- 1Department of Neurology, Friedrich-Baur-Institute, Klinikum der Universität, Ludwig-Maximilians-University, Munich, Germany
- 2Institute of Health Sciences, Universität zu Lübeck, Luebeck, Germany
- 3Clinic for Neurology, Medical Park, Bad Feilnbach, Germany
Introduction: To date, a few studies have used somatosensory evoked potentials (SEP) to demonstrate cortical sensory changes among healthy subjects or to estimate cortical plasticity and rehabilitation prognosis in stroke patients after peripheral electrical stimulation (PES) intervention. The primary aim was to systematically review whether PES has a role in changing latencies and amplitudes of SEPs in healthy subjects and stroke patients. Moreover, we searched for a correlation between sensory and motor function assessments and changes in SEP components of included studies.
Methods: The following databases were searched: Pubmed/MEDLINE, Scopus/ScienceDirect, Web of Science/Clarivate, Cochrane Library, The Physiotherapy Evidence Database (PEDro), and ClinicalTrials.gov. Titles and abstracts, as well as full-text reports, were screened for eligibility by two independent reviewers according to a priori defined eligibility criteria. There were no study limitations concerning the treatment of the upper limb, lower limb, or torso with PES.
Results: The final systematic search resulted in 11,344 records, however only 10 were evaluated. We could not find enough evidence to confirm use of SEP as a predictor to estimate the rehabilitation prognosis after stroke. However, we found a correlation between different sensory and motor function assessments and changes in SEP components. The stroke studies involving PES that initiate a voluntary contraction used for a specific movement or task indicate a positive relationship and correlation to assessments of motor function. It could be indicated that PES have a predictive impact of sensory reorganization, as mirrored by the change in SEP amplitude and latency. However, it is not possible to verify the degree of connectivity between SEP and cortical plasticity. To confirm this hypothesis, we propose the conduction of randomized controlled trials in healthy volunteers and stroke patients.
Systematic review registration: https://doi.org/10.17605/OSF.IO/U7PSY.
Introduction
Peripheral electric stimulation (PES) is a rehabilitative technology that uses electrical currents to the peripheral nerves. It has been proposed that somatosensory stimulation in the form of electromyographically triggered neuromuscular electrical stimulation to the peripheral nerve can influence functional measures of motor performance in stroke patients and can additionally produce changes in cortical excitability (1, 2). In this way, PES provides restoration of walking or arm movements in individuals with complete or incomplete spinal cord injury, stroke, or other upper motor neuron lesion (2–4).
The literature offers multiple terms for peripheral electrical stimulation: transcutaneous electric nerve stimulation (TENS) (5–8), functional electrical stimulation (9–12), cutaneous electrical stimulation (13), somatosensory stimulation (14), neuromuscular electrical stimulation (1, 15) or combination of terms “percutaneous” and “neuromodulation”.
Sheffler and Chae (16) devoted important consideration to the use of electrical stimulation for motor relearning. They described three types of electrical stimulation available for motor learning: functional electrical stimulation (FES), electromyography or biofeedback mediated FES, and application of neuroprostheses. In the first case the patient is a passive participant in the FES training and no cognitive investment is necessary. The second type of exercises combines afferent feedback information with FES induced repetitive movements. During training with neuroprosthesis, functional tasks can be performed (2). The multiple PES terms used in the present review can be classified into one or more stimulation types described by Sheffler and Chae. Furthermore, for all terms the same technique is being used: placing surface electrodes on the skin overlaying sensory-motor nerve structures, establishing an electric field between two electrodes and ions, generating a current in the tissue. In the following text, only the term PES will be used exclusively.
In many studies, it has been found that the stroke patient's walking speed, endurance, and coordination improved with the use of PES (2, 17–19). The same modality on motor cortical excitability is described by recording motor evoked potentials (20–22), transcranial magnetic stimulation (23, 24) or fMRI (1, 25). On the other hand, the influence of PES on somatosensory function has been frequently overlooked in clinical context and research in the field of stroke rehabilitation (15). Prediction of upper limb (UL) and lower limb (LL) motor recovery in stroke patients is generally based on clinical examination (26). The prognosis is typically based on clinical impression, incorporating clinical and demographic factors such as stroke severity and age (27). Moreover, clinicians cannot know whether the prognoses they make at the acute stage are correct unless they do not routinely assess each of their patients several months later (27). This gestalt approach can produce differing opinions about prognosis and these seem to produce variation in discharge planning (28). According to Feys et al. (26) the combination of the motor score and somatosensory evoked potentials (SEPs) is best able to predict an outcome especially in the acute stroke phase, since neurophysiological measures alone are of limited value in predicting a long-term effect. The finding by Kato et al. (29) who examined the SEPs of the median and the tibial nerves in patients with hemorrhagic lesions, confirmed that 60 out of 65 arms (92.3%) and 50 out of 62 legs (80.6%) showed abnormalities in SEPs. These findings may indicate SEP measures quantifying latencies, thresholds, and evoked responses at high stimulator intensities had high reliability and require small sample sizes to power a study adequately (30). Therefore, the validation of SEP as a new standard neurophysiological tool for assessing the rehabilitation prognosis after stroke seems a reasonable decision.
SEPs are time-locked potentials evoked by electric stimulation of the sensory or mixed peripheral nerves and recorded along with the large fiber somatosensory (dorsal column–medial lemniscus) pathway. SEPs record transmission of an electrical signal/action potential between recording sites along the impulse pathway, thereby allowing the identification of abnormalities that help to localize a lesion (31).
Urasaki et al. (8) found that the dorsal column nucleus has the main role in CNS sensory amplification and that PES suppresses this amplification phenomenon in the medial lemniscus pathway. Consequently, use of PES as an intervention to verify SEP as a new standard neurophysiological tool could be a good method to observe changes in cortical somatosensory pathways.
The effects of PES on somatosensory cortical representation in healthy subjects have not been fully investigated yet, since little is known about the functional features of mismatch deviant and standard responses across different sensory brain modalities (32). This controversy of sensory changes and cortical plasticity persists in stroke patients and changes in corticomotor excitability still remains elusive (33).
To our knowledge, the role of PES in changing latencies and amplitudes of SEPs in healthy subjects or whether effects of PES aiming at motor rehabilitation after stroke have an impact on the improvement of pathological SEPs have not yet been studied. Furthermore, one of the aims of the present study was to examine the evidence on sensorimotor assessment and changes in SEP components after PES treatment for clinical correlations, so that SEP can be used at best as a predictor for estimating rehabilitation prognosis after stroke.
Materials and methods
The study protocol was prospectively registered at the open science framework (OSF) with the registration DOI: https://doi.org/10.17605/OSF.IO/YW6PT on the 14th of March 2021 and an update protocol was registered in OSF (https://doi.org/10.17605/OSF.IO/U7PSY) on the 27th of August 2022. The PICO (34) model was implemented to answer the primary clinical questions: Do the effects of PES on motor rehabilitation in post-stroke patients have an impact on the latencies and amplitudes of pathological SEPs and does PES alter the latencies and amplitudes of SEPs in healthy subjects? (Table 1).
This systematic review was conducted using “The Preferred Reporting Items for Systematic reviews and Meta-Analyses (PRISMA) statement 2020” (35) and followed recommendations from the Cochrane handbook (36).
Study selection
A team of four healthcare professionals, including two physiotherapists (MM, AJ), and two physicians (PY, BS), established the study's aim, its primary outcome measures, the search strategy, and its eligibility criteria. The search construct consisted of two main subjects: peripheral electric stimulation and somatosensory evoked potentials. The following databases were searched: Pubmed/MEDLINE, Scopus/ScienceDirect, Web of Science/Clarivate, Cochrane library database, The Physiotherapy Evidence Database (PEDro), and ClinicalTrials.gov. The cut-off date of the search was the 28th of August 2022.
Because only 8 eligible studies were identified with the initial search, it was decided to repeat the search, include additional databases, use a revised search strategy, and publish an update registration protocol (see above). All search strategies (from the first search and from the update search) can be found in each registration protocol in OSF. Screening of all articles published in English and German was performed independently by two authors (MM, AJ) using the Rayyan QCRI software (37) and no automation tools were used in the process. The research team defined inclusion and exclusion criteria in advance. Reference sections of relevant review and research articles were used to identify additional pertinent articles. The full text of articles identified by the title and/or abstract as possibly applicable was retrieved, and the final decision on the inclusion was made by both reviewers independently. Disagreements between reviewers were resolved by consulting a third and fourth reviewers (PY, BS). The mesh term SEP was introduced in 1982 by PubMed, consequently the search time limit was set from January of the same year. If required, additional information was requested from the article authors. Data regarding the number of probands, study design, duration of treatments, and PES adjustments (Table 2) were extracted from each report. In addition, the researchers evaluated all found cortical latencies and amplitudes of SEPs fragments. The data of SEP fragments from healthy individuals and from patients with stroke regarding PES are presented separately to avoid misunderstanding of the evaluated fragments (Tables 3, 4).
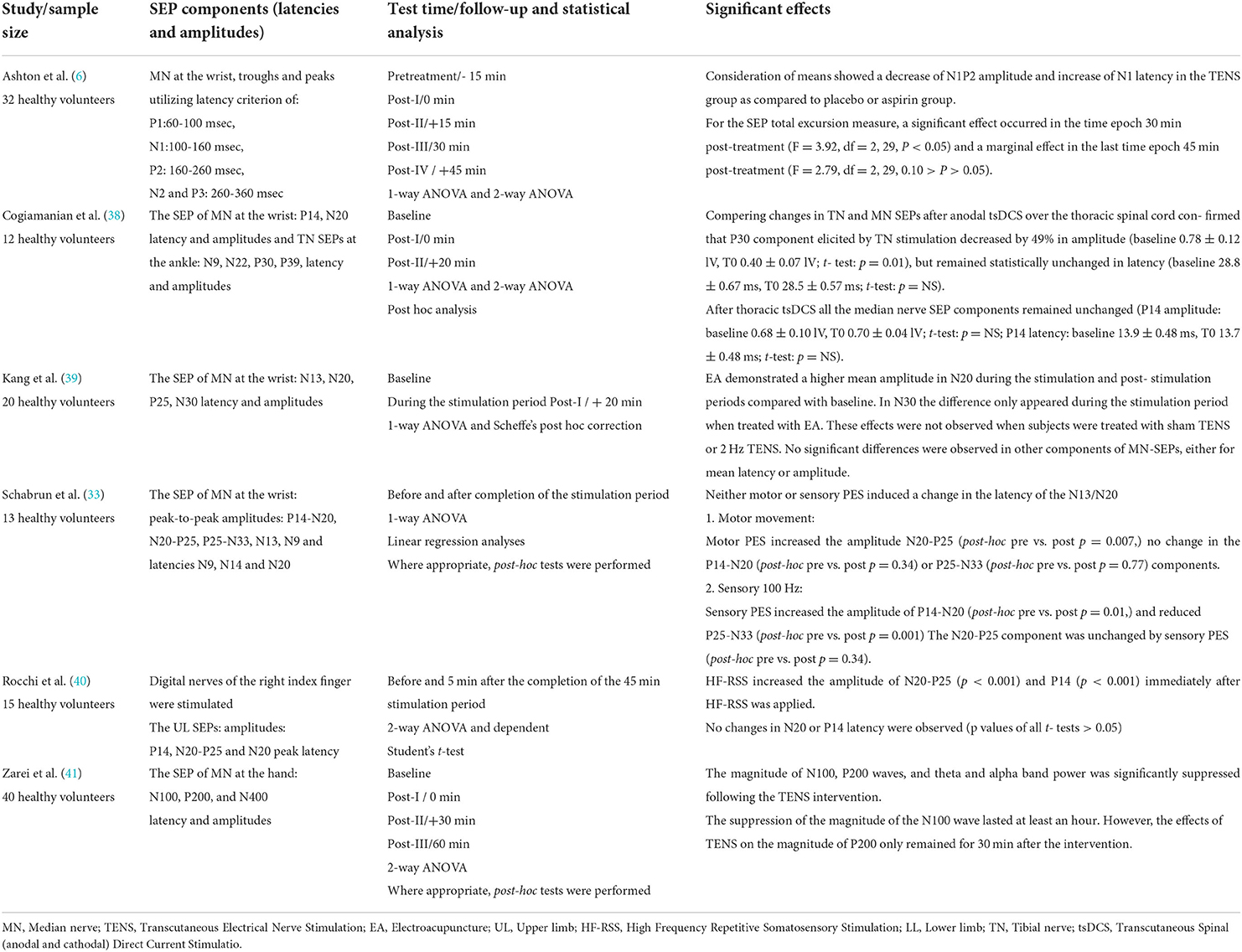
Table 3. Latencies and Amplitudes of SEP Components—Pre–Posttest—Studies made on healthy volunteers.
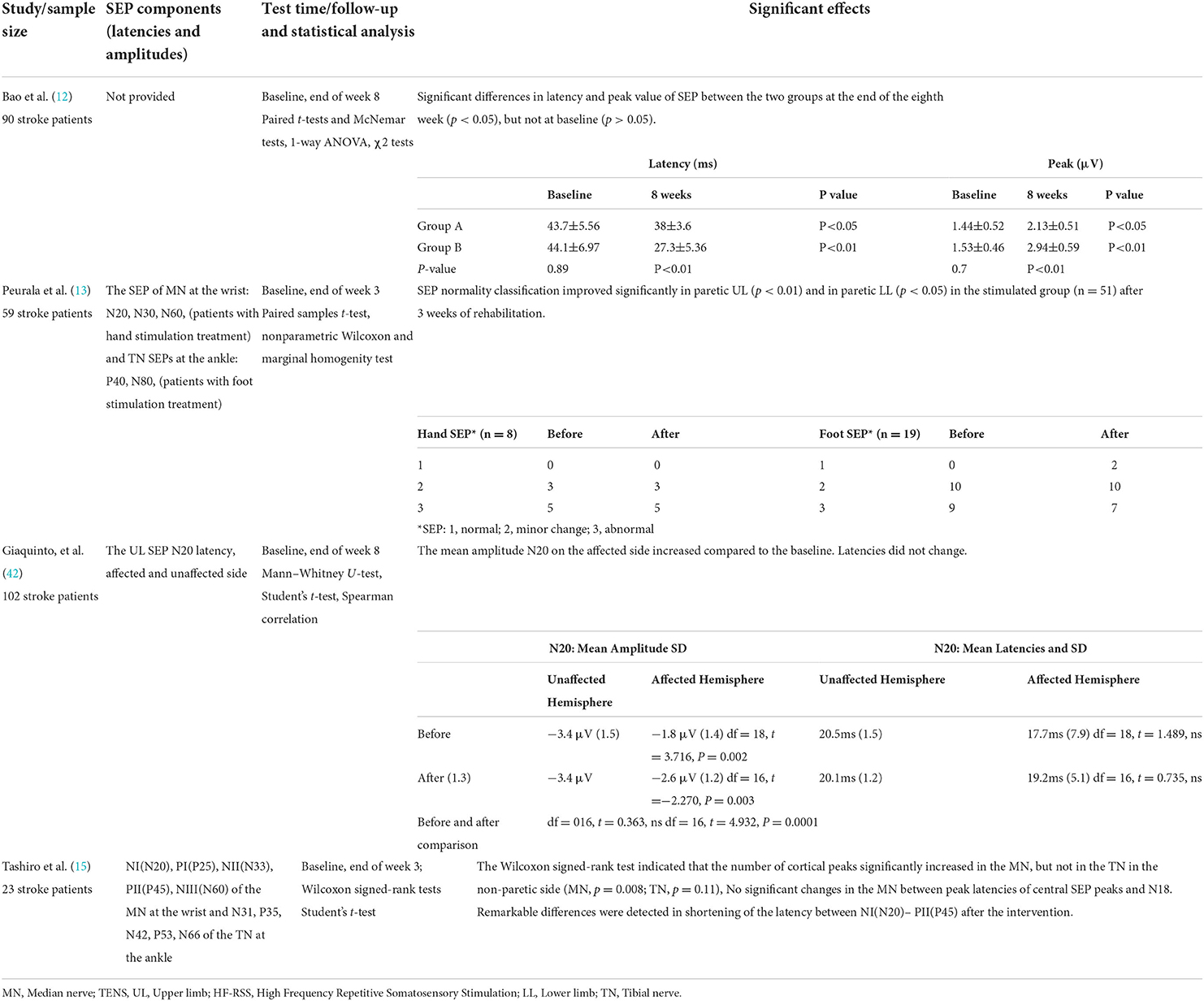
Table 4. Latencies and Amplitudes of SEP Components—Pre–Posttest –Studies made on stroke population.
Exclusion/inclusion criteria
All type of non-randomized and randomized intervention studies were included. Moreover, intervention studies with no control group were also included since it was anticipated that the available data to answer the research question would be limited. No restrictions were set with regard to the body parts treated (UL, LL or torso) with PES. Studies in which only electroacupuncture was used were excluded since the piercing through dermis can affect additional neurological afferent pathways associated with pain giving misleading SEP results (43). Studies that measured SEP only during the intervention without follow-up measure were excluded since the study search is limited on SEP use as a change predictor. No limits were set regarding the outcome measures used to determine motor impairment and/or functional performance. Data from abstracts, letters, pilot studies, case studies and review articles were excluded from the study. Studies involving children and animals were not considered either. No limitations were applied regarding the type of stroke (ischemic or hemorrhagic), the time elapsed since the last occurrence, or the stroke location. In the text, the term stroke is used for both, ischemic and hemorrhagic stroke. The studies which focused on the effect of electrical stimulation on any of the following conditions were excluded: spinal cord injuries, Parkinson's disease, multiple sclerosis, pain or cranial nerve. Furthermore, the studies that used transcranial direct current stimulation, transcranial magnetic stimulation, or deep brain stimulation were excluded.
Methodological quality
The Cochrane risk of bias in non-randomized studies (ROBINS-I) tool developed by Sterne et al. (44) was used to assess the risk of bias of observational studies that compare health effects of two or more interventions. ROBINS-I is a tool for evaluating risk of bias in estimates of the comparative effectiveness (harm or benefit) of interventions from studies that did not use randomization to allocate units (individuals or clusters of individuals) to comparison groups. ROBINS-I'S fundamental underlying principle is to compare the risk of bias associated with the current evaluated non-randomized trial with a target randomized controlled trial (RCT) hypothesized to be conducted with the same group of participants, even though this RCT may not be feasible or ethical (45). The ROBINS-I tool includes seven domains to assess the risk of bias that may arise in a non-randomized study: (1) bias due to confounding; (2) bias in selection of participants into the study; (3) bias in classification of interventions; (4) bias due to deviations from intended interventions; (5) bias due to missing data; (6) bias in measurement of outcomes (or detection bias); (7) bias in selections of the reported results. The categories for risk of bias judgments are Low risk, Moderate risk, Serious risk and Critical risk. The risk of bias is first assessed for each domain, and then the overall judgement of the study's risk of bias is made (44).
The “Quality Assessment Tool for Before-After (Pre-post)” developed by the National Institutes of Health (NIH) was used to rate the methodological quality of pre-post studies without a control group (46). The questions in the NIH quality assessment tool were designed to help reviewers focus on the key concepts for evaluating the internal validity of a study. Critical appraisal of a study involves considering the potential for selection bias, information bias, measurement bias, or confounding. Examples of confounding include co-interventions, differences at baseline in patient characteristics, and other issues addressed throughout the tool which can be found in Table 5. High risk of bias translates to a rating of poor quality; low risk of bias translates to a rating of fair and good quality (46).
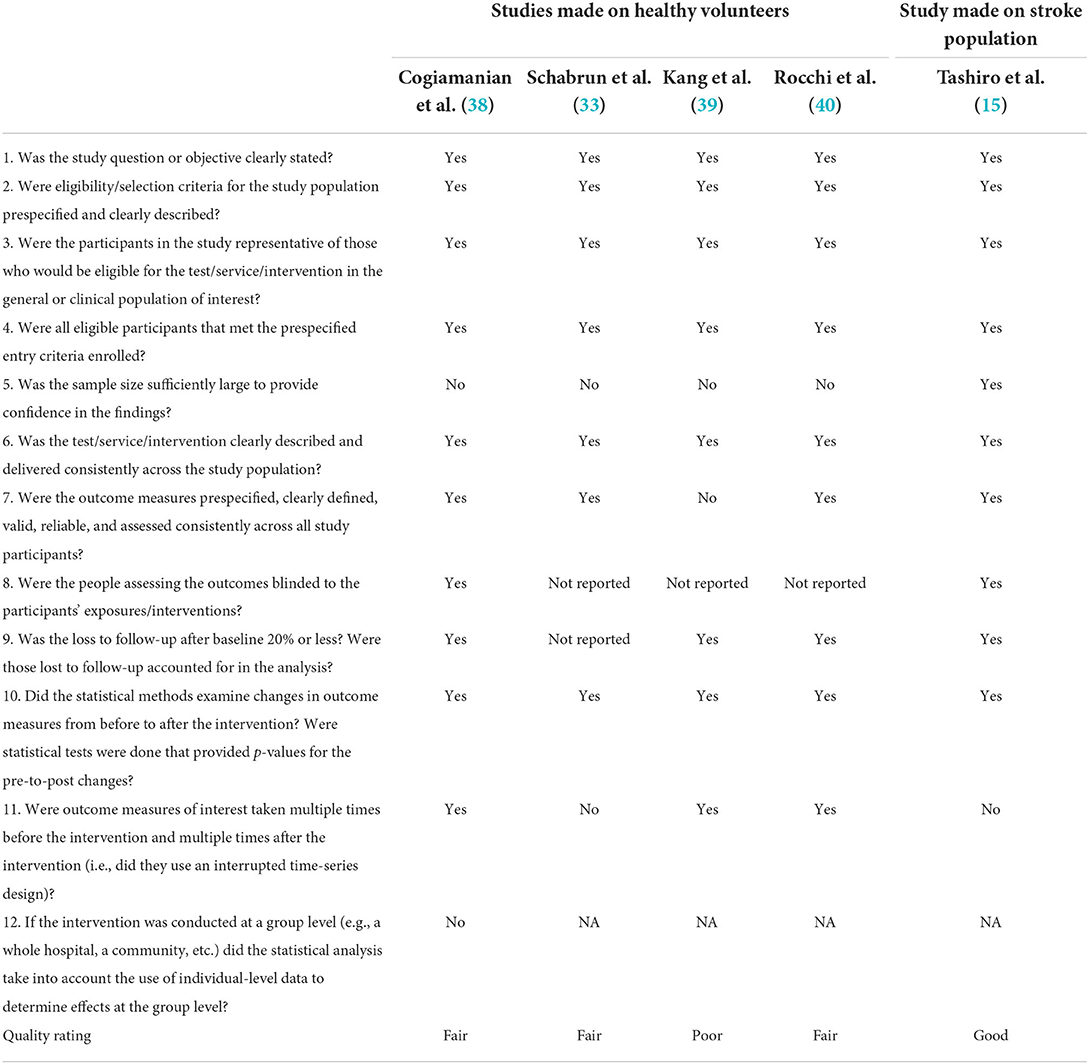
Table 5. Methodological quality of included studies according to the “Quality Assessment Tool for Before-After (Pre-post)”.
The overall certainty of evidence and strength of recommendation was assessed using the Grades of Recommendation Assessment, Development and Evaluation (GRADE handbook) methodology (47). According to the GRADE approach, the evidence is graded as high, moderate, low, or very low certainty of evidence. Furthermore, a body of evidence from observational studies begins with a low certainty of evidence-rating which could be downgraded due to five reasons: risk of bias, indirectness, inconsistency, imprecision and publication bias (36). There are three factors that permit rating up the certainty of evidence: large magnitude of an effect, dose-response gradient, and effect of plausible residual confounding (47).
Results
The systematic search resulted in 11,351 references. The search from Pubmed/MEDLINE database resulted in 2,963 records, Scopus/ScienceDirect database resulted in 1,882 records, Cochrane library database resulted in 4,176 records, Web of Science/Clarivate resulted in 4,877 records and the database PEDro resulted in 10 records. The registry ClinicalTrials.gov was searched manually, and four studies were included for further evaluation. We excluded 2,561 duplicate studies using Rayyan QCRI software (37). Based on the titles and abstracts 69 reports were included for full-text reading. Additionally, six articles were found after the screening of reference lists. Ten articles were included in the review after applying the inclusion and exclusion criteria. The search process is presented in the PRISMA flow diagram (Figure 1).
Intervention procedure and the time frame between the SEP measurements
The different forms of stimulation, stimulation devices, location of PES, amplitude, duration, and frequency pulse as well as test time and follow-up of SEP were examined in each study. All data are summarized in Table 2. The total amount of participants in the reviewed studies was 496. Of these, 364 were stroke patients and 132 were healthy participants. Five studies involved one (12, 41, 42) or two (6, 13) control groups. The study by Kang et al. (39) applied: Sham TENS, 2Hz TENS or 2Hz electroacupuncture and the study from Schabrun et al. (33) applied Motor Movement PES or Sensory PES 100 Hz intervention. On the other hand Tashiro et al. (15) used SEP of the tibial nerve as a reference to SEP for the median nerve and Cogiamanian et al. (38) measured five subjects from the first group a second time using sham stimulation. A wide range of sensory-motor assessments was used to examine the effect of PES in studies of stroke patients and healthy participants (Table 2). The assessment of SEP in two studies on stroke patients (12, 42) was performed at baseline and 8 weeks post PES intervention. In the other two studies (13, 15) the assessment was performed at baseline and 3 weeks post PES intervention. The SEP in healthy participants in all six studies was assessed before, at baseline, and 0,15/20/30/45/60 min after the intervention (6, 33, 38–41). In the majority of studies on stroke patients the SEP measurements were performed on the median nerve. In the study by Peurala et al. (13) SEPs on the UL were performed in those patients who received hand stimulation and SEPs on the LL were performed in those patients who received foot stimulation while in the study from Tashiro et al. (15) SEPs from tibial nerves were used as a control measurement. Bao et al. (12) reported an improvement of latency and peak value of SEPs between the two groups at the end of the 8th week without further explanation of how the measurement was performed. No study showed a loss of peaks after the intervention. Details about SEP changes in latencies and amplitudes components can be found in Tables 3, 4. The following body location were stimulated with PES: tenth thoracic spinal vertebra (38), shoulder (38, 42), arm, hand or fingers (6, 13, 15, 33, 40–42), lower limb and foot (12, 13, 39) and hip (42). The found data about stimulation form, stimulation devices, location of PES, amplitude, duration, and frequency pulse as well as test time and follow-up of SEP could not be standardized so we decided to analyzed data separately.
Methodological quality of included studies
Five non-randomized studies (6, 12, 13, 41, 42) with serious to moderate risk of bias (Figure 2) and five pre-post studies (15, 33, 38–40) without a control group with poor to good methodological quality (Table 5) were included and assessed in the present review. No randomized trials were found. Low overall risk of bias in non-randomized trials, which can be compared to a well-performed randomized trial, was not found in any of the included studies (6, 12, 13, 41, 42). Due to the fact that the Robins-I tool uses strict criteria to evaluate confounding bias, one study was classified as having serious risk of bias (42). Four studies were evaluated as having moderate risk of bias because the data was collected retrospectively (12), insufficient information was given about the potential confounding bias (13, 41), or no explanation of the source of information about intervention status was reported (6). In accordance with the NIH tool, one pre-post study had good (15), tree studies had fair (33, 38, 40), and one had poor (39) methodological quality. The eligibility criteria and the outcome measures were prespecified, clearly defined, valid, reliable, and assessed consistently across all study participants, except for the study by Kang et al. (39). According to the NIH tool, the sample size should be large enough to provide confidence in the findings and outcome assessors should be blinded to the participants' exposures, and interventions. Only the study by Tashiro et al. (15) managed to meet these important criteria.
The overall certainty of evidence was very low for all outcomes (Supplementary Table 1). All available evidence was downgraded for limitations in study design and risk of bias (6, 12, 13, 15, 33, 38–42), imprecision (6, 12, 13, 15, 33, 38–42), or indirectness (6, 12, 39, 41) to very low certainty of evidence. There were no legit reasons to rate up the certainty of evidence. Thus, there is insufficient evidence for or against the use of SEP to monitor therapeutic effects.
Synthesis of results
It was planned to perform a meta-analysis, if enough homogenous data were available, using mean difference and random effects model. However, quantitative synthesis was not possible due to limited and heterogenous eligible studies. Therefore, a qualitative synthesis was performed. Table 6 features a summary of SEP latency and amplitude outcomes in studies using same measurement instruments.
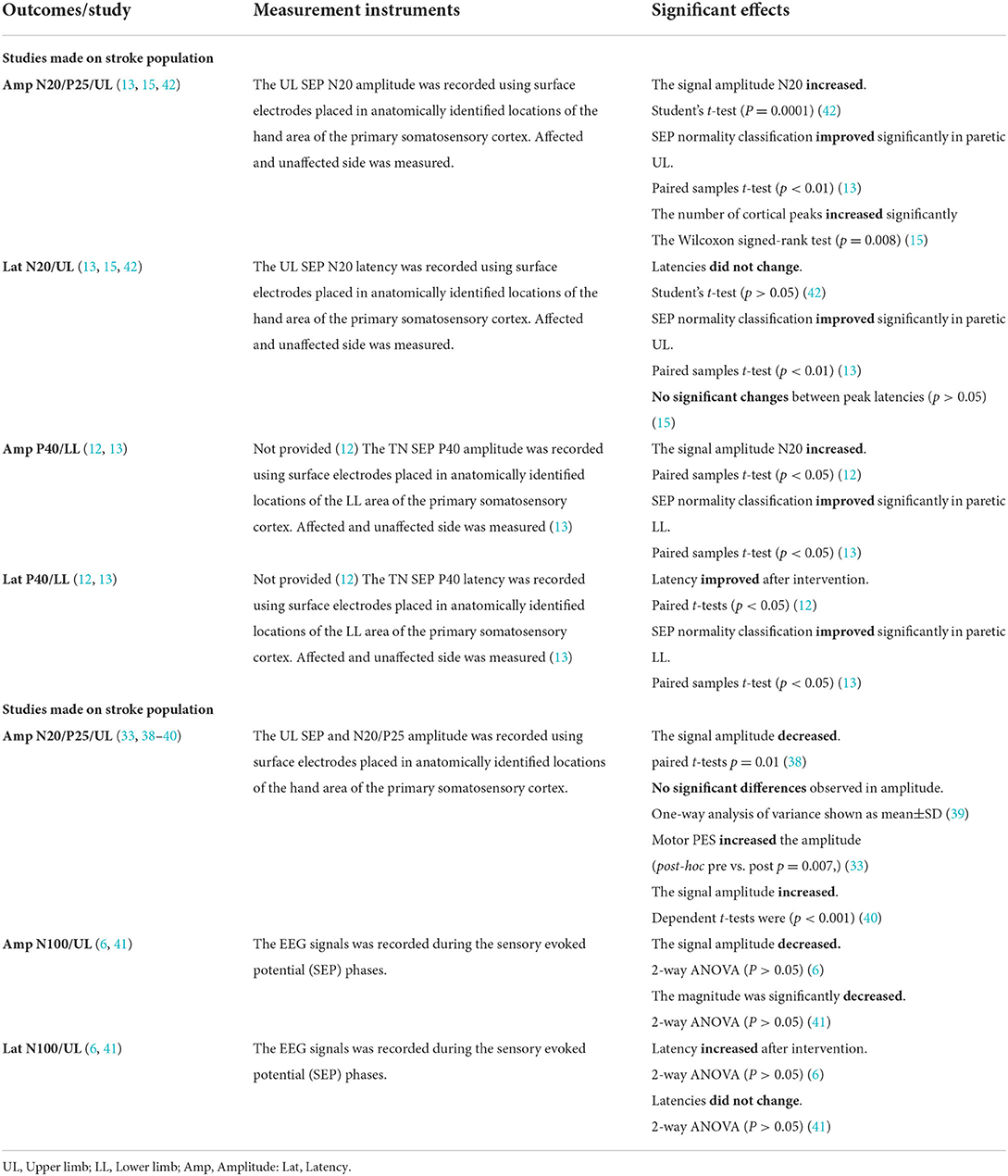
Table 6. SEP latency and amplitude outcomes synthesis in studies using same measurement instruments.
Discussion
Studies made on healthy volunteers
By evaluating motor function and control, corresponding to changes in SEP components in healthy participants, the study by Rocchi et al. (40) suggested that high frequency repetitive somatosensory stimulation leads to improved performance in behavioral tests of temporal discrimination and contributes to improved performance in tests of spatial detection. Nevertheless, high frequency repetitive somatosensory stimulation also affects short-latency inhibition in M1. Together these changes in S1 and M1 may underlie reported improvements in manual motor performance (40). The correlation in healthy individuals between SEP and similar neurophysiological procedures as described in the study by Schabrun et al. (33). The magnitude and direction of the change in corticomotor excitability induced by sensory and motor PES was positively correlated with the difference in the cortical SEP components (r = 0.71, p < 0.001), as confirmed by linear regression between cortical SEP components (N20-P25 and P25-N33) and corticomotor excitability motor evoked potential (MEP) amplitude. Similar changes as already described in the study from Rocchi et al. (40) showed a correlation between PES, high-frequency oscillations analysis and N20-P25 recovery curve. The first conclusion considered from the obtained results is a good validity between SEP, TMS and its correlation with PES. In other terms, not only somatosensory brain areas are affected through PES. Moreover, the motoric brain regions are part of this process. Despite those fact it is necessary to note that all studies made on healthy volunteers assess SEP maximum 1 h after PES and that the studies (38, 41) showed selectively reduced amplitudes in primary somatosensory cortex direct after stimulation. However, we did not identify enough data to provide a clear relationship between SEP and motor performance subsequently to PES in healthy individuals. The lack of observation studies over extended periods of time is the main problem when it comes to drawing a clear conclusion about the impact of PES on SEP.
Studies made on stroke patients
In the stroke study (13) SEP and Modified Motor Assessment Scale results were not compared, but both measures showed improvement. Moreover, in a study (42) significant negative correlation between the time interval for the appearance of somatosensory event-related potentials and the functional independence measure (FIM) score at the time of discharge (r = −0.53, p < 0.01). The study on stroke patients by Tashiro et al. (15) observed significant improvements in behavioral assessment scores for proprioception followed by PES interventions. We could conclude that assessments of motor performance correspond to changes in SEP components in UL and LL. Additionally, the studies on stroke patients involving PES that initiate a voluntary contraction used for a specific movement or task (12, 13, 15, 42), indicate a positive relationship and correlation to assessments of motor function. This hypothesis is supported by findings in a meta-analysis on stroke motor recovery of UL functions (48) and therapeutic effects of peroneal stimulation on gait and motor recovery (18). Moreover, simple sensory stimulation, unrelated to the movement, was of limited functional value for motor recovery for the rehabilitation of the hand in stroke patients and no correlation was described (49).
SEP results in healthy subjects compared to stroke patients
Somatosensory event-related potentials accompanied with SEP in a study from Giaquinto et al. (42) were adequate to follow changes in primary somatosensory area N20. The Bao et al. (12) found significantly improved latency and peak value of SEP and MEP. Furthermore, those changes respond to sensory and motor nerve conduction velocity at the end of the 8 week (p < 0.05). This finding indicates the relevance of evaluating electrophysiological methods and may verifies the use of SEP in stroke patients. All SEP set on stroke patients demonstrated several subcortical or cortical reorganization changes after treatment with PES on the paretic side. However, an unrelated time frame and insufficient data were collected to analyze the relationship between the form of stimulation, pulse amplitude, pulse duration, or location of stimulation and changes in SEP. Perhaps it should be emphasized that in stroke studies in which high pulse amplitude inducing muscle contraction was delivered, the increase of amplitude N20-P25 (15, 42) was seen. The same was observed in healthy participants (33, 40). In order to confirm this hypothesis, we suggest conduction of randomized controlled study on healthy subjects and stroke patients using standard SEP procedure define by Muzyka et al. (31), and clearly described used PES parameter. Based on the GRADE approach to assess the quality of evidence, no outcome that provided a strong recommendation was found. There is thus far insufficient evidence to support a decision for or against use of SEP to monitor therapeutic effects and the results of this analysis cannot be generalizable.
It is known that there are substantial anatomical interconnections linking the brain's motor and somatosensory regions. Cortical motor areas receive direct inputs from primary and second somatosensory cortex and inversely, somatosensory areas get direct cortical inputs from primary motor cortex, premotor cortex, and from supplementary motor area (21, 50). A change in somatosensory function in association with motor learning would seem to be a natural by-product of this anatomical connectivity (21, 50). Findings in this review suggest that PES may shift the response of somatosensory to motor areas of the brain. On the other hand, it could be hypothesized that SEP can indirectly recognize the changes in motor area of the brain. Moreover, it appears that SEPs have sufficient sensitivity to detect even the smallest changes in action potential of neural cortical network after stroke and is probably able to assess the effect of various sensory therapies: cryotherapy, thermotherapy, occupational tactile therapy, or robotic tactile therapy more directly.
Limitations of the study
First, we cannot confirm that all PES studies were identified because the meaning of the term “peripheral electrical stimulation” varies widely and is understood differently. We tried to minimize this limitation by searching more databases. Second, we were aware that EEG measurement can also be used to record SEP, and the lack of keywords and terms to describe this process limited our desire to include all of these studies. However, we used the term “evoked potentials” to increase the number of studies identified in our database search and to include studies using EEG. We also tried to use all PES terms indexed in PubMed to find an optimal data set and minimize this limitation.
Conclusion
From the results of this review, the repetitive task-oriented treatment enriched with PES could likely become a different approach to be applied in stroke patients to improve daily living activities since we have hints that PES may impact changes in motor neuroplasticity. We suggest that more studies (especially RCTs) should be conducted to evaluate whether SEP measures can be used to monitor the therapeutic effects of PES in the rehabilitation of stroke patients, as there is insufficient evidence to do so but SEP remains a promising tool to estimate rehabilitation prognosis after stroke.
Data availability statement
The original contributions presented in the study are included in the article/Supplementary material, further inquiries can be directed to the corresponding author.
Author contributions
MM, AJ, BS, and PY contributed to conception and design of the study. MM organized the database and wrote the first draft of the manuscript. MM and AJ performed the qualitative analysis. All authors contributed to manuscript revision, read, and approved the submitted version.
Acknowledgments
We appreciate the help of Prof. Stephan Kröger from Ludwig-Maximilians-University Munich, Institute for Physiology and Prof. Joachim Liepert, head of the Department of Neurorehabilitation Kliniken Schmieder Allensbach, for critical reading of the article.
Conflict of interest
The authors declare that the research was conducted in the absence of any commercial or financial relationships that could be construed as a potential conflict of interest.
Publisher's note
All claims expressed in this article are solely those of the authors and do not necessarily represent those of their affiliated organizations, or those of the publisher, the editors and the reviewers. Any product that may be evaluated in this article, or claim that may be made by its manufacturer, is not guaranteed or endorsed by the publisher.
Supplementary material
The Supplementary Material for this article can be found online at: https://www.frontiersin.org/articles/10.3389/fneur.2022.1036891/full#supplementary-material
Abbreviations
PES, peripheral electrical stimulation; TENS, transcutaneous electric nerve stimulation; FES, functional electrical stimulation; SEP, somatosensory evoked potentials; UL, upper limb; LL, lower limb; CNS, central nerve system; MEP, motor evoked potential; FIM, Functional Independence Measure; NIH, National Institutes of Health; RCT, randomized controlled trial.
References
1. Kimberley TJ, Lewis SM, Auerbach EJ, Dorsey LL, Lojovich JM, Carey JR. Electrical stimulation driving functional improvements and cortical changes in subjects with stroke. Exp Brain Res. (2004) 154:450–60. doi: 10.1007/s00221-003-1695-y
2. Bajd T, Crt M, Munih M. Functional electrical stimulation with surface electrodes. J Autom Control. (2008) 18:3–9. doi: 10.2298/JAC0802003B
3. Marquez-Chin C, Popovic MR. Functional electrical stimulation therapy for restoration of motor function after spinal cord injury and stroke: a review. Biomed Eng Online. (2020) 19:34. doi: 10.1186/s12938-020-00773-4
4. Moll I, Vles JSH, Soudant DLHM, Witlox AMA, Staal HM, Speth LAWM, et al. Functional electrical stimulation of the ankle dorsiflexors during walking in spastic cerebral palsy: a systematic review. Dev Med Child Neurol. (2017) 59:1230–6. doi: 10.1111/dmcn.13501
5. Laufer Y, Elboim-Gabyzon M. Does sensory transcutaneous electrical stimulation enhance motor recovery following a stroke? A systematic review. Neurorehabil Neural Repair. (2011) 25:799–809. doi: 10.1177/1545968310397205
6. Ashton H, Golding JF, Marsh VR, Thompson JW. Effects of transcutaneous electrical nerve stimulation and aspirin on late somatosensory evoked potentials in normal subjects. Pain. (1984) 18:377–86. doi: 10.1016/0304-3959(84)90050-2
7. Golding JF, Ashton H, Marsh R, Thompson JW. Transcutaneous electrical nerve stimulation produces variable changes in somatosensory evoked potentials, sensory perception and pain threshold: clinical implications for pain relief. J Neurol Neurosurg Psychiatry. (1986) 49:1397–406. doi: 10.1136/jnnp.49.12.1397
8. Urasaki E, Wada SI, Yasukouchi H, Yokota A. Effect of transcutaneous electrical nerve stimulation (TENS) on central nervous system amplification of somatosensory input. J Neurol. (1998) 245:143–8. doi: 10.1007/s004150050194
9. Straudi S, Baroni A, Mele S, Craighero L, Manfredini F, Lamberti N, et al. Effects of a robot-assisted arm training plus hand functional electrical stimulation on recovery after stroke: a randomized clinical trial. Arch Phys Med Rehabil. (2020) 101:309–16. doi: 10.1016/j.apmr.2019.09.016
10. Gandolla M, Ward NS, Molteni F, Guanziroli E, Ferrigno G, Pedrocchi A. The neural correlates of long-term carryover following functional electrical stimulation for stroke. Neural Plast. (2016) 2016:4192718. doi: 10.1155/2016/4192718
11. Berenpas F, Weerdesteyn V, Geurts AC, Van Alfen N. Long-term use of implanted peroneal functional electrical stimulation for stroke-affected gait: the effects on muscle and motor nerve. J Neuroeng Rehabil. (2019) 16:1–10. doi: 10.1186/s12984-019-0556-2
12. Bao X, Luo JN, Shao YC, Tang ZQ, Liu HY, Liu H, et al. Effect of functional electrical stimulation plus body weight-supported treadmill training for gait rehabilitation in patients with poststroke: a retrospective case-matched study. Eur J Phys Rehabil Med. (2020) 56:34–40. doi: 10.23736/S1973-9087.19.05879-9
13. Peurala SH, Pitkänen K, Sivenius J, Tarkka IM. Cutaneous electrical stimulation may enhance sensorimotor recovery in chronic stroke. Clin Rehabil. (2002) 16:709–16. doi: 10.1191/0269215502cr543oa
14. Sawaki L, Wu CWH, Kaelin-Lang A, Cohen LG. Effects of somatosensory stimulation on use-dependent plasticity in chronic stroke. Stroke. (2006) 37:246–7. doi: 10.1161/01.STR.0000195130.16843.ac
15. Tashiro S, Mizuno K, Kawakami M, Takahashi O, Nakamura T, Suda M, et al. Neuromuscular electrical stimulation-enhanced rehabilitation is associated with not only motor but also somatosensory cortical plasticity in chronic stroke patients: an interventional study. Ther Adv Chronic Dis. (2019) 10:204062231988925. doi: 10.1177/2040622319889259
16. Sheffler LR, Chae J. Neuromuscular electrical stimulation in neurorehabilitation. Muscle Nerve. (2007) 35:562–90. doi: 10.1002/mus.20758
17. Stein RB, Everaert DG, Thompson AK, Su Ling Chong, Whittaker M, Robertson J, et al. Long-term therapeutic and orthotic effects of a foot drop stimulator on walking performance in progressive and nonprogressive neurological disorders. Neurorehabil Neural Repair. (2010) 24:152–67. doi: 10.1177/1545968309347681
18. Dunning K, O'Dell MW, Kluding P, McBride K. Peroneal stimulation for foot drop after stroke. Am J Phys Med Rehabil. (2015) 94:649–64. doi: 10.1097/PHM.0000000000000308
19. Popović DB. Advances in functional electrical stimulation (FES). J Electromyography Kinesiol. (2014) 24:795–802. doi: 10.1016/j.jelekin.2014.09.008
20. Everaert DG, Thompson AK, Su Ling Chong, Stein RB. Does functional electrical stimulation for foot drop strengthen corticospinal connections? Neurorehabil Neural Repair. (2010) 24:168–77. doi: 10.1177/1545968309349939
21. Tinazzi M, Zarattini S, Valeriani M, Romito S, Farina S, Moretto G, et al. Long-lasting modulation of human motor cortex following prolonged transcutaneous electrical nerve stimulation (TENS) of forearm muscles: Evidence of reciprocal inhibition and facilitation. Exp Brain Res. (2005) 161:457–64. doi: 10.1007/s00221-004-2091-y
22. Mima T, Oga T, Rothwell J, Satow T, Yamamoto JI, Toma K, et al. Short-term high-frequency transcutaneous electrical nerve stimulation decreases human motor cortex excitability. Neurosci Lett. (2004) 355:85–8. doi: 10.1016/j.neulet.2003.10.045
23. Fernandez-del-Olmo M, Alvarez-Sauco M, Koch G, Franca M, Marquez G, Sanchez JA, et al. How repeatable are the physiological effects of TENS? Clin Neurophysiol. 2008;119(8):1834–9. doi: 10.1016/j.clinph.2008.04.002
24. Kofler M, Fuhr P, Leis AA, Glocker FX, Kronenberg MF, Wissel J, et al. Modulation of upper extremity motor evoked potentials by cutaneous afferents in humans. Clin Neurophysiol. (2001) 112:1053–63. doi: 10.1016/S1388-2457(01)00540-5
25. Zheng X, Chen D, Yan T, Jin D, Zhuang Z, Tan Z, et al. A randomized clinical trial of a functional electrical stimulation mimic to gait promotes motor recovery and brain remodeling in acute stroke. Behav Neurol. (2018) 2018:8923520. doi: 10.1155/2018/8923520
26. Feys H, Van Hees J, Bruyninckx F, Mercelis R, De Weerdt W. Value of somatosensory and motor evoked potentials in predicting arm recovery after a stroke. J Neurol Neurosurg Psychiatry. (2000) 68:323–31. doi: 10.1136/jnnp.68.3.323
27. Stinear CM, Smith M-C, Byblow WD. Prediction tools for stroke rehabilitation. Stroke. (2019) 50:3314–22. doi: 10.1161/STROKEAHA.119.025696
28. Cormier DJ, Frantz MA, Rand E, Stein J. Physiatrist referral preferences for postacute stroke rehabilitation. Medicine. (2016) 95:e4356. doi: 10.1097/MD.0000000000004356
29. Kato H, Sugawara Y, Ito H, Onodera K, Sato C, Kogure K. Somatosensory evoked potentials following stimulation of median and tibial nerves in patients with localized intracerebral hemorrhage: correlations with clinical and CT findings. J Neurol Sci. (1991) 103:172–8. doi: 10.1016/0022-510X(91)90160-9
30. Brown KE, Lohse KR, Mayer IMS, Strigaro G, Desikan M, Casula EP, et al. The reliability of commonly used electrophysiology measures. Brain Stimul. (2017) 10:1102–11. doi: 10.1016/j.brs.2017.07.011
31. Muzyka IM, Estephan B. Somatosensory evoked potentials. Handb Clin Neurol. (2019) 160:523–40. doi: 10.1016/B978-0-444-64032-1.00035-7
32. Zhao C, Valentini E, Hu L. Functional features of crossmodal mismatch responses. Exp Brain Res. (2014) 233:617–29. doi: 10.1007/s00221-014-4141-4
33. Schabrun SM, Ridding MC, Galea MP, Hodges PW, Chipchase LS. Primary sensory and motor cortex excitability are co-modulated in response to peripheral electrical nerve stimulation. PLoS ONE. (2012) 7:1–7. doi: 10.1371/journal.pone.0051298
34. Eriksen MB, Frandsen TF. The impact of PICO as a search strategy tool on literature search quality: a systematic review. J Med Libr Assoc. (2018) 106:420–31. doi: 10.5195/jmla.2018.345
35. Page MJ, McKenzie JE, Bossuyt PM, Boutron I, Hoffmann TC, Mulrow CD, et al. The PRISMA 2020 statement: an updated guideline for reporting systematic reviews. BMJ. (2021) 372:n71. doi: 10.1136/bmj.n71
36. Higgins JPT, Thomas J, Chandler J, Cumpston M, Li T, Page MJ WV editors. Cochrane Handbook for Systematic Reviews of Interventions Version 6.3. (2022). Available online at: www.training.cochrane.org/handbook (assessed September 1, 2022).
37. Ouzzani M, Hammady H, Fedorowicz Z, Elmagarmid A. Rayyan-a web and mobile app for systematic reviews. Syst Rev. (2016) 5:1–10. doi: 10.1186/s13643-016-0384-4
38. Cogiamanian F, Vergari M, Pulecchi F, Marceglia S, Priori A. Effect of spinal transcutaneous direct current stimulation on somatosensory evoked potentials in humans. Clin Neurophysiol. (2008) 119:2636–40. doi: 10.1016/j.clinph.2008.07.249
39. Kang YT, Liao YS, Hsieh CL. Different effects of transcutaneous electric nerve stimulation and electroacupuncture at ST36-ST37 on the cerebral cortex. Acupunct Med. (2015) 33:36–41. doi: 10.1136/acupmed-2014-010650
40. Rocchi L, Erro R, Antelmi E, Berardelli A, Tinazzi M, Liguori R, et al. High frequency somatosensory stimulation increases sensori-motor inhibition and leads to perceptual improvement in healthy subjects. Clin Neurophysiol. (2017) 128:1015–25. doi: 10.1016/j.clinph.2017.03.046
41. Zarei AA, Jadidi AF, Lontis ER, Jensen W. Short-term suppression of somatosensory evoked potentials and perceived sensations in healthy subjects following tens. IEEE Trans Biomed Eng. (2021) 68:2261–9. doi: 10.1109/TBME.2021.3051307
42. Giaquinto S, Mascio M, Fraioli L. The physiopathological bases of recovery processes: The bases of stroke rehabilitation. The cassino project. Clin Exp Hypertens. (2002) 24:543–53. doi: 10.1081/CEH-120015331
43. Liu S, Wang ZF, Su YS, Ray RS, Jing XH, Wang YQ, et al. Somatotopic organization and intensity dependence in driving distinct NPY-expressing sympathetic pathways by electroacupuncture. Neuron. (2020) 108:436–50.e7. doi: 10.1016/j.neuron.2020.07.015
44. Sterne JA, Hernán MA, Reeves BC, Savović J, Berkman ND, Viswanathan M, et al. ROBINS-I: A tool for assessing risk of bias in non-randomised studies of interventions. BMJ. (2016) 355:4–10. doi: 10.1136/bmj.i4919
45. Schünemann HJ, Cuello C, Akl EA, Mustafa RA, Meerpohl JJ, Thayer K, et al. GRADE guidelines: 18. How ROBINS-I and other tools to assess risk of bias in nonrandomized studies should be used to rate the certainty of a body of evidence. J Clin Epidemiol. (2019) 111:105–14. doi: 10.1016/j.jclinepi.2018.01.012
46. National Institutes of Health. Quality Assessment Tool for Before-After Studies. (2014). Available online at: https://www.nhlbi.nih.gov/health-pro/guidelines/in-develop/cardiovascular-risk-reduction/tools/cohort (accessed September 1, 2022).
47. Schünemann H, Brozek J, Guyatt G, Oxman A. Handbook for Grading the Certainty of Evidence and the Strength of Recommendations Using the GRADE Approach. Hamilton, ON: GRADE Working Group 2013 (2013).
48. Bolton DAE, Cauraugh JH, Hausenblas HA. Electromyogram-triggered neuromuscular stimulation and stroke motor recovery of arm/hand functions: a meta-analysis. J Neurol Sci. (2004) 223:121–7. doi: 10.1016/j.jns.2004.05.005
49. Hummelsheim H, Maier-Loth ML, Eickhof C. The functional value of electrical muscle stimulation for the rehabilitation of the hand in stroke patients. Scand J Rehabil Med. (1997) 29:3–10.
Keywords: peripheral electrical stimulation, somatosensory evoked potentials (SEP), stroke rehabilitation, sensory and motor recovery, somatosensory cortex
Citation: Mijic M, Jung A, Schoser B and Young P (2022) Use of peripheral electrical stimulation on healthy individual and patients after stroke and its effects on the somatosensory evoked potentials. A systematic review. Front. Neurol. 13:1036891. doi: 10.3389/fneur.2022.1036891
Received: 05 September 2022; Accepted: 20 October 2022;
Published: 18 November 2022.
Edited by:
Carlos Luque-Moreno, University of Seville, SpainReviewed by:
Auwal Abdullahi, Bayero University Kano, NigeriaManuel Albornoz-Cabello, Sevilla University, Spain
Copyright © 2022 Mijic, Jung, Schoser and Young. This is an open-access article distributed under the terms of the Creative Commons Attribution License (CC BY). The use, distribution or reproduction in other forums is permitted, provided the original author(s) and the copyright owner(s) are credited and that the original publication in this journal is cited, in accordance with accepted academic practice. No use, distribution or reproduction is permitted which does not comply with these terms.
*Correspondence: Marko Mijic, bWFya28ubWlqaWMmI3gwMDA0MDttZWQudW5pLW11ZW5jaGVuLmRl