement disorders. Curr Opin Neurol. (2013) 26:442–7. doi: 10.1097/WCO.0b013e3283633953
77. Gupta A, Lang AE. Psychogenic movement disorders. Curr Opin Neurol. (2009) 22:430–6. doi: 10.1097/WCO.0b013e32832dc169
78. van Poppelen D, Saifee TA, Schwingenschuh P, Katschnig P, Bhatia KP, Tijssen MA, et al. Attention to self in psychogenic tremor. Mov Disord. (2011) 26:2575–6. doi: 10.1002/mds.23911
79. Schrag AE, Mehta AR, Bhatia KP, Brown RJ, Frackowiak RSJ, Trimble MR, et al. The functional neuroimaging correlates of psychogenic vs. organic dystonia. Brain : J Neurol. (2013) 136(Pt 3):770–81. doi: 10.1093/brain/awt008
80. Pareés I, Saifee TA, Kassavetis P, Kojovic M, Rubio-Agusti I, Rothwell JC, et al. Believing is perceiving: mismatch between self-report and actigraphy in psychogenic tremor. Brain. (2012) 135(Pt 1):117–23. doi: 10.1093/brain/awr292
81. Edwards MJ, Lang AE, Bhatia KP. Stereotypies: a critical appraisal and suggestion of a clinically useful definition. Mov Disord. (2012) 27:179–85. doi: 10.1002/mds.23994
82. Niehaus DJ, Emsley RA, Brink P, Stein DJ. Stereotypies: prevalence and association with compulsive and impulsive symptoms in college students. Psychopathology. (2000) 33:31–5. doi: 10.1159/000029116
83. Muthugovindan D, Singer H. Motor stereotypy disorders. Curr Opin Neurol. (2009) 22:131–6. doi: 10.1097/WCO.0b013e328326f6c8
84. Tan A, Salgado M, Fahn S. The characterization and outcome of stereotypical movements in non-autistic children. Mov Disord. (1997) 12:47–52. doi: 10.1002/mds.870120109
85. Mahone EM, Bridges D, Prahme C, Singer HS. Repetitive arm and hand movements (complex motor stereotypies) in children. J Pediatrics. (2004) 145:391–5. doi: 10.1016/j.jpeds.2004.06.014
86. Harris KM, Mahone EM, Singer HS. Non-autistic motor stereotypies: clinical features and longitudinal follow-up. Pediatric Neurol. (2008) 38:267–72. doi: 10.1016/j.pediatrneurol.2007.12.008
87. MacDonald R, Green G, Mansfield R, Geckeler A, Gardenier N, Anderson J, et al. Stereotypy in young children with autism and typically developing children. Res Dev Disabil. (2007) 28:266–77. doi: 10.1016/j.ridd.2006.01.004
89. Shukla T, Pandey S. Stereotypies in adults: a systematic review. Neurol Neurochir Pol. (2020) 54:294–304. doi: 10.5603/PJNNS.a2020.0058
90. Singer HS. Motor stereotypies. Semin Pediatr Neurol. (2009) 16:77–81. doi: 10.1016/j.spen.2009.03.008
91. Zentall SS, Zentall TR. Optimal stimulation: a model of disordered activity and performance in normal and deviant children. Psychol Bull. (1983) 94:446–71. doi: 10.1037/0033-2909.94.3.446
92. Kates WR, Lanham DC, Singer HS. Frontal white matter reductions in healthy males with complex stereotypies. Pediatr Neurol. (2005) 32:109–12. doi: 10.1016/j.pediatrneurol.2004.09.005
93. Kulisevsky J, Berthier ML, Avila A, Roig C. Unilateral parkinsonism and stereotyped movements following a right lenticular infarction. Mov Disord. (1996) 11:752–4. doi: 10.1002/mds.870110630
94. Maraganore DM, Lees AJ, Marsden CD. Complex stereotypies after right putaminal infarction: a case report. Mov Disord. (1991) 6:358–61. doi: 10.1002/mds.870060418
95. Houdayer E, Walthall J, Belluscio BA, Vorbach S, Singer HS, Hallett M. Absent movement-related cortical potentials in children with primary motor stereotypies. Mov Disord. (2014) 29:1134–40. doi: 10.1002/mds.25753
96. Chartoff EH, Marck BT, Matsumoto AM, Dorsa DM, Palmiter RD. Induction of stereotypy in dopamine-deficient mice requires striatal D1 receptor activation. Proc Natl Acad Sci U S A. (2001) 98:10451–6. doi: 10.1073/pnas.181356498
97. Canales JJ, Graybiel AM. A measure of striatal function predicts motor stereotypy. Nat Neurosci. (2000) 3:377–83. doi: 10.1038/73949
98. Fog R. On stereotypy and catalepsy: studies on the effect of amphetamines and neuroleptics in rats. Acta Neurol Scand Suppl. (1972) 50:3–66.
99. Arnt J. Antistereotypic effects of dopamine D-1 and D-2 antagonists after intrastriatal injection in rats. pharmacological and regional specificity. Naunyn Schmiedebergs Arch Pharmacol. (1985) 330:97–104. doi: 10.1007/BF00499901
100. Hotz G, Helm-Estabrooks N. Perseveration. part i: a review. Brain injury. (2009) 9:151–9. doi: 10.3109/02699059509008188
101. Liepmann H. Die perseveration. Ueber Storungen des Handelns bei Gerhirnkranken. Berlin: Karger. (1905) 5:115–27.
102. Luria AR. Two kinds of motor perseveration in massive injury of the frontal lobes. Brain. (1965) 88:1–10. doi: 10.1093/brain/88.1.1
103. Fung VS, Morris JG, Leicester J, Soo YS, Davies L. Clonic perseveration following thalamofrontal disconnection: a distinctive movement disorder. Mov Disord. (1997) 12:378–85. doi: 10.1002/mds.870120317
104. Stuss DT, Knight RT. Principles of Frontal Lobe Function. Oxford: Oxford University Press (2013).
105. McEnaney KW, Butter CM. Perseveration of responding and non-responding in monkeys with orbital frontal ablations. J Comp Physiol Psychol. (1969) 68:558–61. doi: 10.1037/h0027639
106. Wells M, Jacques R, Montero Odasso M. Thalamic infarct presenting as catastrophic life-threatening event in an older adult. Aging Clin Exp Res. (2011) 23:320–2. doi: 10.1007/BF03337758
107. Lee MS, Marsden CD. Movement disorders following lesions of the thalamus or subthalamic region. Mov Disord. (1994) 9:493–507. doi: 10.1002/mds.870090502
108. Aron AR, Poldrack RA. Cortical and subcortical contributions to stop signal response inhibition: role of the subthalamic nucleus. J Neurosci. (2006) 26:2424–33. doi: 10.1523/JNEUROSCI.4682-05.2006
109. Nambu A, Tokuno H, Takada M. Functional significance of the cortico-subthalamo-pallidal 'hyperdirect' pathway. Neurosci Res. (2002) 43:111–7. doi: 10.1016/S0168-0102(02)00027-5
110. Chen W, de Hemptinne C, Miller AM, Leibbrand M, Little SJ, Lim DA, et al. Prefrontal-subthalamic hyperdirect pathway modulates movement inhibition in humans. Neuron. (2020) 106:579–88. doi: 10.1016/j.neuron.2020.02.012
111. Hartmann A, Millet B. Repetitive movements and behaviors in neurological and psychiatric practice: distinctions and similarities between tourette disorder and obsessive-compulsive disorder. Rev Neurol (Paris). (2018) 174:199–202. doi: 10.1016/j.neurol.2018.01.364
112. Luigjes J, Lorenzetti V, de Haan S, Youssef GJ, Murawski C, Sjoerds Z, et al. Defining Compulsive Behavior. Neuropsychol Rev. (2019) 29:4–13. doi: 10.1007/s11065-019-09404-9
113. Leonard HL, Lenane MC, Swedo SE, Rettew DC, Gershon ES, Rapoport JL. Tics and Tourette's disorder: a 2- to 7-year follow-up of 54 obsessive-compulsive children. Am J Psychiatry. (1992) 149:1244–51. doi: 10.1176/ajp.149.9.1244
114. Pitman RK, Green RC, Jenike MA, Mesulam MM. Clinical comparison of Tourette's disorder and obsessive-compulsive disorder. Am J Psychiatry. (1987) 144:1166–71. doi: 10.1176/ajp.144.9.1166
115. O'Sullivan RL, Mansueto CS, Lerner EA, Miguel EC. Characterization of trichotillomania. a phenomenological model with clinical relevance to obsessive-compulsive spectrum disorders. Psychiatr Clin North Am. (2000) 23:587–604. doi: 10.1016/S0193-953X(05)70182-9
116. Saxena S, Rauch SL. Functional neuroimaging and the neuroanatomy of obsessive-compulsive disorder. Psychiatr Clin North Am. (2000) 23:563–86. doi: 10.1016/S0193-953X(05)70181-7
117. Piras F, Piras F, Chiapponi C, Girardi P, Caltagirone C, Spalletta G. Widespread structural brain changes in OCD: a systematic review of voxel-based morphometry studies. Cortex. (2015) 62:89–108. doi: 10.1016/j.cortex.2013.01.016
118. Dayan A, Berger A, Anholt GE. Enhanced action tendencies in obsessive-compulsive disorder: an ERP study. Behaviour Research and Therapy. (2017) 93:13–21. doi: 10.1016/j.brat.2017.03.005
119. Takashima S, Najman FA, Ramos RT. Disruption of volitional control in obsessive-compulsive disorder: evidence from the bereitschaftspotential. Psychiatry Res Neuroimaging. (2019) 290:30–7. doi: 10.1016/j.pscychresns.2019.06.007
120. Lhermitte F. ‘Utilisation Behaviour' and its relation to lesions of the frontal lobes. Brain. (1983). 106:237–55. doi: 10.1093/brain/106.2.237
121. Besnard J, Allain P, Aubin G, Osiurak F, Chauviré V, Etcharry-Bouyx F, et al. Utilization behavior: clinical and theoretical approaches. J Int Neuropsychol Soc. (2010) 16:453–62. doi: 10.1017/S1355617709991469
122. Brüne M. Ethological remarks on mannerisms. Psychopathology. (1998) 31:188–96. doi: 10.1159/000029039
123. Kaufmann C, Agalawatta N, Malhi GS. Catatonia: stereotypies, mannerisms and perseverations. Austr J Psychiatry. (2018) 52:391–3. doi: 10.1177/0004867418765669
124. Lees AJ. Odd and unusual movement disorders. J Neurol Neurosurg Psychiatry. (2002) 72:i17–i21. doi: 10.1136/jnnp.72.suppl_1.i17
Keywords: semivoluntary movement, tics, functional movement disorders, stereotypy, perseveration, compulsion, utilization behavior, mannerism
Citation: Virameteekul S and Bhidayasiri R (2022) We Move or Are We Moved? Unpicking the Origins of Voluntary Movements to Better Understand Semivoluntary Movements. Front. Neurol. 13:834217. doi: 10.3389/fneur.2022.834217
Received: 13 December 2021; Accepted: 21 January 2022;
Published: 21 February 2022.
Edited by:
Pramod Kumar Pal, National Institute of Mental Health and Neurosciences (NIMHANS), IndiaReviewed by:
Matteo Bologna, Sapienza University of Rome, ItalyShweta Prasad, National Institute of Mental Health and Neurosciences (NIMHANS), India
Copyright © 2022 Virameteekul and Bhidayasiri. This is an open-access article distributed under the terms of the Creative Commons Attribution License (CC BY). The use, distribution or reproduction in other forums is permitted, provided the original author(s) and the copyright owner(s) are credited and that the original publication in this journal is cited, in accordance with accepted academic practice. No use, distribution or reproduction is permitted which does not comply with these terms.
*Correspondence: Roongroj Bhidayasiri, cmJoJiN4MDAwNDA7Y2h1bGFwZC5vcmc=