- 1Synthetic, Perceptive, Emotive and Cognitive Systems Laboratory, Institute for Bioengineering in Catalonia, Barcelona, Spain
- 2Division of Biokinesiology and Physical Therapy, University of Southern California, Los Angeles, CA, United States
Large doses of movement practice have been shown to restore upper extremities' motor function in a significant subset of individuals post-stroke. However, such large doses are both difficult to implement in the clinic and highly inefficient. In addition, an important reduction in upper extremity function and use is commonly seen following rehabilitation-induced gains, resulting in “rehabilitation in vain”. For those with mild to moderate sensorimotor impairment, the limited spontaneous use of the more affected limb during activities of daily living has been previously proposed to cause a decline of motor function, initiating a vicious cycle of recovery, in which non-use and poor performance reinforce each other. Here, we review computational, experimental, and clinical studies that support the view that if arm use is raised above an effective threshold, one enters a virtuous cycle in which arm use and function can reinforce each other via self-practice in the wild. If not, one enters a vicious cycle of declining arm use and function. In turn, and in line with best practice therapy recommendations, this virtuous/vicious cycle model advocates for a paradigm shift in neurorehabilitation whereby rehabilitation be embedded in activities of daily living such that self-practice with the aid of wearable technology that reminds and motivates can enhance paretic limb use of those who possess adequate residual sensorimotor capacity. Altogether, this model points to a user-centered approach to recovery post-stroke that is tailored to the participant's level of arm use and designed to motivate and engage in self-practice through progressive success in accomplishing meaningful activities in the wild.
Introduction
Current rehabilitation of upper extremities (UEs) in clinical settings often fails to improve the quality of life of people who have had a stroke for two main reasons. First, whereas principle-based (1) rehabilitation focused on improving UE function or on reducing impairment requires very large doses of intensive movement practice (2–5), such doses are far from being the norm in clinical settings, at least in the US (6) and in Europe (7). Second, rehabilitation is often “in vain”, as an important reduction in function and use is commonly seen subsequent to rehabilitation-induced gains. For example, it has been shown that patients experience functional deterioration during the 4 years following hospital discharge, which puts them back to where they were just 2 months post-stroke (8). Similarly, in a re-analysis of UE use of the immediate treatment group of the EXCITE trial, one-quarter of the participants showed a marked decrease in use in the 2 years following treatment (9).
Here, we propose for mild to moderately impaired stroke survivors that increasing daily use of the more affected UE following motor therapy can solve the two above problems: if goal-directed UE movements in daily activities are seen as a single practice movement, such “self-practice” could potentially provide the large dose of movements and sensorimotor feedback needed to improve performance. The improvement in performance could then counteract the deteriorations in use by engaging the patients in a virtuous cycle of recovery, in which high levels of use and function reinforce each other (10) (Figure 1A). In contrast, a low level of use below a threshold may initiate a vicious cycle in which non-use and poor performance reinforce each other (Figure 1B) (10–12), leading to progressive deterioration in motor function and a further reduction in use.
In the following, we review earlier work that has led to the concept of virtuous and vicious cycles and more recent mechanistic models that yield such cycles. We then review experimental and clinical studies supporting such a “use it and improve it or lose it” phenomenon and the existence of an effective threshold in use and function separating the virtuous and vicious cycles. We next review recent work that aims at enhancing UE use in daily activities, including our recent work using ecological momentary assessment (EMA), and propose how to further develop the efficacy of embedding rehabilitation in the wild for those who possess enough residual sensorimotor capacity to benefit from more practice with motivational reminders to use the paretic limb.
Acquired Non-use and the Origin of the Virtuous/Vicious Cycles of Recovery Post-stroke
The human motor system is highly redundant, offering multiple possible behavioral solutions to achieve a goal. When the behavior deviates from that observed in neurotypical individuals, we refer to the behavior as “compensatory” (13). For instance, individuals post-stroke frequently use their less affected hand to perform reaching movements. Because of the often large motor and sensory deficits immediately following stroke, the initial compensation is “mandatory”. However, as performance improves due to spontaneous recovery or rehabilitation, or both, the movements with the more affected arm are now possible but often not performed spontaneously (14, 15). Such “a non-use”, which is measured by the difference between what the patient can do when instructed and what the patient does when given a choice (16–18), was originally described as a learning process according to which preference for the less affected arm due to past unsuccessful repeated attempts to use the more affected UE (12, 19).1 It was also proposed that acquired non-use subsequently causes a loss of motor function leading to the acquisition of compensatory behaviors (12, 19, 21). Note that besides the loss of function, there may be other factors originating and perpetuating these compensatory behaviors, such as higher effort requirements associated with using the more affected limb (22, 23) and sensory perception and attention deficits after stroke. A re-analysis of baseline data from the EXCITE trial (24) shown in Figure 2A illustrates the often-considerable extent (and variability) of such acquired non-use in the chronic stroke population.
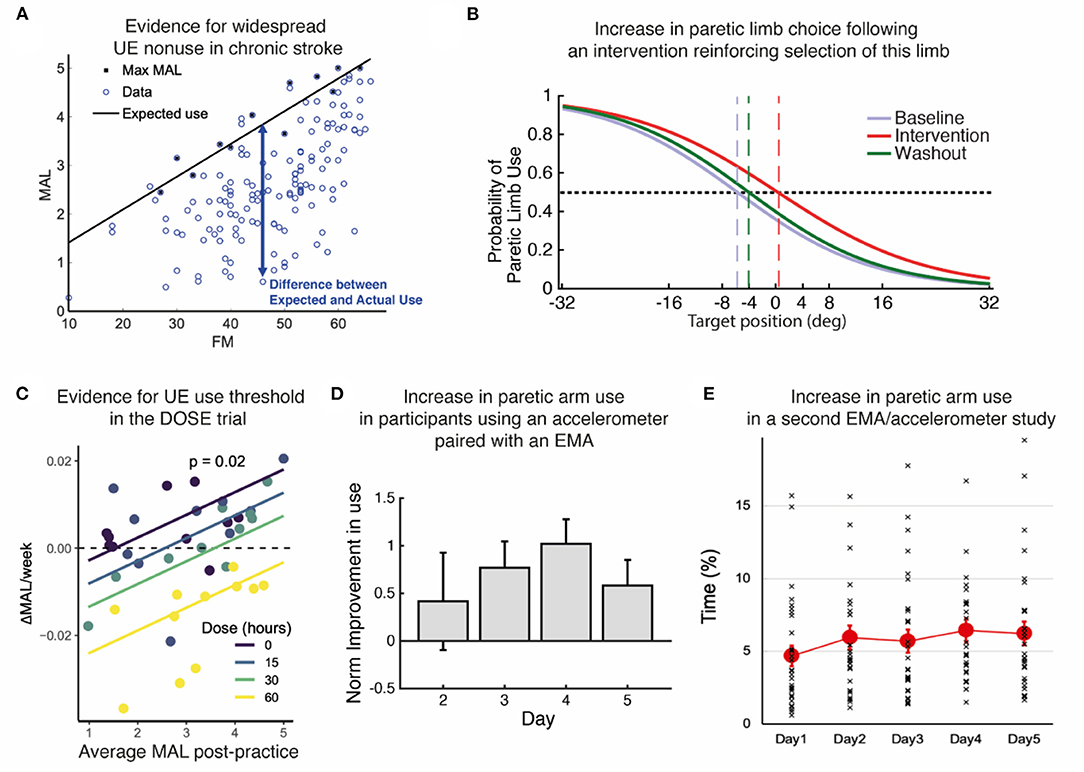
Figure 2. Summary of evidence. (A) Re-analysis of baseline data from the EXCITE trial (24) shows prevalent UE non-use beyond what is expected from impairment levels. Motor Activity Log Amount of Use sub-scale (MAL) as a function of the Upper Extremity Fugl Meyer (FM). We estimated the maximal use MaxMAL given impairment by the maximum MAL in each bin of 2 FM points (black line: MaxMAL = 0.067 × FM + 0.74). (B) Increase in paretic arm choice following an intervention that focuses on reinforcing the selection of this limb (25). Logistic fit of all subject's probabilities of paretic limb choice against target direction before (baseline), during, and after (washout) the intervention. Vertical dashed lines indicate targets with an equal probability of being reached with either arm. (C) Evidence for a threshold following practice in the DOSE study (26). The average weekly change in Motor Activity Log-Quality of Movement (MAL) following supervised practice is positively modulated by the average MAL post-practice for each dose. The intersections of the regression lines with the horizontal dashed line show the thresholds for each dose. Colored lines: retention rates as a function of average post-practice MAL for different doses; dots: individual retention rates. (D) Increase in paretic arm use in our first study using an accelerometer-embedded bracelet device paired with an EMA (25). Mean change in the activity of the paretic limb estimated by the wearable system across participants with respect to day 1 across days of intervention in which patients received haptic feedback and arm activity reports (days 2–4), and immediately after (day 5). (E) Small but statistically significant increase in paretic arm use over 5 days in our second study with an EMA / accelerometer combination (27). Solid color circles: daily average duration of unimanual right (paretic) arm/hand movements. Error bars, standard errors. Cross (×): individual use duration as a % of accelerometer wearing time.
Acquired non-use has long been recognized to be a major issue facing patients post-stroke, but only recently has there been an attempt to operationalize this complex phenomenon where multiple physiological (e.g., sensorimotor impairment, lesion load), behavioral (e.g., expected success and effort), and psychological factors (e.g., self-efficacy, perceived effort) intersect (20, 28, 29). Although little is known about how these multiple factors interact to create acquired non-use, interventions that aim at reducing non-use have been developed. In particular, constraint-induced movement therapy (CIMT), which forces the use of the more affected by restraining the less affected limb with a mitt, while intensively training the use of the more affected arm, has been shown to reduce non-use (11, 24, 30, 31). However, CIMT is indicated for only ~15% of all stroke cases (e.g., being able to voluntarily extend the wrist and at least two fingers through a specified range of motion) (32). In addition, the mitt can only be worn by a few patients, requires supervision, and is often disliked. Importantly, the mitt prevents bimanual tasks, which make up the majority of daily arm and hand activities (33, 34). Furthermore, the common factor in CIMT and its variants is the delivery of a high number of repetitions. Besides compromising treatment adherence (35), large doses of practice are difficult to implement in the clinic, and, as we recently showed, highly inefficient (i.e., with low gain in outcome per hour of practice) (26).
Virtuous and Vicious Cycles Arise From Plastic Interactions Between Motor and Decision-Making Systems: Insights From a Computational Model
Inspired by Taub's ideas, Han et al. proposed a computational model of brain plasticity and motor learning to unmask non-linear interactions between arm use and functional motor recovery (10). The model contains two main plastic neural processes: (1) A bilateral model of the motor cortex/cerebellum networks that generate reaching movements and that is (unilaterally) lesioned by stroke, and (2) a decision-making process, loosely based on the basal ganglia, that selects the arm to reach a given target. Motor performance is updated via plastic processes in the motor networks that reduce both errors and variability in movements. Motor decisions to choose one arm or the other depend on the between-arm comparison of expected future rewards, or “action values”, which are updated via plastic processes that aim at reducing reward prediction errors. Such choice mechanism is in line with studies showing that reinforcement modulates hand selection (36) and that effort plays a role in motor decisions (37–40). We confirmed since that arm selection depends on a context-dependent linear combination of the expected success and the anticipated cost for both arms in both neuro-typical (23) and post-stroke (20) individuals.
The Han et al. model predicted that if stroke suddenly decreases motor performance, the value of the more affected UE is down-regulated because of reach failures, leading to acquired non-use and compensatory choice of the less affected arm (10). In addition, the model predicted that recovery is bistable: following treatment, performance is either improving (recovery) or deteriorating. (Simulated) patients who use the affected arm above a threshold experience improved performance via “self-practice”. In turn, the amelioration of impaired performance increases use. The patients thus enter a chain of events in which improved performance leads to increase in use, which further improves performance and so on, resulting in a continuous process of improvement. Such a chain of events is called a “virtuous cycle” (Merriam Webster dictionary, 2022; Figure 1A). In contrast, (Simulated) patients who do not use the affected arm above this threshold enter a chain of events in which deteriorated performance leads to decrease in use, which further deteriorates performance, and so on, resulting in a continuous process of deterioration. Such a chain of events is called a “vicious cycle” (Merriam Webster dictionary, 2022; Figure 1B), with rehabilitation becoming “in vain”.
Recent Evidence for the Virtuous and Vicious Cycles and the Threshold
Determining the threshold in use and function separating the virtuous and vicious cycles would allow for evidence-based decision-making of treatment schedules, preventing “rehabilitation in vain” and improving clinical outcomes. Recent work has been pursued toward the determination of such a threshold at the group level. In an animal study with rats who received focal ischemia, MacLellan et al., following the threshold hypothesis of the Han et al. model, theorized that functional benefit occurs only if a threshold of rehabilitation intensity is achieved (41). Skilled reaching improved in rats with unlimited access to the reaching apparatus in the dark but not when reaching was restricted. In addition, an enriched environment did not benefit the restricted group. This study showed that a critical threshold of rehabilitation intensity was required to obtain functional benefit.
Following the threshold prediction in the Han et al. model, we (42) performed a retrospective analysis of data from the EXCITE trial (24). We compared use of the paretic UE (assessed via the subjective Motor Activity Log Amount of Use scale) 1 week after therapy to use a year later. The paretic UE function (assessed via the Wolf Motor Function Test Functional Ability Scale-FAS) measured immediately after therapy predicted, on average, long-term changes of arm use: for about two-thirds of participants with function above a threshold (3.5/5.0 FAS), use improved. Below this threshold, use decreased. In a second re-analysis of the EXCITE trial data, this time using all repeated measures of UE use (assessed via the Motor Activity Log Amount of Use Scale) and function in the 2 years following treatment, we studied putative non-linear interactions between UE function and use (9). For this purpose, we largely simplified the Han et al. model via first-order non-linear dynamical models of change in use and function, which were fitted to the EXCITE data using a Bayesian regression framework. A model with reciprocal interactions between arm function and use was the best fitting model and accounted for the virtuous and vicious cycles. Furthermore, we found that therapy increased the parameter that modulated the effect of UE function on use. Simulations showed that increasing this parameter, which can be thought of as the confidence to use the arm for a given level of function (i.e., self-efficacy for paretic limb use), led to an increase in spontaneous use and the development of a virtuous cycle by decreasing the threshold.
In the recent DOSE clinical trial (4), in which participants were randomized into groups that varied in the duration of scheduled therapy (i.e., 0, 15, 30, or 60 h), we observed a dose response for the Motor Activity Log-Quality of Movement. In a later analysis of UE use in the 6 months following training (26), we modeled the change in use during and following task practice. Analysis of the model's retention rates in terms of UE use (i.e., Motor Activity Log Quality of Movement scale) demonstrated that when use was relatively high and above a threshold, it kept increasing. Interestingly, such an effect was more pronounced in the lower dosage groups because retention following task practice was worse in the higher dosage groups (see Figure 2C).
Whereas, the above studies from our group tested the threshold hypothesis with self-reported UE use at home, a recent study with stroke survivors in the subacute stage (43) tested the threshold hypothesis using data from a wearable device, the Manumeter, which has been shown to measure the amount of arm and hand movements (44). As predicted, spontaneous paretic hand use measured at home did not increase until the participants reached a certain level of function captured by a standardized clinical scale of motor dexterity (i.e., Box and Blocks Test). Recently, Chen et al. (27) monitored in the wild paretic limb use both alone (unimanual) and with the less affected limb (bimanual) over 5 days in a group of chronic stroke survivors with a wide range of FM motor scores (i.e., 20–66) and found in a subsequent analysis (45) that it was only in 16 out of 30 participants who had FM score > 50 that average unimanual paretic use time (% accelerometer wearing time) increased from 5 to ~15% as FM score increased to 66. In contrast, bimanual arm use linearly increased from 10 to 40% as FM score increased from 20 to 66. Thus, the paretic arm is being used to a greater extent in those with FM < 50, if it is embedded in the context of bimanual activities of daily living than when it is required to function alone. This suggests that there is a different impairment threshold for bimanual upper extremity activity that is much lower than that for unimanual paretic limb use. It also justifies our initial premise that those who are mild to moderately impaired (not exclusively those with mild impairment) are the ones who stand to benefit from this approach.
Toward a Paradigm Shift: Embedding Rehabilitation in Activities of Daily Living With the Aid of Wearable Technology That Enhances Paretic Limb Use
The Han et al. model and the supporting evidence reviewed above suggest that increasing daily use (unimanual and bimanual) of the more affected UE following motor therapy can offer a solution to the problem of low dose of therapy observed in clinical settings and that of rehabilitation in vain. Because neurotypical and adults post-stroke make thousands of purposeful UE movements in daily activities (46), and such movements, via the feedback provided by the environment, could each be seen as single practice movements, increasing such self-practice could provide the large dose of movements needed to improve performance and then use.
In line with this vision, we explored a new method to promote arm use in stroke patients by boosting their confidence in more affected UE function. Participants with hemiparesis were exposed to reduced errors while performing arm shooting movements in a non-immersive virtual-reality system (25). Unaware of the manipulations, participants reported making internal attributions of the success they experienced through training and showed a higher probability of using their more affected arm (Figure 2B). We obtained similar results in a pilot study evaluating the effect of using an accelerometer-embedded bracelet device paired with ecological momentary assessment (“EMA” delivered on a smartphone) to monitor the amount of arm use and provide knowledge of progress in chronic stroke survivors (47). Participants received hourly haptic feedback and visual activity reports indicating the change from baseline in paretic arm use. The results showed a general increase in use of the more affected arm, and this increase was retained after feedback suppression (Figure 2D). Recently, we showed the feasibility of such EMA and sensor combination with thirty mild-severely motor-impaired stroke survivors (27). We found that the simple act of probing about arm use produced a small but significant increase (~10 min) in paretic arm use over 5 days (Figure 2E). In the same study, an analysis of EMA responses along with the quantitative accelerometer data revealed that social context (i.e., not alone) and self-efficacy for paretic arm/hand use complement an individual's motor capability (i.e., FM score) and play essential roles in paretic arm/hand use behavior in the natural environment (45, 48). Previous work from one of us showed that including social interaction in stroke VR-based motor rehabilitation enhances performance (49). Altogether, these studies illustrate the importance of social context, confidence, and reinforcement in restoring non-pathological hand selection patterns in stroke survivors. Future studies should shed light on which specific disability profiles would benefit the most from this type of intervention, as well as investigate whether this improvement in spontaneous use transfers to the participant's activities and increases independence.
For maximum effectiveness, the above studies suggest a personalized schedule of practice based on an individualized determination of use thresholds (unimanual and bimanual) via wearable sensors. When the different thresholds are estimated to be reached, supervised practice via EMA could be phased out, as UE use for daily activities would continue to increase. For individuals with UE use below these thresholds, strategies to overcome barriers to use in the natural environment would be needed to foster more effective engagement in self-practice (e.g., beginning with primarily bimanual tasks and then transitioning to more difficult unimanual paretic limb tasks). Thus, the next step is to determine this threshold for each individual patient. Repeated measurement of UE use in daily activities via sensors between bouts of therapy could yield precise threshold information. However, a difficulty is to monitor (via the sensors) and promote (via EMA) daily tasks that are at the “just right challenge” to maximize plastic processes involved in motor recovery. One solution is to use EMA + sensors (27) in conjunction with EMI (ecological momentary intervention) to design the optimal intervention strategy (48). As clearly shown by an early monkey study (50), not all repetitions yield plastic changes in the motor system: only the precision grasps, which had to be learned, and not the power grasps, were associated with motor cortical map plasticity. Thus, intelligent EMAs with sophisticated sensors that can monitor both arm and hand movements (e.g., bimanual and unimanual), such as the Manumeter, would need to promote a set of challenging tasks to practice, with this set varying during recovery to maintain challenge. Methods to define challenging task sets based on impairment levels have been proposed, e.g., (51). Note that in this scheme, the neurorehabilitation clinicians play an important role, as they need to assess whether or not particular movements, task components, and whole tasks are assigned so that real-world practice is engaging and productive.
In conclusion, the computational, experimental, and clinical studies reviewed above point to a user-centered approach to recovery post-stroke: besides the more traditional role of neurorehabilitation in enhancing motor function, and in line with standard therapy recommendations, we suggest that embedding rehabilitation in activities of daily living could largely improve long-term outcomes of stroke survivors. The development and validation of wearable devices for objective longitudinal monitoring and promotion of paretic arm/hand use both unimanually and bimanually may be key for implementing this rehabilitation approach that supports large doses of self-practice.
Data Availability Statement
The raw data supporting the conclusions of this article will be made available by the authors, without undue reservation.
Ethics Statement
Figure 2A presents results from a re-analysis of data from the EXCITE trial [(24), JAMA]. The trial was approved by the respective institutional review boards of each participating site of the EXCITE trial. The patients/participants provided their written informed consent to participate in this study.
Author Contributions
All authors listed have made a substantial, direct, and intellectual contribution to the work and approved it for publication.
Funding
This work was supported by NIH grants R21NS120274 to NS, R01HD065438 to NS and CW, R41HD104296 to CW, and the RGS@home project from H2020-EU, EIT Health (ID:19277).
Conflict of Interest
NS is a co-founder of Motion Scientific, Inc., a company that is developing rehabilitation technologies. The terms of this arrangement have been reviewed and approved by the University of Southern California, Los Angeles in accordance with its conflict of interest policies. CW is a member of the data safety and monitoring board for Enspire DBS Therapy, Inc (DBS is Deep Brain Stimulation) and receives an honorarium for her services. She is a member of the external advisory board for MicroTransponder, Inc. and receives payment for her consulting. CW is Editor of the 6th edition of Motor Control and Learning, published by Human Kinetics, Inc and receives royalty payments. CW is also an Editor for the 2nd Edition of Stroke Recovery and Rehabilitation, published by DemosMedical Publishers and receives royalty payments.
The remaining author declares that the research was conducted in the absence of any commercial or financial relationships that could be construed as a potential conflict of interest.
Publisher's Note
All claims expressed in this article are solely those of the authors and do not necessarily represent those of their affiliated organizations, or those of the publisher, the editors and the reviewers. Any product that may be evaluated in this article, or claim that may be made by its manufacturer, is not guaranteed or endorsed by the publisher.
Acknowledgments
We thank Cheol Han, Yukikazu Hidaka, Sujin Kim, Chunji Wang, Yi-An Chen, John Monterosso, Michael Arbib, Steve Wolf, Rini Varghese, Marika Demers, Jens Nirme, Armin Duff, Martina Maier, and Paul Verschure for fruitful discussions that have led to the ideas proposed here.
Footnotes
1. ^The original term “learned non-use” (LNU) was developed from studies in a monkey deafferentation model that is not the same as a stroke model. In spite of these different models, Taub et al. perpetuated the use of the term in their human stroke model work. However, there is no consensus as to what extent the observed non-use post-stroke is acquired through operant conditioning of early attempts to use the paretic limb that failed/negative reinforcement, or due to other factors such as diminished attention from reduced sensory perception, etc. Therefore, instead of the term LNU, we use “acquired non-use” to acknowledge the discrepancy between the deafferentation and stroke models. In our empirical and modeling work (10, 17, 20), we have developed an objective measure of “acquired non-use” using a paradigm first introduced by Sterr et al. (16) where we take the difference in the tasks or targets attempted in a “free” (spontaneous) condition in which the participant can choose either arm to capture the target, to that in a “forced” condition in which the participant must use the paretic limb to capture the target. The degree to which the “forced” condition is greater than the “free” condition captures the magnitude of spontaneous non-use and is consistent with the Andrews and Stewart article (14), “He can, but does he.” Our objective measure highlights the action selection process in arm use that precedes the actual behavior and that is of great interest to rehabilitation scientists who seek to develop interventions to promote the selection of the paretic limb spontaneously in the natural environment.
References
1. Maier M, Ballester BR, Verschure P. Principles of neurorehabilitation after stroke based on motor learning and brain plasticity mechanisms. Front Syst Neurosci. (2019) 13:74. doi: 10.3389/fnsys.2019.00074
2. Daly JJ, McCabe JP, Holcomb J, Monkiewicz M, Gansen J, Pundik S. Long-dose intensive therapy is necessary for strong, clinically significant, upper limb functional gains and retained gains in severe/moderate chronic stroke. Neurorehabil Neural Repair. (2019) 33:523–37. doi: 10.1177/1545968319846120
3. Ward NS, Brander F, Kelly K. Intensive upper limb neurorehabilitation in chronic stroke: outcomes from the Queen Square programme. J Neurol Neurosurg Psychiatry. (2019) 90:498–506. doi: 10.1136/jnnp-2018-319954
4. Winstein C, Kim B, Kim S, Martinez C, Schweighofer N. Dosage matters. Stroke. (2019) 50:1831–7. doi: 10.1161/STROKEAHA.118.023603
5. Ballester BR, Ward NS, Brander F, Maier M, Kelly K, Verschure P. Relationship between intensity and recovery in post-stroke rehabilitation: a retrospective analysis. J Neurol Neurosurg Psychiatry. (2021) 93:226–28. doi: 10.1136/jnnp-2021-326948
6. Lang CE, Macdonald JR, Reisman DS, Boyd L, Jacobson Kimberley T, Schindler-Ivens SM, et al. Observation of amounts of movement practice provided during stroke rehabilitation. Arch Phys Med Rehabil. (2009) 90:1692–8. doi: 10.1016/j.apmr.2009.04.005
7. European Stroke Organisation (ESO) Executive Committee; ESO Writing Committee. Guidelines for management of ischaemic stroke and transient ischaemic attack 2008. Cerebrovasc Dis. (2008) 25:457–507. doi: 10.1159/000131083
8. Meyer S, Verheyden G, Brinkmann N, Dejaeger E, De Weerdt W, Feys H, et al. Functional and motor outcome 5 years after stroke is equivalent to outcome at 2 months: follow-up of the collaborative evaluation of rehabilitation in stroke across Europe. Stroke. (2015) 46:1613–9. doi: 10.1161/STROKEAHA.115.009421
9. Hidaka Y, Han CE, Wolf SL, Winstein CJ, Schweighofer N. Use it and improve it or lose it: interactions between arm function and use in humans post-stroke. PLoS Comput Biol. (2012) 8:e1002343. doi: 10.1371/journal.pcbi.1002343
10. Han CE, Arbib MA, Schweighofer N. Stroke rehabilitation reaches a threshold. PLoS Comput Biol. (2008) 4:e1000133. doi: 10.1371/journal.pcbi.1000133
11. Taub E, Uswatte G, Elbert T. New treatments in neurorehabilitation founded on basic research. Nat Rev Neurosci. (2002) 3:228–36. doi: 10.1038/nrn754
12. Taub E, Uswatte G, Mark VW, Morris DM. The learned nonuse phenomenon: implications for rehabilitation. Eura Medicophys. (2006) 42:241–56.
13. Levin MF, Kleim JA, Wolf SL. What do motor recovery and compensation mean in patients following stroke? Neurorehabil Neural Repair. (2009) 23:313–9. doi: 10.1177/1545968308328727
14. Andrews K, Stewart J. Stroke recovery: he can but does he? Rheumatol Rehabil. (1979) 18:43–8. doi: 10.1093/rheumatology/18.1.43
15. Michielsen ME, Selles RW, Stam HJ, Ribbers GM, Bussmann JB. Quantifying nonuse in chronic stroke patients: a study into paretic, nonparetic, and bimanual upper-limb use in daily life. Arch Phys Med Rehabil. (2012) 93:1975–81. doi: 10.1016/j.apmr.2012.03.016
16. Sterr A, Freivogel S, Schmalohr D. Neurobehavioral aspects of recovery: assessment of the learned nonuse phenomenon in hemiparetic adolescents. Arch Phys Med Rehabil. (2002) 83:1726–31. doi: 10.1053/apmr.2002.35660
17. Han CE, Kim S, Chen S, Lai YH, Lee JY, Osu R, et al. Quantifying arm nonuse in individuals poststroke. Neurorehabil Neural Repair. (2013) 27:439–47. doi: 10.1177/1545968312471904
18. Bakhti KKA, Mottet D, Schweighofer N, Froger J, Laffont I. Proximal arm non-use when reaching after a stroke. Neurosci Lett. (2017) 657:91–6. doi: 10.1016/j.neulet.2017.07.055
19. Taub E, Crago JE, Burgio LD, Groomes TE, Cook EW 3rd, DeLuca SC, et al. An operant approach to rehabilitation medicine: overcoming learned nonuse by shaping. J Exp Anal Behav. (1994) 61:281–93. doi: 10.1901/jeab.1994.61-281
20. Kim S, Han C, Kim B, Winstein C, Schweighofer N. Effort, success, and side of lesion determine arm choice in chronic stroke survivors with mild-to-moderate impairment. J Neurophysiol. (2022) 127:255–56. doi: 10.1152/jn.00532.2020
21. Knapp HD, Taub E, Berman AJ. Movements in monkeys with deafferented forelimbs. Exp Neurol. (1963) 7:305–15. doi: 10.1016/0014-4886(63)90077-3
22. Sunderland A, Tuke A. Neuroplasticity, learning and recovery after stroke: a critical evaluation of constraint-induced therapy. Neuropsychol Rehabil. (2005) 15:81–96. doi: 10.1080/09602010443000047
23. Schweighofer N, Xiao Y, Kim S, Yoshioka T, Gordon J, Osu R. Effort, success, and nonuse determine arm choice. J Neurophysiol. (2015) 114:551–9. doi: 10.1152/jn.00593.2014
24. Wolf SL, Winstein CJ, Miller JP, Taub E, Uswatte G, Morris D, et al. (2006). Effect of constraint-induced movement therapy on upper extremity function 3 to 9 months after stroke: the excite randomized clinical trial. JAMA. 296, 2095–2104. doi: 10.1001/jama.296.17.2095
25. Ballester BR, Nirme J, Duarte E, Cuxart A, Rodriguez S, Verschure P, et al. The visual amplification of goal-oriented movements counteracts acquired non-use in hemiparetic stroke patients. J Neuroeng Rehabil. (2015) 12:50. doi: 10.1186/s12984-015-0039-z
26. Wang C, Winstein C, D'Argenio DZ, Schweighofer N. The efficiency, efficacy, and retention of task practice in chronic stroke. Neurorehabil Neural Repair. (2020) 34:881–90. doi: 10.1177/1545968320948609
27. Chen YA, Demers M, Lewthwaite R, Schweighofer N, Monterosso JR, Fisher BE, et al. A novel combination of accelerometry and ecological momentary assessment for post-stroke paretic arm/hand use: feasibility and validity. J Clin Med. (2021) 10:1328–44. doi: 10.3390/jcm10061328
28. Buxbaum LJ, Varghese R, Stoll H, Winstein CJ. Predictors of arm nonuse in chronic stroke: a preliminary investigation. Neurorehabil Neural Repair. (2020) 34:512–22. doi: 10.1177/1545968320913554
29. Hirsch T, Barthel M, Aarts P, Chen YA, Freivogel S, Johnson MJ, et al. A first step toward the operationalization of the learned non-use phenomenon: a Delphi Study. Neurorehabil Neural Repair. (2021) 35:383–92. doi: 10.1177/1545968321999064
30. Wolf SL, Lecraw DE, Barton LA, Jann BB. Forced use of hemiplegic upper extremities to reverse the effect of learned nonuse among chronic stroke and head-injured patients. Exp Neurol. (1989) 104:125–32. doi: 10.1016/S0014-4886(89)80005-6
31. Kwakkel G, Veerbeek JM, van Wegen EE, Wolf SL. Constraint-induced movement therapy after stroke. Lancet Neurol. (2015) 14:224–34. doi: 10.1016/S1474-4422(14)70160-7
32. Viana R, Teasell R. Barriers to the implementation of constraint-induced movement therapy into practice. Top Stroke Rehabil. (2012) 19:104–14. doi: 10.1310/tsr1902-104
33. Varghese R, Kutch JJ, Schweighofer N, Winstein CJ. The probability of choosing both hands depends on an interaction between motor capacity and limb-specific control in chronic stroke. Exp Brain Res. (2020) 238:2569–79. doi: 10.1007/s00221-020-05909-5
34. Kilbreath SL, Heard RC. Frequency of hand use in healthy older persons. Aust J Physiother. (2005) 51:119–22. doi: 10.1016/s0004-9514(05)70040-4
35. Page SJ, Levine P, Sisto S, Bond Q, Johnston MV. Stroke patients' and therapists' opinions of constraint-induced movement therapy. Clin Rehabil. (2002) 16:55–60. doi: 10.1191/0269215502cr473oa
36. Stoloff RH, Taylor JA, Xu J, Ridderikhoff A, Ivry RB. Effect of reinforcement history on hand choice in an unconstrained reaching task. Front Neurosci. (2011) 5:41. doi: 10.3389/fnins.2011.00041
37. Cos I, Belanger N, Cisek P. The influence of predicted arm biomechanics on decision making. J Neurophysiol. (2011) 105:3022–33. doi: 10.1152/jn.00975.2010
38. Wang W, Dounskaia N. Load emphasizes muscle effort minimization during selection of arm movement direction. J Neuroeng Rehabil. (2012) 9:70. doi: 10.1186/1743-0003-9-70
39. Shadmehr R, Huang HJ, Ahmed AA. A representation of effort in decision-making and motor control. Curr Biol. (2016) 26:1929–34. doi: 10.1016/j.cub.2016.05.065
40. Wang C, Xiao Y, Burdet E, Gordon J, Schweighofer N. The duration of reaching movement is longer than predicted by minimum variance. J Neurophysiol. (2016) 116:2342–5. doi: 10.1152/jn.00148.2016
41. MacLellan CL, Keough MB, Granter-Button S, Chernenko GA, Butt S, Corbett D. A critical threshold of rehabilitation involving brain-derived neurotrophic factor is required for poststroke recovery. Neurorehabil Neural Repair. (2011) 25:740–8. doi: 10.1177/1545968311407517
42. Schweighofer N, Han CE, Wolf SL, Arbib MA, Winstein CJ. A functional threshold for long-term use of hand and arm function can be determined: predictions from a computational model and supporting data from the Extremity Constraint-Induced Therapy Evaluation (EXCITE) Trial. Phys Ther. (2009) 89:1327–36. doi: 10.2522/ptj.20080402
43. de Lucena DS, Rowe J, Chan V, Reinkensmeyer DJ. Magnetically counting hand movements: validation of a calibration-free algorithm and application to testing the threshold hypothesis of real-world hand use after stroke. Sensors. (2021) 21:1502. doi: 10.3390/s21041502
44. Friedman N, Rowe JB, Reinkensmeyer DJ, Bachman M. The manumeter: a wearable device for monitoring daily use of the wrist and fingers. IEEE J Biomed Health Inform. (2014) 18:1804–12. doi: 10.1109/JBHI.2014.2329841
45. Chen YA, Lewthwaite R, Schweighofer N, Monterosso J, Fisher BE, Winstein C. The essential role of social context and self-efficacy in daily paretic arm/hand use after stroke: An ecological momentary assessment study with accelerometry. (2022). [In Preparation].
46. Yang CL, Liu J, Simpson LA, Menon C, Eng JJ. Real-world functional grasping activity in individuals with stroke and healthy controls using a novel wearable wrist sensor. Neurorehabil Neural Repair. (2021) 35:929–37. doi: 10.1177/15459683211041312
47. Ballester BR, Lathe A, Duarte E, Duff A, Verschure PF. A wearable bracelet device for promoting arm use in stroke patients. NEUROTECHNIX. (2015) 24–31. doi: 10.5220/0005662300240031
48. Demers M, Winstein CJ. A perspective on the use of ecological momentary assessment and intervention to promote stroke recovery and rehabilitation. Top Stroke Rehabil. (2021) 28:594–605. doi: 10.1080/10749357.2020.1856557
49. Ballester BR, i Badia SB, Verschure PF. Including social interaction in stroke VR-based motor rehabilitation enhances performance: a pilot study. Presence. (2012) 21:490–501. doi: 10.1162/PRES_a_00129
50. Plautz EJ, Milliken GW, Nudo RJ. Effects of repetitive motor training on movement representations in adult squirrel monkeys: role of use versus learning. Neurobiol Learn Mem. (2000) 74:27–55. doi: 10.1006/nlme.1999.3934
Keywords: stroke, neurorehabilitation, learned non-use, computational neurorehabilitation, decision-making, compensatory movement, wearable sensors
Citation: Ballester BR, Winstein C and Schweighofer N (2022) Virtuous and Vicious Cycles of Arm Use and Function Post-stroke. Front. Neurol. 13:804211. doi: 10.3389/fneur.2022.804211
Received: 29 October 2021; Accepted: 03 February 2022;
Published: 29 March 2022.
Edited by:
Andreas R. Luft, University of Zurich, SwitzerlandReviewed by:
Niamh C. Kennedy, Ulster University, United KingdomJanis J. Daly, University of Florida, United States
Copyright © 2022 Ballester, Winstein and Schweighofer. This is an open-access article distributed under the terms of the Creative Commons Attribution License (CC BY). The use, distribution or reproduction in other forums is permitted, provided the original author(s) and the copyright owner(s) are credited and that the original publication in this journal is cited, in accordance with accepted academic practice. No use, distribution or reproduction is permitted which does not comply with these terms.
*Correspondence: Nicolas Schweighofer, schweigh@usc.edu