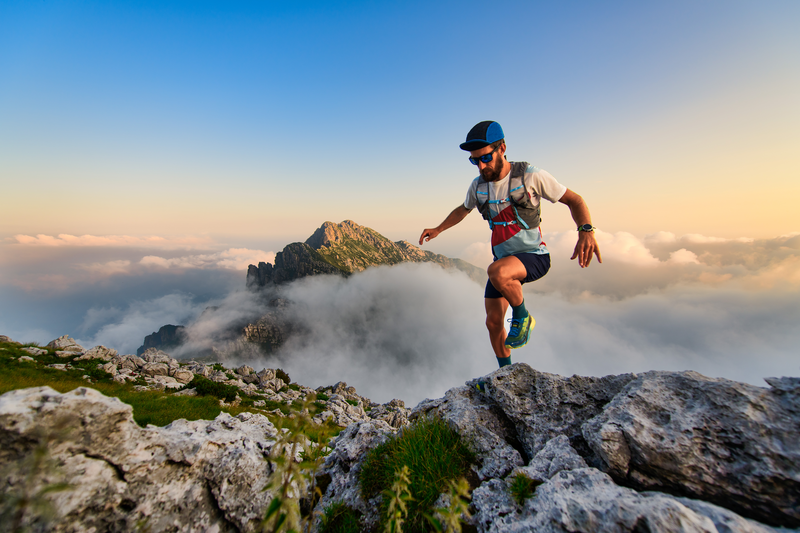
94% of researchers rate our articles as excellent or good
Learn more about the work of our research integrity team to safeguard the quality of each article we publish.
Find out more
REVIEW article
Front. Neurol. , 23 December 2022
Sec. Neuromuscular Disorders and Peripheral Neuropathies
Volume 13 - 2022 | https://doi.org/10.3389/fneur.2022.1049850
This article is part of the Research Topic The expanding clinical and genetic basis of Adult Inherited Neurometabolic Disorders View all 5 articles
Cerebrotendinous Xanthomatosis represents a rare and underdiagnosed inherited neurometabolic disorder due to homozygous or compound heterozygous variants involving the CYP27A1 gene. This bile acid metabolism disorder represents a key potentially treatable neurogenetic condition due to the wide spectrum of neurological presentations in which it most commonly occurs. Cerebellar ataxia, peripheral neuropathy, spastic paraparesis, epilepsy, parkinsonism, cognitive decline, intellectual disability, and neuropsychiatric disturbances represent some of the most common neurological signs observed in this condition. Despite representing key features to increase diagnostic index suspicion, multisystemic involvement does not represent an obligatory feature and can also be under evaluated during diagnostic work-up. Chenodeoxycholic acid represents a well-known successful therapy for this inherited metabolic disease, however its unavailability in several contexts, high costs and common use in patients at late stages of disease course limit more favorable neurological outcomes for most individuals. This review article aims to discuss and highlight the most recent and updated knowledge regarding clinical, pathophysiological, neuroimaging, genetic and therapeutic aspects related to Cerebrotendinous Xanthomatosis.
Cerebrotendinous Xanthomatosis (CTX) or Cerebral Cholesterinosis (MIM #213700) is a rare autosomal recessive inherited metabolic lipid-storage disorder related to bile acid biosynthesis pathways (1). CTX is caused by bi-allelic pathogenic variants in CYP27A1 (2q35), which codes sterol 27-hydroxylase, a mitochondrial enzyme of cytochrome P450 oxidase system. Reduction of the activity of this enzyme leads to increased formation and storage of abnormal lipid content in several tissues, especially tendons, lenses and peripheral, and central nervous system (2).
Several hundreds of cases have been reported since the first description in 1937 by Van Bogaert (3). Current data suggest that the disease appears to be substantially underdiagnosed: the incidence in the United States is between 1:72,000 and 1:150,000, and the disease frequency among Sephardim Jews of Morocco has been estimated to be 6 per 70,000 (4, 5). More than 400 individuals with CTX have been reported worldwide (6), with larger groups of affected individuals being reported in the medical literature from Italy, the Netherlands, Germany, Japan, China, Turkey, Israel, and Spain. CTX incidence ranging from 1:134.970 to 1:461.358 in Europeans, 1:263.222 to 1:468.624 in Africans, 1:71,677 to 1:148,914 in Americans, 1:64,267 to 1:64,712 in East Asians and 1:36,072 to 1:75,601 in South Asians (7).
The aim of the present article is to present current evidence on the main clinical, biochemical, radiologic and treatment aspects of CTX.
CTX is caused by pathogenic variants in CYP27A1, which leads to of deficiency in sterol 27-hydroxylase, a mitochondrial enzyme with a key role in cholesterol metabolism and bile acid synthesis pathways. Multiple variants associated with CTX have been identified, including missense, insertion/deletions, splice-site and nonsense variants, and there is no known clear genotype-phenotype correlation (1, 7).
Bile acid synthesis occurs in two main metabolic pathways. The classical pathway initiates with 7α-hydroxylation of cholesterol, in which the enzyme cholesterol 7α-hydroxylase acts. The alternative pathway's first step is 27-hydroxylation of cholesterol, catalyzed by sterol 27-hydroxylase, leading to oxidation of side chains of different sterol intermediates (8). In CTX, the impaired activity of CYP27A1 compromises the formation of chenodeoxycholic acid (CDCA) and cholic acid, to a lesser extent. The loss of the negative feedback effect of CDCA on cholesterol 7α-hydroxylase results in increased levels of 7α-hydroxy-4-cholesten-3-one and its metabolites in the classical pathway (Figure 1). Elevated serum levels of cholesterol and urine bile acids as glucuronides are found (11). The increased cholesterol metabolites adhere to tissues. Furthermore, there are raised levels of other abnormal pathological intermediates, such as cholestanol. Their accumulation mainly in the brain, eye lenses and tendons cause progressive neurologic dysfunction, cataract and xanthomas, respectively, which are some of the classic clinical manifestations of the disease. However, there is a wide number of phenotypes with diverse systemic and neuropsychiatric symptoms (12).
Figure 1. Biochemical pathways of bile acid metabolism and pathophysiological mechanisms involved in CTX. Both alternative (acidic) and classic (neutral) pathways in liver metabolism are represented in blue color. Primary bile acids (Cholic acid and Chenodeoxycholic acid) are represented in orange. Secondary and tertiary bile salts are showed in red. Brain neurometabolic steps are represented in black squares, as well as molecular targets (receptors) of CDCA and CA (1, 9, 10).
Accumulation of cholestanol in the brain is still not fully understood, since it does not efficiently cross the blood-brain barrier (BBB) (7, 12). Impairment or increased permeability of the BBB has been suggested, possibility endorsed by the high levels of cholestanol and apolipoprotein B found in the cerebrospinal fluid (CSF) in patients with CTX. This change of the BBB may be an effect of circulating bile alcohol glucuronides (1). Nevertheless, some studies have shown an intact BBB in CTX patients, indicating that the increased cholestanol may result from insufficient removal or from synthesis of cholestanol in the brain from cholesterol or another precursor. Furthermore, the bile acid precursor 7α-hydroxy-4-cholesten-3-one crosses the BBB and can be converted to cholestanol by neurons, astrocytes, microglia, and human monocyte-derived macrophages (1, 7) (Figure 1).
The lesions in CTX present significant deposits of cholesterol as well as cholestanol, although serum cholesterol levels are usually normal. However, elevated serum levels of lathosterol and phytosterol are found, indicating increased de novo synthesis and incremented intestinal absorption of cholesterol, respectively (11). Also, regarding the metabolism of cholesterol, patients with CTX develop early atherosclerosis and xanthomas, which might be related to diminished transport of peripheral cholesterol to the liver, since the levels of 27-hydroxycholesterol, the product of the 27-hydroxylase activity that passes more efficiently the cell membranes, are significantly reduced (1, 13). Increased cytoplasmic Nε-carboxymethyl-lysine related to oxidative stress dysfunction has been also evidence in foamy histiocytes from the dentate nucleus (14).
The diagnosis of CTX is mainly based on clinical, neuroradiological, genetic, and biochemical findings. The clinical presentation of the disease is highly heterogeneous, which can lead to significant diagnosis delay. In young people, CTX-related findings are primarily bilateral juvenile cataracts (82%), chronic diarrhea (31%), and intellectual disability (48–74%) (1, 15). In adults, these factors are added to the appearance of tendon xanthomas (76%), as well as psychiatric disturbances (11.4%) and neurological disorders, such as peripheral neuropathy (45%), cerebellar ataxia (36–83%), movement disorders (parkinsonism, dystonia, myoclonus, postural tremor), cognitive decline (87%), and spastic paraparesis and other pyramidal signs (64–92%) (1, 6, 15, 16) (Figure 2).
Figure 2. Natural history, disease progression and symptom and sign timeline in each phenotype of CTX (classical, spinal, and “non-neurological” forms), according to the age of symptom-onset (1, 6, 7, 12, 17, 18).
In childhood, the earliest manifestations may be infantile diarrhea and neonatal cholestatic jaundice. Typically, jaundice is self-limited and transient, without severe complications, and associated with elevated conjugated hyperbilirubinemia, liver transaminases and alkaline phosphatase. Gamma glutamyl transferase serum levels are generally normal or slightly elevated. The marked reduction of CDCA content does not stimulate the activation and expression of farnesoid X receptor, leading to reduced bile salt exportation and transportation in bile canaliculi (1, 19). There are, however, rare descriptions of severe neonatal cholestasis in patients with CTX, leading to very early lethal progression or evolving with the need of liver transplantation (19). Chronic unexplained infancy-onset diarrhea is the most common gastrointestinal scenario (76% of patients) and occurs due to the presence of bile alcohol in the intraluminal region and relative absence of CDCA. Steatorrhea, cholestanol, cholesterol and fatty acids are absent in the stool content, as well as malabsorption and failure to thrive are not usually observed (1). CDCA represents a highly effective therapy for symptomatic diarrhea remission. Bilateral juvenile cataract is also a common finding, being described in 85% of patients (20). During juvenile and adulthood periods, optic neuropathy and premature retinal vessel atherosclerosis may represent additional neuro-ophthalmological complications (1). Neurological signs and tendinous xanthomas often develop after cataracts appear. Xanthomas may be present in 71% of patients with CTX, appearing in the 1st to 3rd decade of life, being more common in late adolescence and early adulthood. They are represented by large amounts of foamy macrophages full filled with complex lipid crystal cleft structures (1, 7, 12). They appear more frequently in the Achilles tendon but can also be identified in the tibial tuberosity, triceps, and fingers tendons (Figure 3). Tendon involvement in inherited metabolic disorders is not limited to xanthomata in CTX and may also occur in familial hypercholesterolaemia type 3 (PCSK9), sitosterolemia (ABCG8), ataxia with vitamin E deficiency (TTPA), hyperlipoproteinemia type III (APOE), primary hypoalphalipoproteinemia type 2 (APOA1), Alagille syndrome (JAG1), and rarely in congenital hypophosphatasia, ochronosis and galactosemia (21). Other typical examination findings in familial hypercholesterolemia, such as corneal arcus and eyelid xanthelasmata, are not observed in CTX.
Figure 3. Clinical examination findings in CTX. Note the presence of tendon xanthoma (white arrows) located in the anterior tibial tuberosity (A) and the Achilles tendon (B). Pes cavus (white arrowhead) is also another possible feature (C).
Neurological dysfunction is almost always present, with onset usually in late adolescence or early adulthood (12) (Figure 2). Psychiatric symptoms (behavioral disorders, depression, hallucinations, agitation), dementia, and intellectual disability can be present (15). Intellectual disability is commonly one of the most common neurological complications in CTX starting during the first decade of life (12). Pyramidal (spasticity and hyperreflexia) and cerebellar signs (progressive ataxia and dysarthria) are frequent. Although less common, movement disorders, such as parkinsonism, dystonia, myoclonus, and tremor have been reported. Dystonia is mostly multifocal, with reports of blepharospasm, oromandibular, cervical, and limb dystonia (22). Both positive and negative myoclonus have been reported as one of the earlier movement disorders features of CTX, mainly involving upper extremities, which can have a polyminimyoclonus pattern, resembling intention, or action tremor (23). Palatal myoclonus with pharyngeal, laryngeal, and lingual involvement may also be present (22). Seizures, peripheral neuropathy (that may be axonal, demyelinating or mixed), motor, or sensorimotor (24, 25) and pes cavus are also possible features (Figure 3). Later, with advancing age, other frequent findings can be observed, such as premature atherosclerosis, osteoporosis, and cardiovascular disease, which include ischemic heart disease, mitral valve insufficiency, abdominal aortic aneurysm, coronary artery dissection, and thickening of the interatrial septum due to lipomatous hypertrophy (1). There are also descriptions of cardiac autonomic dysfunction, ventricular tachycardia, and atrial fibrillation in the disease (7, 26). Osteoporosis represents a challenging chronic complication of CTX and commonly leads to important morbidity, especially in lately diagnosed patients and generally with poor response to CDCA therapy (1, 2, 6, 7). Childhood and juvenile-onset osteoporosis may be also a possible early complication of CTX and probably underdiagnosed during the first decades of life (1, 6).
Despite ataxia is usually considered the main gait disturbance presented by patients with CTX, pyramidal findings are more frequent than cerebellar signs (15) and cases of spinal xanthomatosis, characterized sometimes as pure forms of spastic paraparesis, have been reported in the literature (27–29). A recent literature review on spinal xanthomatosis, reviewed 34 cases, reporting a mean age of onset of the neurological symptoms of 24 years, with most cases presenting with complex hereditary spastic paraplegia (HSP) phenotype, presenting dementia, ataxia, polyneuropathy, seizures, and psychiatric disease as the complicating feature. Interestingly, 23.5% reported patients had spastic paraplegia as the sole neurological phenotype and only 31% of patients with spinal xanthomatosis presented xanthomas. On the other hand, cataracts and chronic diarrhea were frequent features being present in 78 and 65% of cases, respectively (30). Since the report by Burguez et al. (29) the center of one of the authors of the present manuscript (Saute JA) has been screening CYP27A1 in the investigation of patients with HSP suspicion. Among 115 screened families, CTX was diagnosed in six of them, representing 5% of this cohort in southern Brazil (Saute JA personal communication), confirming that HSP phenotype should lead to CTX suspicion with biochemical or genetic screening for the disease.
The presence of two of four clinical hallmarks (premature cataracts, diarrhea, progressive neurologic signs, tendon xanthomas) should trigger comprehensive biochemical testing for CTX (31). Ophthalmologists may notice unexplained bilateral cataracts, which are a common symptom, especially in children and teenagers (32). Given that these are among of the early indications and symptoms, the association of juvenile cataracts and chronic diarrhea is particularly significant (33–35). Additionally, children and adolescents with psychiatric disorders including autism spectrum disorder, attention deficit hyperactivity disorder (ADHD), irritability, aggressive outbursts, or oppositional-defiant disorder should undergo further testing, especially in the context of consanguinity or in the presence of cataracts or chronic diarrhea (36).
In clinical practice, the Mignarri index of suspicion can be used to calculate the CTX prediction score and guide the best diagnostic approach for each individual. This index assigns different scores to certain groups of findings (Table 1): (i) family history, (ii) systemic signs, and (iii) neurological involvement (37). The highest indicator score (100) is attributable to “very strong indicator” (A), including positive family history of a sibling with CTX (A1), and/or presence of tendon xanthomas (A2). “Strong indicator” (B) with an individual score of 50 is given to the presence of consanguineous parents (B1), and/or juvenile-onset cataracts (B2), childhood-onset chronic diarrhea (B3), prolonged unexplained neonatal jaundice (B4), and/or ataxia or spastic paraparesis (B5), dentate nuclei signal changes at brain MRI (B6), and/or intellectual disability or psychiatric disturbances (B7). “Moderate indicator” (C) is attributable to individual score of 25 and given to early osteoporosis (C1), and/or epilepsy (C2), parkinsonism (C3), and polyneuropathy (C4) (7, 37, 38). Plasma cholestanol level assessment is indicated in patients with Mignarri scores ≥100. With previous high levels of plasma cholestanol or a Mignarri score ≥200 (including at least 1 “very strong indicator” or 4 “strong indicator”), there is a formal indication for genetic analysis of CYP27A1 gene (2, 37, 39, 40). The clinical use of the Mignarri score should not limit the early investigation of patients with clinical features highly suggestive of CTX diagnosis (i.e., juvenile cataracts, childhood-onset chronic diarrhea), even in the absence of score values higher than 100 or 200 points or other clinical signs.
Table 1. Mignarri suspicion index for CTX [adapted and modified from (37)].
As for the biochemical characteristics that provide subsidies to aid in the diagnosis, high serum concentrations of cholestanol are the main diagnostic marker of CTX (7). Elevation of other cholesterol precursors, such as 7-dehydrocholesterol and 8-dehydrocholesterol, is also commonly observed in plasma testing in CTX (41). High levels of bile alcohols, such as glucuronides, can be found in bile, plasma, and urine and are biomarkers for CTX. In tissues, cholesterol tends to be increased, while in plasma its concentration is normal or reduced. Other bile acid precursors in plasma and bile (such as lathosterol, lanosterol) are increased. Classical CTX form usually leads to significantly higher plasma levels of cholestanol than atypical forms and spinal CTX. Plasma levels of cholestanol and abnormal intermediates of bile acid synthesis may be elevated also in chronic cholestatic biliary tract diseases, such as Primary biliary cirrhosis and Progressive Familial Intrahepatic Cholestasis type 3 (ABCB4), and in inherited metabolic disorders, such as Niemann-Pick disease type C, sitosterolemia, familial hypercholesterolemia, and peroxisomal biogenesis disorders (1, 6, 42, 43). Drugs which promote abnormal activity of bile acid metabolism, such as intravenous propofol during total anesthesia, may lead to similar metabolic profiles to primary bile acid synthesis disorders (44). There is also important variation in plasma cholestanol levels in different ethnic and age groups, mainly comparing neonatal, childhood and adult (45). Chronic steroid use may reduce plasma cholestanol levels, leading to potential false-negative results and normal values (46), while hypothyroidism may lead to increased levels (1). In CSF analysis, it is possible to find high levels of cholestanol, cholesterol, fragments of apolipoprotein B, apolipoprotein-A1, and albumin (1, 7, 11). Liver biopsy may demonstrate the presence of electrodense deposits dispersed in the cytoplasm and crystal formation (1, 6, 39), although they are not routinely indicated. The quantification of the bile acid precursor 7 alpha-hydroxy-4-cholesten-3-one is being proposed as a rapid and potentially alternative diagnostic test for CTX (7, 12), as well as an optimal therapeutic biomarker during clinical follow-up (47).
Sequencing of CYP27A1 should be performed in all patients with a suspected diagnosis of CTX. Some authors have suggested genetic testing in patients with high cholestanol levels or with very high clinical suspicion (34), but nowadays the accessibility of genetic testing is greater than dosing cholestanol for most centers. Bi-allelic pathogenic variants combined with typical clinical findings are diagnostic of CTX, but variants of unknown significance should always be confirmed with plasma cholestanol analysis (6). As genetic testing methods became more accessible and available for investigation in most centers specialized in Rare Diseases, organizing knowledge about potential clinic-genetic correlations in CTX has become a major challenge in clinical practice (Table 2). The development of specific diagnostic criteria for CTX became an essential measure for diagnostic purposes and future clinical trials (Table 3).
Table 2. Variants described in CYP27A1 gene and the main clinical and genetic correlations associated with CTX (1, 2, 4–7, 9, 17, 18, 20, 29, 48).
Table 3. Diagnostic criteria and categories for CTX, based on Sekijima's (17) and modified from Stelten's criteria (6).
Regarding differential diagnoses, sitosterolemia, familial hypercholesterolemia (both of which can also manifest with tendon xanthomas), Smith-Lemli-Opitz syndrome (characterized by elevated 7-dehydrocholesterol, which may also be present in some CTX patients), other inborn errors of bile acid metabolism (such as HSP type 5A), and non-specific liver disease are among the disorders with features similar to CTX (40, 50–52). Progressive neurologic symptoms, as well as cataracts and chronic diarrhea, can distinguish CTX from these disorders (34, 50). Congenital diarrhea and Alagille syndrome are important differential diagnoses in childhood onset, as well as other causes of neonatal jaundice. In adult patients, differential diagnosis is made with other causes of progressive neurologic disease, such as HSP, hereditary cerebellar ataxias, multiple sclerosis, leukodystrophies, mitochondrial disease, histiocytosis, and other causes of acquired ataxia, and in these cases tendon xanthomas and cataracts are among the most important clues for CTX (53).
The considerable diagnostic delay before the correct diagnosis and proper treatment might be prevented if CTX was included in national newborn screening programs. Newborn screening with dried blood spots is considered by several groups a key step for early diagnosis and treatment (54). However, inclusion in newborn screening programs should be done cautiously and initially in a research context due to the likelihood of detecting mild variants that may remain asymptomatic for a longer period without treatment (6). There is not a definite metabolite or battery tier to perform metabolic or genetic neonatal screening for CTX. However, the most characteristic biomarker in CTX positive newborns after screening was 5-β-cholestane-3α,7α,12α,25-tetrol,3-O-β-D-glucuronide (GlcA-tetrol) (54, 55), and both GlcA-tetrol and the ratio of GlcA-tetrol to tauro-chenodeoxycholic acid from dried blood spots represent the most accurate diagnostic biomarkers for newborn screening (6, 55, 56).
The typical neuroimaging finding of CTX is T2-weighted (T2W) hyperintensity in the dentate nucleus, with cerebellar hypointensity occasionally being seen in the late stage as a result of hemosiderin depositions and microhemorrhages following cerebellar vacuolation (57).
A review article of 38 patients has shown brain MRI abnormalities in 84% of patients, with supra and infratentorial cortical atrophy, subcortical and periventricular white matter abnormalities, brainstem lesions, cerebellar atrophy and other cerebellar parenchymal abnormalities involving the dentate nuclei and the surrounding white matter as the main findings (9). T2W and FLAIR brain MRI may show symmetric hyperintense lesions in the periventricular white matter, posterior limbs of internal capsules, globus pallidum, cerebral peduncles extending into the substantia nigra, anterior region of the pons, inferior olive, or in the cerebellar parenchyma, involving the dentate nuclei and the surrounding white matter, which were hypointense on T1W and diffusion-weighted images (DWI) (7, 40) (Figures 4, 5).
Figure 4. Neuroimaging findings in CTX. (A) Sagittal T1W brain MR imaging (MRI) disclosed mild cerebellar atrophy. (B–G) Axial brain MRI showed hyperintensity involving cerebellar white matter and dentate nuclei (white arrows) in FLAIR (B–E) and T2W imaging (F, G). Axial brain MRI showing mild hypointensity involving cerebellar white matter and dentate nuclei in T1W (H) and SWI sequences (I). Coronal brain MRI showing hyperintensity (white arrow) involving cerebellar white matter and dentate nuclei in T2W imaging (J).
Figure 5. Neuroimaging patterns observed in CTX. (A) Sagittal T1-weighted brain MRI showed hypointense signal in deep cerebellar white matter (white arrow). Axial brain MRI disclosed signal change in deep cerebellar white matter (white arrows) hypointense in T1W (B) and hyperintense in T2W (C) and FLAIR sequences (D). Axial brain MRI disclosed hyperintensity in deep cerebellar white matter in DWI (E) and ADC sequences (F). Coronal brain MRI showed hypointensity in the deep white matter in T1W imaging (G), as well as corresponding hyperintensity (white arrows) in T2W imaging (H).
The dentate nuclei may present with hypointensities on T2W/FLAIR and susceptibility-weighted (SW) images over time. T2W/FLAIR signal abnormalities in the dentate nuclei were the most common findings in patients with CTX (30). The reasons for preferential involvement of the dentate nucleus remain unclear (12, 30). A significant clinical-imaging correlation was only found between the extent of dentate hyperintense lesions and disability expressed by the modified Rankin Scale (30).
The distribution of lesions along corticospinal tracts or in the cerebellum were consistent with the clinical presentation of pyramidal or cerebellar signs, whereas abnormalities of the substantia nigra may be associated with Parkinsonian features (30, 40).
Spinal xanthomatosis may present with non-enhancing long T2W hyperintense lesions predominantly involving the central and posterior cord (Figure 6). One study has found it to have a relatively mild clinical course, compared with the classic form of the disease (30). This case series with 33 patients reported that patients usually presented pyramidal signs and 48% had dorsal column signs. One of the patients presented with late-diagnosed CTX and after treatment discontinuation had psychiatric symptoms and marked spinal xanthomatosis (rare), which manifested as spastic paraparesis in the absence of xanthomas. Spinal MRI revealed new linear hyperintensities of the corticospinal and gracile tracts (30, 57).
Figure 6. Neuroradiological features in spinal CTX. (A) Sagittal brain MRI showed normal cerebellar and corpus callosum structure. Axial brain MRI showed mild hyperintensity in dentate nucleus in FLAIR (B) and T2W sequences (C), as well as hyperintensity in dentate nucleus in coronal T2W imaging (D). Sagittal spine MRI showed hyperintense (white arrows) in T2W imaging (E) and isointense change in the dorsal column of the cervical spinal cord in T1-weighted imaging (F). Sagittal thoracic spine MRI showed normal structure (G). Axial cervical spine MRI showed marked hyperintensity of the dorsal column (white arrows) in T1W (H) and T2W imaging (I–K).
Neuroimaging findings, despite being highly variable among patients, generally disclose features which are rarely reversible in multi-imaging modalities after treatment with CDCA, even in individuals with early diagnosis of CTX (13). There is not, however, a direct correlation between the severity of neurological compromise and the extension of dentate nuclei and white matter involvement, including patients with severe motor compromise and cognitive decline with unremarkable neuroimaging studies. Neuroimaging features are quite similar in both adult and childhood-onset cases, despite a more typical pattern being identified in adult patients (1, 13, 57).
In summary, brain MRI shows diffuse cerebellar atrophy; ex-vacuum dilatation of the IV ventricle; symmetric hyperintensity of the dentate nuclei and of the cerebellar white matter with some associated DWI hypointensities in the adjacent zone; a soft hyperintensity on T2W and FLAIR may be noted in the superior cerebellar peduncles and in the pyramidal tracts; initial signs of cerebral atrophy may also present be with enlargement of both the insular and frontal spaces (13).
Additionally, magnetic resonance spectroscopy (MRS) may reveal typical lipid peaks, increased choline, and decreased N-acetyl-aspartate peaks in the involved regions, which indicates extensive axonal damage and mitochondrial dysfunction (40). Functional dopaminergic studies showed presynaptic denervation, which is consistent with the mild improvement with levodopa in some patients (58, 59).
Usually tendon xanthomas appear as hypo- to isointense on T1W images and showed low to intermediate signal on T2W images. Bilateral Achilles tendons were most frequently involved. CT scans may show soft tissue enlargement with areas of low attenuation. This may be related to abnormal lipid deposition (13, 38, 57).
Generally, neurologic and neuropsychologic evaluations, plasma cholestanol concentration, brain MRI, echocardiography, and total-body bone density should be evaluated annually; more frequent surveillance may be indicated in newly diagnosed patients until biochemical indicators of disease stabilize. Of note, because cholestanol levels may require considerable time to return to a normal range (reduction of 91 μmol/l in an average follow-up of 34 months) after specific treatment initiation, other substrates in the cholestanol pathways, such as intermediate bile alcohols, could be used for short-term follow-up or as surrogate outcomes in clinical trials (7).
In CTX pathophysiology, there is markedly reduced production of bile acids, especially chenodeoxycholic acid (CDCA), and to a lesser extent cholic acid (CA). As CDCA and CA have a negative physiologically feedback on 7-α-hydroxylase, the rate-limiting enzyme of bile acids synthetic pathway, in CTX 7-α-hydroxylase activity is highly enhanced (60). This results in reduced synthesis of CDCA, high production of cholestanol and its subsequent accumulation in different tissues, as well as normal or low levels of cholestanol in plasma and bile alcohols in urine (61). Evidence that cholestanol may be neurotoxic is supported by the finding of cholestanol deposition and apoptosis in neuronal cells, most notably Purkinje cells, in the cerebellum of rats fed a 1% cholestanol diet (62). CDCA also blocks and antagonizes GABAA and NMDA receptors (63) (Figure 1).
Given the reduced synthesis of CDCA and high production of cholestanol, as well as the recent evidence that cholestanol may be neurotoxic, CDCA has become the standard of care for CTX patients. It prevents the accumulation of cholestanol by inhibiting bile acid synthesis through a negative feedback pathway (31). This drastically lowers plasma cholestanol concentrations in patients and its accumulation in tissues. While initial studies with CDCA reported clear short-term clinical benefit in most patients with CTX, long-term studies have rather reported stabilization in some patients (61). In 1984, one of the first studies was published that spoke in favor of long-term benefit from CDCA therapy in outcomes such as decreased serum cholestanol, improved neurological examination and electroencephalographic findings (16). Despite this, there have been no specific randomized placebo-controlled clinical trials of CDCA in patients with CTX to this date.
CDCA treatment typically does not significantly reduce tendon xanthomas or improve cataracts, but can stabilize or improve neurologic manifestations, including cognitive deterioration, pyramidal tract signs, and cerebellar deficits (15). Therefore, given the natural course of CTX, the primary aim of treatment is stabilization or improvement of neurological signs and symptoms based on results of retrospective trials (31).
While most CTX patients do well in response to CDCA therapy, others continue to deteriorate neurologically, especially patients diagnosed over the age of 25 years who already have significant neurologic disease (64). When significant neurologic pathology has occurred, the effect of treatment seems to be limited (15). Early diagnosis and treatment in CTX are imperative to prevent potentially irreversible neurological damage and it changes the disease course in a positive way, alleviating both the neurologic and systemic symptoms of CTX. In a study with 43 CTX patients with a follow-up of 8 years, cognitive impairment (74%), premature cataracts (70%), tendon xanthomas (77%), and neurologic disease (81%) were the most frequent conditions, and treatment with CDCA improved symptoms in 57% of patients, despite of 20% continued to deteriorate (64).
In the largest retrospective cohort study of CDCA treatment with 56 patients, all patients diagnosed and treated before the age of 24 had complete resolution of previous neurologic symptoms and no new onset symptoms, while 61% of patients diagnosed and treated after the age of 24 had neurologic deterioration, with parkinsonism as the main treatment resistant feature (6). These findings suggest that CDCA treatment should be instituted as soon as possible, and that early diagnosis is paramount to good outcomes in this disease (65).
The currently recommended dosing for CDCA ranges from 5 to 15 mg/kg per day in children and 750 mg per day, in three divided doses, in adults. There is a formal recommendation of a slowly progressive dosing introduction with 500 mg per day, for 2 weeks, followed by a weekly increase of 250 mg per day, until the recommended dose is reached (6). If serum cholestanol or urine bile alcohols remain elevated after 3 months, CDCA may be raised up to 1,000 mg per day. For children and adolescents, it is recommended an initial dose of 5 mg/kg per day, in three divided doses. Few specific adverse events or safety concerns have been reported for CTX patients treated with CDCA, with most reports indicating no major adverse events. Discontinuation of therapy due to adverse events occurs in <5% of cases (31). Hepatotoxicity is considered the major concern with CDCA, however in most cases with minor serum aminotransferase elevations (66). Hepatotoxicity leads to the need of dosing adjustment (67), despite in most cases minor serum transaminase elevations (up to three times the upper limit of normality) represent a transient phenomenon with complete resolution in up to 6 months after drug discontinuation. Patients with serum aminotransferase levels over three times the upper limit of normality and evolving with recurrence of such laboratory changes after reintroduction of CDCA may discontinue therapy. CDCA restart is recommended generally at lower initial doses of 5 mg/kg per day and maintained at such dosing with no significant complications (31). If patients evolve with persistent diarrhea or severe gastrointestinal complaints, transient reduction of the recommended dosing is performed until improvement of symptoms, when effective dosage is restarted. CDCA therapy is contraindicated in patients with moderate to severe hepatocyte dysfunction, intrahepatic cholestasis, primary biliary cirrhosis, sclerosing cholangitis, biliary pancreatitis, biliary gastrointestinal fistula, acute cholecystitis or cholangitis, or biliary tract obstruction (31, 64). Patients with absolute contraindication to CDCA use or severe adverse events may potentially benefit from the alternative use of CA (68).
Early reports of combination therapy with low doses of CDCA with HMG-CoA reductase inhibitor pravastatin suggested that this combination reduced plasma levels of cholestanol and avoided the increase in triglyceride and low-density lipoprotein (LDL)-cholesterol with CDCA alone; however, the follow-up period was too short to detect relevant clinical changes (69). Other case reports also favored the combination of CDCA with HMG-CoA reductase inhibitors like simvastatin and atorvastatin, with biochemical response and reports of improvements of peripheral neuropathy and cognitive symptoms when statin was added to CDCA (70, 71). LDL-apheresis with CDCA and HMG-CoA reductase inhibitor is another possible approach, despite the consistent reduction of cholestanol to normal or even subnormal levels, a definite improvement of clinical symptoms was not noted with this aggressive cholestanol lowering therapy (72, 73). CDCA alone presents stronger evidence on clinical outcomes than combined therapy with CDCA and HMG-CoA reductase inhibitor. In a recent consensus statement with Delphi method, the expert panelists considered CDCA alone the preferred first line therapy for CTX, but also considered that combination therapy with HMG-CoA reductase inhibitor improves/stabilizes the prognosis. The panel disagreed that LDL apheresis improves/stabilizes prognosis (6).
Previous studies have also evaluated the possible role of CA therapy in the management of patients with CTX with variable patterns of clinical response, especially regarding neurological involvement (49, 74). Through the suppression of endogenous bile acid biosynthesis by negative feedback mechanisms, similar to CDCA, CA supplementation provided significant reduction in the urinary excretion and serum production of intermediate biomarkers of bile acid biosynthesis pathway (75). A retrospective Franco-Belgian multicentric study evaluated the safety and efficacy of CA in the treatment of both CDCA-naïve and non-naïve patients with CTX (60). More than 80% of individuals had clinical improvement or stabilization and marked reduction of plasma cholestanol levels during treatment period, disclosing a possible role of CA as a second-line or alternative therapy in patients who presented moderate to severe side effects with CDCA therapy (60). There is currently no consensus regarding the use of CA as a monotherapy in CTX (6). Other studies have evaluated the potential role of several other compounds, such as cholestyramine (76), hydrophilic ursodeoxycholic acid (76), ursodeoxycholic acid (76) and, in biochemical and clinical parameters of patients with CTX, however no significant responses or benefits were observed (76).
Finally, preclinical studies showed that single intravenous administration of adeno-associated virus (AAV) expressing CYP27A1 directed to liver provided full metabolic restoration of the disease in a transgenic mice model of CTX in a greater extent than CDCA (77), being a promising therapeutic option that should be pursued by future studies.
Age at definite diagnosis and treatment introduction as early as possible represents the most important prognostic factors related to treatment responses and outcomes, especially in asymptomatic patients or individuals without significant neuropsychiatric involvement (1, 6, 7). Progressive reduction of plasma cholestanol levels after treatment initiation leads to slowing clinical progression of CTX (6). Patients with typical brain MRI involvement of the deep cerebellar white matter with vacuolation seen as hypointense on T1-weighted and FLAIR sequences generally evolve with worse prognosis (60), as well as the absence of dentate nuclei signal changes is generally associated with better prognosis (6). Some CYP27A1 pathogenic variants have been also associated with more severe neurological and multisystemic involvement and then worse prognosis (Table 2). As CDCA represents a specific drug therapy developed for continuous use, long-term treatment availability for initiation and maintenance represents a key issue for better neurological and systemic outcomes and increased life expectancy (6).
CTX is a rare and potentially treatable genetic disease that results in multisystemic involvement. The main cause of disability results from neurologic manifestations including pyramidal signs, ataxia, and cognitive impairment. Neuroimaging with typical T2W hyperintensity in the dentate nucleus, bilateral juvenile cataracts and the presence of tendon xanthomas are important clues for diagnosis. Treatment with CDCA is safe and appears to be effective based on results of retrospective studies, especially if initiated early. Prompt diagnosis, possibly with neonatal screening, may significantly reduce the burden of this disease.
PN and AB: conception and design, acquisition of data, analysis and interpretation of data, writing of the first draft, review and critique, and final approval of the version. RR, SV, DA, VG, HF, CS, DD, AP, and WP: conception and design, analysis and interpretation of data, writing of the first draft, review and critique, and final approval of the version. JS, PS, and PB-N: conception and design, acquisition of data, writing of the first draft, review and critique, and final approval of the version. All authors contributed to the article and approved the submitted version.
The authors declare that the research was conducted in the absence of any commercial or financial relationships that could be construed as a potential conflict of interest.
All claims expressed in this article are solely those of the authors and do not necessarily represent those of their affiliated organizations, or those of the publisher, the editors and the reviewers. Any product that may be evaluated in this article, or claim that may be made by its manufacturer, is not guaranteed or endorsed by the publisher.
1. Koyama S, Sekijima Y, Ogura M, Hori M, Matsuki K, Miida T, et al. Cerebrotendinous Xanthomatosis: molecular pathogenesis, clinical spectrum, diagnosis, and disease-modifying treatments. J Atheroscler Thromb. (2021) 28:905–25. doi: 10.5551/jat.RV17055
2. Islam M, Hoggard N, Hadjivassiliou M. Cerebrotendinous Xanthomatosis: diversity of presentation and refining treatment with chenodeoxycholic acid. Cerebellum Ataxias. (2021) 8:1–7. doi: 10.1186/s40673-021-00128-2
3. Van Bogaert L, Scherer HJ, Froehlich A, Epstein E. Une deuxieme observation de cholesterinose tendineuse avec symptomes cerebraux. Ann Med. (1937) 42:69–101.
4. Berginer VM, Abeliovich D. Genetics of Cerebrotendinous Xanthomatosis (CTX): an autosomal recessive trait with high gene frequency in sephardim of Moroccan origin. Am J Med Genet. (1981) 10:151–7. doi: 10.1002/ajmg.1320100209
5. Appadurai V, DeBarber A, Chiang P-W, Patel SB, Steiner RD, Tyler C, et al. Apparent underdiagnosis of Cerebrotendinous Xanthomatosis revealed by analysis of ~60,000 human exomes. Mol Genet Metab. (2015) 116:298–304. doi: 10.1016/j.ymgme.2015.10.010
6. Stelten BML, Dotti MT, Verrips A, Elibol B, Falik-Zaccai TC, Hanman K, et al. Expert opinion on diagnosing, treating and managing patients with Cerebrotendinous Xanthomatosis (CTX): a modified Delphi study. Orphanet J Rare Dis. (2021) 16:353. doi: 10.1186/s13023-021-01980-5
7. Salen G, Steiner RD. Epidemiology, diagnosis, and treatment of Cerebrotendinous Xanthomatosis (CTX). J Inherit Metab Dis. (2017) 40:771–81. doi: 10.1007/s10545-017-0093-8
8. Mast N, Anderson KW, Lin JB Li Y, Turko IV, Tatsuoka C, et al. Cytochrome P450 27A1 deficiency and regional differences in brain sterol metabolism cause preferential cholestanol accumulation in the cerebellum. J Biol Chem. (2017) 292:4913–24. doi: 10.1074/jbc.M116.774760
9. Nie S, Chen G, Cao X, Zhang Y. Cerebrotendinous Xanthomatosis: a comprehensive review of pathogenesis, clinical manifestations, diagnosis, and management. Orphanet J Rare Dis. (2014) 9:179. doi: 10.1186/s13023-014-0179-4
10. Monteiro-Cardoso VF, Corlianò M, Singaraja RR. Bile acids: a communication channel in the gut-brain axis. Neuromolecular Med. (2021) 23:99–117. doi: 10.1007/s12017-020-08625-z
11. Mignarri A, Magni A, Del Puppo M, Gallus GN, Björkhem I, Federico A, et al. Evaluation of cholesterol metabolism in Cerebrotendinous Xanthomatosis. J Inherit Metab Dis. (2016) 39:75–83. doi: 10.1007/s10545-015-9873-1
12. Wong JC, Walsh K, Hayden D, Eichler FS. Natural history of neurological abnormalities in Cerebrotendinous Xanthomatosis. J Inherit Metab Dis. (2018) 41:647–56. doi: 10.1007/s10545-018-0152-9
13. Orabona GDA, Dato C, Oliva M, Ugga L, Dotti MT, Fratta M, et al. Multi-imaging study in a patient with Cerebrotendinous Xanthomatosis: radiology, clinic and pathology correlation of a rare condition. BJR Case Rep. (2020) 6:20190047. doi: 10.1259/bjrcr.20190047
14. Gonzalez-Cuyar LF, Hunter B, Harris PLR, Perry G, Smith MA, Castellani RJ. Cerebrotendinous Xanthomatosis: case report with evidence of oxidative stress. Redox Rep. (2007) 12:119–24. doi: 10.1179/135100007X200173
15. Stelten BML, Huidekoper HH, Van De Warrenburg BPC, Brilstra EH, Hollak CEM, Haak HR, et al. Long-term treatment effect in Cerebrotendinous Xanthomatosis depends on age at treatment start. Neurology. (2019) 92:e83–95. doi: 10.1212/WNL.0000000000006731
16. Berginer VM, Foster NL, Sadowsky M, Townsend JA III, Siegel GJ, Salen G. Psychiatric disorders in patients with Cerebrotendinous Xanthomatosis. Am J Psychiatry. (1988) 145:354–7. doi: 10.1176/ajp.145.3.354
17. Sekijima Y, Koyama S, Yoshinaga T, Koinuma M, Inaba Y. Nationwide survey on cerebrotendinous xanthomatosis in Japan. J Hum Genet. (2018) 63:271–80. doi: 10.1038/s10038-017-0389-4
18. Cao LX, Yang M, Liu Y, Long WY, Zhao GH. Chinese patient with Cerebrotendinous Xanthomatosis confirmed by genetic testing: a case report and literature review. World J Clin Cases. (2020) 8:5446–56. doi: 10.12998/wjcc.v8.i21.5446
19. Gong JY, Setchell KDR, Zhao J, Zhang W, Wolfe B, Lu Y, et al. Severe neonatal cholestasis in Cerebrotendinous Xanthomatosis: genetics, immunostaining, mass spectrometry. J Pediatr Gastroenterol Nutr. (2017) 65:561–8. doi: 10.1097/MPG.0000000000001730
20. Gallus GN, Dotti MT, Federico A. Clinical and molecular diagnosis of Cerebrotendinous Xanthomatosis with a review of the mutations in the CYP27A1 gene. Neurol Sci. (2006) 27:143–9. doi: 10.1007/s10072-006-0618-7
21. Abate M, Salini V, Andia I. Tendons involvement in congenital metabolic disorders. Adv Exp Med Biol. (2016) 920:117–22. doi: 10.1007/978-3-319-33943-6_10
22. Stelten BML, van de Warrenburg BPC, Wevers RA, Verrips A. Movement disorders in Cerebrotendinous Xanthomatosis. Parkinsonism Relat Disord. (2019) 58:12–6. doi: 10.1016/j.parkreldis.2018.07.006
23. Aktuglu Zeybek C, Gunduz A, Enver EO, Tezen D, Kiykim E, Kiziltan ME. Movement disorders in the early-diagnosed Cerebrotendinous Xanthomatosis: an electrophysiological study. Parkinsonism Relat Disord. (2020) 80:12–4. doi: 10.1016/j.parkreldis.2020.09.002
24. Pilo B, Blas G, Sobrido MJ, Navarro C, Grandas F, Barrero FJ, et al. Neurophysiological study in Cerebrotendinous Xanthomatosis. Muscle Nerve. (2011) 43:531–6. doi: 10.1002/mus.21905
25. Pedroso JL, Pinto WB, Souza PV, Santos LT, Abud IC, Avelino MA, et al. Early-onset epilepsy as the main neurological manifestation of Cerebrotendinous Xanthomatosis. Epilepsy Behav. (2012) 24:380–1. doi: 10.1016/j.yebeh.2012.04.121
26. Rossi M, Wainsztein N, Merello M. Cardiac involvement in movement disorders. Mov Disord Clin Pract. (2021) 8:651–68. doi: 10.1002/mdc3.13188
27. Verrips A, Nijeholt GJ, Barkhof F, Van Engelen BG, Wesseling P, Luyten JA, et al. Spinal xanthomatosis: a variant of Cerebrotendinous Xanthomatosis. Brain. (1999) 122(Pt 8):1589–95. doi: 10.1093/brain/122.8.1589
28. Saute JA, Giugliani R, Merkens LS, Chiang JPW, DeBarber AE, de Souza CFM. Look carefully to the heels! a potentially treatable cause of spastic paraplegia. J Inherit Metab Dis. (2015) 38:363–4. doi: 10.1007/s10545-014-9745-0
29. Burguez D, Polese-Bonatto M, Scudeiro LAJ, Björkhem I, Schöls L, Jardim LB, et al. Clinical and molecular characterization of hereditary spastic paraplegias: a next-generation sequencing panel approach. J Neurol Sci. (2017) 383:18–25. doi: 10.1016/j.jns.2017.10.010
30. Atallah I, San Millán D, Benoît W, Campos-Xavier B, Superti-Furga A, Tran C. Spinal Cerebrotendinous Xanthomatosis: a case report and literature review. Mol Genet Metab Rep. (2021) 26:100719. doi: 10.1016/j.ymgmr.2021.100719
31. Verrips A, Dotti MT, Mignarri A, Stelten BML, Verma S, Federico A. The safety and effectiveness of chenodeoxycholic acid treatment in patients with Cerebrotendinous Xanthomatosis: two retrospective cohort studies. Neurol Sci. (2020) 41:943–9. doi: 10.1007/s10072-019-04169-8
32. Cruysberg J, Wevers R, Van Engelen B, Pinckers A, van Spreeken A, Tolboom J. Ocular and systemic manifestations of Cerebrotendinous Xanthomatosis. Am J Ophthalmol. (1995) 120:597–604. doi: 10.1016/S0002-9394(14)72206-8
33. Cruysberg JR, Wevers RA, Tolboom JJ. Juvenile cataract associated with chronic diarrhea in pediatric Cerebrotendinous Xanthomatosis. Am J Ophthalmol. (1991) 112:606–7. doi: 10.1016/S0002-9394(14)76874-6
34. Berginer VM, Gross B, Morad K, Kfir N, Morkos S, Aaref S, et al. Chronic diarrhea and juvenile cataracts: think Cerebrotendinous Xanthomatosis and treat. Pediatrics. (2009) 123:143–7. doi: 10.1542/peds.2008-0192
35. Degos B, Nadjar Y, Amador MM, Lamari F, Sedel F, Roze E, et al. Natural history of Cerebrotendinous Xanthomatosis: a paediatric disease diagnosed in adulthood. Orphanet J Rare Dis. (2016) 11:41. doi: 10.1186/s13023-016-0419-x
36. Fraidakis MJ. Psychiatric manifestations in Cerebrotendinous Xanthomatosis. Transl Psychiatry. (2013) 3:e302. doi: 10.1038/tp.2013.76
37. Mignarri A, Gallus GN, Dotti MT, Federico A. A suspicion index for early diagnosis and treatment of Cerebrotendinous Xanthomatosis. J Inherit Metab Dis. (2014) 37:421–9. doi: 10.1007/s10545-013-9674-3
38. Makary MS, Kisanuki YY, Amin NN, Slone HW. Teaching neuroimages: Cerebrotendinous Xanthomatosis: a rare treatable adult-onset lipid storage disease. Neurology. (2018) 90:e637–8. doi: 10.1212/WNL.0000000000004967
39. Kisa PT, Yildirim GK, Hismi BO, Dorum S, Kusbeci OY, Topak A, et al. Patients with Cerebrotendinous Xanthomatosis diagnosed with diverse multisystem involvement. Metab Brain Dis. (2021) 36:1201–11. doi: 10.1007/s11011-021-00714-7
40. Ma C, Ren Y-D, Wang J-C, Wang C-J, Zhao J-P, Zhou T, et al. The clinical and imaging features of Cerebrotendinous Xanthomatosis: a case report and review of the literature. Medicine. (2021) 100:e24687. doi: 10.1097/MD.0000000000024687
41. Sain-van der Velden MGM, Verrips A, Prinsen BHCMT, Barse M, Berger R, Visser G. Elevated cholesterol precursors other than cholestanol can also be a hallmark for CTX. J Inherit Metab Dis. (2008) 31(Suppl. 2):S387–93. doi: 10.1007/s10545-008-0963-1
42. Porter FD, Scherrer DE, Lanier MH, Langmade SJ, Molugu V, Gale SE, et al. Cholesterol oxidation products are sensitive and specific blood-based biomarkers for Niemann-Pick C1 disease. Sci Transl Med. (2010) 2:56ra81. doi: 10.1126/scitranslmed.3001417
43. Yavas AK, Çavdarli B, Uzun ÖÜ, Uncuoglu A, Gündüz M. A novel etiologic factor of highly elevated cholestanol levels: progressive familial intrahepatic cholestasis. J Pediatr Endocrinol Metab. (2020) 33:665–9. doi: 10.1515/jpem-2019-0314
44. Claesen JLA, Koomen E, Schene IF, Jans JJM, Mast N, Pikuleva IA, et al. Misdiagnosis of CTX due to propofol: the interference of total intravenous propofol anesthesia with bile acid profiling. J Inherit Metab Dis. (2020) 43:843–51. doi: 10.1002/jimd.12219
45. Gelzo M, Di Taranto MD, Sica C, Boscia A, Papagni F, Fortunato G, et al. Age-related changes of cholestanol and lathosterol plasma concentrations: an explorative study. Lipids Health Dis. (2019) 18:235. doi: 10.1186/s12944-019-1176-3
46. Siman-Tov T, Meiner V, Gadoth N. Could steroids mask the diagnosis of Cerebrotendinous Xanthomatosis? J Neurol Sci. (2006) 243:83–6. doi: 10.1016/j.jns.2005.10.019
47. Lütjohann D, Stellaard F, Björkhem I. Levels of 7alpha-hydroxycholesterol and/or 7alpha-hydroxy-4-cholest-3-one are the optimal biochemical markers for the evaluation of treatment of cerebrotendinous Xanthomatosis. J Neurol. (2020) 267:572–3. doi: 10.1007/s00415-019-09650-0
48. Pavanello S, Angelici L, Hoxha M, Cantone L, Campisi M, Tirelli AS, et al. Sterol 27-hydroxylase polymorphism significantly associates with shorter telomere, higher cardiovascular and type-2 diabetes risk in obese subjects. Front Endocrinol. (2018) 9:309. doi: 10.3389/fendo.2018.00309
49. Polak Y, Jacobs BAW, Kemper EM. Pharmacy compounded medicines for patients with rare diseases: lessons learned from chenodeoxycholic acid and cholic acid. Front Pharmacol. (2021) 12:758210. doi: 10.3389/fphar.2021.758210
50. Moghadasian MH, Salen G, Frohlich JJ, Scudamore CH. Cerebrotendinous Xanthomatosis: a rare disease with diverse manifestations. Arch Neurol. (2002) 59:527–9. doi: 10.1001/archneur.59.4.527
51. Björkhem I, Diczfalusy U, Lövgren-Sandblom A, Starck L, Jonsson M, Tallman K, et al. On the formation of 7-ketocholesterol from 7-dehydrocholesterol in patients with CTX and SLO. J Lipid Res. (2014) 55:1165–72. doi: 10.1194/jlr.P048603
52. Pedley TA, Emerson RG, Warner CL, Rowland LP, Salen G. Treatment of Cerebrotendinous Xanthomatosis with chenodeoxycholic acid. Ann Neurol. (1985) 18:517–8. doi: 10.1002/ana.410180418
53. Teive HAG, Camargo CHF, Pereira ER, Coutinho L, Munhoz RP. Inherited metabolic diseases mimicking hereditary spastic paraplegia (HSP): a chance for treatment. Neurogenetics. (2022) 23:167–77. doi: 10.1007/s10048-022-00688-3
54. DeBarber AE, Kalfon L, Fedida A, Sheffer VF, Ben Haroush S, Chasnyk N, et al. Newborn screening for Cerebrotendinous Xanthomatosis is the solution for early identification and treatment. J Lipid Res. (2018) 59:2214–22. doi: 10.1194/jlr.M087999
55. Hong X, Daiker J, Sadilek M, DeBarber AE, Chiang J, Duan J, et al. Toward newborn screening of Cerebrotendinous Xanthomatosis: results of a biomarker research study using 32,000 newborn dried blood spots. Genet Med. (2020) 22:1606–12. doi: 10.1038/s41436-020-0846-x
56. Vaz FM, Bootsma AH, Kulik W, Verrips A, Wevers RA, Schielen PC, et al. A newborn screening method for Cerebrotendinous Xanthomatosis using bile alcohol glucuronides and metabolite ratios. J Lipid Res. (2017) 58:1002–7. doi: 10.1194/jlr.P075051
57. Zhang Y, Sun Y-M, Li H. Teaching neuroimage: symmetric deep cerebellar white matter T2 and susceptibility-weighted imaging hypointense lesions in a case of Cerebrotendinous Xanthomatosis. Neurology. (2021) 97:e968–9. doi: 10.1212/WNL.0000000000012154
58. Mignarri A, Falcini M, Vella A, Giorgio A, Gallus GN, Del Puppo M, et al. Parkinsonism as neurological presentation of late-onset Cerebrotendinous Xanthomatosis. Parkinsonism Relat Disord. (2012) 18:99–101. doi: 10.1016/j.parkreldis.2011.06.004
59. Rubio-Agusti I, Kojovic M, Edwards MJ, Murphy E, Chandrashekar HS, Lachmann RH, et al. Atypical parkinsonism and Cerebrotendinous Xanthomatosis: report of a family with corticobasal syndrome and a literature review. Mov Disord. (2012) 27:1769–74. doi: 10.1002/mds.25229
60. Mandia D, Chaussenot A, Besson G, Lamari F, Castelnovo G, Curot J, et al. Cholic acid as a treatment for Cerebrotendinous Xanthomatosis in adults. J Neurol. (2019) 266:2043–50. doi: 10.1007/s00415-019-09377-y
61. Amador MDM, Masingue M, Debs R, Lamari F, Perlbarg V, Roze E, et al. Treatment with chenodeoxycholic acid in Cerebrotendinous Xanthomatosis: clinical, neurophysiological, and quantitative brain structural outcomes. J Inherit Metab Dis. (2018) 41:799–807. doi: 10.1007/s10545-018-0162-7
62. Inoue K, Kubota S, Seyama Y. Cholestanol induces apoptosis of cerebellar neuronal cells. Biochem Biophys Res Commun. (1999) 256:198–203. doi: 10.1006/bbrc.1998.9497
63. Schubring SR, Fleischer W, Lin JS, Haas HL, Sergeeva OA. The bile steroid chenodeoxycholate is a potent antagonist at NMDA and GABA(A) receptors. Neurosci Lett. (2012) 506:322–6. doi: 10.1016/j.neulet.2011.11.036
64. Duell PB, Salen G, Eichler FS, DeBarber AE, Connor SL, Casaday L, et al. Diagnosis, treatment, and clinical outcomes in 43 cases with Cerebrotendinous Xanthomatosis. J Clin Lipidol. (2018) 12:1169–78. doi: 10.1016/j.jacl.2018.06.008
65. Stelten BML, Nijeholt GJLA, Hendriks E, Kluijtmans LAJ, Wevers RA, Verrips A. Long-term MRI findings in patients with Cerebrotendinous Xanthomatosis treated with chenodeoxycholic acid. Neurology. (2022) 99:559–66. doi: 10.1212/WNL.0000000000201112
66. Huidekoper HH, Vaz FM, Verrips A, Bosch AM. Hepatotoxicity due to chenodeoxycholic acid supplementation in an infant with Cerebrotendinous Xanthomatosis: implications for treatment. Eur J Pediatr. (2016) 175:143–6. doi: 10.1007/s00431-015-2584-7
67. Yahalom G, Tsabari R, Molshatzki N, Ephraty L, Cohen H, Hassin-Baer S. Neurological outcome in Cerebrotendinous Xanthomatosis treated with chenodeoxycholic acid: early versus late diagnosis. Clin Neuropharmacol. (2013) 36:78–83. doi: 10.1097/WNF.0b013e318288076a
68. Bartholdi D, Zumsteg D, Verrips A, Wevers RA, Sistermans E, Hess K, et al. Spinal phenotype of Cerebrotendinous Xanthomatosis – a pitfall in the diagnosis of multiple sclerosis. J Neurol. (2004) 251:105–7. doi: 10.1007/s00415-004-0221-x
69. Kuriyama M, Tokimura Y, Fujiyama J, Utatsu Y, Osame M. Treatment of cerebrotendinous xanthomatosis: effects of chenodeoxycholic acid, pravastatin, and combined use. J Neurol Sci. (1994) 125:22–8. doi: 10.1016/0022-510X(94)90237-2
70. Verrips A, Wevers RA, Van Engelen BG, Keyser A, Wolthers BG, Barkhof F, et al. Effect of simvastatin in addition to chenodeoxycholic acid in patients with Cerebrotendinous Xanthomatosis. Metabolism. (1999) 48:233–8. doi: 10.1016/S0026-0495(99)90040-9
71. Luyckx E, Eyskens F, Simons A, Beckx K, Van West D, Dhar M. Long-term follow-up on the effect of combined therapy of bile acids and statins in the treatment of Cerebrotendinous Xanthomatosis: a case report. Clin Neurol Neurosurg. (2014) 118:9–11. doi: 10.1016/j.clineuro.2013.12.008
72. Ito S, Kuwabara S, Sakakibara R, Oki T, Arai H, Oda S, et al. Combined treatment with LDL-apheresis, chenodeoxycholic acid and HMG-CoA reductase inhibitor for Cerebrotendinous Xanthomatosis. J Neurol Sci. (2003) 216:179–82. doi: 10.1016/j.jns.2003.07.005
73. Dotti MT, Lütjohann D, von Bergmann K, Federico A. Normalisation of serum cholestanol concentration in a patient with Cerebrotendinous Xanthomatosis by combined treatment with chenodeoxycholic acid, simvastatin and LDL apheresis. Neurol Sci. (2004) 25:185–91. doi: 10.1007/s10072-004-0320-6
74. Pierre G, Setchell K, Blyth J, Preece MA, Chakrapani A, McKiernan P. Prospective treatment of Cerebrotendinous Xanthomatosis with cholic acid therapy. J Inherit Metab Dis. (2008) 31:S241–5. doi: 10.1007/s10545-008-0815-z
75. Koopman BJ, Wolthers BG, van der Molen JC, Waterreus RJ. Bile acid therapies applied to patients suffering from Cerebrotendinous Xanthomatosis. Clin Chim Acta. (1985) 152:115–22. doi: 10.1016/0009-8981(85)90182-2
76. Batta AK, Salen G, Tint GS. Hydrophilic 7 beta-hydroxy bile acids, lovastatin, and cholestyramine are ineffective in the treatment of Cerebrotendinous Xanthomatosis. Metabolism. (2004) 53:556–62. doi: 10.1016/j.metabol.2003.12.003
77. Lumbreras S, Ricobaraza A, Baila-Rueda L, Gonzalez-Aparicio M, Mora-Jimenez L, Uriarte I, et al. Gene supplementation of CYP27A1 in the liver restores bile acid metabolism in a mouse model of Cerebrotendinous Xanthomatosis. Mol Ther Methods Clin Dev. (2021) 22:210–21. doi: 10.1016/j.omtm.2021.07.002
Keywords: Cerebrotendinous Xanthomatosis, lipid storage disease, CYP27A1, chenodeoxycholic acid, inherited metabolic disorders, inborn errors of metabolism
Citation: Nóbrega PR, Bernardes AM, Ribeiro RM, Vasconcelos SC, Araújo DABS, Gama VCV, Fussiger H, Santos CF, Dias DA, Pessoa ALS, Pinto WBVR, Saute JAM, Souza PVS and Braga-Neto P (2022) Cerebrotendinous Xanthomatosis: A practice review of pathophysiology, diagnosis, and treatment. Front. Neurol. 13:1049850. doi: 10.3389/fneur.2022.1049850
Received: 21 September 2022; Accepted: 08 December 2022;
Published: 23 December 2022.
Edited by:
Mamede De Carvalho, University of Lisbon, PortugalReviewed by:
Radhika Dhamija, Mayo Clinic, United StatesCopyright © 2022 Nóbrega, Bernardes, Ribeiro, Vasconcelos, Araújo, Gama, Fussiger, Santos, Dias, Pessoa, Pinto, Saute, Souza and Braga-Neto. This is an open-access article distributed under the terms of the Creative Commons Attribution License (CC BY). The use, distribution or reproduction in other forums is permitted, provided the original author(s) and the copyright owner(s) are credited and that the original publication in this journal is cited, in accordance with accepted academic practice. No use, distribution or reproduction is permitted which does not comply with these terms.
*Correspondence: Paulo Victor Sgobbi de Souza, cHZzZ29iYmlAZ21haWwuY29t
†These authors have contributed equally to this work
Disclaimer: All claims expressed in this article are solely those of the authors and do not necessarily represent those of their affiliated organizations, or those of the publisher, the editors and the reviewers. Any product that may be evaluated in this article or claim that may be made by its manufacturer is not guaranteed or endorsed by the publisher.
Research integrity at Frontiers
Learn more about the work of our research integrity team to safeguard the quality of each article we publish.