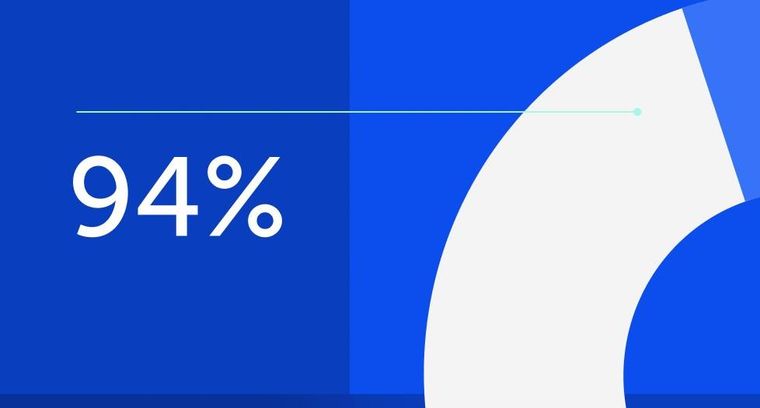
94% of researchers rate our articles as excellent or good
Learn more about the work of our research integrity team to safeguard the quality of each article we publish.
Find out more
BRIEF RESEARCH REPORT article
Front. Neurol., 13 May 2022
Sec. Neurotrauma
Volume 13 - 2022 | https://doi.org/10.3389/fneur.2022.778697
Objectives: Intrathecal baclofen (ITB) is commonly used for reduction of spasticity in chronic spinal cord injury (SCI). Its clinical effect is well-known; however, exact mechanisms of long-term effect of continuous ITB administration (cITBa) on modulation of cortical processes have not been elucidated. The aim of this study was to evaluate changes in motor cortex activation for healthy upper limbs in comparison to impaired lower limbs by functional magnetic resonance imaging (fMRI).
Methods: Ten subjects (eight males, 20–69 years) with thoracic SCI presenting no voluntary movements of lower limbs (except one) were enrolled in the fMRI study. fMRI at 1.5T with a finger tapping paradigm and mental movement simulating foot flexion on the dominant side were performed before, 3 months, and 1 year after start of cITBa. fMRI data processing was carried out using FMRI Expert Analysis Tool (FEAT), part of FSL. A second-level analysis was carried out using FLAME stages 1 and 2. The level of spasticity was assessed with the Modified Ashworth scale (MAS).
Results: Continuous ITB significantly decreased limb spasticity in all the subjects (group MAS spasticity dropped from 3 to 0.3). The second-level analysis (Z > 1.6, cluster significance threshold p =0.05) revealed increased activation of the primary sensorimotor cortex of the foot between baseline and 3 months, and 3 months and 1 year.
Conclusion: Increased sensorimotor cortex activation with spasticity reduction after cITBa may reflect distant functional reorganization because of long-term mediated neuroplastic changes in the sensorimotor cortex. Better understanding of modulation of brain function in SCI after cITBa may influence the field of neurorehabilitation.
Spasticity is a severe clinical manifestation of upper motor neuron lesions. It has been reported in 10–65% of patients with chronic spinal cord injury (SCI) (1–4). Neural connectivity after SCI is altered in the spinal cord; however, reorganization of the brain is considered to be an important mechanism following SCI, requiring adaptive changes in surviving circuitries.
Spasticity in subjects with SCI may be alleviated by non-pharmacological treatment (5), and pharmacotherapy is the most common treatment (6–8). A wide variety of drugs is recommended, with different action sites, but the most widely used medicine is baclofen, a gamma-aminobutyric acid (GABA B) receptor agonist. A very good effect of continuous intrathecal baclofen (cITB) administration using pump delivery systems has been demonstrated in generalized SCI spasticity (9–12); however, associated complications influence its utility and acceptance (13–17). Intrathecal baclofen delivery concentrates the drug in the cerebrospinal fluid at higher levels, and patients can use concentrations of baclofen <100 of those used orally. Thus, the central side effects of oral baclofen, such as drowsiness, fatigue, and difficulties to concentrate, appear to be minimized with intrathecal administration. Moreover, long-term intrathecal baclofen delivery, even at a high dose, has only a minor influence on cognitive functions (18).
To this date, little is known about pathophysiological mechanisms involved in spinal spasticity after neuromodulatory treatment as cITB delivery. ITB is considered to act differently at the spinal, brainstem, and cortical levels (19, 20). The impact of neuronal inhibition on the functional activity of the brain has already been studied with baclofen as a GABA(B) receptor agonist generally decreasing metabolic activity (21). Increased activation of the sensorimotor cortex has been observed by functional magnetic resonance imaging (fMRI) 1 month after baclofen pump implantation in one patient with multiple sclerosis (22). Sensorimotor network changes after chronic SCI treated by intrathecal baclofen delivery has not yet been systematically studied.
The aim of this study is to determine by fMRI whether long-term administration of cITB has any effect on cortical plasticity changes over time in patients with SCI. We studied a selected population of patients with chronic thoracic SCI and compared fMRI data prior to baclofen pump implantation, 3 months after, and 1 year after cITB pump delivery.
From our database, we selected 15 patients with chronic SCI who fulfill the criteria for ITB pump implantation, e.g., low response to oral antispastic treatment and severe limb spasticity of 3 or 4 according to the Modified Ashworth scale (MAS), who underwent a positive clinical trial with ITB administration through a temporarily inserted intrathecal catheter. For further analysis to keep the homogeneity of lesion localization, we finally selected 10 subjects (eight males, aged 19–69 years, mean of 46) with only thoracic SCI. We excluded patients with traumatic brain injury as well as patients with peripheral nerve lesions or any neurological condition influencing the brain or spine (e.g., multiple sclerosis). Therefore, thoracic spine lesion is, in our cohort, the only neurological and neuroradiological condition that impairs motor functions. To achieve this selection, all the subjects underwent brain and spinal cord magnetic resonance imaging (MRI) before cITB pump implantation, and diagnostic brain MRI was performed in each fMRI session.
All the subjects were neurologically examined by an experienced neurologist (IS) in terms of motor, sensory, and cognitive functions before, and 3 months and 1 year after cITB pump implantation. The clinical testing of patients used standard scales, the AIS Scale (American Association of Spinal Cord injury Impairment Scale) of spinal cord function after injury (23) and the MAS of muscle hypertonia (grades 0–4) (24). The MAS is the most accepted clinical tool used to measure the level of muscle tone (25).
In this study, evaluation of spasticity using MAS was performed every visit before and 3 months and 1 year after cITB pump implantation. This evaluation was performed again by the experienced neurologist (IS). The MAS was assessed separately for dorsal and plantar flexions of the foot and flexion and extension of the knees and hip. The values were averaged and calculated as mean MAS for each subject. The daily dose of baclofen was assessed every visit according to the level of spasticity. The patients were not under supervision with specific rehabilitation; they exercised at home and performed their normal daily activities.
All the subjects have also been examined with fMRI techniques using a detailed protocol of motor functions. All the patients were informed, in a comprehensible manner, about the risks arising from fMRI and the possibility to withdraw from the study at any time. Pump function was tested within 30 min after the completion of the fMRI procedure to avoid any complication, e.g., unsuccessful restart of the function after a possible brief stop of pumping during the examination. To further limit potential risks from the MRI examination, a 1.5 T scanner (Siemens Avanto, Erlangen, Germany) equipped with quantum gradient coils and a standard head coil) was used (lower static magnetic field induces lower force to the device compared to 3T).
Two motor tasks were employed: finger-tapping and foot flexion were studied by 1.5T fMRI with movement on the dominant side (the data of two left-handed subjects was flipped in the x axis to have the activation on the same side of the image).
The tasks were performed before and 3 months and 1 year after cITB pump implantation. The patients were scanned while performing two tasks: (1) imaginary movement of impaired foot and (2) real finger movement with the healthy hand. All the subjects had preserved finger movements and performed sequential finger movements at a rate of approximately 1 movement per second. In the second task, the subjects were asked to imagine performing the movement of repetitive dorsal and plantar flexions at the same frequency as for the hand finger movements.
In both tasks, a block paradigm was used (TR = 3,000 ms, TR = 51 ms, voxel size 3 mm isotropic): after two dummy pulses, 16 volumes (48 s) of rest data and 16 volumes (48 s) of task data were repeatedly acquired. A total of four pairs of conditions were performed for a total acquisition length of each fMRI experiment of 6.5 min. Inside the bore of the scanner, the task was performed with the eyes open, and instructions were given with a color square in the middle of the visual field, red to stop and green to start the task. While performing the active movement task, the subjects were carefully observed to follow the pattern of the design (in an imaginary task, no such check is possible).
Standard morphological examinations (T1- and T2-weighted imaging, FLAIR) were further performed to exclude brain pathology.
FMRI data processing was carried out using FMRI Expert Analysis Tool (FEAT) Version 6.00, part of FSL (FMRIB Software Library, www.fmrib.ox.ac.uk/fsl). In the first-level analysis, two dummy pulses were removed, temporal high-pass filter was 90 s, and brain extraction and 6-mm spatial smoothing were applied. Motion correction was performed using MCFLIRT. A statistical analysis for this step was performed using FILM prewhitening and both standard and extended motion parameters. The second-level analysis was carried out using FLAME stages 1 and 2, and Z (Gaussianized T/F) statistic images were thresholded using clusters determined with Z > 1.6 and a (corrected) cluster significance threshold of P =0.05 (26, 27).
All the patients had SCI because of a fall or a motor vehicle accident and were immediately operated after SCI; only one patient (no. 6) suffered from spinal arterial-venous malformation (AVM) and he underwent embolization of AVM twice. This subject was included, as the level of lesion was identical to that in the other subjects; however, his loss of motor function was incomplete (AIS C). All the remaining subjects had paraplegia (AIS A) with loss of motor function. Detailed clinical characteristics of the patient group are summarized in Table 1.
The level of limb spasticity assessed by the Modified Ashworth scale (MAS) significantly decreased in all the subjects, whereas group MAS limb spasticity dropped from 3 to 0.75 after 3 months of cITB treatment, and to 0.3 1 year after cITB pump implantation. All the patients had continuous baclofen administration, with an average baclofen dose of 142.2 μg/day (range 90–300 μg/day) 3 months after cITB pump implantation and 215.5 μg/day (range 125–400 μg/day) 1 year after cITB pump implantation. During the whole study, no ITB drug side effects (e.g., overdose, excessive weakness, drowsiness, and withdrawal syndrome), complications resulting from pump implantation, and failure of the pump system, as well as complications during standard baclofen filling of the reservoir were observed.
Diagnostic brain MRI was performed every fMRI session and read as negative by an experienced neuroradiologist (JK).
After the first-level statistical analysis (see Methods above), the second-level analysis was performed on the data from both tasks, comparing pairwise baseline to 3 months and 3 months to 12 months: in the mental foot flexion task, an increase in fMRI activation was detected in the comparison between pre-implant and 3 months of follow-up, as well as between 3 and 12 months of examination (Figure 1), predominantly in the bilateral primary motor areas. Between baseline and 3 months, the increase is not only visible in the primary motor area but also in the parietal lobes, sensorimotor area, parts of occipital regions, and in a small cluster in the left cerebellar hemisphere. Between 3 and 12 months, the activation increased again in the primary motor cortex and in the bilateral frontopolar and temporobasal regions.
Figure 1. Difference in activation between [(A,C), left column) baseline and 3 months after baclofen pump implantation, and [(B,D), right column] 3 and 12 months after implantation. Increase in foot in both, (A) baseline and 3 months comparison and (B) 3 and 12 months, (C) Increase in hand in the first 3 months, and decrease (!) (D) between 3 and 12 months. Statistic images were thresholded non-parametrically using clusters determined with Z > 1.6 and a (corrected) cluster significance threshold of P = 0.05. For full statistical output, please see Supplementary Materials.
In the real motor finger tapping task 3 months after pump implantation, an increase in activation was present in the apical part of both cerebellar hemispheres (more on the right) and in the central region (more on the left). Between months 3 and 12, a nearly identical image is seen in an inverted manner: there is a statistically significant decrease in activation (confirmed by testing baseline-12 months with no difference, data not shown). In upper limb, an increase between the baseline and month 3 was detected when MAS was used as a primary measure, including the order of the examination as a confound factor (see Supplementary Material).
Continuous intrathecal baclofen administration (cITBa) profoundly relieving spasticity in patients with chronic thoracic SCI was associated with increased activation of the sensorimotor cortex of plegic legs 3 months and 1 year after cITBa, which may reflect distant functional reorganization of the sensorimotor network at the cortical level. The activation observed in the primary motor areas, parietal lobes, sensorimotor area, parts of occipital regions and cerebellum may help to compensate for functional deficit following SCI.
Recovery of function following injury in the nervous system requires adaptive changes in surviving circuitries. Neural connectivity has not only been shown to be altered after SCI in the spinal cord but also in the brain (28, 29). Resting-state fMRI in animal studies (30) after complete thoracic SCI showed that the functional connectivity between the primary motor and primary sensory areas was significantly decreased, and that the connectivity between the primary motor and motivation areas was increased and, thus, explained as a time-dependent compensatory upregulation of “motor functional motivation”. Motor and sensory deficits after SCI result in functional reorganization of the sensorimotor network (28, 31), which has also been documented by several task-evoked functional magnetic resonance imaging human studies (32). In a meta-analysis of fMRI studies, reorganization primarily occurred in the sensorimotor system of the brain after SCI, implying that brain functions involved in sensorimotor demands can still be preserved in this condition (33). In addition, a whole-brain meta-analysis revealed increased activation in the cerebellum, and this increase was positively correlated with lesion level and injury severity.
In a longitudinal study on patients with quadriplegia (32), a gradual reduction in the extent of activation in the primary motor cortex was detected, which was accompanied by a transient increase in the activity of sensorimotor areas. Nakanishi (34) recently investigated 8 subjects with chronic SCI by fMRI and found that the subjects with SCI showed higher grip force steadiness, smaller activation in the primary motor cortex, and deactivation of the visual cortex. Min et al. (35) performed resting-state fMRI and presented opposing findings of increased functional connectivity between the primary motor cortex and other motor areas. They concluded that motor components in the motor network increased in functional connectivity in order to compensate for motor deficits. In our study, patients with SCI and plegic lower limbs showed increased activity in the primary motor cortex after ITB delivery, and this observation was accompanied with wider activation in the sensorimotor area, parietal lobes, visual cortex, and cerebellum. One explanation for this observation may be that long-term sensory deprivation due to SCI increases cortical excitability and accelerates the plasticity of other brain areas, but therapeutic-mediated mechanisms may also play an important role in functional restoration (36).
Patients with chronic SCI in the thoracic region have more activated areas in the somatosensory cortex for the healthy upper limbs (29). Motor function after the injury may be enhanced, as individuals with SCI need their remaining body parts to compensate for lost function and to adapt their daily life with their remaining intact limbs (34). A similar observation was also made in this study with the real motor finger tapping task. Three months after ITB pump implantation, an increase in activation was present in the central region. One year after ITB pump implantation, we observed functional “normalization” with a decrease in activation in this region. A possible explanation may be the good clinical response to ITB treatment, with the overall relief of leg spasticity inducing adaptive mechanisms in brain and spinal cord connections. However, the observed brain reorganization could be not only due to pharmacotherapy and decrease in spasticity but also natural compensatory neuroplastic changes. These changes may also depend on the level of rehabilitation and individual activities of daily living. Similarly, it can be observed with botulinum toxin treatment for focal spasticity, where botulinum toxin becomes gradually accepted as a promising tool to correct maladaptive plastic changes in the sensorimotor cortex in stroke and dystonia (37, 38).
In the last few years, an increasing number of studies have also presented the cerebellum as another important subcortical brain structure in patients with chronic SCI (31, 33, 39). Chen (40) performed sensory-task related fMRI on subjects with incomplete SCI and documented the existence of an alternative pathway in the impairment of somatosensory function after SCI, which consists of the ipsilateral cerebellum, brainstem, and contralateral postcentral gyrus. In our study, we also found a significant increase in fingers movement-induced fMRI activation of several brain areas, especially in sensorimotor control and motor learning. Our data support that the successive increase in activation in mental tasks may reflect a functional reorganization of the motor cortex involved in impaired leg function. The increase in activation during the first examination 3 months after the implantation may be explained by the co-activation of lower-limb motor areas and the cerebellum in the early adaptation stage, which returns to normal after stabilizing the daily dosage of baclofen.
GABAergic pathways are involved in the regulation of muscle tone. In chronic SCI, there is a decrease in GABA, the inhibitory neurotransmitter involved in presynaptic inhibition (41). As we have already known, loss of supraspinal control and GABA dysfunction in the spinal cord are some of the pathophysiological mechanisms involved in spasticity (42); thus, it may be possible that the continuous administration of baclofen (GABA analog) modulating the spasticity in our patients with SCI contributes, to some extent, to the observed motor cortical changes.
We conclude that long-term ITB administration relieves spasticity and results in various changes in the morphological substrate of neural networks with distant functional reorganization of the sensorimotor network at the cortical level due to long-lasting neuroplastic changes. We are currently unable to determine what plays a major role in above mentioned long-term brain reorganization if pharmacotherapy with decrease of spasticity, GABA modulation, the natural course of the disease or combination of all mechanisms.
Detailed understanding of the mechanisms of corticospinal plasticity in motor learning will help to improve restoration of motor functions after spinal cord injury and may have broader applications in the field of neurorehabilitation.
This study has implications for clinical outcomes of patients with SCI, demonstrating how continuous baclofen delivery relieves spasticity in these subjects together with long-term modulation of motor cortex activity. Better knowledge of this motor cortex activation/inhibition may help in rehabilitation/restoration of the sensorimotor network after chronic spinal cord lesions. Future studies are needed to understand how the natural course of chronic SCI develops by predicting clinical presentations of severe spasticity responding to a variety of personalized therapeutic approaches, including pharmacological treatment such as intrathecal baclofen delivery.
Due to logistic and ethical barriers in patient recruitment including overall cost and proximity to MRI facilities and cITB treatment, we included only treated cITB group. We tried to get the group homogeneity as much as possible in terms of localization of the thoracic spinal cord lesion and similar clinical picture with lower extremities impairment. Thus, only one subject was able to have a voluntary movement in his lower limbs. He performed the same “mental” tests as the others. The small sample size of our group limits the application of these findings to the broader SCI population.
Future studies involving a representative sample of cITB and non-cITB patients as well as patients treated with a different drug for spasticity are needed to further validate motor cortex activation in terms of natural course of chronic SCI and/or baclofen-specific effect.
The raw data supporting the conclusions of this article will be made available by the authors, without undue reservation.
This study involving human participants were reviewed and approved by the Local Ethical Committee of University Hospital (Ethical Committee of Královské Vinohrady University Hospital, No. EK-VP/16/0/2016). All subjects provided their written informed consent to participate in this study and were informed of the possibility to withdraw from the study at any time.
IŠ and JK had a major role in the conceptualization and design of the study, data analyses, interpretation of the findings, and writing of the manuscript. JK also conducted functional magnetic resonance data assessment. Both authors contributed to the article and approved the submitted version.
This research was supported by the program Cooperation - Neuroscience, Charles University, and by a grant from the Ministry of Health of the Czech Republic-DRO (NHH, 00023884).
The authors declare that the research was conducted in the absence of any commercial or financial relationships that could be construed as a potential conflict of interest.
All claims expressed in this article are solely those of the authors and do not necessarily represent those of their affiliated organizations, or those of the publisher, the editors and the reviewers. Any product that may be evaluated in this article, or claim that may be made by its manufacturer, is not guaranteed or endorsed by the publisher.
The authors would like to thank Professor Robert Jech, MD, PhD (Center for Interventional Therapy of Movement Disorders, Department of Neurology, Charles University, 1st Faculty of Medicine and General University Hospital in Prague) for his advice on data preparation.
The Supplementary Material for this article can be found online at: https://www.frontiersin.org/articles/10.3389/fneur.2022.778697/full#supplementary-material
All Z (Gaussianized T/F) statistic images were thresholded non-parametrically using clusters determined with Z > 1.6 and a (corrected) cluster significance threshold of P = 0.05.
Supplementary Figure S1. All slices from Figure 1 (see Figure 1 legend for details).
Supplementary Figure S2. Task for the upper limb, comparison between baseline and 3-month follow-up studies. Areas where activation is higher have higher Modified Ashworth scale (MAS) values, order of the examination is used as a confound factor.
Supplementary Figure S3. Task for the upper limb, comparison between 3- and 12-month follow-up studies. Areas where activation is higher have higher MAS values, order of the examination is used as a confound factor.
1. Adams MM, Hicks AL. Spasticity after spinal cord injury. Spinal Cord. (2005) 43:577–86. doi: 10.1038/sj.sc.3101757
3. Holtz KA, Lipson R, Noonan VK, Kwon BK, Mills PB. Prevalence and effect of problematic spasticity after traumatic spinal cord injury. Arch Phys Med Rehabil. (2017) 98:1132–8. doi: 10.1016/j.apmr.2016.09.124
4. DiPiro ND Li C, Krause JS. A longitudinal study of self-reported spasticity among individuals with chronic spinal cord injury. Spinal Cord. (2018) 56:218–25. doi: 10.1038/s41393-017-0031-5
5. Naro A, Leo A, Russo M, Casella C, Buda A, Crespantini A, et al. Breakthroughs in the spasticity management: Are non-pharmacological treatments the future? J ClinNeurosci. (2017) 39:16–27. doi: 10.1016/j.jocn.2017.02.044
6. Otero-Romero S, Sastre-Garriga J, Comi G, Hartung HP, SoelbergSørensen P, Thompson AJ, et al. Pharmacological management of spasticity in multiple sclerosis: Systematic review and consensus paper. MultScler. (2016) 22:1386–96. doi: 10.1177/1352458516643600
7. Taricco M, Pagliacci MC, Telaro E, Adone R. Pharmacological interventions for spasticity following spinal cord injury: results of a Cochrane systematic review. EuraMedicophys. (2006) 42:5–15.
8. Lui J, Sarai M, Mills PB. Chemodenervation for treatment of limb spasticity following spinal cord injury: a systematic review. Spinal Cord. (2015) 53:252–64. doi: 10.1038/sc.2014.241
9. McIntyre A, Mays R, Mehta S, Janzen S, Townson A, Hsieh J, et al. Examining the effectiveness of intrathecal baclofen on spasticity in individuals with chronic spinal cord injury: a systematic review. J Spinal Cord Med. (2014) 37:11–8. doi: 10.1179/2045772313Y.0000000102
10. Stempien L, Tsai T. Intrathecal baclofen pump use for spasticity: a clinical survey. Am J Phys Med Rehabil. (2000) 79:536–41. doi: 10.1097/00002060-200011000-00010
11. Boviatsis EJ, Kouyialis AT, Korfias S, Sakas DE. Functional outcome of intrathecal baclofen administration for severe spasticity. ClinNeurolNeurosurg. (2005) 107:289–95. doi: 10.1016/j.clineuro.2004.09.007
12. Zahavi A, Geertzen JHB, Staal M, Rietman JS. Long term effect (more than five years) of intrathecal baclofen on impairment, disability and quality of life in patients with severe spasicity of spinal origin. J NeurolNeurosurg Psychiatry. (2004) 75:1553–7. doi: 10.1136/jnnp.2003.014282
13. Dario A, Tomei G. A benefit-risk assessment of baclofen in severe spinal spasticity. Drug Saf. (2004) 27:799–818 doi: 10.2165/00002018-200427110-00004
14. Draulans N, Vermeersch K, Degraeuwe B, Meurrens T, Peers K, Nuttin B, et al. Intrathecal baclofen in multiple sclerosis and spinal cord injury: complications and long-term dosage evolution. ClinRehabil. (2013) 27:1137–43. doi: 10.1177/0269215513488607
15. Stetkarova I, Yablon SA, Kofler M, Stokic DS. Procedure- and device-related complications of intrathecal baclofen administration for management of adult muscle hypertonia: a review. Neurorehabil Neural Repair. (2010) 24:609–19. doi: 10.1177/1545968310363585
16. Stetkarova I, Brabec K, Vaško P, Mencl L. Intrathecal baclofen in spinal spasticity: frequency and severity of withdrawal syndrome. Pain Physician. (2015) 18:E633–41. doi: 10.36076/ppj.2015/18/E
17. Saulino M. Ivanhoe, McGuire JR, Ridley B, Shilt JS, Boster AL. Best practices for intrathecal baclofen therapy: patient selection. Neuromodulation. (2016) 19:607–15. doi: 10.1111/ner.12447
18. Stetkarova I, Krámská L, Keller J. Improvement of memory functions in chronic spinal cordinjury after long-term intrathecal baclofen delivery for spasticity relief. Neuromodulation. (2021) 24:1199–203. doi: 10.1111/ner.13340
19. Kumru H, Stetkarova I, Schindler C, Vidal J, Kofler M. Neurophysiological evidence for muscle tone reduction by intrathecal baclofen at the brainstem level. ClinNeurophysiol. (2011) 122:1229–37. doi: 10.1016/j.clinph.2010.09.010
20. Stetkarova I, Kofler M. Differential effect of baclofen on cortical and spinal inhibitory circuits. Clin Neurophysiol. (2013) 124:339–45 doi: 10.1016/j.clinph.2012.07.005
21. Nasrallah FA, Griffin JL, Balcar VJ, Rae C. Understanding your inhibitions: modulation of brain cortical metabolism by GABA(B) receptors. J Cereb Blood Flow Metab. (2007) 27:1510–20. doi: 10.1038/sj.jcbfm.9600453
22. Guerrera S, Morabito R, Baglieri A, Corallo F, Ciurleo R, De Luca R, et al. Cortical reorganization in multiple sclerosis after intrathecal baclofen therapy. Neurocase. (2014) 20:225–9. doi: 10.1080/13554794.2013.770872
23. Marino RJ, Barros T, Biering-Sorensen F, Burns SP, Donovan WH, Graves DE, et al. International standards for neurological classification of spinal cord injury. J Spinal Cord Med. (2003) 26:S50–S56. doi: 10.1080/10790268.2003.11754575
24. Bohannon RW, Smith MB. Inter-rater reliability of a modified Ashworth scale of muscle spasticity. Phys Ther. (1987) 67:206–7. doi: 10.1093/ptj/67.2.206
25. Meseguer-Henarejos AB, Sánchez-Meca J, López-Pina JA, Carles-Hernández R. Inter- and intra-rater reliability of the Modified Ashworth Scale: a systematic review and meta-analysis. Eur J Phys Rehabil Med. (2018) 54:576–90. doi: 10.23736/S1973-9087.17.04796-7
26. Worsley KJ. Statistical analysis of activation images. In: Jezzard P, Matthews PM, Smith SM (Eds). Functional MRI: An Introduction to Methods OUP. (2001). doi: 10.1093/acprof:oso/9780192630711.003.0014
27. Friston KJ, Worsley KJ, Frackowiak RSJ, Mazziotta JC, Evans AC. Assessing the significance of focal activations using their spatial extent. Hum Brain Mapp. (1994) 1:210–20. doi: 10.1002/hbm.460010306
28. Mohammed H, Hollis ER 2nd. Cortical reorganization of sensorimotor systems and the role of intracortical circuits after spinal cord injury. Neurotherapeutics. (2018). doi: 10.1007/s13311-018-0638-z
29. Kaushal M, Oni-Orisan A, Chen G, Li W, Leschke J, Ward BD, et al. Evaluation of whole-brain resting-state functional connectivity in spinal cord injury: a large-scale network analysis using network-based statistic. J Neurotrauma. (2017) 34:1278–82 doi: 10.1089/neu.2016.4649
30. Matsubayashi K, Nagoshi N, Komaki Y, Kojima K, Shinozaki M, Tsuji O, et al. Assessing cortical plasticity after spinal cord injury by using resting-state functional magnetic resonance imaging in awake adult mice. Sci Rep. (2018) 8:14406. doi: 10.1038/s41598-018-32766-8
31. Solstrand Dahlberg L, Becerra L, Borsook D, Linnman C. Brain changes after spinal cord injury, a quantitative meta-analysis and review. NeurosciBiobehav Rev. (2018) 90:272–93. doi: 10.1016/j.neubiorev.2018.04.018
32. Jurkiewicz MT, Mikulis DJ, Fehlings MG, Verrier MC. Sensorimotor cortical activation in patients with cervical spinal cord injury with persisting paralysis. Neurorehabil Neural Repair. (2010) 24:136–40. doi: 10.1177/1545968309347680
33. Wang W, Xie W, Zhang Q, Liu L, Liu J, Zhou S, et al. Reorganization of the brain in spinal cord injury: a meta-analysis of functional MRI studies. Neuroradiology. (2019) 61:1309–18. doi: 10.1007/s00234-019-02272-3
34. Nakanishi T, Nakagawa K, Kobayashi H, Kudo K, Nakazawa K. Specific brain reorganization underlying superior upper limb motor function after spinal cord injury: a multimodal MRI study. Neurorehabil Neural Repair. (2021) 29:1545968321989347. doi: 10.1177/1545968321989347
35. Min YS, Park JW, Jin SU, Jang KE, Nam HU, Lee YS, et al. Alteration of resting-state brain sensorimotor connectivity following spinal cord injury: a resting-state functional magnetic resonance imaging study. J Neurotrauma. (2015) 32:1422–7. doi: 10.1089/neu.2014.3661
36. Serradj N, Agger SF, Hollis ER 2nd. Corticospinal circuit plasticity in motor rehabilitation from spinal cord injury. Neurosci Lett. (2017) 652:94–104. doi: 10.1016/j.neulet.2016.12.003
37. Hok P, Veverka T, Hluštík P, Nevrlý M, Kanovský P. The central effects of botulinum toxin in dystonia and spasticity. Toxins (Basel). (2021) 13:155. doi: 10.3390/toxins13020155
38. Veverka T, Hok P, Otruba P, Zapletalova O, Kukolova B, Tudos Z, et al. Botulinum toxin modulatesposteriorparietalcortexactivation in post-stroke spasticity oftheupper limb. Front Neurol. (2019) 10:495. doi: 10.3389/fneur.2019.00495
39. Li X, Chen Q, Zheng W, Chen X, Wang L, Qin W, Li K, Lu J, Chen N. Inconsistency between cortical reorganization and functional connectivity alteration in the sensorimotor cortex following incomplete cervical spinal cord injury. Brain Imaging Behav. (2019). doi: 10.1007/s11682-019-00190-9
40. Chen Q, Zheng W, Chen X, Li X, Wang L, Qin W, et al. Reorganization of the somatosensory pathway after subacute incomplete cervical cord injury. Neuroimage Clin. (2019) 21:101674. doi: 10.1016/j.nicl.2019.101674
41. Naftchi NE, Schlosser W, Horst WD. Correlation of changes in the GABA-ergic system with the development of spasticity in paraplegic cats. Adv Exp Med Biol. (1979) 123:431–50. doi: 10.1007/978-1-4899-5199-1_27
42. Kakinohana O, Hefferan MP, Nakamura S, Kakinohana M, Galik J, Tomori Z, et al. Development of GABA-sensitive spasticity and rigidity in rats after transient spinal cord ischemia: a qualitative and quantitative electrophysiological and histopathological study. Neuroscience. (2006) 141(3):1569-83. doi: 10.1016/j.neuroscience.2006.04.083
Keywords: intrathecal baclofen, functional MRI, plasticity, motor cortex activity, spinal cord injury
Citation: Štětkářová I and Keller J (2022) Modulation of Motor Cortex Activity After Intrathecal Baclofen Delivery in Chronic Thoracic Spinal Cord Injury. Front. Neurol. 13:778697. doi: 10.3389/fneur.2022.778697
Received: 17 September 2021; Accepted: 04 April 2022;
Published: 13 May 2022.
Edited by:
Petr Hluštík, Palacký University, CzechiaReviewed by:
Catherine R. Jutzeler, ETH Zürich, SwitzerlandCopyright © 2022 Štětkářová and Keller. This is an open-access article distributed under the terms of the Creative Commons Attribution License (CC BY). The use, distribution or reproduction in other forums is permitted, provided the original author(s) and the copyright owner(s) are credited and that the original publication in this journal is cited, in accordance with accepted academic practice. No use, distribution or reproduction is permitted which does not comply with these terms.
*Correspondence: Ivana Štětkářová, aXZhbmEuc3RldGthcm92YUBmbmt2LmN6
Disclaimer: All claims expressed in this article are solely those of the authors and do not necessarily represent those of their affiliated organizations, or those of the publisher, the editors and the reviewers. Any product that may be evaluated in this article or claim that may be made by its manufacturer is not guaranteed or endorsed by the publisher.
Research integrity at Frontiers
Learn more about the work of our research integrity team to safeguard the quality of each article we publish.