- 1School of Chinese Materia Medica, Beijing University of Chinese Medicine, Beijing, China
- 2School of Traditional Chinese Medicine, Beijing University of Chinese Medicine, Beijing, China
- 3Beijing Institute of Traditional Chinese Medicine, Beijing University of Chinese Medicine, Beijing, China
- 4Ethnic Medicine Characteristic Diagnosis and Treatment Center, Guangxi International Zhuang Medicine Hospital, Nanning, China
Mental health has become a new challenge in cancer treatment, with a high prevalence of depression in patients with cancer. Albiflorin (AF) and paeoniflorinn (PF) are isomers extracted from the root of Paeoniae Radix Alba (Baishao in Chinese), belonging to the monoterpene glycosides, and multiple studies have been conducted on their antidepression and anti-cancer effects. However, the effects of AF and PF on cancer-related depression are unclear. Therefore, the current study aims to investigate whether the two isomers are able to exert antidepressant-like effects and understand the underlying mechanisms in a rat model, established by combining irradiation with chronic restraint stress and solitary confinement. Our results demonstrate a significant regulation of AF and PF in the pharmacodynamic index, including the peripheral blood, organ index, behavioral traits, and HPA axis, relative to control rats. In serum and cerebral cortex metabonomics analysis, AF and PF showed a significantly restorative trend in abnormal biomarkers and regulating ether lipid metabolism, alanine, aspartate, glutamate metabolism, tryptophan metabolism, carnitine metabolism, arachidonic acid metabolism, arginine and proline metabolism pathway. Eight potential biomarkers were further screened by means of receiver operating characteristic (ROC) analysis. The data indicate that AF and PF could effectively ameliorate a depression-like state in the model rats, and the mechanism may be associated with the regulation of the neuroendocrine immune system and disrupted metabolic pathways. Further experiments are warranted to comprehensively evaluate the antidepressant effects of AF and PF in cancer-related depression. This study provides a better insight into the action mechanisms of antidepression of TCM, and provides a new perspective for the therapy of cancer-related depression.
Introduction
Cancer has a high morbidity and mortality worldwide: global cancer statistics for 36 cancers in 185 countries estimated 19.3 million new cancer cases and 10.0 million cancer deaths occurred in 2020 (1). Although several interventions, such as radiotherapy and chemotherapy, have improved the survival rates of cancer, new challenges have emerged, including psychological or mental health, with the prevalence of depression being high in patients with cancer (2, 3). A cross-sectional analysis of clinical data found that the prevalence of major depression ranged from 5.6 to 13.1% in several common cancers (4). Cancer-related psychological distress may limit or prevent the treatment course and recovery (5), reduce quality of life and increase health-care costs (6), while, even more problematically, some patients have suicidal thoughts (7). Health departments and cancer commissions in the US and the UK have all called for more attention to be paid to the depression in cancer patients (4). However, because of the lack of awareness among medical personnel and family caregivers, distress in cancer patients is frequently overlooked and under-treated (8). Furthermore, there are few effective pharmacotherapies (9, 10). Therefore, more attention and effective interventions are urgently needed.
Paeoniae Radix Alba (Baishao, PRA), derived from the dried root of Paeonia lactiflora Pall., is one of the most commonly used medicinal herbs in China (11), with the characteristics of nourishing the blood and smoothing the liver. Modern medical research has found that PRA has good analgesic, sedative and anti-inflammatory effects, and it is usually indicated for painful conditions, menstrual disorders and viral infections (12). Simultaneously, there are reports in the literature regarding the anticancer activities of PRA (13), and its major active constituents, paeoniflorin (PF) (14), and total glucosides of paeony (TGP) (15). However, few anticancer studies regarding albiflorin (AF), a monoterpene glycoside that is isomeric with PF (16), have been reported, which may be related to the difficulty found in its isolation and purification. The good news here is that our team has successfully isolated and purified AF and PF with high purity, and the method has also been obtained a Chinese patent certificate (ZL 201110184287.4). The hematopoietic effects of AF and PF were proven on radiotherapy-induced and chemotherapy-induced myelosuppression mice in our previous papers (17, 18), for which a Chinese patent certificate has also been obtained (ZL 201380078231.8). Concurrently, multiple studies have demonstrated that formulations of PRA have good antidepressant effects, including PF (19, 20), AF (21, 22), and Chinese prescription, such as Xiaoyaosan (23, 24), Sinisan (25), and Chaihu-Shu-Gan-San (26). Compared with the existing antidepressants in western medicine, which have a single mechanism of action, are ineffective and have obvious side effects in some patients, these formulations have the advantages of rapid action, high safety, and multi-target action (27), which may offer a new option for the treatment of depression.
In this paper, taking advantage of previous research, we propose the hypothesis that AF and PF have antidepressant-like effects on depression in radiotherapy. To confirm our hypothesis, we established a rat model by combining irradiation with chronic restraint stress and solitary confinement, according to the state of depression in radiotherapy patients in a clinic, using the method previously confirmed by our team (28). Then, metabonomics was applied to investigate the therapeutic mechanisms (Figure 1). Our research provides an experimental basis for the clinical application of the antidepression effects of AF and PF in cancer radiotherapy patients.
Materials and Methods
Chemicals and Materials
MS-grade acetonitrile, methanol, isopropanol, water, ammonium acetate and foric acid were purchased from Fisher (Waltham, MA, USA). The blood routine test, adrenocorticotropic hormone, corticosterone and the hypothalamic kit were provided by Beijing Sinouk Institute of Biological Technology. Paeoniae Radix Alba (PRA) was purchased from YuLong Chinese medicine Company (Anguo, China) and authenticated before preparation by Prof. Zhang-Jian Jun, Beijing University of Chinese Medicine. The extract of PRA was extracted with water, and subject to vacuum concentrated drying (paeoniflorin =1.8% > 1.2% specified in Pharmacopeia of the People's Republic of China). Albiflorin (AF, purity = 98.6%) and paeoniflorin (PF, purity = 96.7%) were prepared in our library (patent number ZL 201110184287.4, China).
Animal Experiment
A total of 40 male Sprague Dawley rats, weighing 200 ± 20 g, were housed for 5 days under controlled conditions before the experiments took place. The investigation was conducted in accordance with the Experimental Animal Ethics Committee of Beijing University of Chinese Medicine (No. BUCM-4-2017041807-2007). Throughout the study, all animals were kept under standard conditions regarding temperature (22 ± 2°C) and humidity (50 ± 10%), with a 12 h light/dark cycle. After several days of habituation, all the rats were randomly assigned to the following five groups: (1) normal group (N), (2) model group (M), (3) PRA group (PRA), (4) AF group (AF) and (5) PF group (PF), with each experimental group consisting of eight mice. With the exception of those from the control and model group, rats were intragastrically (i.g.) pretreated with the Paeoniae Radix Alba (2 g/kg), PF (30 mg/kg), and AF (30 mg/kg) for 21 days according to the previous study (16–18). The rats, with the exception of the normal group, were individually housed and received chronic restraint stress in the form of restricted movement for 3 h (8:00 a.m.−11:00 a.m.) for 21 days. On the 8th day, the model rats received total body irradiation of 3.5 Gy Co60γ-rays at a dose rate of 1.60 Gy. After irradiation, the administration of chronic restraint stress was continued for another 14 consecutive days.
Sample Collection and Preparation
After the intragastric administration of PRA, PF and AF on the 21st day, all of the rats were anesthetized with a peritoneal injection of chloral hydrate, and blood samples were collected quickly in tubes from the abdominal aorta. Serum was obtained by means of centrifugation at 3,000 × g and 4°C for 10 min, and was frozen immediately at −80°C prior to analysis. For metabonomics analysis, serum was thawed at 4°C, and the samples were divided into two parts, namely the polar and non-polar parts. During the preparation of polar parts 100 μl of serum was aliquoted into a labeled 1.5 ml microcentrifuge tube, to which 300 μl of acetonitrile was added; this mixture was thoroughly mixed in a vortex mixer for 15 s, and the protein precipitate was pelleted in a centrifuge operating at 4°C and at 12,000 rpm for 10 min. We then transferred 100 μl of the supernatant into a 200 μl vial insert for analysis. In the preparation of non-polar parts, 100 μl of serum was aliquoted into a labeled 1.5 ml microcentrifuge tube and 600 μl chloroform/methanol (3:1) was added; the mixture was then ultrasonicated for 1 h, and then we added 100 μl of water, and mixed thoroughly. The mixture was then centrifuged at 4°C and at 12,000 rpm for 10 min. We took 300 μl of subnatant to dry, and then added 400 μl isopropyl alcohol/acetonitrile (1:1), and dissolved it using ultrasonication. This mixture was centrifuged at 12,000 rpm for 10 min, followed by transferring 100 μl of the supernatant into a 200 μl vial insert for analysis. Cerebral cortex tissue samples were weighed and ground, and 1 ml of methanol was added in order for the samples to mix well. The samples were thoroughly mixed in a vortex mixer for 30 min, and the protein precipitate was pelleted in a centrifuge operating at 4°C and at 12,000 rpm for 10 min. We then transferred 100 μl of supernatant into a 200 μl vial insert for analysis.
Pharmacodynamic Index
Body Weight
During the experiment, the rats were weighed every week, and their weight was recorded.
Organ Index
After anesthesia and blood collection, the abdominal cavity was quickly opened and the thymus, spleen and adrenal gland were separated on ice and weighed. Thymus (spleen, adrenal gland) index = thymus (spleen, adrenal gland) weight/body weight.
Sucrose Preference Test
Rats were adapted to a 1% sucrose bottle in an individual cage for 24 h, then one bottle of 1% sucrose water and one bottle of tap water were given. The intake volumes were recorded, on days 0 and 21 of the experiment. Then, the sucrose consumption rate (%) = sucrose consumption/(water consumption + sucrose consumption) × 100%.
Open Field Test
On the 21st day of restraint, this test was carried out in an independent, quiet and dark room. Rats were placed in the center of an open box (80 cm × 80 cm × 40 cm), which had 25 equal squares (16 × 16 cm2) on the bottom with white lines, while the sides and bottom were black. The activity of the rat was observed and recorded within 3 min. The horizontal activity was based on the number of squares crossed by rat, and the vertical activity was based on the number of times the rat was upright (two forelimbs are more than 1 cm above the ground). After each rat completed the test, the open box was treated with alcohol to avoid leaving the smell of the previous rat.
Elevated Plus-Maze Test
On the 21st day of restraint, this test was carried out. The elevated plus-maze was composed of two opposing open arms (45 cm × 10 cm), two opposing closed arms (45 cm × 10 cm), and a central platform (10 cm × 10 cm) connecting the four arms, in the shape of a cross, and the inner wall and bottom were black, 50 cm above the ground. Rats were placed in the center of the central platform facing any open arm, and then we observed the rat's activity for 5 min, including: (1) the number of open arm entries, (2) the residence time in open arms, (3) the number of closed arm entries, (4) the residence time in closed arms. After one rat was measured, the maze was cleaned with a towel dipped in a low concentration of alcohol, and then the next test was carried out after the alcohol was dispersed.
Biochemical Indicators Examination
The contents of white blood cells (WBC), red blood cells (RBC) and hemoglobin (HGB) of peripheral blood were measured by an automatic blood cell analysis counter. Corticotropin-releasing hormone (CRH), adrenocorticotropic hormone (ACTH), and corticosterone (CORT) were measured using the rat enzyme linked immunosorbent assay (ELISA) kit according to the manufacturer's instructions.
HPLC-LTQ/Orbitrap MS Analysis
The Thermo Scientific™ Dionex™ UltiMate™ 3000 Rapid Separation LC (RSLC) system performed UHPLC separations with a reversed phase C18 column or a hydrophilic interaction liquid chromatography column using the gradient conditions. RP separation for lipids: For C18 separation, mobile phase A was acetonitrile/water (60/40) and mobile phase B was isopropanol/ acetonitrile (90/10); both A and B contained 0.1% formic acid and 10 mmol/L ammonium acetate. The gradient conditions for reversed phase C18 separation were 0–2 min, 80%−70% A, 20%−30% B; 2–5 min, 70%−55% A, 30%−45% B; 5–6.5 min, 55%−40% A, 45%−60% B; 6.5–12 min, 40%−35% A, 60%−65% B; 12–14 min, 35%−15% A, 65%−85% B; 14–17.5 min, 15%−0% A, 85%−100% B; 17.5–18 min, 0%−0% A, 100%−100% B; 18–18.1 min, 0%−80% A, 100%−20% B; 18.1–19.5 min, 80%−80% A, 20%−20% B. The column was a HSS T3 column (2.1 × 100 mm, 1.8 μm, water) operated at 45°C. The flow rate was 300 μl/min, and the injection volume was 1 μl. HILIC separation: For HILIC separation, mobile phase A was acetonitrile and mobile phase B was water; both A and B contained 0.1% formic acid and 10 mmol/L ammonium acetate. The gradient conditions were 0–1 min, 95%−95% A, 5%−5% B; 1–7 min, 95%−50% A, 5%−50% B; 7–9 min, 50%−50% A, 50%−50% B; 9–9.1 min, 50%−95% A, 50%−5% B; 9.1–13 min, 95%−95% A, 5%−5% B. The column was a BEH Amide column (2.1 × 100 mm, 1.7 μm, waters) operated at 40°C. The flow rate was 300 μl/min, and the injection volume was 1 μl.
A Thermo Scientific™ Q Exactive™ hybrid quadrupole Orbitrap mass spectrometer equipped with a HESI-II probe was employed. The positive and negative HESI-II spray voltages were 3.7 and 3.5 kV, respectively, the heated capillary temperature was 320°C, the sheath gas pressure was 30 psi, the auxiliary gas setting was 10 psi, and the heated vaporizer temperature was 300°C. Both the sheath gas and the auxiliary gas were nitrogen. The collision gas was also nitrogen, at a pressure of 1.5 mTorr. The parameters of the full mass scan were as follows: a resolution of 70,000, an auto gain control target under 1 × 106, a maximum isolation time of 50 ms, and an m/z range of 150–1,500. The LC-MS system was controlled using Xcalibur 2.2 SP1.48 software (Thermo Fisher Scientific), and data were collected and processed with the same software.
Pattern Recognition Analysis and Data Processing
The raw data were analyzed using the Progenesis QI software for peak alignment, peak extraction and normalization. The extraction peak times of the non-polar part and polar part were 1–19 and 1–12 min, respectively, and the peak extraction intensity was limited to mode 3. Finally, to form a table detailing the retention time, mass/charge ratio and peak intensity, SIMCA14.1 software (Umetrics, Umea, Sweden) was used to perform principal component analysis (PCA) and partial least squares discriminate analysis (PLS-DA). According to the variable importance in the projection (VIP) of the Orthogonal PLS-DA (OPLS-DA) model (VIP > 1), we combined it with the P-value of the t-test (P < 0.05) to find the differentially expressed metabolites. At the same time, the structure of potential biomarkers was determined based on the fragmentation information of mass spectrometry and the accurate mass number, combined with HMDB, KEGG and other databases (error limit: 0.01 Da). Potential biomarkers were analyzed in MetaboAnalyst 4.0 (http://www.metaboanalyst.ca/), and screening impact value was greater than 0.1 pathways for potential related metabolic pathways. The Pearson correlation analysis between metabolites and pharmacodynamic index was also carried out on the MetaboAnalyst.
Statistical Analysis
The data were analyzed using one-way analysis of variance (ANOVA) of IBM SPSS Statistics 20, followed by Tukey's test as a post hoc test. The values are expressed as the mean ± standard deviation (SD). Values of P < 0.05 were accepted as being statistically significant. All figures were created using GraphPad Prism® software 7.0.
Results
Intervention Effects of Albiflorin and Paeoniflorin on the Pharmacodynamic Index on Model Rats
The pharmacodynamic index, including body weight, peripheral blood, organ index, and HPA axis, is displayed in Figure 2, and the behavioral experiment is shown in Tables 1, 2. With the prolongation of the modeling time, the rats became significantly lower in weight compared with the normal group (P < 0.001), and PRA, AF and PF significantly increased the body weight on the 21st day (P < 0.01, P < 0.001; Figure 2A).
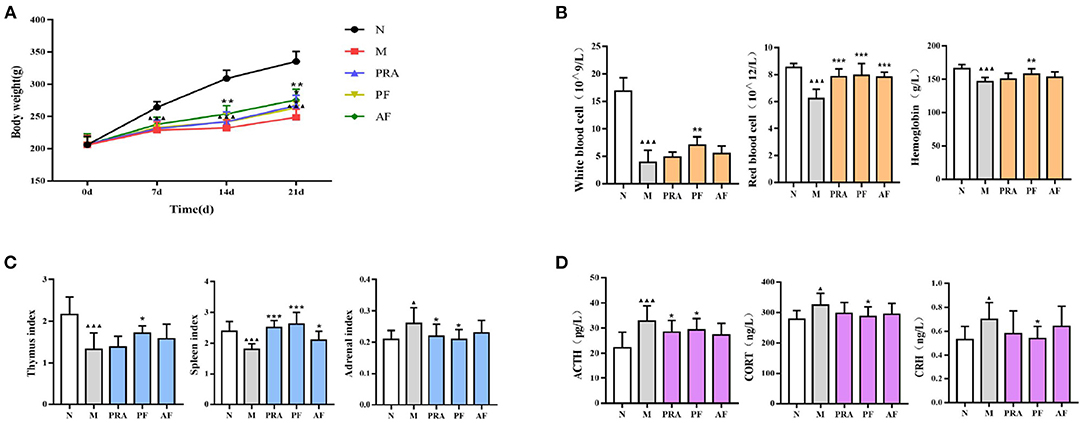
Figure 2. Changes in the pharmacodynamic index among groups. (A) The body weight of rats; (B) white blood cell, red blood cell and hemoglobin count in the peripheral blood; (C) thymus, spleen and adrenal organ index; (D) the HPA axis. The data are expressed as mean ± SD (n = 8). ▴P < 0.05, ▴▴▴P < 0.001, compared with the normal group; *P < 0.05, **P < 0.01, ***P < 0.001, compared with the model group. N, normal group; M, model group; PRA, Aqueous extract of Paeoniae Radix Alba group; PF, paeoniflorin group; AF, albiflorin group.
The WBC, RBC and HGB count in peripheral blood was used to evaluate body immunity, which in the model group was significantly decreased compared with the normal group (P < 0.001), while the WBC, RBC and HGB count in the PF group was significantly increased (P < 0.01, P < 0.001), and AF can increased RBC significantly (P < 0.001; Figure 2B).
The organ index can also be use to evaluate immunity. Compared with the normal group, the thymus index and the spleen index of the model group were significantly decreased (P < 0.001), and the adrenal index was significantly increased (P < 0.05). PRA significantly increased the spleen index and decreased the adrenal index (P < 0.001, P < 0.05). PF significantly increased the thymus and the spleen index (P < 0.001, P < 0.05), and decreased the adrenal index (P < 0.05), while AF increased the spleen index (P < 0.05; Figure 2C).
The HPA axis plays an important role in the pathogenesis of depression. In the results, compared with the normal group, the ACTH, CORT and CRH content in model group was significantly decreased (P < 0.01, P < 0.001), PF significantly increased these contents (P < 0.05), and the paeoniflorin group had an increased ACTH content (P < 0.05), while AF also demonstrated an increase (Figure 2D).
From the behavioral experiment, in which sugar water consumption, open field and elevated plus maze movement were restricted (P < 0.05, P < 0.01, P < 0.001), AF and PF can ameliorate behavioral deficits (P < 0.05, P < 0.01, P < 0.001). The pharmacodynamic index results indicated that AF and PF can antagonize depression and blood deficiency in model rats by regulating the neuroendocrine-immune network.
Metabolic Profiles Analysis Based on PCA and OPLS-DA Analysis
PCA is a non-supervisory model analysis method, which can classify the data according to their similarity, and can more truly reflect the differences between groups and identify the variation within groups. In PCA, R2X and Q2 are generally used to judge the quality of the model. R2X represents the interpretation rate of the model, and Q2 represents the predictable variables of the model (29). Generally, the greater the value of R2X and Q2, the more reliable the model is. The PCA analysis results of the QC sample and the normal group and the model group are shown in Figures 3, 4, respectively. As can be seen, the relative aggregation of QC samples demonstrated that the system had good repeatability, and the collected data can be further studied. The model group were obviously separated from the normal group. The R2X of the non-polar part was 0.736, and the R2X of the polar part was 0.614 in the serum sample, while in the cerebral cortex, the non-polar part's R2X was 0.734 and the polar part's R2X was 0.510, indicating that most of the data in the sample were used to establish the principal components, and the model was basically established successfully.
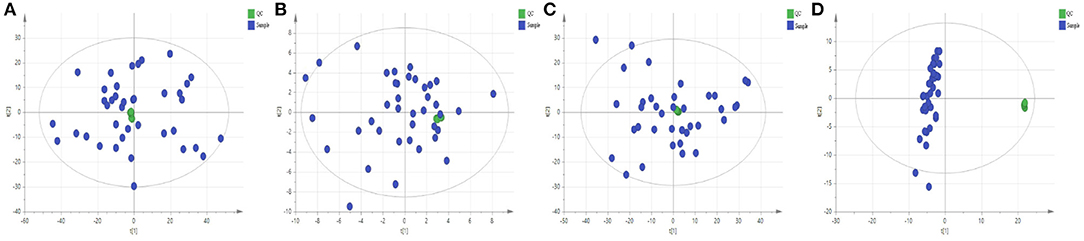
Figure 3. Quality control sample evaluation. (A) Positive mode of polar part of the serum; (B) Positive mode of non-polar part of the serum; (C) Positive mode of polar part of the cerebral cortex; (D) Positive mode of non-polar part of the cerebral cortex.
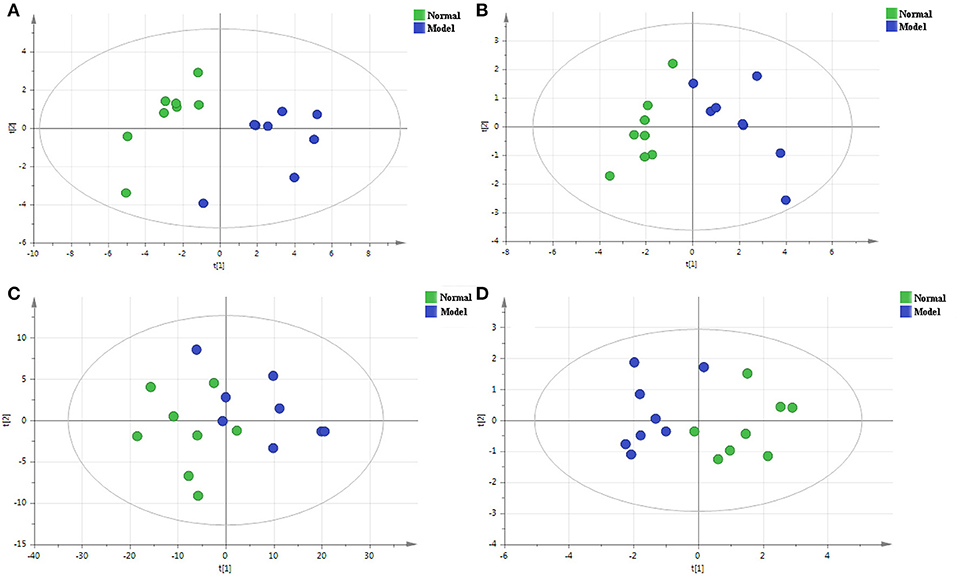
Figure 4. The PCA score plots of the normal group and the model group in the positive mode of the non-polar part (A) and the positive mode of the polar part (B) of the serum, and in the positive mode of the non-polar part (C) and the positive mode of polar part (D) of the cerebral cortex.
Compared with PCA, OPLS-DA is a supervisory model analysis method. The main parameters to judge the quality of the model are R2Y (the value represents the interpretation rate of the model) and Q2 (the value represents the prediction rate of the model). According to PCA, serum samples of the normal group and the model group were different to some extent. In order to obtain metabolite information leading to such significant differences, the supervisory multidimensional statistical method OPLS-DA was used to analyze the data, and the results are shown in Figure 5. In the serum sample, for the non-polar part, R2Y = 0.988 and Q2 = 0.898, and for the polar part, R2Y = 0.908 and Q2 = 0.686, in the cerebral cortex sample, for the non-polar part, R2Y = 0.729 and Q2 = 0.42; and the polar part, R2Y = 0.93and Q2 = 0.774, indicating that the model was established successfully. The permutation test plots and S-plot of OPLS-DA are shown in Figure 6.
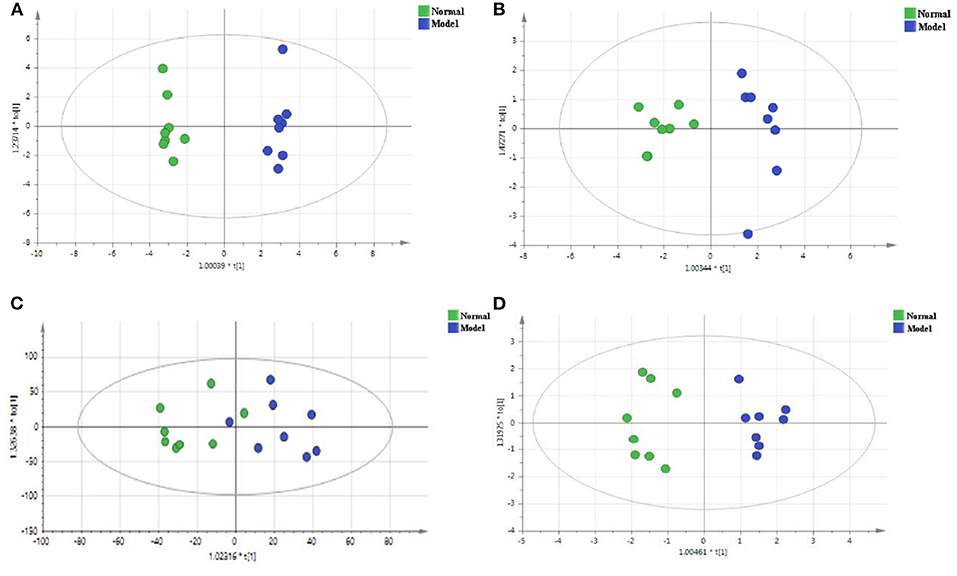
Figure 5. The OPLS-DA score plots of the normal group and the model group in the positive mode of the non-polar part (A) and the positive mode of the polar part (B) of the serum, and in the positive mode of the non-polar part (C) and the positive mode of the polar part (D) of the cerebral cortex.
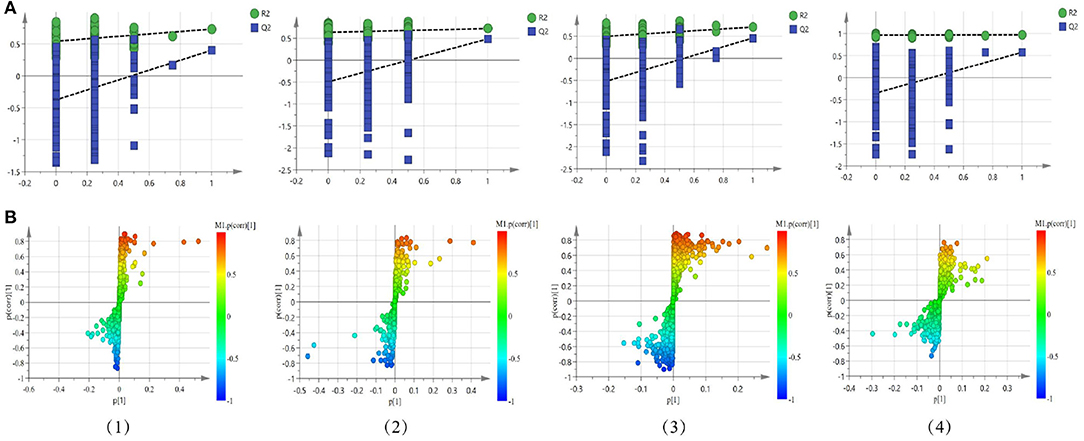
Figure 6. Permutation test plots (A) and S-plot (B) of OPLS-DA between the normal group and the model group in the positive mode of the non-polar part (1) and the positive mode of the polar part (2) of the serum, and in the positive mode of the non-polar part (3) and the positive mode of the polar part (4) of the cerebral cortex.
Identification of Potential Metabolites
In OPLS-DA, the potential biomarkers of metabolites were filtered by VIP > 1, excluding variables with large confidence intervals and connecting with the P-value of the peak area of the t-test (P < 0.05). In accordance with the above principle, 20 biomarkers and 12 biomarkers were, respectively, determined in the serum and the cerebral cortex. The detailed results are shown in Table 3. Metabolites displayed significant differences among the PRA, AF and PF groups and the model group. In the serum, PRA had an effect on 15 biomarkers (P < 0.05 or P < 0.01 or P < 0.001), AF had an effect on 11 biomarkers (P < 0.05 or P < 0.001), and PF had an effect on 10 biomarkers (P < 0.05 or P < 0.01). Meanwhile, in the cerebral cortex, LysoPE and another eight different metabolites were significantly reduced by PRA (P < 0.05 or P < 0.01 or P < 0.001). PF had significant effects on seven different metabolites (P < 0.05 or P < 0.01), and AF had similar effects to PF, significantly reducing the contents of seven different metabolites (P < 0.05 or P < 0.01). The above results indicate that the administration group demonstrated certain antidepressant effects.
Receiver operating characteristic (ROC) analysis was used to further evaluate the reliability of potential biomarkers (30), with the area under the ROC curve (AUC) > 0.9 as the standard, eight differential metabolites were further screened, five in a serum sample and three in a cerebral cortex sample, which may become potential markers of AF and PF for antidepression effects. The detailed results are shown in Figures 7, 8.
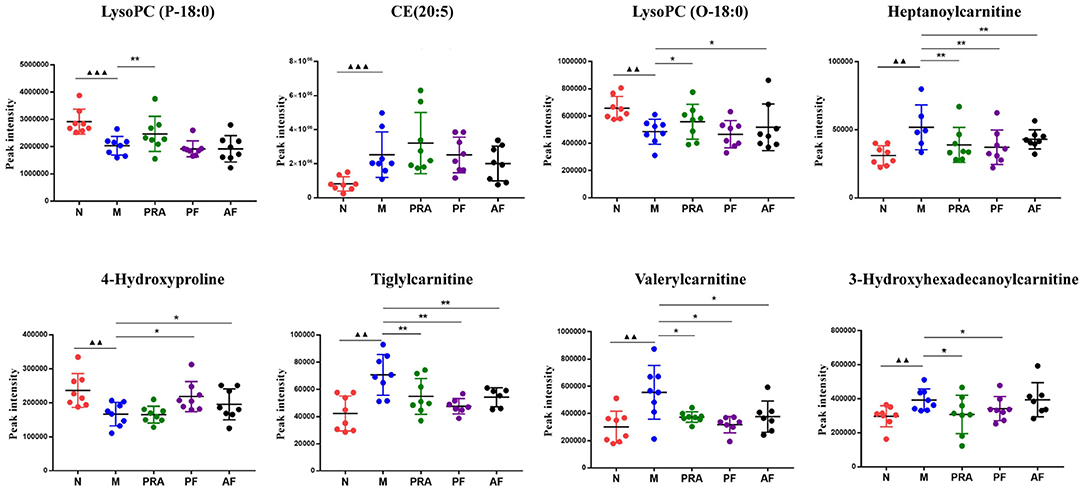
Figure 8. Potential biomarkers in the serum and cerebral cortex. ▴▴▴P < 0.001, compared with the normal group; *P < 0.05, **P < 0.01, compared with the model group. N, normal group; M, model group; PRA, Aqueous extract of Paeoniae Radix Alba group; PF, paeoniflorin group; AF, albiflorin group. ▴▴p < 0.01, compared with the normal group.
Metabolic Pathway Analysis
Metabolic pathway analyses were performed with MetaboAnalyst 4.0, an easy-to-use web-based tool suite (31). As shown in Figure 9, the metabolic pathways of serum were ether lipid metabolism; glycerophospholipid metabolism; alanine, aspartate, and glutamate metabolism; tryptophan metabolism; and arginine and proline metabolism, while in the cerebral cortex, the metabolic pathways were linoleic acid metabolism; alpha-linoleic acid metabolism; glycerophospholipid metabolism; and arachidonic acid metabolism.
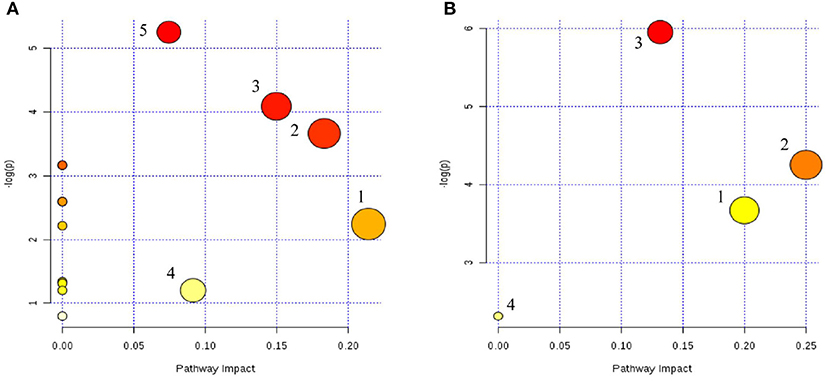
Figure 9. Serum (A) and cerebral cortex (B) differential metabolite-related metabolic pathways. (A) (1) Lipid metabolism; (2) glycerophospholipid metabolism; (3) alanine, aspartate, and glutamate metabolism; (4) tryptophan metabolism; and (5) arginine and proline metabolism. (B) (1) Linoleic acid metabolism; (2) alpha-linoleic acid metabolism; (3) glycerophospholipid metabolism; (4) arachidonic acid metabolism.
The same metabolic pathway was seen in two samples, which may reflect the characteristics of multi-target drug action.
Correlation Analysis Between Potential Biomarkers and Biochemical Indicators
Correlation analysis can reflect the relationship between different metabolites in metabolic pathways. The Pearson correlation analysis based on the MetaboAnalyst website (http://www.metaboanalyst.ca/) was used to screen the correlation analysis between 20 different metabolites in the serum, 12 different metabolites in the cerebral cortex and indicators of WBC, RBC, HGB, ACTH, CORT, CRH, the thymus index, spleen index and adrenal index. r > 0.5 or r < −0.5 is considered to be correlated with each other, and the results are shown in Figure 10. The colors in the figure represent the correlation values between different metabolites, with red representing a positive correlation and blue representing a negative correlation.
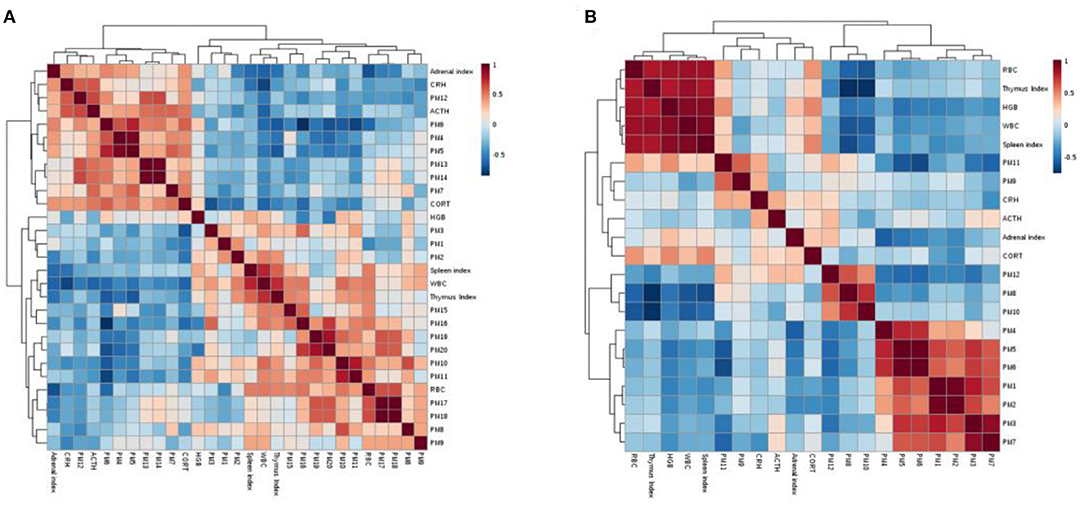
Figure 10. Serum (A) and cerebral cortex (B) correlation analysis between potential biomarkers and biochemical indices.
There is a certain correlation between potential biomarkers, between potential biomarkers and biochemical indicators, and between biochemical indicators. For example, in the correlation analysis of biomarkers and biochemical indicators in the cerebral cortex, WBC, RBC, the thymus index and the spleen index are negatively correlated with valerylcarnitine and tiglycarnitine (r = −0.598; −0.569; −0.552; −0.5597;−0.738; −0.722; −00.635; and −0.569, respectively); these correlation results will help to better reveal the antidepression mechanism of drugs.
Discussion
Depression is a persistent and recurring mental illness, perceived as a multifactorial condition that involves neural, endocrine, and immune pathways (32). The Global Burden of Disease Study 2019 showed that depression has become one of the 10 major diseases contributing to the increased Global Burden of Disease, and the sixth reason affecting the disability adjusted life years (33). Patients undergoing and post radiotherapy with comorbid depression are negatively affected, demonstrating a decline in their of quality of life, an increase in medical costs, the limitation of their treatment course and recovery, and so on (34). These patients have to endure the double pain of cancer and depression, and they urgently need to receive more attentions and effective interventions.
Due to its few adverse effects, multi-mechanisms and multi-targets, traditional Chinese medicine is considered as a valuable reservoir for the development of novel antidepressant and anticancer drugs (35, 36). Paeoniae Radix Alba (PRA) is a traditional Chinese medicine that exhibits the unambiguous effects of nourishing the blood and smoothing the liver, and is frequently used for treating diseases with liver-qi stagnation and blood-deficiency syndrome (LSBD) in China (37). Cancer radiotherapy patients often have depression (38), fatigue (39) and malnutrition (40) symptoms, and according to TCM theories, they can be categorized into LSBD syndrome. Therefore, on the one hand, patients need to regulate their emotions to alleviate depressive symptoms, and they also need to tonify their qi and blood to enhance immunity. We therefore provide an illustrative case to show whether albiflorin (AF) and paeoniflorin (PF), extracted from PRA, had antidepression effects and to explore their mechanisms.
An appropriate animal model is crucial for unraveling the effects and mechanisms of medicine. Unlike usual depression, cancer-related depression has a defect in immune function, so both depression and immunocompromised states need to be considered in the modeling. Therefore, considering the broad spectrum and multiple factors of cancer-related depression and the treatment of cancer, we explored the rat model and successfully established the model by combining irradiation with chronic restraint stress and solitary confinement (28), in a multiple stress reaction, which can simulate the state of cancer-related depression patients to a certain extent, such as decreased peripheral blood count, decreased immunity and a series of depressive symptoms. From the perspective of TCM, the model rats not only present the endocrine-immune system disorders caused by the deficiency of yin blood in the liver meridian, but also show changes in the neuroendocrine system disorders caused by the stagnation of liver qi. This is also consistent with the view that the pathogenesis of depression is involved in the neuroendocrine-immune network (41, 42). In this paper, pharmacological studies showed that depression-like behavioral deficits in model groups and the lowered or increased levels of the peripheral hemogram, HPA axis, and organ index were ameliorated by AF and PF. Metabonomics research found 20 and 12 biomarkers in the serum and cerebral cortex of the model, respectively, and eight potential biomarkers were further screened in ROC analysis. AF and PF can regulate several of these metabolites, involving multiple metabolic pathways, and there was no significant difference in the regulatory effects of AF and PF, which may be due to the relatively similar chemical structure between the two.
There is increasing evidence that the neuroendocrine immune system is closely related to the occurrence of depression (41). The immune system affects the secretion of neurotransmitters in the nervous system and peptide hormones in the endocrine system through cytokines and hormones secreted by immune cells. On the contrary, immune cells can express a variety of neurotransmitters and hormone receptors, neurotransmitters and hormones produced by the neuroendocrine system that are used in immune cells. Through the interaction of these small signal molecules, the neuroendocrine immune network is formed. Peripheral blood and organ indexes are important immune indexes, which are closely related to the function of the immune system (43). Red blood cells, white blood cells and hemoglobin are the most important cell components in the blood, white blood cell count is an easy marker for the estimation of immune function (44). Studies have found that anemia or abnormal erythrocyte parameters such as red blood cell count, hemoglobin in patients with depression and changes in erythrocyte may be associated with the risk of depression (45, 46). While the thymus and spleen are important immune organs in the body, the organ indexes can be used to evaluate the immunity of organisms (47). In this experiment, the red blood cell count, white blood cell count, hemoglobin and the thymus index and the spleen index of rats in the model group were significantly decreased, indicating that the body had abnormal immune system function, which was consistent with the literature reports. AF and PF could significantly increase the thymus index and spleen index, improving the abnormal immune function of the body. With increasing blood–brain barrier permeability, peripheral cytokines can be actively transported into the CNS, subsequently, followed by a reduction in serotonin neurotransmission and the activation of the HPA axis (41). As the hub of the neuroendocrine network, the HPA axis plays an important role in the pathogenesis of depression, with the dysregulation of the HPA axis (48), increasing extracellular glutamate levels and glutamate neurotransmission, and then impacting hippocampal neurogenesis, and through glucocorticoids, the HPA axis is involved in anti-inflammatory, pro-inflammatory and other immune regulatory processes (49). In major depressive disorder, the glucocorticoid receptors' sensitivity decreased, leading to the reduction in the HPA axis' negative feedback mechanism to maintain homeostasis, which leads to the hypersecretion of CRH, ACTH and cortisol (50). In mice with breast cancer concurrent with depression, the content of the HPA axis, such as CORT, ACTH, and CRH, were increased (51). Our study results are consistent with the literature which indicates that the model rats were in a state of depression, and paeoniflorin and albiflorin could play an antidepressant role by regulating the HPA axis. Sucrose preference, open field test and elevated plus-maze are classic indicators used to evaluate animal depression or anxiety (52, 53). In this study, various behavioral traits of model rats were significantly changed, suggesting that the rats were in a state of depression. AF and PF can effectively alleviate this state. Thus, pharmacological studies have shown that AF and PF have antidepression effects, the primary mechanism of which may be related to the regulation of neuroendocrine immune system abnormalities.
Metabonomics exhibits an impressive and ever-increasing coverage of endogenous compounds, focuses on the holistic investigation of multi-parametric metabolite responses of living systems, which is consistent with the holistic view of TCM and the multi-mechanism and multi-target characteristics of TCM and has been widely applied on TCM research (54, 55). We applied the metabonomics method to research the changes in serum and cerebral cortex metabolites, and to explore the action mechanism of drugs. The results showed that multiple metabolites and pathways were involved.
Ether esters have a certain influence on the permeability and fluidity of cell membranes, and are also involved in intercellular signal transduction, antioxidant scavenging, oxidative stress reactions and other processes (56). Abnormal secretion of ether esters is found in mental illness, like Alzheimer's disease (57). Phosphatidylcholine (PC) is the most abundant phospholipid in membrane bilayers. PC has anti-inflammatory effects, and can regulate systemic inflammation through the brain–gut axis (58). Lysophosphatidylcholines (LPC) is produced by the hydrolysis of PC catalyzed by phosphatase A, which also has important roles in membrane permeability and fluidity. Previous studies suggested that chronic unpredictable stress mice and mice treated with antidepressant drugs shown abnormal phospholipid contents (59, 60), which was also confirmed in several clinical studies, and some scholars believe that lipids are an effective target for preventive medicine of psychiatric disorders (61). In our study, PC and LPC as potential biomarkers were involved in ether ester and glycerolphospholipid metabolism, and the content of PC and LysoPC significantly decreased in model rats, while AF and PF can regulate this abnormality, affecting the metabolism of ester and glycerol phospholipid, playing a role in antidepression. However, one point should be noted— during the screening of differences, the contents of the metabolites phosphatidylcholine and lysophosphatidylcholine decreased in the serum of model rats, but increased in the cerebral cortex. This result suggests that the pathogenesis of the disease and the mechanism of action of drugs may have different effects in different biological samples, and also verifies the functional characteristics of multi-component and multi-target of TCM.
Glutamine has a certain effect on the nervous system and immune system (62, 63). One study found that CNS glutamate regulation and the antidepressant effect maybe influenced by inhibiting glutamate release by peripheral cancer cells (64). Clinical studies have also shown that patients with major depressive disorder had abnormal glutamate and glutamine levels (65), and glutamate can cause HPA axis hyperactivity (66). At the same time, the glutamine blockade can overcome tumor immune evasion (67); this is consistent with the results of pharmacological studies. Asparagine is converted from aspartic acid and plays an important role in the pathogenesis of depression (68), asparagine endopeptidase cleaves protein substrates on the C-terminal side of asparagine, and deletion of it can reduce anxiety- and depressive-like behaviors in mice (69). Our study results show that the contents of glutamine and asparagine in the model group were decreased, indicating that the model rats were in a certain state of depression, with abnormal metabolism of alanine, aspartic acid and glutamic acid, and AF and PF could increase their contents and play a therapeutic role.
Kynurenin is an important substance in tryptophan metabolism, and a reduction in its content will lead to abnormal tryptophan metabolism. More and more studies have found that tryptophan-kynurenin metabolism plays an important role in depression, which is manifested as the abnormal secretion of tryptophan-kynurenin (70, 71). Tryptophan metabolism also closely associated with inflammation, TNF-α and other inflammatory factors, can activate 2,3-dioxygenase oxygen (IDO) and tryptophan enzyme (TDO) through inflammatory signaling pathways, increase tryptophan decomposition and reduce its peripheral content. At the same time, this inflammatory factor can cause the brain tryptophan metabolism to increase when its content is lower. Finally, it can lead to abnormal urinary acid and its related metabolic pathways (72). Tryptophan is also the precursor of serotonin (5-HT). The abnormal metabolism of tryptophan causes the abnormal metabolism of 5-HT neurotransmitters, manifested as insufficient synthesis and transmission disorder, and is closely related to the pathogenesis of depression (73), which is consistent with the previous research results of our research group (16). In this experiment, the content of kynurenine in the model rats decreased, indicating that the rats showed depression-like behavior and abnormal tryptophan metabolism. AF and PF could affect tryptophan metabolism by increasing the content of kynurenine.
The potential biomarkers Glutamine, Citrulline and 4-hydroxyproline are involved in arginine and proline metabolism. Citrulline is phosphorylated by ornithine and carbamyl, and is also an arginine metabolite, which is one of the core reactions of the urea cycle, and participates in the NO metabolic pathway, which is under the catalysis of endothelial nitric oxide synthase (eNOS), Meanwhile, arginine combines with oxygen to produce NO, and L-citrulline is the end product of NO production (74). The related literatures has reported that the L-arginine and L-citrulline concentrations were significantly lower in severe depression patients than in healthy controls, which is a possible explanation for the decrease in NO metabolites in depression patients (74). At the same time, in stress-loaded senescence-accelerated mice, L-arginine reduces oxidative damage and enhances mitochondrial functions in the brain and exerts excellent anti-stress effects (75), and abnormal metabolism of arginine and proline metabolism in rats and patients with depression (76, 77). In our research, the contents of glutamine, citrulline and 4-hydroxyproline in the serum of model rats were significantly decreased, which was consistent with the literature reports. AF and PF increased their contents, and then affected the metabolism of arginine and proline.
Carnitine is an amino acid derivative, synthesized from the essential amino acids methionine and lysine. It plays an important role in the oxidative stress, fat metabolism and energy metabolism of the body, and is involved in the synthesis of fat, the transportation and oxidation of fatty acids, and the utilization of ketone bodies (78). The increase in carnitine content can reduce the content of acetyl-CoA and fatty acids in the body; fatty acids are the precursors of active substances such as prostaglandins, and participate in the process of inflammation and immunity in the body. Carnitine also can prevent the programmed cell death of immune cells (79). The decrease in their content will affect the process of immune regulation in the body. As the short-chain ester of carnitine, acetyl-L-carnitine (ALC) has received more attention in antidepression, including mediating the PI3K/AKT/BDNF/VGF signaling pathway (80), and brain energy metabolism (81). ALC is considered a promising antidepressant, especially for older patients (82, 83). Under the action of carnitine acyltransferase in vivo, L-carnitine uses acylcarnitine as a carrier to deliver short-chain acyl groups in the mitochondria to the outer mitochondrial cell membrane, and transfer the long-chain fatty acids outside the membrane to the membrane, so that they can perform β-oxidation in the mitochondrial matrix to produce energy (84, 85), and maintain the balance of energy metabolism in the body. The decrease in carnitine content will increase the concentration of acetyl-CoA in the mitochondria. Meanwhile, the fatty acids outside the mitochondrial cell membrane cannot enter the cell membrane, which affects the Krebs cycle. In this experiment, the contents of L-octylcarnitine and other carnitines in the serum and L-carnitine, butyrylcarnitine and other carnitines in the cerebral cortex of model rats increased, suggesting that the bodies of model rats demonstrated β-abnormal oxidative function and an imbalance of energy metabolism, which are consistent with the literature reports, indicating that the model rats are in a certain state of depression. AF and PF can reduce the content of carnitine metabolites, regulate the body's energy metabolism, and exert antidepressive effects.
Glycerol phospholipids can be decomposed to form hemolytic lecithin and arachidonic acid under the action of phospholipase, and the change in glycerol phospholipid content can lead to the change in arachidonic acid content in vivo (81). Arachidonic acid is a kind of unsaturated fatty acid, that can be in the ring oxidase, and it can undergo lipid oxygenation under the action of enzymes to a variety of important bioactive substances such as leukotrienes and prostaglandins, which help to increase vascular elasticity, reduce blood viscosity, and regulate blood's physiological activity, such as in the body's inflammatory response and immune response processes (86, 87). Arachidonic acid plays an important role in brain diseases and depression—derivatives of arachidonic acid can increase dopamine release by activating the cannabinoid type-1 receptor (88, 89). The pharmacological research results of this study show that the metabolism of relevant indexes of peripheral hemogram is abnormal, and the function of blood cells is reduced in model rats. The metabonomic research results show that the content of phosphatidylcholine in the brain tissue of model rats is increased, indicating that there is an abnormal metabolism of arachidonic acid in the body, which impairs the relevant functions of the immune system. AF and PF can play an antidepressant role by reducing the content of phosphatidylcholine and then affecting the metabolism of arachidonic acid.
Conclusions
The present studies suggest that AF and PF caused a significant improvement in the pharmacodynamic index by modulating the neuroendocrine-immune network and regulating abnormal metabolic pathways (Figure 11) and demonstrate that the metabolites may be useful as potential markers for diagnosing and monitoring cancer-related depression. These findings may supply beneficial hints for the treatment of cancer-related depression, and thus deserve further clinical investigations.
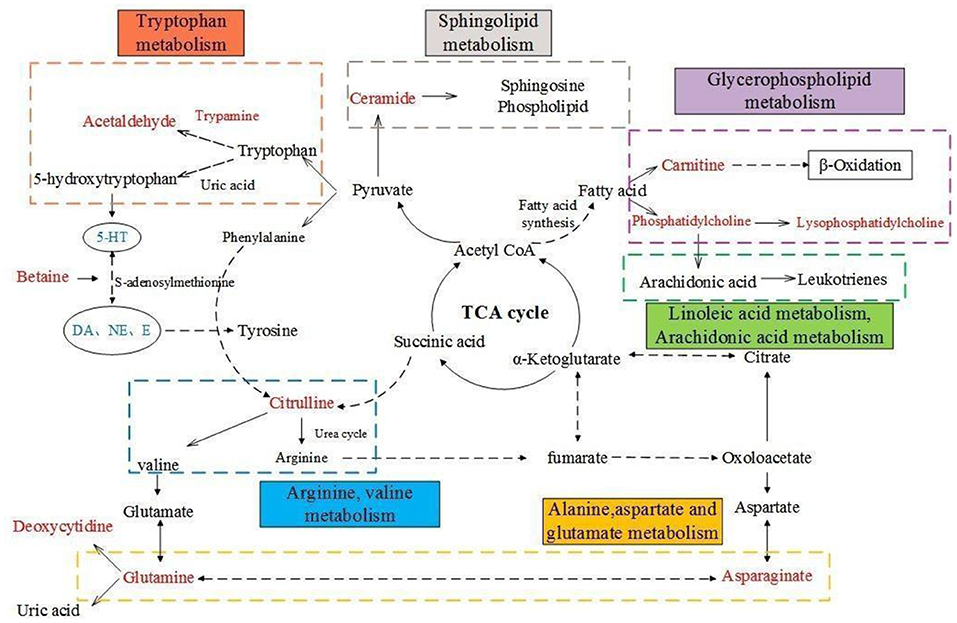
Figure 11. Overall metabolic pathway map of model rats. Red characters are metabolites for screening, blue are pharmacodynamic index; Solid line is one-step reaction, dotted line is multi-step reaction.
Data Availability Statement
The original contributions presented in the study are included in the article/supplementary material, further inquiries can be directed to the corresponding authors.
Ethics Statement
The animal study was reviewed and approved by Experimental Animal Ethics Committee of Beijing University of Chinese Medicine.
Author Contributions
Experiments designed and supervised: LW and JZ. Experiments conducted: DZ, NY, and CW. Resources and formal analysis: CH. Original manuscript wrote: DZ. Manuscript reviewed and edited: YZ, WF, and JZ. All authors have read and agreed to the published version of the manuscript.
Funding
This work was supported by the National Natural Science Foundation of China (81473370) and National Key R&D Program of China (2018YFC1706800).
Conflict of Interest
The authors declare that the research was conducted in the absence of any commercial or financial relationships that could be construed as a potential conflictof interest.
Publisher's Note
All claims expressed in this article are solely those of the authors and do not necessarily represent those of their affiliated organizations, or those of the publisher, the editors and the reviewers. Any product that may be evaluated in this article, or claim that may be made by its manufacturer, is not guaranteed or endorsed by the publisher.
References
1. Sung H, Ferlay J, Siegel RL, Laversanne M, Soerjomataram I, Jemal A, et al. Global cancer statistics 2020: GLOBOCAN estimates of incidence and mortality worldwide for 36 cancers in 185 countries. CA Cancer J Clin. (2021) 71:209–49. doi: 10.3322/caac.21660
2. Wang YH, Li JQ, Shi JF, Que JY, Liu JJ, Lappin JM, et al. Depression and anxiety in relation to cancer incidence and mortality: a systematic review and meta-analysis of cohort studies. Mol Psychiatry. (2020) 25:1487–99. doi: 10.1038/s41380-019-0595-x
3. Mohile SG, Dale W, Somerfield MR, Schonberg MA, Boyd CM, Burhenn PS, et al. Practical assessment and management of vulnerabilities in older patients receiving chemotherapy: ASCO Guideline for Geriatric Oncology. J Clin Oncol. (2018) 36:2326–47. doi: 10.1200/JCO.2018.78.8687
4. Walker J, Hansen CH, Martin P, Symeonides S, Ramessur R, Murray G, et al. Prevalence, associations, and adequacy of treatment of major depression in patients with cancer: a cross-sectional analysis of routinely collected clinical data. Lancet Psychiatry. (2014) 1:343–50. doi: 10.1016/S2215-0366(14)70313-X
5. Bortolato B, Hyphantis TN, Valpione S, Perini G, Maes M, Morris G, et al. Depression in cancer: the many biobehavioral pathways driving tumor progression. Cancer Treat Rev. (2017) 52:58–70. doi: 10.1016/j.ctrv.2016.11.004
6. Mausbach BT, Decastro G, Schwab RB, Tiamson-Kassab M, Irwin SA. Healthcare use and costs in adult cancer patients with anxiety and depression. Depress Anxiety. (2020) 37:908–15. doi: 10.1002/da.23059
7. Rogiers A, Leys C, De Cremer J, Awada G, Schembri A, Theuns P, et al. Health-related quality of life, emotional burden, and neurocognitive function in the first generation of metastatic melanoma survivors treated with pembrolizumab: a longitudinal pilot study. Support Care Cancer. (2020) 28:3267–78. doi: 10.1007/s00520-019-05168-3
8. Erim DO, Bensen JT, Mohler JL, Fontham ETH, Song LX, Farnan L, et al. Patterns and predictors of self-reported clinical diagnosis and treatment for depression in prostate cancer survivors. Cancer Med. (2019) 8:3648–58. doi: 10.1002/cam4.2239
9. Po WW, Thein W, Khin PP, Khing TM, Han KWW, Park CH, et al. Fluoxetine simultaneously induces both apoptosis and autophagy in human gastric adenocarcinoma cells. Biomol Ther. (2020) 28:202–10. doi: 10.4062/biomolther.2019.103
10. Panjwani AA, Li ME. Recent trends in the management of depression in persons with cancer. Curr Opin Psychiatr. (2021) 34:448–59. doi: 10.1097/yco.0000000000000727
11. Ye XW, Deng YL, Xia LT, Ren HM, Zhang JL. Uncovering the mechanism of the effects of Paeoniae Radix Alba on iron-deficiency anaemia through a network pharmacology-based strategy. BMC Complement Med Ther. (2020) 20:1–16. doi: 10.1186/s12906-020-02925-4
12. Yan BJ, Shen ML, Fang JY, Wei DN, Qin LP. Advancement in the chemical analysis of Paeoniae Radix (Shaoyao). J Pharm Biomed Anal. (2018) 160:276–88. doi: 10.1016/j.jpba.2018.08.009
13. Wu ZL, Yang LN, He L, Wang LA, Peng LS. Systematic elucidation of the potential mechanisms of core chinese materia medicas in treating liver cancer based on network pharmacology. Evid Based Complement Alternat Med. (2020) 2020:1–13. doi: 10.1155/2020/4763675
14. Xiang YJ, Zhang Q, Wei SJ, Huang C, Li ZS, Gao YX. Paeoniflorin: a monoterpene glycoside from plants of Paeoniaceae family with diverse anticancer activities. J Pharm Pharmacol. (2020) 72:483–95. doi: 10.1111/jphp.13204
15. Zhang ZH, Xie DD, Xu S, Xia MZ, Zhang ZQ, Geng H, et al. Total glucosides of paeony inhibits lipopolysaccharide-induced proliferation, migration and invasion in androgen insensitive prostate cancer cells. PLoS ONE. (2017) 12:1–16. doi: 10.1371/journal.pone.0182584
16. Zhu YL, Wang LY, Zhao DP, Wang CL, Zhang R, Fei WT, et al. Antidepressant-like effects of albiflorin involved the NO signaling pathway in rats model of chronic restraint stress. Chin J Nat Med. (2020) 18:872–80. doi: 10.1016/S1875-5364(20)60030-9
17. Zhu YL, Wang LY, Yang ZH, Wang JX, Li W, Zhou JY, et al. Hematopoietic effects of paeoniflorin and albiflorin on radiotherapy-induced myelosuppression mice. Evid Based Complement Alternat Med. (2016) 2016:1–8. doi: 10.1155/2016/5789381
18. Zhu YL, Wang LY, Wang JX, Wang C, Wang CL, Zhao DP, et al. Protective effects of paeoniflorin and albiflorin on chemotherapy-induced myelosuppression in mice. Chin J Nat Med. (2016) 14:599–606. doi: 10.1016/S1875-5364(16)30070-X
19. Li YC, Zheng XX, Xia SZ, Li Y, Deng HH, Wang X, et al. Paeoniflorin ameliorates depressive-like behavior in prenatally stressed offspring by restoring the HPA axis- and glucocorticoid receptor- associated dysfunction. J Affect Disord. (2020) 274:471–81. doi: 10.1016/j.jad.2020.05.078
20. Yu JB, Zhao ZX, Peng R, Pan LB, Fug J, Ma SR, et al. Gut microbiota-based pharmacokinetics and the antidepressant mechanism of paeoniflorin. Front Pharmacol. (2019) 10:1–12. doi: 10.3389/fphar.2019.00268
21. Wang YL, Wang JX, Hu XX, Chen L, Qiu ZK, Zhao N, et al. Antidepressant-like effects of albiflorin extracted from Radix paeoniae Alba. J Ethnopharmacol. (2016) 179:9–15. doi: 10.1016/j.jep.2015.12.029
22. Liu P, Chen JJ, Ma S, Zhang JJ, Zhou JY. Albiflorin attenuates mood disorders under neuropathic pain state by suppressing the hippocampal NLRP3 inflammasome activation during chronic constriction injury. Int J Neuropsychopharmacol. (2021) 24:64–76. doi: 10.1093/ijnp/pyaa076
23. Liu XJ, Lv M, Wang YZ, Zhao D, Zhao SJ, Li SY, et al. Deciphering the compatibility rules of traditional Chinese medicine prescriptions based on NMR metabolomics: a case study of Xiaoyaosan. J Ethnopharmacol. (2020) 254:1–11. doi: 10.1016/j.jep.2020.112726
24. Chen CC, Yin QC, Tian JS, Gao XX, Qin XM, Du GH, et al. Studies on the potential link between antidepressant effect of Xiaoyao San and its pharmacological activity of hepatoprotection based on multi-platform metabolomics. J Ethnopharmacol. (2020) 249:1–15. doi: 10.1016/j.jep.2019.112432
25. Shen CK, Cao KR, Cui SN, Cui YF, Mo HX, Wen WH, et al. SiNiSan ameliorates depression-like behavior in rats by enhancing synaptic plasticity via the CaSR-PKC-ERK signaling pathway. Biomed Pharmacother. (2020) 124:1–14. doi: 10.1016/j.biopha.2019.109787
26. Chang X, Jia HM, Zhou C, Zhang HW, Yu M, Yang JS, et al. Role of Bai-Shao towards the antidepressant effect of Chaihu-Shu-Gan-San using metabonomics integrated with chemical fingerprinting. J Chromatogr B Analyt Technol Biomed Life Sci. (2015) 1006:16–29. doi: 10.1016/j.jchromb.2015.10.007
27. Wang P, Gao XX, Liang ML, Fang Y, Jia JP, Tian JS, et al. Dose-effect/toxicity of bupleuri radix on chronic unpredictable mild stress and normal rats based on liver metabolomics. Front Pharmacol. (2021) 12:1–23. doi: 10.3389/fphar.2021.627451
28. Zhang JJ, Li YX, Wang JX, Li W, Wang C, Qu SS, et al. Establishment of rat model of blood deficiency and liver depression syndrome induced by the method of chronic restraint stress combined with radiation. China J Tradit Chin Med Pharm. (2014) 29:2176–9.
29. Wang J, Tian RC, Shan YH, Li JJ, Gao HX, Xie CJ, et al. Metabolomics study of the metabolic changes in hepatoblastoma cells in response to NTCP/SLC10A1 overexpression. Int J Biochem Cell Biol. (2020) 125:1–9. doi: 10.1016/j.biocel.2020.105773
30. Bajaj JS, Fan S, Thacker LR, Fagan A, Gavis E, White MB, et al. Serum and urinary metabolomics and outcomes in cirrhosis. PLoS ONE. (2019) 14:1–17. doi: 10.1371/journal.pone.0223061
31. Chong J, Soufan O, Li C, Caraus I, Li SZ, Bourque G, et al. MetaboAnalyst 4.0: towards more transparent and integrative metabolomics analysis. Nucleic Acids Research. (2018) 46(W1):W486–94; 1–9. doi: 10.1093/nar/gky310
32. Makris AP, Karianaki M, Tsamis KI, Paschou SA. The role of the gut-brain axis in depression: endocrine, neural, and immune pathways. Hormones. (2021) 20:1–12. doi: 10.1007/s42000-020-00236-4
33. Vos T. Global burden of 369 diseases and injuries in 204 countries and territories, 1990-2019: a systematic analysis for the Global Burden of Disease Study 2019. Lancet. (2020) 396:1204–22.
34. Walker J, Waters RA, Murray G, Swanson H, Hibberd CJ, Rush RW, et al. Better off dead: Suicidal thoughts in cancer patients. J Clin Oncol. (2008) 26:4725–30.
35. Li C, Huang JY, Cheng YC, Zhang YW. Traditional Chinese medicine in depression treatment: from molecules to systems. Front Pharmacol. (2020) 11:1–11. doi: 10.3389/fphar.2020.00586
36. Yan ZK, Lai ZJ, Lin JM. Anticancer properties of traditional Chinese medicine. Comb Chem High Throughput Screen. (2017) 20:423–9. doi: 10.2174/1386207320666170116141818
37. Zhao DP, Zhang JJ, He C, Wang S, Tang RY, Hou Y, et al. Study on mechanism of nourishing blood and smoothing liver effects of Paeoniae Radix Alba based on metabolomics information. Chinese Tradit Herbal Drugs. (2017) 48:3412–8.
38. Williams PA, Cao S, Yang DY, Jennelle RL. Patient-reported outcomes of the relative severity of side effects from cancer radiotherapy. Support Care Cancer. (2020) 28:309–16. doi: 10.1007/s00520-019-04820-2
39. Forster T, Jakel C, Akbaba S, Krug D, Krempien R, Uhl M, et al. Fatigue following radiotherapy of low-risk early breast cancer - a randomized controlled trial of intraoperative electron radiotherapy versus standard hypofractionated whole-breast radiotherapy: the COSMOPOLITAN trial (NCT03838419). Radiat Oncol. (2020) 15:1–10. doi: 10.1186/s13014-020-01581-9
40. Zhang ZH, Wan Z, Zhu Y, Wan HW. Predictive validity of the GLIM criteria in treatment outcomes in cancer patients with radiotherapy. Clin Nutr. (2022) 41:855–61. doi: 10.1016/j.clnu.2022.02.011
41. Li C, Huang BS, Zhang YW. Chinese herbal medicine for the treatment of depression: effects on the neuroendocrine-immune network. Pharmaceuticals. (2021) 14:1–28. doi: 10.3390/ph14010065
42. Lan XY, Yu H, Chen QJ, Zhai S, Zhang CF, Li F, et al. Effect of liquiritin on neuroendocrine-immune network in menopausal rat model. Phytother Res. (2020) 34:2665–74. doi: 10.1002/ptr.6696
43. Deng XL, Fu YJ, Luo S, Luo X, Wang Q, Hu MH, et al. Polysaccharide from radix codonopsis has beneficial effects on the maintenance of t-cell balance in mice. Biomed Pharmacother. (2019) 112:1–7. doi: 10.1016/j.biopha.2019.108682
44. Kohler-Forsberg O, Sylvia LG, Bowden CL, Calabrese JR, Thase ME, Shelton RC, et al. Correlation between white blood cell count and mood-stabilising treatment response in two bipolar disorder trials. Acta Neuropsychiatr. (2019) 31:230–4. doi: 10.1017/neu.2019.19
45. Wysokinski A, Szczepocka E. Red blood cells parameters in patients with acute schizophrenia, unipolar depression and bipolar disorder. Psychiatr Danub. (2018) 30:323–30. doi: 10.24869/psyd.2018.323
46. Oh DJ, Han JW, Bae JB, Kim HS, Suh SW, Byun S, et al. Erythrocyte characteristics and the risk of depression in late life: a population-based prospective study. J Am Med Dir Assoc. (2020) 21:1677–83. doi: 10.1016/j.jamda.2020.05.012
47. Li WF, Hu XY, Wang SP, Jiao ZR, Sun TY, Liu TQ, et al. Characterization and anti-tumor bioactivity of astragalus polysaccharides by immunomodulation. Int J Biol Macromol. (2020) 145:985–97. doi: 10.1016/j.ijbiomac.2019.09.189
48. Keller J, Gomez R, Williams G, Lembke A, Lazzeroni L, Urphy GM, et al. HPA axis in major depression: cortisol, clinical symptomatology and genetic variation predict cognition. Mol Psychiatry. (2017) 22:527–36. doi: 10.1038/mp.2016.120
49. Troubat R, Barone P, Leman S, Desmidt T, Cressant A, Atanasova B, et al. Neuroinflammation and depression: a review. Eur J Neurosci. (2021) 53:151–71. doi: 10.1111/ejn.14720
50. Menke A. Is the HPA axis as target for depression outdated, or is there a new hope? Front Psychiatry. (2019) 10:1–8. doi: 10.3389/fpsyt.2019.00101
51. Meng P, Han YS, Yang Q, Yang H, Zhu Q, Lin XY, et al. Xiaoyao Kangai Jieyu Fang, a Chinese herbal formulation, ameliorates cancer-related depression concurrent with breast cancer in mice via promoting hippocampal synaptic plasticity. Evid Based Complement Alternat Med. (2018) 2018:1–12. doi: 10.1155/2018/3967642
52. Zhou XD, Shi DD, Zhang ZJ. Antidepressant and anxiolytic effects of the proprietary Chinese medicine Shexiang Baoxin pill in mice with chronic unpredictable mild stress. J Food Drug Anal. (2019) 27:221–30. doi: 10.1016/j.jfda.2018.08.001
53. Gall Z, Farkas S, Albert A, Ferencz E, Vancea S, Urkon M, et al. Effects of chronic cannabidiol treatment in the rat chronic unpredictable mild stress model of depression. Biomolecules. (2020) 10:1–16. doi: 10.3390/biom10050801
54. Wang YQ, Li SJ, Man YH, Zhuang G. Serum metabonomics coupled with HPLC-LTQ/orbitrap MS and multivariate data analysis on the ameliorative effects of Bidens bipinnata L. in hyperlipidemic rats. J Ethnopharmacol. (2020) 262:1–10. 10.1016/j.jep.2020.113196. doi: 10.1016/j.jep.2020.113196
55. Lin L, Zhang SB, Lin YX, Liu W, Zou BR, Cai Y, et al. Untargeted metabolomics analysis on Cicer arietinium L.-induced amelioration in T2D rats by UPLC-Q-TOF-MS/MS. J Ethnopharmacol. (2020) 261:1–11. doi: 10.1016/j.jep.2020.113013
56. Drechsler R, Chen SW, Dancy BCR, Mehrabkhani L, Olsen CP. HPLC-based mass spectrometry characterizes the phospholipid alterations in ether-linked lipid deficiency models following oxidative stress. PLoS ONE. (2016) 11:1–22. doi: 10.1371/journal.pone.0167229
57. Sakr F, Dyrba M, Brauer A, Teipel S, Alzheimer's Disease Neuroimaging Initiative. Association of lipidomics signatures in blood with clinical progression in preclinical and prodromal Alzheimer's disease. J Alzheimers Dis. (2022) 85:1115–27. doi: 10.3233/JAD-201504
58. Tan W, Zhang QJ, Dong ZJ, Yan YB, Fu YK, Liu XB, et al. Phosphatidylcholine ameliorates LPS-induced systemic inflammation and cognitive impairments via mediating the gut-brain axis balance. J Agric Food Chem. (2020) 68:14884–95. doi: 10.1021/acs.jafc.0c06383
59. Faria R, Santana MM, Aveleira CA, Simoes C, Maciel E, Melo T, et al. Alterations in phospholipidomic profile in the brain of mouse model of depression induced by chronic unpredictable stress. Neuroscience. (2014) 273:1–11. doi: 10.1016/j.neuroscience.2014.04.042
60. Xue SS, Zhou CH, Xue F, Liu L, Cai YH, Luo JF, et al. The impact of repetitive transcranial magnetic stimulation and fluoxetine on the brain lipidome in a rat model of chronic unpredictable stress. Prog Neuropsychopharmacol Biol Psychiatry. (2020) 102:1–12. doi: 10.1016/j.pnpbp.2020.109946
61. Schneider M, Levant B, Reichel M, Gulbins E, Kornhuber J, Muller CP. Lipids in psychiatric disorders and preventive medicine. Neurosci Biobehav Rev. (2017) 76:336–62. doi: 10.1016/j.neubiorev.2016.06.002
62. Cruzat V, Rogero MM, Keane KN, Curi R, Newsholme P. Glutamine: metabolism and immune function, supplementation and clinical translation. Nutrients. (2018) 10:1–31. doi: 10.3390/nu10111564
63. Deters BJ, Saleem M. The role of glutamine in supporting gut health and neuropsychiatric factors. Food Sci Human Wellness. (2021) 10:149–54. doi: 10.1016/j.fshw.2021.02.003
64. Nashed MG, Ungard RG, Young K, Zacal NJ, Seidlitz EP, Fazzari J, et al. Behavioural effects of using sulfasalazine to inhibit glutamate released by cancer cells: a novel target for cancer-induced depression. Sci Rep. (2017) 7:1–11. doi: 10.1038/srep41382
65. Shirayama Y, Takahashi M, Osone F, Hara A, Okubo T. Myo-inositol, glutamate, and glutamine in the prefrontal cortex, hippocampus, and amygdala in major depression. Biol Psychiatry Cogn Neurosci Neuroimaging. (2017) 2:196–204. doi: 10.1016/j.bpsc.2016.11.006
66. Evanson NK, Herman JP. Metabotropic glutamate receptor-mediated signaling dampens the HPA axis response to restraint stress. Physiol Behav. (2015) 150:2–7. doi: 10.1016/j.physbeh.2015.02.027
67. Leone RD, Zhao L, Englert JM, Sun IM, Oh MH, Sun IH, et al. Glutamine blockade induces divergent metabolic programs to overcome tumor immune evasion. Science. (2019) 366:1013–21. doi: 10.1126/science.aav2588
68. Baranyi A, Amouzadeh-Ghadikolai O, von Lewinski D, Breitenecker RJ, Rothenhausler HB, Robier C, et al. Revisiting the tryptophan-serotonin deficiency and the inflammatory hypotheses of major depression in a biopsychosocial approach. PeerJ. (2017) 5:1–24. doi: 10.7717/peerj.3968
69. Gao J, Li K, Du LF, Yin HQ, Tan XY, Yang Z. Deletion of asparagine endopeptidase reduces anxiety- and depressive-like behaviors and improves abilities of spatial cognition in mice. Brain Res Bull. (2018) 142:147–55. doi: 10.1016/j.brainresbull.2018.07.010
70. Wang QS, Yan K, Li KD, Gao LN, Wang X, Liu HB, et al. Targeting hippocampal phospholipid and tryptophan metabolism for antidepressant-like effects of albiflorin. Phytomedicine. (2021) 92:1–13. doi: 10.1016/j.phymed.2021.153735
71. Milaneschi Y, Allers KA, Beekman ATF, Giltay EJ, Keller S, Schoevers RA, et al. The association between plasma tryptophan catabolites and depression: the role of symptom profiles and inflammation. Brain Behav Immun. (2021) 97:167–75. doi: 10.1016/j.bbi.2021.07.007
72. Chen LM, Bao CH, Wu Y, Liang SH, Wang D, Wu LY, et al. Tryptophan-kynurenine metabolism: a link between the gut and brain for depression in inflammatory bowel disease. J Neuroinflammation. (2021) 18:1–13. doi: 10.1186/s12974-021-02175-2
73. Zheng RY, Xiong WF, He J, Wang X, Wang RR, Hao Y. Effects of Fuhe decoction on behaviors and monoamine neurotransmitters in different brain regions of CUMS combined with social isolation depression model rats. J Tradit Chin Med Sci. (2020) 7:10. doi: 10.1016/j.jtcms.2020.07.009
74. Hess S, Baker G, Gyenes G, Tsuyuki R, Newman S, Le Melledo JM. Decreased serum L-arginine and L-citrulline levels in major depression. Psychopharmacology. (2017) 234:3241–7. doi: 10.1007/s00213-017-4712-8
75. Pervin M, Unno K, Konishi T, Nakamura Y. L-arginine exerts excellent anti-stress effects on stress-induced shortened lifespan, cognitive decline and depression. Int J Mol Sci. (2021) 22:1–14. doi: 10.3390/ijms22020508
76. Cui YF, Cao KR, Lin HY, Cui SN, Shen CK, Wen WH, et al. Early-life stress induces depression-like behavior and synaptic-plasticity changes in a maternal separation rat model: gender difference and metabolomics study. Front Pharmacol. (2020) 11:1–13. doi: 10.3389/fphar.2020.00102
77. Du Y, Wei JX, Zhang ZJ, Yang X, Wang M, Wang Y, et al. Plasma metabolomics profiling of metabolic pathways affected by major depressive disorder. Front Psychiatry. (2021) 12:1–9. doi: 10.3389/fpsyt.2021.644555
78. Longo N, Frigeni M, Pasquali M. Carnitine transport and fatty acid oxidation. Biochim Biophys Acta Mol Cell Res. (2016) 1863:2422–35. doi: 10.1016/j.bbamcr.2016.01.023
79. Moon JS, Nakahira K, Chung KP, DeNicola GM, Koo MJ, Pabon MA, et al. NOX4-dependent fatty acid oxidation promotes NLRP3 inflammasome activation in macrophages. Nat Med. (2016) 22:1002–12. doi: 10.1038/nm.4153
80. Wang W, Lu Y, Xue Z, Li C, Wang C, Zhao X, et al. rapid-acting antidepressant-like effects of acetyl-L-carnitine mediated by PI3K/AKT/BDNF/VGF signaling pathway in mice. Neuroscience. (2015) 285:281–91. doi: 10.1016/j.neuroscience.2014.11.025
81. Ferreira GC, McKenna MC. L-Carnitine and acetyl-l-carnitine roles and neuroprotection in developing brain. Neurochem Res. (2017) 42:1661–75. doi: 10.1007/s11064-017-2288-7
82. Sergi G, Pizzato S, Piovesan F, Trevisan C, Veronese N, Manzato E. Effects of acetyl-L-carnitine in diabetic neuropathy and other geriatric disorders. Aging Clin Exp Res. (2018) 30:133–8. doi: 10.1007/s40520-017-0770-3
83. Suzuki H, Hibino H, Inoue Y, Mikami K. The benefits of the concomitant use of antidepressants and acetyl-L-carnitine in the treatment of moderate depression. Asian J Psychiatr. (2019) 41:84–5. doi: 10.1016/j.ajp.2017.10.023
84. Morkholt AS, Wiborg O, Nieland JGK, Nielsen S, Nieland JD. Blocking of carnitine palmitoyl transferase 1 potently reduces stress-induced depression in rat highlighting a pivotal role of lipid metabolism. Sci Rep. (2017) 7:1–9. doi: 10.1038/s41598-017-02343-6
85. Mota SI, Pita I, Aguas R, Tagorti S, Virmani A, Pereira FC, et al. Mechanistic perspectives on differential mitochondrial-based neuroprotective effects of several carnitine forms in Alzheimer's disease in vitro model. Arch Toxicol. (2021) 95:2769–84. doi: 10.1007/s00204-021-03104-1
86. Tallima H, El Ridi R. Arachidonic acid: physiological roles and potential health benefits - a review. J Adv Res. (2018) 11:33–41. doi: 10.1016/j.jare.2017.11.004
87. Regulska M, Szuster-Gluszczak M, Trojan E, Leskiewicz M, Basta-Kaim A. The emerging role of the double-edged impact of arachidonic acid-derived eicosanoids in the neuroinflammatory background of depression. Curr Neuropharmacol. (2021) 19:278–93. doi: 10.2174/1570159X18666200807144530
88. Gantz SC, Bean BP. Cell-autonomous excitation of midbrain dopamine neurons by endocannabinoid-dependent lipid signaling. Neuron. (2017) 93:1375–87. doi: 10.1016/j.neuron.2017.02.025
Keywords: albiflorin, paeoniflorin, depression, irradiation, metabonomics, mechanisms
Citation: Zhao D, Zhang J, Zhu Y, He C, Fei W, Yue N, Wang C and Wang L (2022) Study of Antidepressant-Like Effects of Albiflorin and Paeoniflorin Through Metabolomics From the Perspective of Cancer-Related Depression. Front. Neurol. 13:828612. doi: 10.3389/fneur.2022.828612
Received: 25 December 2021; Accepted: 13 June 2022;
Published: 08 July 2022.
Edited by:
Magda Tsolaki, Aristotle University of Thessaloniki, GreeceReviewed by:
Clara Grosso, LAQV Network of Chemistry and Technology, PortugalKefeng Li, University of California, San Diego, United States
Chengyuan Lin, Hong Kong Baptist University, Hong Kong SAR, China
Mohsen Norouzinia, Shahid Beheshti University of Medical Sciences, Iran
Mostafa Meshref, Al-Azhar University, Egypt
Li-Na Gao, Jining Medical University, China
Copyright © 2022 Zhao, Zhang, Zhu, He, Fei, Yue, Wang and Wang. This is an open-access article distributed under the terms of the Creative Commons Attribution License (CC BY). The use, distribution or reproduction in other forums is permitted, provided the original author(s) and the copyright owner(s) are credited and that the original publication in this journal is cited, in accordance with accepted academic practice. No use, distribution or reproduction is permitted which does not comply with these terms.
*Correspondence: Linyuan Wang, d2FuZ2x5JiN4MDAwNDA7YnVjbS5lZHUuY24=; Jianjun Zhang, emhhbmdqaWFuanVuJiN4MDAwNDA7YnVjbS5lZHUuY24=