- 1Department of Otorhinolaryngology-Head and Neck Surgery, Xinhua Hospital, Shanghai Jiaotong University School of Medicine, Shanghai, China
- 2Shanghai Jiaotong University School of Medicine Ear Institute, Shanghai, China
- 3Shanghai Key Laboratory of Translational Medicine on Ear and Nose Diseases, Shanghai, China
Hearing loss affecting about 2/1000 newborns is the most common congenital disease. Genetic defects caused approximately 70% of patients who have non-syndromic hearing loss. We recruited 13 Chinese Han deafness families who tested negative for GJB2, SLC26A4, and mitochondrial 12S rRNA. The probands of each family were performed whole-exome sequencing (WES) or targeted next-generation sequencing (NGS) for known deafness genes to study for pathogenic causes. We found four novel mutations of CDH23, one novel mutation of MYO15A, one novel mutation of TMC1, one novel mutation of PAX3, and one novel mutation of ADGRV1, one novel CNV of ADGRV1, and one novel CNV of STRC. Hearing loss is a highly hereditary and heterogeneous disease. The results in the limited samples of this study show that Usher and Waardenburg syndrome-related genes account for a major proportion are strongly associated with Chinese Han hearing loss patients negative for GJB2, SLC26A4, and mitochondrial 12S rRNA, followed by STRC resulting in mild to moderate deafness.
Introduction
Hearing loss is the most common congenital disease affecting about 2/1,000 newborns (1). Approximately 70% of non-syndromic hearing loss is caused by genetic defects (2). Autosomal recessive non-syndromic deafness, the most common form of hearing loss, is usually pre-lingual and accounts for 80% of non-syndromic hereditary deafness. Autosomal dominant non-syndromic deafness, which is often post-lingual, accounts for the remaining 20% (3). Mitochondria and X-linked inheritance account for only 1–2% of non-syndromic deafness. About 30% of genetic deafness is associated with about 700 symptoms described to date, leading to syndromic deafness (4). To date, more than 44 syndromic deafness genes and 100 non-syndromic deafness genes have been mapped (http://hereditaryhearingloss.org).
Currently, next-generation sequencing (NGS) is increasingly applied in clinics to enable accurate diagnosis. In this study, we recruited 13 Chinese Han deafness families negative for GJB2, SLC26A4 and mitochondrial 12S rRNA. The probands of each family were performed targeted NGS for known hearing loss genes or whole-exome sequencing (WES) to study for pathogenic causes.
Subjects and methods
Subjects collection and audiological evaluations
Thirteen patients (HL1~13) were recruited from the Department of Otorhinolaryngology-Head and Neck Surgery of Xinhua Hospital affiliated with Shanghai Jiaotong University School of Medicine. Informed consent was approved from all subjects to participate in this study from October 1, 2018 to December 31, 2020. For child participants, written consent will be sought from their parents or guardians. All patients had a detailed medical history and a thorough examination to rule out noise, trauma, pregnancy infection, and other non-genetic factors. All affected subjects were evaluated by audiological examinations, including otoscopy, pure-tone audiometry (PTA), distortion product otoacoustic emissions (DPOAEs), and auditory brainstem response (ABR). Magnetic resonance imaging (MRI) was performed on the HL13 proband. The research was approved by the Ethics Committee of Xinhua Hospital affiliated to Shanghai Jiaotong University School of Medicine (No. XHEC-D-2021-060).
Targeted NGS
Genomic DNA of all family members was extracted from whole peripheral blood leukocytes. Using polymerase chain reaction (PCR) amplification, GJB2, SLC26A4, and the mitochondrial 12S rRNA exon were directly sequenced first in 13 probands. A panel of 415 hearing loss–related genes was performed by targeted NGS in 12 probands excluding HL6 (Supplementary Table 1). Data processing including targeted gene capturing, filtering of multiple databases for variations, and bioinformation analysis was previously reported in detail (5). Potential causative mutations, which were detected by targeted NGS, were identified for each proband using Sanger sequencing. Where possible, a co-segregation analysis of all family members was also conducted.
Whole exome sequencing
The whole exome sequence of the HL6 proband was sequenced in the Illumina platform by the NextSeq500 sequencer, and the obtained reads by whole exome sequence were mapped to the human genome reference sequence hg19.
SNP arrays
We used SNP arrays to detect the chromosomal regions of CNV identified by targeted NGS in the HL5. SNP arrays were performed as previously reported in detail (5).
Multiplex ligation-dependent probe amplification
The SALSA® MLPA® probe mixes P461-A1 DIS (MRC-Holland, Amsterdam, The Netherlands) was used to identify deletion/duplication of STRC-CATSPER2 in HL6 and HL7 family members, according to the manufacturer's instructions. The PCR amplification products were analyzed on ABI 3500 Genetic Analyzer (Life-Technologies, Carlsbad, CA) using Gene Marker 1.91 software (Soft Genetics, State College, PA).
Results
Clinical manifestations
There are eight female and five male probands. Those affected individuals ranged in age from 14 months to 49 years. Patients from 13 Chinese families all had congenital, bilateral, and sensorineural hearing loss. They all come to the doctor because they have failed newborn hearing screening or were diagnosed with hearing abnormalities in infancy. Their hearing loss was relatively stable, with the exception of a mild progression of HL5 proband. Hearing loss was defined as varying degrees, including moderate, severe, and profound hearing loss. Click on the auditory brainstem response thresholds was 60–70 dBs for HL5, 40 dBs for HL6, 60–70 dBs for HL7, 40 dBs for the left ear, and 90 dBs for the right ear of HL11 proband. The other probands had profound hearing loss. The HL5 proband was diagnosed with retinitis pigmentosa by an ophthalmologist as a teenager including symptoms of small vision, night blindness, and amblyopia. The HL10 proband had a heterochromia iridis, and her father had a heterochromia iridis but no hearing loss. The HL11 proband had excessive freckles, and his father had excessive freckles but no hearing loss. The HL12 proband had hydronephrosis. Ear malformation was observed in the HL13 proband by MRI, including abnormal enlargement of inner ear canal, bone defect of cochlear apex and skull base, and cerebrospinal fluid.
Genetic findings
To detect possible causative variations by target NGS or WES, nonsynonymous variants were filtered, with minor allele frequencies greater than 0.005 for autosomal recessive, and minor allele frequencies greater than 0.0005 for autosomal dominant. Candidate causative mutations are summarized in Table 1. In 8 recessive families, bi-allelic mutations were found in known deafness genes, part of which were identified by parental genotyping including p.H3204R and p.N2356K in USH2A (OMIM 608400), p.Q2137X and p.W778X in CDH23 (OMIM 605516), c.3795 + 5 G>A and c.7051_7054 + 1dup in CDH23 (OMIM 605516), p.R1898Q and c.10258_10260 del in MYO15A (OMIM 602666), c.12177_12181delGGTTG and a duplication in ADGRV1 (OMIM 602851), partial or whole gene deletion in STRC (OMIM 606440), c.16 + 1C>T and c.535 + 5G>A in TMC1 (OMIM 606706) (Table 1). In 4 dominant families, four heterozygous variants associated with dominant deafness were identified, including c.534_535 in GGAGGCAGAGGAA in PAX3 (OMIM 606597), c.1174-2A > T in PAX3 (OMIM 606597), p.T303T in MITF (OMIM 156845), and p.Y113H in PROKR2 (OMIM 607123), as well as partial co-segregating with the phenotype (Figure 1). In the HL13 proband, we detected the hemizygous deletion of POU3F4 gene by targeted sequencing, which is consistent with the clinical phenotype of the patient. The co-separation of the reported mutations was confirmed from the hearing phenotype of the extended family members by Sanger sequencing (Figures 1, 2). Of the 19 mutations identified in this study, 10 were reported to be associated with deafness for the first time (Table 1, Figure 2).
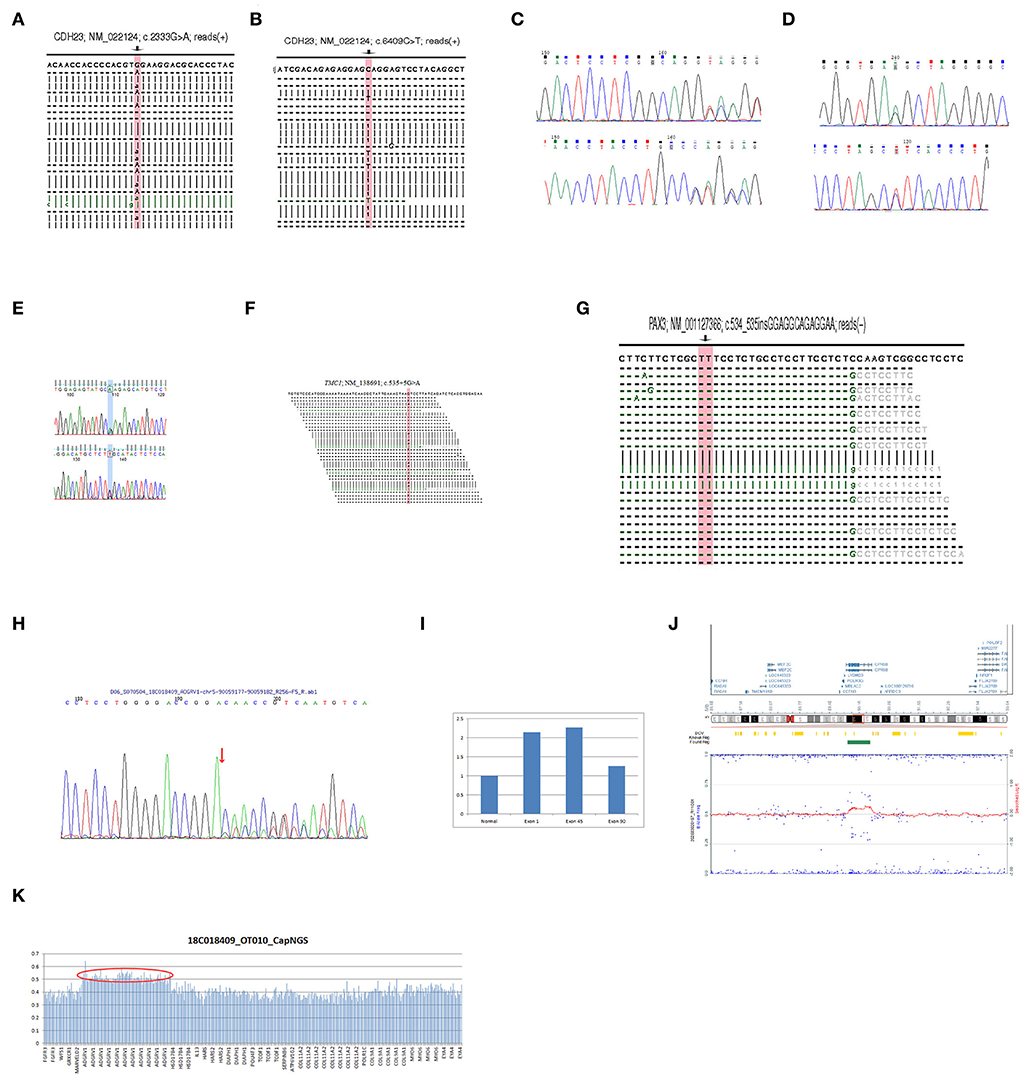
Figure 2. (A) The NGS result of the mutation c.2333G > A in CDH23 of HL2 proband. (B) The NGS result of the mutation c.6409C > T in CDH23 of HL2 proband. (C) The Sanger sequencing result of the mutation c.3579 + 5G > A in CDH23 of HL3 proband. (D) The Sanger sequencing result of the mutation c.7051_7054 + 1dup in CDH23 of HL3 proband. (E) The Sanger sequencing result of the mutation c.5693G > A in MYO15A of HL4 proband. (F) The NGS result of the mutation c.535 + 5G > A in TMC1 of HL8 proband. (G) The NGS result of the mutation c.534_535insGGAGGCAGAGGAA in PAX3 of HL9 proband. (H) The Sanger sequencing result of the mutation c.12177_12181delGGTTG in ADGRV1 of HL5 proband. (I) The qPCR results of the exon 1, 45, and 90 in ADGRV1 of the HL5 proband contrast to normal. (J) The SNP array result of the proband HL5. (K) The NGS result of the HL5 proband.
Using targeted NGS and SNP arrays, the HL5 proband was found to replicate approximately 544 kb in chromosome region 5q14.3 [arr5q14.3 (89887683-90431463) X3] (Figure 2). Whole exon sequencing revealed a 99 kb of copy number variation in the HL6 proband at 15q15.3 (43888567-43988112), including STRC, CKMT1A, CKMT1B, and CATSPER2 genes. Using MLPA, we found that the sibling in the HL6 family with the same symptoms also had the same CNV (Figure 3). In the HL7 family, the results of WES and MLPA revealed that the proband had a homozygous deletion in exon 8 and 10 of CKMT1B gene, and exon 19, 23–25 of STRC gene, heterozygous deletion of exon 1, 2, 4, 7, and 12 of CATSPER2 gene. Using MLPA, we detected heterozygous deletion of exon 8, 10 of CKMT1B gene, and exon 19, 23–25 of STRC gene in the father of the proband; heterozygous deletion in exon 8 and 10 of CKMT1B gene, exon 19, 23–25 of STRC gene, and exon 1, 2, 4, 7, and 12 of CATSPER2 gene in the mother of the proband (Figure 3).
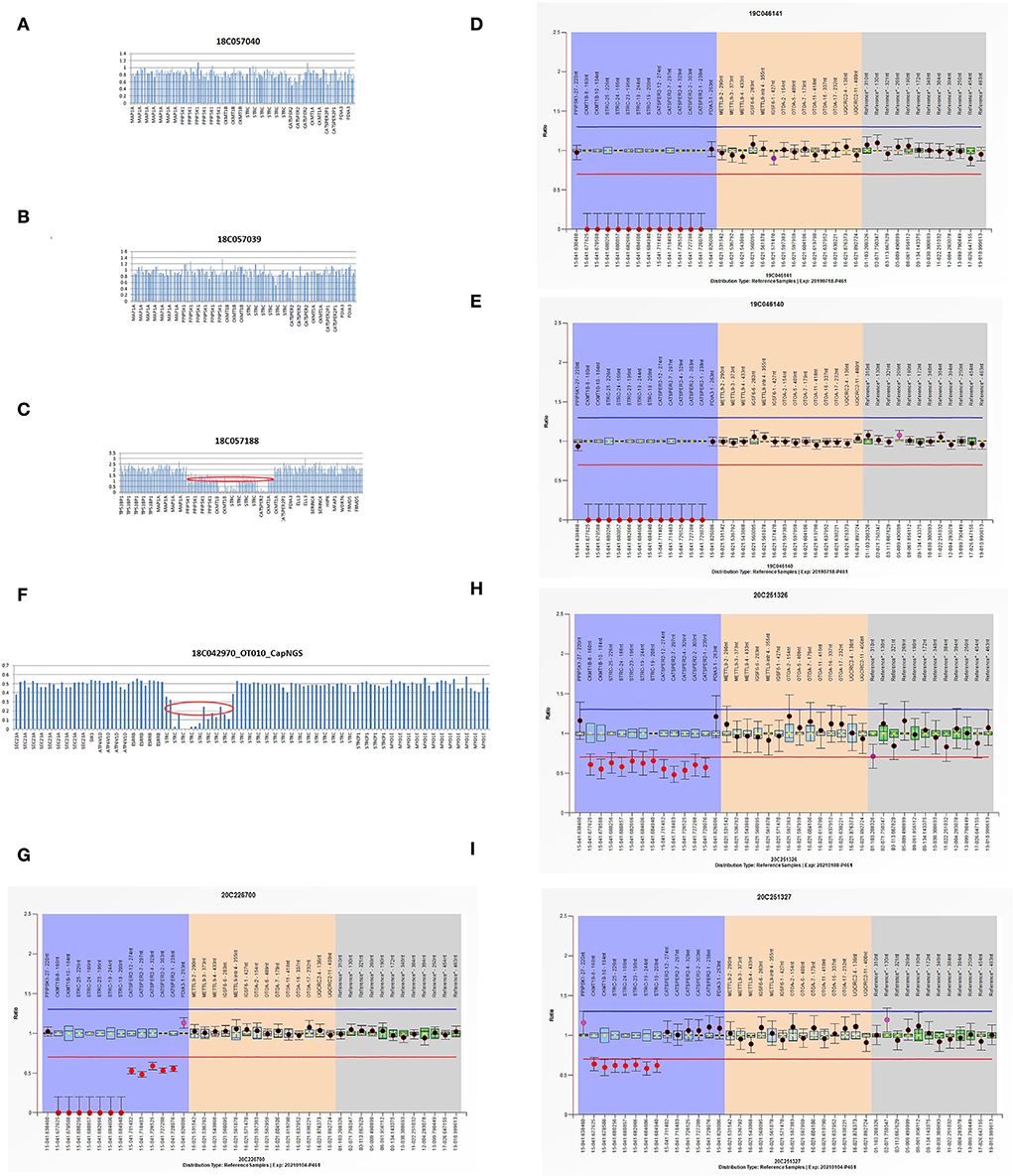
Figure 3. (A–C) The WES results of I:1, I:2, and II:1 in HL6 family. (D,E) The MLPA results of II:1 and II:2 in HL6 family. (F) The NGS result of HL7 proband. (G–I) The MLPA results of II:1, I:2, and I:1 in HL7 family.
Discussion
In CDH23, homozygous nonsense, frameshift, some missense and splice site mutations, or compound heterozygotes combined of these above USH1D alleles are considered hypomorphic alleles with no sufficient retinal, vestibular, and auditory cochlear function leading to USH1D. Conversely, missense mutations in CDH23 are associated with non-syndromic DFNB12 deafness. The DFNB12 allele maintains normal retinal and vestibular function and is dominant to the USH1D allele phenotypically, even in the presence of the USH1D allele (6). In this study, patients were young at the time of diagnosis. While there are no obvious vestibular and retina symptoms, based on the above principles, we can make a USH1D diagnosis in this study due to the nonsense and splice site mutations of CDH23 in the proband HL2 and HL3. ADGRV1 is a pathogenic gene of USH2C, which is belonging to USH2. The primary clinical manifestation of USH2 is congenital moderate to severe deafness, and onset of retinitis pigmentosa within 1–20 years of life, but without vestibular impairment. Besnard et al. (7) concluded that ADGRV1 mutations account for 6.4% and a small but significant proportion of mutations that cause USH2. Newly research suggests gene dysfunction associated with Usher syndrome is the second-leading genetic cause of hereditary sensorineural hearing loss after connexin dysfunction (8). In this study, because the probands of HL1, 2, and 3 were still young and only showed hearing problems, they will develop into Usher syndrome in the future. The proband HL5 was over 30 years old, and he showed typical symptoms of the Usher syndrome, including hearing loss, small vision, night blindness, and amblyopia.
Of all congenital sensorineural deafness, STRC mutations reported for the first time in 2001 are currently estimated to account for ~5–6% (9). However, given variable STRC allele frequencies existing in different races, there may be a higher proportion, and an increasing number of cases are reporting copy number variation relevant to clinical in the STRC locus (10). STRC, with 99.6% coding sequence identity, is closely linked to the pseudogene and is a challenge for the analysis. The gene CATSPER2, a neighboring gene to STRC, is responsible for sperm motility and leads to deafness infertility syndrome in males, most commonly with sequential deletion of both STRC and CATSPER2 genes. Women with this serial loss only suffer from hearing loss (11). The study indicates that, after the GJB2 gene which is the majority of mild to moderate inherited deafness, STRC deletion accounts for the second most common cause (12). Due to racial differences and insufficient attention, the role of STRC gene mutations in the pathogenic of hereditary deafness in China is less reported. The results show that copy number variation of STRC gene is not uncommon in clinics. Next-generation sequencing can identify such cases. Combining with the MLPA method, patients can be accurately diagnosed with the STRC gene mutation, which requires us to pay more attention to its pathogenicity in the clinic.
Waardenburg syndrome is susceptible to being misdiagnosed as autosomal recessive due to PAX3 spontaneous mutation and ignores MITF-related freckle phenotype. It is a de novo mutation c.534_535ins GGAGGCAGAGGAA of PAX3 in the HL9 proband. C.1174-2A > T in PAX3 of the HL10 proband and c.909G >A in MITF of the HL11 proband were inherited from their fathers, respectively, with heterochromia iridis or excessive freckles but no hearing loss.
DFNX2 (X-linked deafness type 2), with clinical features, typically include progressive mixed hearing loss, stapes fixation, and temporal bone anomalies, is the most common type of X-linked deafness in humans (13, 14). The POU3F4 mutations account for approximately 50% of genetic causes of DFNX2 (15). Affected men showed mixed hearing loss or less commonly, only sensorineural hearing loss. Typical manifestations of MRI are characterized by hypoplasia of the cochlear base, thickening of the base of stapes floor, loss of the bony modiolus, expansion of internal acoustic meatus, and abnormally wide communication between the cochlear base and the auditory bone (16). In our study, we investigated the HL13 proband was characterized by structural abnormalities of inner ear, X-linked recessive inheritance, and hemizygous deletion in POU3F4.
Our research resulted in a limited number of sporadic family samples, and the genetic results of some families are lack of validation by other affected family members, which needs a further evaluation from other relevant studies, especially those novel mutations. Our results also further verified the pathogenicity of the reported mutation sites in the hearing loss population.
Conclusion
The results of the limited samples of this study show that hearing loss is a highly genetic heterogeneous disease. In hearing loss patients negative of GJB2, SLC26A4, and mitochondrial 12S rRNA, Usher and Waardenburg syndrome-related genes account for a major proportion in Chinese Han families, followed by STRC causing mild to moderate hearing loss.
Data availability statement
The datasets presented in this study can be found in online repositories. The names of the repository/repositories and accession number(s) can be found at: https://www.ncbi.nlm.nih.gov/, PRJNA876030.
Ethics statement
The studies involving human participants were reviewed and approved by the Ethics Committee of Xinhua Hospital affiliated to Shanghai Jiaotong University School of Medicine. Written informed consent to participate in this study was provided by the participants' legal guardian/next of kin.
Author contributions
LS and ZL wrote the paper. XW, JS, YL, and YH collected and analyzed the data. JY and LS designed the research. All authors contributed to the article and approved the submitted version.
Funding
This research was supported by grants from the National Natural Science Foundation of China, Grant/Award Numbers: 81873698.
Acknowledgments
The authors thank the family members for their cooperation.
Conflict of interest
The authors declare that the research was conducted in the absence of any commercial or financial relationships that could be construed as a potential conflict of interest.
Publisher's note
All claims expressed in this article are solely those of the authors and do not necessarily represent those of their affiliated organizations, or those of the publisher, the editors and the reviewers. Any product that may be evaluated in this article, or claim that may be made by its manufacturer, is not guaranteed or endorsed by the publisher.
Supplementary material
The Supplementary Material for this article can be found online at: https://www.frontiersin.org/articles/10.3389/fneur.2022.1048218/full#supplementary-material
References
1. Morton CC, Nance WE. Newborn hearing screening–a silent revolution. N Engl J Med. (2006) 354:2151–64. doi: 10.1056/NEJMra050700
2. Shearer AE, Smith RJ. Genetics: advances in genetic testing for deafness. Curr Opin Pediatr. (2012) 24:679–86. doi: 10.1097/MOP.0b013e3283588f5e
3. Liu X, Xu L, Zhang S, Xu Y. Epidemiological and genetic studies of congenital profound deafness in the general population of Sichuan, China. Am J Med Genet. (1994) 53:192–5. doi: 10.1002/ajmg.1320530214
4. Koffler T, Ushakov K, Avraham KB. Genetics of hearing loss: syndromic. Otolaryngol Clin N Am. (2015) 48:1041–61. doi: 10.1016/j.otc.2015.07.007
5. Sun L, Wang X, Hou S, Liang M, Yang J. Identification of MYO6 copy number variation associated with cochlear aplasia by targeted sequencing. Int J Pediatr Otorhinolaryngol. (2020) 128:109689. doi: 10.1016/j.ijporl.2019.109689
6. Schultz JM, Bhatti R, Madeo AC, Turriff A, Muskett JA, Zalewski CK, et al. Allelic hierarchy of CDH23 mutations causing non-syndromic deafness DFNB12 or Usher syndrome USH1D in compound heterozygotes. J Med Genet. (2011) 48:767–75. doi: 10.1136/jmedgenet-2011-100262
7. Besnard T, Vache C, Baux D, Larrieu L, Abadie C, Blanchet C, et al. Non-USH2A mutations in USH2 patients. Hum Mutat. (2012) 33:504–10. doi: 10.1002/humu.22004
8. Guillaume J, Céline P, Marta S, Clémence J, Evan G, Carl A, et al. Genetics of Usher Syndrome: New Insights from a Meta-analysis. Otol Neurotol. (2019) 40:121–9. doi: 10.1097/MAO.0000000000002054
9. Marková SP, BroŽková DŠ, Laššuthová P, Mészárosová A, Krutová M, Neupauerová J, et al. gene mutations, mainly large deletions, are a very important cause of early-onset hereditary hearing loss in the Czech population. Genet Test Mol Biomarkers. (2018) 22:127–34. doi: 10.1089/gtmb.2017.0155
10. Sloan-Heggen CM, Bierer AO, Shearer AE, Kolbe DL, Nishimura CJ, Frees KL, et al. Comprehensive genetic testing in the clinical evaluation of 1119 patients with hearing loss. Hum Genet. (2016) 135:441–50. doi: 10.1007/s00439-016-1648-8
11. Zhang Y, Malekpour M, Al-Madani N, Kahrizi K, Zanganeh M, Lohr NJ, et al. Sensorineural deafness and male infertility: a contiguous gene deletion syndrome. J Med Genet. (2007) 44:233–40. doi: 10.1136/jmg.2006.045765
12. Kim BJ, Oh DY, Han JH, Oh J, Kim MY, Park HR, et al. Significant Mendelian genetic contribution to pediatric mild-to-moderate hearing loss and its comprehensive diagnostic approach. Genet Med. (2020) 22:1119–28. doi: 10.1038/s41436-020-0774-9
13. Cremers CW, Huygen PL. Clinical features of female heterozygotes in the X-linked mixed deafness syndrome (with perilymphatic gusher during stapes surgery). Int J Pediatr Otorhinolaryngol. (1983) 6:179–85. doi: 10.1016/S0165-5876(83)80118-9
14. Phelps PD, Reardon W, Pembrey M, Bellman S, Luxom L. X-linked deafness, stapes gushers and a distinctive defect of the inner ear. Neuroradiology. (1991) 33:326–30. doi: 10.1007/BF00587816
15. De Kok YJ, Van der Maarel SM, Bitner-Glindzicz M, Huber I, Monaco AP, Malcolm S, Pembrey ME, Ropers HH, Cremers FP. Association between X-linked mixed deafness and mutations in the POU domain gene POU3F4. Science. (1995) 267:685–8. doi: 10.1126/science.7839145
Keywords: deafness, targeted sequencing, whole-exome sequencing, gene mutation, etiological analysis
Citation: Sun L, Lin Z, Wang X, Shen J, Li Y, Huang Y and Yang J (2022) Molecular etiology study of hearing loss in 13 Chinese Han families. Front. Neurol. 13:1048218. doi: 10.3389/fneur.2022.1048218
Received: 19 September 2022; Accepted: 24 October 2022;
Published: 23 November 2022.
Edited by:
Michael Strupp, Ludwig Maximilian University of Munich, GermanyReviewed by:
Andrés Soto-Varela, Complejo Hospitalario Universitario de Santiago, SpainJorge Rey-Martinez, Donostia University Hospital, Spain
Copyright © 2022 Sun, Lin, Wang, Shen, Li, Huang and Yang. This is an open-access article distributed under the terms of the Creative Commons Attribution License (CC BY). The use, distribution or reproduction in other forums is permitted, provided the original author(s) and the copyright owner(s) are credited and that the original publication in this journal is cited, in accordance with accepted academic practice. No use, distribution or reproduction is permitted which does not comply with these terms.
*Correspondence: Jun Yang, yangjun@xinhuamed.com.cn; Lianhua Sun, sunlianhua@xinhuamed.com.cn
†These authors have contributed equally to this work