- 1Hurvitz Brain Sciences Research Program, Sunnybrook Research Institute, Sunnybrook Health Sciences Centre, Toronto, ON, Canada
- 2Division of Neurology, Department of Medicine, University of Toronto, Toronto, ON, Canada
- 3Sleep Laboratory, Sunnybrook Health Sciences Centre, Toronto, ON, Canada
- 4Washington University School of Medicine, St. Louis, MO, United States
- 5Sleep Medicine Division, Department of Neurology, Louisiana State University Health Sciences Center, Shreveport, LA, United States
- 6Ochsner LSU Health Sleep Medicine Center, Shreveport, LA, United States
Background: Undiagnosed obstructive sleep apnea (OSA) is prevalent in neurological practice and significantly contributes to morbidity and mortality. OSA is prevalent in US adults and causes poor quality sleep and significant neurocognitive, cardiovascular, and cerebrovascular impairments. Timely treatment of OSA reduces cardio-cerebrovascular risks and improves quality of life. However, most of the US population has limited systematic access to sleep medicine care despite its clinical significance.
Focus: We discuss the importance of systematic screening, testing, and best-practice management of OSA and hypoventilation/hypoxemia syndromes (HHS) in patients with stroke, neurocognitive impairment, and neuromuscular conditions. This review aims to introduce and describe a novel integrated Mobile Sleep Medicine (iMSM) care model and provide the rationale for using an iMSM in general neurological practice to assist with systematic screening, testing and best-practice management of OSA, HHS, and potentially other sleep conditions.
Key points: The iMSM is an innovative, patient-centered, clinical outcome-based program that uses a Mobile Sleep Medicine Unit—a “sleep lab on wheels”—designed to improve access to OSA management and sleep care at all levels of health care system. The protocol for the iMSM care model includes three levels of operations to provide effective and efficient OSA screening, timely testing/treatment plans, and coordination of further sleep medicine care follow-up. The iMSM care model prioritizes effective, efficient, and patient-centered sleep medicine care; therefore, all parties and segments of care that receive and provide clinical sleep medicine services may benefit from adopting this innovative approach.
Introduction
Undiagnosed and untreated sleep-related breathing disorders (SDB) are prevalent in neurological practice and contribute significantly to the development of morbidity and mortality (1–10). In the US, 70% of adults report obtaining insufficient/“unprotected” sleep at least one night a month, and 11% report obtaining insufficient sleep every night. Sleep-related problems are estimated to affect 50–70 million Americans of all ages and socioeconomic classes (11, 12).
Approximately 25 million US adults suffer from obstructive sleep apnea (OSA)—a leading sleep-disruptive force that breaks down sleep protection and causes insufficient sleep, significant neurocognitive, cardiovascular, and cerebrovascular (CCV) impairments in humans (13). The timely treatment of OSA with positive airway pressure (PAP) therapy protects human sleep, reduces cardio-cerebrovascular risks and improves the quality of human life (4–10, 14).
Currently, 80% of the general US population and about 36 million annually hospitalized patients have limited systematic access to sleep medicine care despite alarming statistics affirming the significant prevalence of OSA in vulnerable populations.
While the prevalence of OSA in an unselected adult population is generally estimated at ~5% (4, 15, 16), the prevalence of OSA in some medical and neurological populations is significantly elevated, ranging from 10 to 82% in patients with epilepsy (1–3), from 50 to 94% in patients with stroke and transient ischemic attack (TIA) (4–10), from 11 to 82% in patients with arterial hypertension (HTN) (17, 18), from 30 to 40% in patients with the acute coronary syndrome (ACS) and myocardial infarction (MI) (4, 14), from 3 to 49% in patients with atrial fibrillation (19–22), from 10 to 43% in patients with congestive heart failure (CHF) (4, 23–25), 23% in patients with traumatic brain injury (TBI) (26), from 21 to 38% in patients with multiple sclerosis (MS) (27, 28), from 20 to 56% in patients with Parkinson's disease (PD) (29–34), 40% in patients with Alzheimer's dementia (35), from 11 to 36% in patients with neuromuscular conditions (36), and 17 to 76% in patients with amyotrophic lateral sclerosis (ALS) (37, 38).
Timely delivered PAP therapy appears to have beneficial effects on patients with OSA in the general population (39, 40), ischemic stroke and TIA (41–43), epilepsy (44–46), HTN ACS/MI (47–50), atrial fibrillation (51–53), CHF (54–56), TBI (26), multiple sclerosis (27), PD (57), Alzheimer's dementia (35, 58–60) and neuromuscular conditions (61, 62), and ALS (16, 62–65).
Timely delivered non-invasive airway pressure therapy (NIPAP) appears to produce beneficial effects on patients with sleep-related hypoventilation/hypoxemia syndromes (HHS) in neuromuscular disorders (61, 62), obesity hypoventilation (66), and ALS (16, 62–65).
The goals of this review are to (1) introduce and describe a novel, integrated Mobile Sleep Medicine care model (iMSM); (2) provide the rationale for the implementation of iMSM in general neurological practice to assist with systematic screening, testing, and the best-practice management of the most common SDB (OSA and HHS) in patients with stroke, neurocognitive impairment, and neuromuscular conditions (67).
The rationale for implementation of iMSM in general neurological practice
Here, we discuss the importance of systematic screening, testing, and best-practice management for OSA and HHS in patients with (1) stroke, (2) neurocognitive impairment, and (3) neuromuscular conditions.
Sleep-disordered breathing in patients with stroke
OSA causes intermittent pauses in breathing (apneas) during sleep, exposing the cardiovascular system to recurrent physiological stressors that activate the sympathetic nervous system, raise blood pressure, and impair vascular endothelial function (68). Such physiological alterations closely link OSA with vascular disease, and OSA is an independent risk factor for HTN (69), atrial fibrillation (70), MI (71), stroke (72), and mortality (73).
OSA is prevalent after stroke/TIA
OSA occurs in more than 70% of stroke/TIA survivors (6), and even 3 years after stroke, the prevalence of OSA remains just as high (74), suggesting that screening for OSA at any point after a cerebrovascular event may provide clinical benefit. Rates of OSA do not vary according to the method of detection used (e.g., polysomnography vs. portable sleep equipment) (6).
OSA negatively impacts outcomes post-stroke
Left untreated, moderate-to-severe post-stroke OSA garners a three-fold increase in mortality (41) and a five-fold increase in recurrent vascular events (75). Moreover, a diagnosis of OSA is an independent predictor of worse functional outcomes at hospital discharge (76, 77) and is associated with longer hospitalization in the rehabilitation setting (78).
Post-stroke treatment of OSA improves outcomes
Treatment of post-stroke OSA using continuous positive airway pressure (CPAP) in randomized trials has been shown to improve functional, and motor outcomes (79–84), increase quality of life (85), reduce daytime sleepiness (81, 85), improve mood (86), and enhance cognition (59, 87). In observational studies, compliance with CPAP has been shown to reduce incident vascular events (75) and mortality (41). Outside the stroke/TIA setting, RCTs have demonstrated beneficial effects of CPAP for blood pressure (88) and lipid control (89).
Clinicians do not routinely screen for OSA after stroke or TIA
Despite its high prevalence and detrimental effect on health, OSA remains underdiagnosed and undertreated after stroke/TIA (90). Detection of OSA after stroke/TIA is challenging because OSA presents atypically in patients with cerebrovascular disease (e.g., the absence of excessive daytime sleepiness or obesity) (91, 92), not surprisingly, stroke clinicians infrequently refer patients for sleep-related investigations (90). Since commonly used screening questionnaires show poor correlations with polysomnography findings in stroke patients (93), objective measures are best suited to accurately diagnose OSA.
In traditional sleep medicine testing model the lack of availability of in-laboratory polysomnography (“Gold Standard”) is a major barrier to efficiency screening for OSA in hospital settings
Technologist-monitored, in-laboratory sleep studies (polysomnography or PSG) are the “Gold Standard” for diagnosing OSA, but limited availability of polysomnography and lengthy wait times frequently prohibit timely evaluations (90, 94). In addition, some patients are unwilling to spend a night in a sleep laboratory. Finally, polysomnography involves costly equipment, an on-site technologist, and high healthcare expenditures.
OSA can be accurately detected using a simple, portable sleep monitoring (“Silver Standard”)
These devices are much less expensive, more accessible, and more convenient and demonstrate good diagnostic performance compared with in-laboratory polysomnography in adult patients with a high pretest probability of moderate to severe obstructive sleep apnea (e.g., the post-stroke/TIA population) (95, 96).
Home/hospital sleep apnea testing (HSAT; “Silver Standard”) has been shown to improve outcomes after stroke/TIA
In a randomized controlled trial involving 250 consecutively recruited stroke/TIA patients, patients were randomized to undergo home/hospital sleep apnea testing (HSAT) vs. in-laboratory polysomnography. Those randomized to HSAT had higher rates of OSA diagnosis and treatment, reduced daytime sleepiness, and improved functional outcomes. Moreover, a cost-effectiveness analysis, including the cost of initial diagnostic tests, broken/lost equipment, etc., revealed that HSAT was economically attractive for detecting OSA compared with in-laboratory sleep testing (97). Overall, this study suggested that ambulatory “Silver Standard” approach to sleep testing may improve clinical outcomes in stroke/TIA patients. The cost-effectiveness of an ambulatory “Silver Standard” approach to sleep testing via the mobile sleep unit would likely extend to other clinical populations at high risk for OSA.
Sleep disorder breathing in Alzheimer's disease/dementia
Cognitive impairment has a devastating impact on society
Approximately 747,000 Canadians live with Alzheimer's disease or another form of dementia (98), and the associated care costs ~$33 billion annually (98). Alzheimer's disease (AD) is the leading cause of dementia worldwide (99). Vascular cognitive impairment (VCI) is the clinical syndrome in which cognitive impairment, encompassing mild cognitive impairment and dementia, can be attributed to vascular disease such as clinically overt stroke and/or silent brain infarction (100, 101). Vascular brain injury accounts for up to 33% of dementia risk according to autopsy studies (102) and frequently co-exists with AD (103), making VCI a significant public health issue (101). Mild cognitive impairment (MCI) causes cognitive problems that do not interfere with everyday life. However, amnestic MCI increases the risk of developing AD (98).
OSA is closely linked with AD and VCI
OSA gives rise to physiological alterations that contribute to the cognitive impairment seen in both AD and VCI, such as transient sympathetic activation, systemic inflammation, endothelial dysfunction in the vasculature of the brain (104), and impaired sleep-dependent memory consolidation through sleep fragmentation (105). Sleep fragmentation from OSA can also lead to impaired glymphatic and vascular drainage of amyloid (106, 107), which is postulated to contribute to the development of AD (108). Furthermore, OSA is strongly associated with cerebrovascular disease and is an independent risk factor for high blood pressure (69), overt (73), and covert stroke (109).
OSA is an independent risk factor for AD, VCI, and MCI
In elderly patients without dementia at baseline, the presence of OSA is an independent risk factor for the development of MCI or dementia (110), and a meta-analysis suggested that OSA is an important modifiable risk factor for dementia and other cognitive impairment (111). Furthermore, given the close association of neurodegeneration with vascular disease, treatment of OSA—a well-established vascular risk factor (68)—may also have important implications for brain health even beyond the potentially beneficial effects on cognition and daily function.
Obstructive sleep apnea is prevalent in AD/VCI
More than 70% of patients with VCI endorse symptoms consistent with OSA (112). Moreover, prior work has demonstrated that nearly 90% of patients with Alzheimer's disease have obstructive sleep apnea when objectively tested (113). Again, rates of OSA do not vary with the method of detection (i.e. “Gold Standard” vs. “Silver Standard”) (6).
Treatment of OSA using CPAP improves cognition
OSA is treated with CPAP, which provides mild air pressure to maintain airway patency during sleep. A review of five systematic reviews and meta-analyses concluded that treatment with CPAP improved attention/vigilance, executive dysfunction, memory, and global cognitive functioning in non-demented individuals (114). A more recent systematic review demonstrated a protective effect that treatment with CPAP has on MCI and AD incidence (115). Several studies have suggested that treating OSA using CPAP in AD patients may slow the rate of cognitive decline (58, 59, 116). In the only randomized controlled trial examining subjects with VCI and OSA, CPAP-treated patients showed improved attention and executive functioning compared to controls who did not receive CPAP (87).
Clinicians do not routinely screen for OSA in patients with AD/VCI
Despite its high prevalence and negative impact on cognition, OSA remains underdiagnosed and undertreated.
Use of polysomnography within traditional sleep medicine testing model is associated with many barriers
Technologist-monitored, overnight, level 1 PSG (“Gold Standard”) is the current standard tool for diagnosing sleep disorders, but high costs, lengthy wait times, and patient unwillingness to spend a night in a sleep laboratory frequently prohibit timely assessments (117). Moreover, the traditional sleep medicine testing model is particularly inconvenient for patients with cognitive impairment, who may depend on others for care and may require a familiar environment to sleep and avoid delirium.
HSAT (“Silver Standard”), has been extensively validated against in-laboratory PSG for detecting obstructive sleep apnea (95, 96, 118). In addition, an unattended sleep study (“Silver Standard”) may be potentially less expensive and more accessible.
HSAT is feasible for use in patients with AD/MCI
Our prior work has demonstrated that the use of unattended sleep study (“Silver Standard”) is feasible in patients with AD/MCI/VCI; >85% of patients who attempted to use unattended sleep study (“Silver Standard”) were able to obtain analyzable data (119).
Sleep-disordered breathing in neuromuscular disorders
In patients with progressive neuromuscular disease (NMD), respiratory failure caused by respiratory muscle weakness is the most common cause of death. Respiratory muscle weakness, changes in chest wall mechanics, and difficulty with airway secretion clearance leads to ineffective alveolar ventilation and both acute and chronic respiratory failure (120).
Physiological change in sleep
During sleep, minute ventilation decreases, muscle activity alters, respiratory workload increases (121), ventilatory response to hypoxemia and hypercapnia declines (122), and upper airway resistance increases (123). In REM sleep, skeletal muscle tone is abolished, while the diaphragm is relatively spared (121). Patients with NMD cannot compensate for physiological changes in sleep, and inadequate alveolar ventilation may first occur during REM sleep. As the disease progresses, hypoventilation may extend to non-REM sleep, and eventually, daytime hypercapnia may occur.
Prevalence of SDB
The prevalence of SDB is high in most NMDs, and chronic respiratory failure occurs with disease progression. A prior study using a home PSG sleep study without CO2 monitoring showed a high prevalence of SDB in a group of chronic neuromuscular disorders. The prevalence of SDB with respiratory disturbance index (RDI) >15/h was 42%, higher than the general population. Respiratory events were primarily hypopneas, and 23% of patients had nocturnal hypoxemia (124). Patients with NMD usually have a normal ventilatory drive (125). Using EMG to monitor the activity of respiratory muscles during PSG in patients with NMD revealed that hypopneas that happened in REM sleep were mostly “central” in nature due to reduced muscle activity, and that nocturnal hypoxemia was inversely correlated with diaphragm strength (126). Studies have confirmed that nocturnal hypoxemia and hypercapnia in REM sleep are common in patients with NMD (127, 128).
Non-invasive ventilation (NIV) significantly benefits the quality of life, survival, and respiratory status in different NMD types
Studies have provided evidence that NIV significantly prolonged survival in patients with ALS (129–132). Early initiation of NIV slowed the rate of forced vital capacity (FVC) decline (131) and improved survival (131, 133). Berlowitz et al. found survival benefits of 11–15.5 months in both bulbar and non-bulbar ALS patients (129). Using NIV ≥4 h were associated with longer survival for patients with ALS (134). NIV was well-tolerated and may improve quality of life and survival in patients with Duchenne muscular dystrophy (DMD) (135, 136) and SMA (137).
Polysomnography (PSG) with capnometry (“Gold Standard”) plays a role in determining the nature and severity of SDB in NMD
SDB in NMD patients includes pseudo-central or diaphragmatic SDB, central sleep apnea, obstructive sleep apnea, nocturnal hypoxemia, and hypoventilation in sleep. Non-invasive ventilation is indicated at the first sign of hypoventilation. A sleep study should be considered with at least one symptom and/or sign related to respiratory muscle weakness such as dyspnea, tachypnea, orthopnea, disturbed sleep, morning headaches, accessory muscle use at rest, paradoxical breathing, daytime fatigue, or daytime sleepiness (ESS > 9) (138). A PSG (“Gold Standard”) can evaluate respiratory muscle weakness during sleep and confirm the need for NIV. The absence of nocturnal hypoxemia does not exclude nocturnal hypercapnia. Nocturnal hypercapnia predicts impending daytime hypercapnia and is an indicator for nocturnal NIV before daytime hypercapnia occurs (139).
Scoring respiratory events during sleep in patients with NMD requires specific expertise. Patients with NMD usually present a decrease in airflow and respiratory effort with or without a reduction in pulse oximetry (SpO2). These events may be associated with increased transcutaneous carbon dioxide (TcCO2). Paradoxical breathing may result from respiratory muscle weakness and should not be interpreted as “obstructive events” (126). Periods of “reduced ventilation” or paradoxical breathing, especially during REM sleep, justify the initiation of NIV in patients with NMD (139).
According to the 2017 AASM guidelines, an unattended sleep study (“Silver Standard”) is not recommended in adults with NMD or known or suspected hypoventilation (140). However, as technology advances, “Silver Standard” sleep study devices that can accurately determine sleep stages plus capnography may be used to evaluate SDB in patients with NMD. The integrated Mobile Sleep Medicine Unit is designed to overcome the shortcomings of the traditional sleep medicine testing model and can deliver the entire spectrum of sleep testing to individuals with NMD. The iMSM includes sleep testing and PAP titration technology, which can be delivered to all levels of patient care from hospital to home, under the supervision of a board-certified sleep medicine physician. It means that we can deliver the Mobile Sleep Unit with portable PSG, capnography, and PAP titration directly to a patient's home and provide virtual monitoring for ventilators if necessary.
In-lab titration of NIV by PSG is not required but recommended by AASM before initiating NIV to identify optimal settings, reduce patient-ventilator asynchrony and improve treatment tolerance
Guidelines to initiate ventilation in NMD defined in 1999 include the presence of symptomatic daytime hypercapnia (PaCO2 ≥ 45 mmHg), nocturnal desaturation (SpO2 ≤ 88% for 5 consecutive minutes), MIP < −60 cmH2O, or FVC < 50% predicted (141). Commonly used NIV modes include Bilevel PAP with a backup rate (BPAP ST), or volume assured pressure support (VAPS) (66). Titration of non-invasive ventilation by attended polysomnography is the gold standard to identify effective parameters to correct SDB, including hypoventilation as well as central, pseudo-central, and obstructive sleep apnea. Patient-ventilator desynchrony may include ineffective effort, triggering asynchrony, cycling asynchrony, etc (142, 143). An in-lab titration of NIV by polysomnography study may improve patients' NIV treatment tolerance by observing and reducing patient-ventilator asynchrony (142).
The AASM consensus in 2010 non-invasive positive pressure ventilation (NPPV) titration with PSG to determine an effective level of nocturnal ventilatory support in patients with chronic alveolar hypoventilation. If the patient is started on NPPV empirically, a PSG would be considered necessary to confirm that the final settings are effective and to identify desynchronization or arousals from leaks (144).
However, logistic issues prevent attended sleep studies from being utilized in patients with NMD. These issues include long wait times, mobility, transportation, lift use, insurance coverage, etc.
Telemonitoring is one of the most revolutionary changes in home-assisted ventilation
Evidence is emerging that telehealth provides timely and cost-effective support for an individual with motor neuron disease (MND). Respiratory assist devices (RAD) and portable home ventilators transmit patient usage and home ventilation machines' efficacy data to a cloud-based platform via wireless networks. These enable physicians to access efficacy data updated daily via a cloud-based platform to provide personalized ventilation machine management at home and individualized care. Physicians may view breath-by-breath waveforms, trends in clinical data, and precise therapy values over periods of days, weeks, and months within 1 to 24-h windows.
Through telemonitoring, physicians can monitor disease progression, titrate modes and settings in a monitored environment and in a stepwise fashion, depending on the patient's symptoms and tolerance. In addition to optimizing device function, clinicians can quickly identify and troubleshoot ventilation issues.
Studies have shown that patients with ALS can be well-managed at home via telemonitoring. Benefits of telemonitoring include reduced ED visits and hospital admissions, increased survival, and improved functional status in patients with ALS (145, 146).
The integrated mobile sleep medicine care model
The integrated Mobile Sleep Medicine Care Model (iMSM) is an innovative, progressive, patient-centered, integrative, complete cycle, clinical-outcome-based program that uses a Mobile Sleep Medicine Unit as a methodological tool—a “sleep lab on wheels”—designed to improve systematic access to OSA management and sleep care for approximately 80% of Americans at all healthcare levels, from hospital to home (67).
The protocol for the iMSM delivery model includes three levels of operations (see Figure 1):
1) Screening.
2) Testing/Treatment.
3) Follow-up.
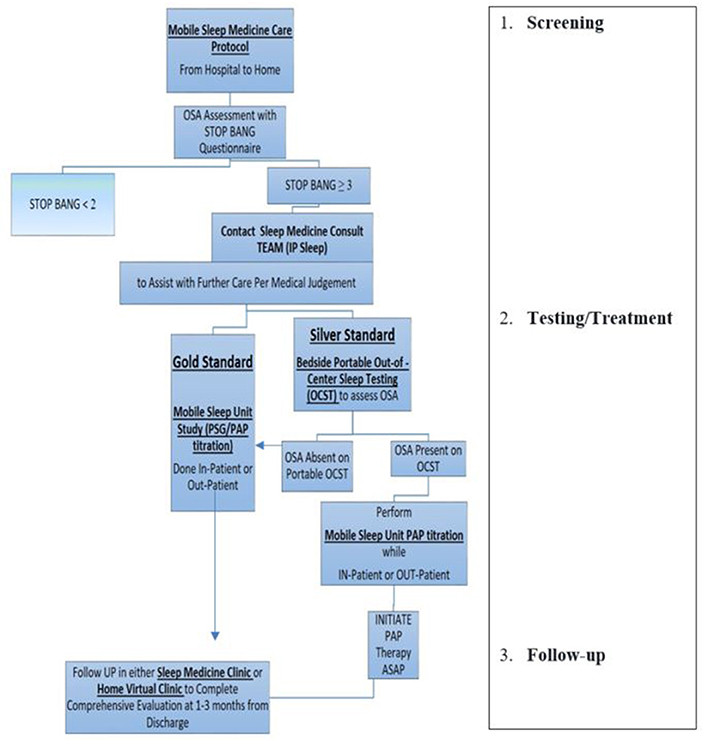
Figure 1. Example of mobile sleep medicine care protocol. (1) Screening: Designed to provide effective and efficient SDB screening for patients admitted to hospital (or any other health care facility, e.g., rehab, specialty/subspecialty clinic, etc.), including patients with a high risk of CCV (stroke, MI, CHF, atrial fibrillation, hypertension, preeclampsia, etc.). After completing SDB screening either in-person, via EMR, or via Tele-Virtual/My Chart system, the Primary Team communicates with the Mobile Sleep Medicine Team/ in-patient Sleep Medicine Consulting Team (IP Sleep) to request sleep consultation for further management. (2) Testing/Treatment: The board-certified sleep medicine physician from the integrated mobile sleep medicine Team will discuss the sleep study results with patients and coordinate further management. Designed to deliver timely sleep medicine expertise to patients who screen positive for OSA. The IP sleep/mobile sleep medicine team evaluates patients and develops the diagnostic and treatment plan using mobile sleep unit technology. (3) Follow-up: IP sleep/mobile sleep medicine team coordinates further sleep medicine care in a sleep clinic, sleep lab, home health, and virtual telemedicine health. https://d11tooehygcg9z.cloudfront.net/My.AASM/AgentsOfChange/587417.pdf; https://aasm.org/winners-of-inaugural-change-agents-competition-propose-new-approaches-for-sleep-apnea-care.
Components of the mobile sleep medicine unit
➢ Module 1. “Gold Standard”: Mobile Polysomnography (mPSG).
➢ Module 2. “Silver Standard”: Portable Out-of-Center Sleep Testing (OCST) (Nox-T3, Embletta, Stardust, ApneaLink Air, MediByte Jr, Alice, Cerebra Sleep System, BresoDx, etc.).
• Synonyms:
– Cardiopulmonary studies (CP).
– hospital/home sleep ambulatory testing (HSAT).
– (OCST) = (CP) = HSAT.
➢ Module 3. PAP titration unit: CPAP/BiPAP/AVAPS/ASV.
Mobile Sleep Unit—“sleep lab on wheels”—is a technological, methodological tool designed to deliver sleep medicine evaluation directly to the patient's bedside. The mobile sleep unit assembles with three functional technological modules representing modern sleep technology, which is the standard of care in the sleep medicine (67).
Mobile sleep medicine unit
Module 1: “Gold Standard”: Mobile PSG-attended type 1 sleep testing device.
• Mobility and portability: Full sleep lab service delivered directly to the patient's bedside, either inside the hospital or at the patient's place of residence.
Module 2: “Silver Standard”: Unattended type 3 sleep testing device named in sleep literature (detailed above).
• Mobility and portability: Type 3 (four channels) sleep lab service is delivered directly to the patient's bedside, either inside the hospital or at the patient's place of residence, with an average set-up time of 5–10 mins.
Module 3: PAP titration unit: CPAP/BiPAP/AVAPS/ASV: Provides titration sleep study “on the spot.”
• Used for PAP titration in combination with Module 1/mobile PSG (“Gold Standard”) to perform mobile-attended PSG/Split or/PAP titration studies.
Cost: Vertical integration payment system (VIPS) model
Designed to obtain collections from sleep-related operations performed at each level of care from hospital to sleep lab/home:
1) Technical charges and professional charges will be collected based on appropriate CTP codes for the type of study performed.
2) Sleep studies (unattended and attended).
3) Clinical in-patient sleep consults and outpatient sleep clinical visits.
a. Collections from each level will be combined to 100% and directed to support mobile sleep unit operations from hospital to home.
b. The sources of reimbursement obtained from each level of care.
a) Acute hospital level of care
i. Screening/evaluation for SDB will bring up diagnosis-related group reimbursement based on the following.
ii. Elevated complexity of comorbidities rule and increased case mix index.
iii. Improvement of hospital performance statistics due to a significant reduction in readmission rates in sensitive CCV population (CHF, chronic respiratory failure, obesity, hypoventilation, stroke, atrial fibrillation, MI, hypertension, seizure disorders, myasthenia gravis, ALS, etc.). All admitted patients in the CCV population are at high risk for SDB and should be evaluated by the inpatient mobile sleep medicine team (IP Sleep Team). The IP sleep team would decide the appropriate delivery (i.e., Gold vs. Silver Standard) for a given patient based on local standards of care and the availability of devices in the community. The mobile sleep medicine model provides flexibility in diagnosing and delivering necessary sleep care without delay.
iv. 1–33% of collections from each level will be directed to support mobile sleep unit operations from hospital to home. These are approximate, proposed figures, which can be modified based on local administrative protocols for utilization of services. Each given IP sleep team would choose its own proportion of collections to fit its needs for sustained clinical operations and to make the service self-sufficient based on local finances, insurance coverage, administrative support, grants, etc.
b) Sleep lab/sleep center: Sleep medicine referrals from inpatient sleep medicine consulting service (IP sleep):
• Will boost the sleep referral base to the internal sleep lab/center by about 1,300 patients per year (5 consults per day × 5 days per week × 52 weeks).
• Will prevent “referral leaks” to external systems and maintain the integrity of collections.
• 1–33% of collections from each level will be directed to support Mobile Sleep Unit Operations from hospital to home.
c) Home health integration: Patient-centered, unattended, or/and attended sleep studies will be conducted at the patient's residence (house, rehab, nursing home, etc.) and achieve the following:
• Integrate mobile sleep medicine care into the home health care model.
• Reduce the operational costs by 50% with a flexible technician: patient ratio (from 1:3 to 1:10) for unattended studies with strong reimbursement for each study performed.
• 1–33% of collections from each level will be directed to support mobile sleep unit operations from hospital to home.
The mobile sleep medicine care model prioritizes effective, efficient, and patient-centered sleep medicine care; therefore, all parties and segments of care that receive and provide clinical sleep medicine services will benefit in various measurable ways (67).
A. Benefits for patients
1) Patient-centered sleep care is delivered directly and conveniently to a patient at any setting/level of care: hospital, home, virtual telemedicine clinic, or in-person clinic.
2) The iMSM improves the overall patient experience by bringing sleep medicine expertise and testing directly to the patient's bedside without the unnecessary delays in care currently observed in the traditional sleep medicine model (e.g., self-scheduling for sleep clinic and sleep lab with long waiting times, and/or multiple missed/canceled appointments, etc.).
3) Improved sleep quality, sleep-related quality of life, participation in recovery and rehab activities due to controlled OSA-related issues: daytime sleepiness, fatigue, concentration and/or memory, altered mental status, delirium, and dyspnea.
B. Benefits for Hospital/Healthcare System
1) Screening/evaluation for SDB will upgrade the case-mix index and bring up diagnosis-related group reimbursement based on:
a) Elevated complexity of comorbidities rule and increased case mix index.
b) Improvement of hospital performance statistics due to:
i. Significant reduction in in-hospital mortality and readmission rates in CCV-sensitive populations (CHF, chronic respiratory failure, obesity hypoventilation, Stroke, atrial fibrillation, MI, hypertension, seizure disorders, neuromuscular disease, myasthenia gravis, ALS, etc.).
ii. Shortened the length of the following:
a. The intensive care unit stays.
b. Hospital stays (i.e., patients with OSA, OHS, post-operative respiratory failure, COPD, CHF, etc.).
iii. Prevention of escalation in the level of care, intubation, transfer to the intensive care unit, a rapid response team.
iv. Prevention of hypoxemia and/or hypoventilation.
v. Control of referral base and prevention of referral leaks (increase referral base to sleep center by 1300 patients per year).
C. Benefits for payors
1) Low cost for improved access to Neuro-Sleep Medicine care for pediatric and adult patients.
2) Reduced costs and more effective management of CCV, AD, and NMD.
D. Benefits for the Academic Neuro-Sleep Medicine Field:
1) Expansion of neurosomnology into all medical settings and levels of care with opportunities to monitor relevant SDB-related clinical outcomes and measure responses to targeted clinical interventions in real time while a patient is moving via levels of care;
2) Establishing the methodological basis for the development of digital evidence-based precision neuro-sleep medicine:
a) To effectively control and manage pertinent comorbidities in CCV, AD, and NMD.
b) Improve recovery and rehabilitation.
3) Removal of the “stigma” of being an “Outpatient Only” specialty with minimal impact on patient's clinical outcomes.
E. National health benefits
1) Overall reduction of SDB-related morbidity and mortality.
2) Data from iMSM would contribute to the development of evidence-based precision sleep medicine.
The integrated iMSM, if implemented in the general neurological practice, would provide the systematic screening, testing, and best-practice management of the most common sleep-related breathing disorders: OSA, HHS and potentially other sleep disorders.
We anticipate that iMSM with mobile sleep unit methodology has the potential for a positive, measurable impact on all parties involved in neurological care, including the neurological patient, the hospital/healthcare system, and the fields of neurology and sleep medicine.
Author's note
The mobile sleep medicine model was presented during the 2021 AASM Sleep Disruptors competition and won the People's Choice Award. Information can be found at the following links: https://d11tooehygcg9z.cloudfront.net/My.AASM/AgentsOfChange/587417.pdf; https://aasm.org/winners-of-inaugural-change-agents-competition-propose-new-approaches-for-sleep-apnea-care.
Author contributions
All authors listed have made a substantial, direct, and intellectual contribution to the work and approved it for publication.
Funding
MB has received in-kind support for his research program from Braebon Medical Corporation, Interaxon, ResMed, and BresoTec. The funders were not involved in the study design, collection, analysis, interpretation of data, the writing of this article, or the decision to submit it for publication.
Conflict of interest
The authors declare that the research was conducted in the absence of any commercial or financial relationships that could be construed as a potential conflict of interest.
Publisher's note
All claims expressed in this article are solely those of the authors and do not necessarily represent those of their affiliated organizations, or those of the publisher, the editors and the reviewers. Any product that may be evaluated in this article, or claim that may be made by its manufacturer, is not guaranteed or endorsed by the publisher.
References
1. Manni R, Terzaghi M, Arbasino C, Sartori I, Galimberti CA, Tartara A. Obstructive sleep apnea in a clinical series of adult epilepsy patients: frequency and features of the comorbidity. Epilepsia. (2003) 44:836–40. doi: 10.1046/j.1528-1157.2003.55702.x
2. Malow BA, Levy K, Maturen K, Bowes R. Obstructive sleep apnea is common in medically refractory epilepsy patients. Neurology. (2000) 55:1002–7. doi: 10.1212/WNL.55.7.1002
3. Chihorek AM, Abou-Khalil B, Malow BA. Obstructive sleep apnea is associated with seizure occurrence in older adults with epilepsy. Neurology. (2007) 69:1823–7. doi: 10.1212/01.wnl.0000279334.78298.d5
4. Lee W, Nagubadi S, Kryger MH, Mokhlesi B. Epidemiology of obstructive sleep apnea: a population-based perspective. Expert Rev Respir Med. (2008) 2:349–64. doi: 10.1586/17476348.2.3.349
5. Bassetti C, Aldrich MS, Chervin RD, Quint D. Sleep apnea in patients with transient ischemic attack and stroke: a prospective study of 59 patients. Neurology. (1996) 47:1167–73. doi: 10.1212/WNL.47.5.1167
6. Johnson KG, Johnson DC. Frequency of sleep apnea in stroke and TIA patients: a meta-analysis. J Clin Sleep Med JCSM. (2010) 6:131–7. doi: 10.5664/jcsm.27760
7. Turkington PM, Bamford J, Wanklyn P, Elliott MW. Prevalence and predictors of upper airway obstruction in the first 24 hours after acute stroke. Stroke. (2002) 33:2037–42. doi: 10.1161/01.STR.0000023576.94311.27
8. Harbison J, Ford GA, James OF, Gibson GJ. Sleep-disordered breathing following acute stroke. QJM. (2002) 95:741–7. doi: 10.1093/qjmed/95.11.741
9. Iranzo A, Santamaria J, Berenguer J, Sanchez M, Chamorro A. Prevalence and clinical importance of sleep apnea in the first night after cerebral infarction. Neurology. (2002) 58:911–6. doi: 10.1212/WNL.58.6.911
10. Parra O, Arboix A, Bechich S, Garcia-Eroles L, Montserrat JM, Lopez JA, et al. Time course of sleep-related breathing disorders in first-ever stroke or transient ischemic attack. Am J Respir Crit Care Med. (2000) 161:375–80. doi: 10.1164/ajrccm.161.2.9903139
11. Centers for Disease Prevention. Perceived insufficient rest or sleep—four states, 2006. MMWR Morb Mortal Wkly Rep. (2008) 57:200–3.
12. Chapman DP, Wheaton AG, Perry GS, Sturgis SL, Strine TW, Croft JB. Household demographics and perceived insufficient sleep among US adults. J Community Health. (2012) 37:344–9. doi: 10.1007/s10900-011-9451-x
13. Colten HR, Altevogt BM, eds. Sleep Disorders and Sleep Deprivation: An Unmet Public Health Problem. Washington (DC): Elseiver (2006).
14. Zhao LP, Tan A, Tai BC, Loo G, Tan HC, Lee CH. Effects of gender on the prevalence of obstructive sleep apnea in patients with coronary artery disease. J Clin Sleep Med JCSM. (2014) 10:1279–84. doi: 10.5664/jcsm.4276
15. Young T, Palta M, Dempsey J, Skatrud J, Weber S, Badr S. The occurrence of sleep-disordered breathing among middle-aged adults. N Engl J Med. (1993) 328:1230–5. doi: 10.1056/NEJM199304293281704
16. Young T, Peppard PE, Gottlieb DJ. Epidemiology of obstructive sleep apnea: a population health perspective. Am J Respir Crit Care Med. (2002) 165:1217–39. doi: 10.1164/rccm.2109080
17. Pedrosa RP, Barros IML, Drager LF, Bittencourt MS, Medeiros AKL, Carvalho LL, et al. OSA is common and independently associated with hypertension and increased arterial stiffness in consecutive perimenopausal women. Chest. (2014) 146:66–72. doi: 10.1378/chest.14-0097
18. Muxfeldt ES, Margallo VS, Guimarães GM, Salles GF. Prevalence and associated factors of obstructive sleep apnea in patients with resistant hypertension. Am J Hypertens. (2014) 27:1069–78. doi: 10.1093/ajh/hpu023
19. Gami AS, Friedman PA, Chung MK, Caples SM, Somers VK. Therapy Insight: interactions between atrial fibrillation and obstructive sleep apnea. Nat Clin Pract Cardiovasc Med. (2005) 2:145–9. doi: 10.1038/ncpcardio0130
20. Guilleminault C, Connolly SJ, Winkle RA. Cardiac arrhythmia and conduction disturbances during sleep in 400 patients with sleep apnea syndrome. Am J Cardiol. (1983) 52:490–4. doi: 10.1016/0002-9149(83)90013-9
21. Gami AS, Hodge DO, Herges RM, Olson EJ, Nykodym J, Kara T, et al. Obstructive sleep apnea, obesity, and the risk of incident atrial fibrillation. J Am Coll Cardiol. (2007) 49:565–71. doi: 10.1016/j.jacc.2006.08.060
22. Szymański FM, Płatek AE, Karpiński G, Kozluk E, Puchalski B, Filipiak KJ. Obstructive sleep apnoea in patients with atrial fibrillation: prevalence, determinants and clinical characteristics of patients in Polish population. Kardiol Pol. (2014) 72:716–24. doi: 10.5603/KP.a2014.0070
23. Sekizuka H, Osada N, Miyake F. Sleep disordered breathing in heart failure patients with reduced versus preserved ejection fraction. Heart Lung Circ. (2013) 22:104–9. doi: 10.1016/j.hlc.2012.08.006
24. Patidar AB, Andrews GR, Seth S. Prevalence of obstructive sleep apnea, associated risk factors, and quality of life among Indian congestive heart failure patients: a cross-sectional survey. J Cardiovasc Nurs. (2011) 26:452–9. doi: 10.1097/JCN.0b013e31820a048e
25. Schulz R, Blau A, Borgel J, Duchna HW, Fietze I, Koper I, et al. Sleep apnoea in heart failure. Eur Respir J. (2007) 29:1201–5. doi: 10.1183/09031936.00037106
26. Castriotta RJ, Wilde MC, Lai JM, Atanasov S, Masel BE, Kuna ST. Prevalence and consequences of sleep disorders in traumatic brain injury. J Clin Sleep Med JCSM. (2007) 3:349–56. doi: 10.5664/jcsm.26855
27. Braley TJ, Segal BM, Chervin RD. Obstructive sleep apnea and fatigue in patients with multiple sclerosis. J Clin Sleep Med JCSM. (2014) 10:155–62. doi: 10.5664/jcsm.3442
28. Brass SD Li CS, Auerbach S. The underdiagnosis of sleep disorders in patients with multiple sclerosis. J Clin Sleep Med. (2014) 10:1025–31. doi: 10.5664/jcsm.4044
29. Diederich NJ, Vaillant M, Leischen M, Mancuso G, Golinval S, Nati R, et al. Sleep apnea syndrome in Parkinson's disease. A case-control study in 49 patients. Mov Disord. (2005) 20:1413–8. doi: 10.1002/mds.20624
30. Cochen De Cock V, Abouda M, Leu S, Oudiette D, Roze E, Vidailhet M, et al. Is obstructive sleep apnea a problem in Parkinson's disease? Sleep Med. (2010) 11:247–52. doi: 10.1016/j.sleep.2009.05.008
31. Arnulf I, Konofal E, Merino-Andreu M, Houeto JL, Mesnage V, Welter ML, et al. Parkinson's disease and sleepiness: an integral part of PD. Neurology. (2002) 58:1019–24. doi: 10.1212/WNL.58.7.1019
32. Baumann C, Ferini-Strambi L, Waldvogel D, Werth E, Bassetti CL. Parkinsonism with excessive daytime sleepiness—a narcolepsy-like disorder? J Neurol. (2005) 252:139–45. doi: 10.1007/s00415-005-0614-5
33. Norlinah MI, Afidah KN, Noradina AT, Shamsul AS, Hamidon BB, Sahathevan R, et al. Sleep disturbances in Malaysian patients with Parkinson's disease using polysomnography and PDSS. Parkinsonism Relat Disord. (2009) 15:670–4. doi: 10.1016/j.parkreldis.2009.02.012
34. Monaca C, Duhamel A, Jacquesson JM, Ozsancak C, Destée A, Guieu JD, et al. Vigilance troubles in Parkinson's disease: a subjective and objective polysomnographic study. Sleep Med. (2006) 7:448–53. doi: 10.1016/j.sleep.2005.12.002
35. Ancoli-Israel S, Klauber MR, Butters N, Parker L, Kripke DF. Dementia in institutionalized elderly: relation to sleep apnea. J Am Geriatr Soc. (1991) 39:258–63. doi: 10.1111/j.1532-5415.1991.tb01647.x
36. Nicolle MW, Rask S, Koopman WJ, George CF, Adams J, Wiebe S. Sleep apnea in patients with myasthenia gravis. Neurology. (2006) 67:140–2. doi: 10.1212/01.wnl.0000223515.15691.26
37. Atalaia A, De Carvalho M, Evangelista T, Pinto A. Sleep characteristics of amyotrophic lateral sclerosis in patients with preserved diaphragmatic function. Amyotroph Lateral Scler. (2007) 8:101–5. doi: 10.1080/17482960601029883
38. Poluéktov MG, Levin Ia I, Levitskii GN, Serdiuk AV, Skvortsova VI. Sleep-related breathing disturbances in motor neuron disease. Zh Nevrol Psikhiatr Im S S Korsakova. (2005) 105:4–9.
39. Lurie A. Obstructive sleep apnea in adults: epidemiology, clinical presentation, and treatment options. Adv Cardiol. (2011) 46:1–42. doi: 10.1159/000327660
40. Sullivan CE, Issa FG, Berthon-Jones M, Eves L. Reversal of obstructive sleep apnoea by continuous positive airway pressure applied through the nares. Lancet. (1981) 1:862–5. doi: 10.1016/S0140-6736(81)92140-1
41. Martinez-Garcia MA, Soler-Cataluna JJ, Ejarque-Martinez L, Soriano Y, Roman-Sanchez P, Illa FB, et al. Continuous positive airway pressure treatment reduces mortality in patients with ischemic stroke and obstructive sleep apnea: a 5-year follow-up study. Am J Respir Crit Care Med. (2009) 180:36–41. doi: 10.1164/rccm.200808-1341OC
42. Bassetti CL, Milanova M, Gugger M. Sleep-disordered breathing and acute ischemic stroke: diagnosis, risk factors, treatment, evolution, and long-term clinical outcome. Stroke. (2006) 37:967–72. doi: 10.1161/01.STR.0000208215.49243.c3
43. Parra O, Sanchez-Armengol A, Bonnin M, Arboix A, Campos-Rodriguez F, Perez-Ronchel J, et al. Early treatment of obstructive apnoea and stroke outcome: a randomised controlled trial. Eur Respir J. (2011) 37:1128–36. doi: 10.1183/09031936.00034410
44. Foldvary-Schaefer N, Andrews ND, Pornsriniyom D, Moul DE, Sun Z, Bena J. Sleep apnea and epilepsy: who's at risk? Epilepsy Behav. (2012) 25:363–7. doi: 10.1016/j.yebeh.2012.08.032
45. Malow BA, Foldvary-Schaefer N, Vaughn BV, Selwa LM, Chervin RD, Weatherwax KJ, et al. Treating obstructive sleep apnea in adults with epilepsy: a randomized pilot trial. Neurology. (2008) 71:572–7. doi: 10.1212/01.wnl.0000323927.13250.54
46. Pornsriniyom D, Kim H, Bena J, Andrews ND, Moul D, Foldvary-Schaefer N. Effect of positive airway pressure therapy on seizure control in patients with epilepsy and obstructive sleep apnea. Epilepsy Behav. (2014) 37:270–5. doi: 10.1016/j.yebeh.2014.07.005
47. Huang Z, Liu Z, Luo Q, Zhao Q, Zhao Z, Ma X, et al. Long-term effects of continuous positive airway pressure on blood pressure and prognosis in hypertensive patients with coronary heart disease and obstructive sleep apnea: a randomized controlled trial. Am J Hypertens. (2015) 28:300–6. doi: 10.1093/ajh/hpu147
48. Alajmi M, Mulgrew AT, Fox J, Davidson W, Schulzer M, Mak E, et al. Impact of continuous positive airway pressure therapy on blood pressure in patients with obstructive sleep apnea hypopnea: a meta-analysis of randomized controlled trials. Lung. (2007) 185:67–72. doi: 10.1007/s00408-006-0117-x
49. Bazzano LA, Khan Z, Reynolds K, He J. Effect of nocturnal nasal continuous positive airway pressure on blood pressure in obstructive sleep apnea. Hypertension. (2007) 50:417–23. doi: 10.1161/HYPERTENSIONAHA.106.085175
50. Garcia-Rio F, Alonso-Fernández A, Armada E, Mediano O, Lores V, Rojo B, et al. CPAP effect on recurrent episodes in patients with sleep apnea and myocardial infarction. Int J Cardiol. (2013) 168:1328–35. doi: 10.1016/j.ijcard.2012.12.015
51. Fein AS, Shvilkin A, Shah D, Haffajee CI, Das S, Kumar K, et al. Treatment of obstructive sleep apnea reduces the risk of atrial fibrillation recurrence after catheter ablation. J Am Coll Cardiol. (2013) 62:300–5. doi: 10.1016/j.jacc.2013.03.052
52. King A. Arrhythmias: CPAP with ablation reduces AF in OSA. Nat Rev Cardiol. (2013) 10:364. doi: 10.1038/nrcardio.2013.78
53. Abe H, Takahashi M, Yaegashi H, Eda S, Tsunemoto H, Kamikozawa M, et al. Efficacy of continuous positive airway pressure on arrhythmias in obstructive sleep apnea patients. Heart Vessels. (2010) 25:63–9. doi: 10.1007/s00380-009-1164-z
54. Kourouklis SP, Vagiakis E, Paraskevaidis IA, Farmakis D, Kostikas K, Parissis JT, et al. Effective sleep apnoea treatment improves cardiac function in patients with chronic heart failure. Int J Cardiol. (2013) 168:157–62. doi: 10.1016/j.ijcard.2012.09.101
55. Reis HV, Borghi-Silva A, Catai AM, Reis MS. Impact of CPAP on physical exercise tolerance and sympathetic-vagal balance in patients with chronic heart failure. Braz J Phys Ther. (2014) 18:218–27. doi: 10.1590/bjpt-rbf.2014.0037
56. Quintão M, Chermont S, Marchese L, Brandão L, Bernardez SP, Mesquita ET, et al. Acute effects of continuous positive air way pressure on pulse pressure in chronic heart failure. Arq Bras Cardiol. (2014) 102:181–6. doi: 10.5935/abc.20140006
57. Neikrug AB, Liu L, Avanzino JA, Maglione JE, Natarajan L, Bradley L, et al. Continuous positive airway pressure improves sleep and daytime sleepiness in patients with Parkinson disease and sleep apnea. Sleep. (2014) 37:177–85. doi: 10.5665/sleep.3332
58. Cooke JR, Ayalon L, Palmer BW, Loredo JS, Corey-Bloom J, Natarajan L, et al. Sustained use of CPAP slows deterioration of cognition, sleep, and mood in patients with Alzheimer's disease and obstructive sleep apnea: a preliminary study. J Clin Sleep Med JCSM. (2009) 5:305–9. doi: 10.5664/jcsm.27538
59. Ancoli-Israel S, Palmer BW, Cooke JR, Corey-Bloom J, Fiorentino L, Natarajan L, et al. Cognitive effects of treating obstructive sleep apnea in Alzheimer's disease: a randomized controlled study. J Am Geriatr Soc. (2008) 56:2076–81. doi: 10.1111/j.1532-5415.2008.01934.x
60. Chong MS, Ayalon L, Marler M, Loredo JS, Corey-Bloom J, Palmer BW, et al. Continuous positive airway pressure reduces subjective daytime sleepiness in patients with mild to moderate Alzheimer's disease with sleep disordered breathing. J Am Geriatr Soc. (2006) 54:777–81. doi: 10.1111/j.1532-5415.2006.00694.x
61. Ji KH, Bae JS, CPAP. therapy reverses weakness of myasthenia gravis: role of obstructive sleep apnea in paradoxical weakness of myasthenia gravis. J Clin Sleep Med. (2014) 10:441–2. doi: 10.5664/jcsm.3626
62. Naseer S, Kolade VO, Idrees S, Naseer S, Chandra A. Improvement in ocular myasthenia gravis during CPAP therapy for sleep apnea. Tenn Med. (2012) 105:33–4.
63. Sancho J, Servera E, Morelot-Panzini C, Salachas F, Similowski T, Gonzalez-Bermejo J. Non-invasive ventilation effectiveness and the effect of ventilatory mode on survival in ALS patients. Amyotroph Lateral Scler Frontotemporal Degener. (2014) 15:55–61. doi: 10.3109/21678421.2013.855790
64. Barthlen GM, Lange DJ. Unexpectedly severe sleep and respiratory pathology in patients with amyotrophic lateral sclerosis. Eur J Neurol. (2000) 7:299–302. doi: 10.1046/j.1468-1331.2000.00044.x
65. Kleopa KA, Sherman M, Neal B, Romano GJ, Heiman-Patterson T. Bipap improves survival and rate of pulmonary function decline in patients with ALS. J Neurol Sci. (1999) 164:82–8. doi: 10.1016/S0022-510X(99)00045-3
66. Selim BJ, Wolfe L, Coleman JM 3rd, Dewan NA. Initiation of noninvasive ventilation for sleep related hypoventilation disorders: advanced modes and devices. Chest. (2018) 153:251–65. doi: 10.1016/j.chest.2017.06.036
67. Chernyshev OY, Chi L, Toedebusch B. The Mobile Sleep Medicine Care Model for OSA Management in Acute Stroke Cardiovascular disease: From Hospital to Home. (2021). Available online at: https://d11tooehygcg9z.cloudfront.net/My.AASM/AgentsOfChange/587417.pdf; https://aasm.org/winners-of-inaugural-change-agents-competition-propose-new-approaches-for-sleep-apnea-care (accessed April 10, 2022).
68. Bradley TD, Floras JS. Obstructive sleep apnoea and its cardiovascular consequences. Lancet. (2009) 373:82–93. doi: 10.1016/S0140-6736(08)61622-0
69. Peppard PE, Young T, Palta M, Skatrud J. Prospective study of the association between sleep-disordered breathing and hypertension. N Engl J Med. (2000) 342:1378–84. doi: 10.1056/NEJM200005113421901
70. Mehra R, Benjamin EJ, Shahar E, Gottlieb DJ, Nawabit R, Kirchner HL, et al. Association of nocturnal arrhythmias with sleep-disordered breathing: the Sleep Heart Health Study. Am J Respir Crit Care Med. (2006) 173:910–6. doi: 10.1164/rccm.200509-1442OC
71. Marin JM, Carrizo SJ, Vicente E, Agusti AG. Long-term cardiovascular outcomes in men with obstructive sleep apnoea-hypopnoea with or without treatment with continuous positive airway pressure: an observational study. Lancet. (2005) 365:1046–53. doi: 10.1016/S0140-6736(05)71141-7
72. Arzt M, Young T, Finn L, Skatrud JB, Bradley TD. Association of sleep-disordered breathing and the occurrence of stroke. Am J Respir Crit Care Med. (2005) 172:1447–51. doi: 10.1164/rccm.200505-702OC
73. Yaggi HK, Concato J, Kernan WN, Lichtman JH, Brass LM, Mohsenin V. Obstructive sleep apnea as a risk factor for stroke and death. N Engl J Med. (2005) 353:2034–41. doi: 10.1056/NEJMoa043104
74. Cadilhac DA, Thorpe RD, Pearce DC, Barnes M, Rochford PD, Tarquinio N, et al. Sleep disordered breathing in chronic stroke survivors. A study of the long term follow-up of the SCOPES cohort using home based polysomnography. J Clin Neurosci. (2005) 12:632–7. doi: 10.1016/j.jocn.2004.08.014
75. Martinez-Garcia MA, Galiano-Blancart R, Roman-Sanchez P, Soler-Cataluna JJ, Cabero-Salt L, Salcedo-Maiques E. Continuous positive airway pressure treatment in sleep apnea prevents new vascular events after ischemic stroke. Chest. (2005) 128:2123–9. doi: 10.1378/chest.128.4.2123
76. Good DC, Henkle JQ, Gelber D, Welsh J, Verhulst S. Sleep-disordered breathing and poor functional outcome after stroke. Stroke. (1996) 27:252–9. doi: 10.1161/01.STR.27.2.252
77. Mansukhani MP, Bellolio MF, Kolla BP, Enduri S, Somers VK, Stead LG. Worse outcome after stroke in patients with obstructive sleep apnea: an observational cohort study. J Stroke Cerebrovasc Dis. (2011) 20:401–5. doi: 10.1016/j.jstrokecerebrovasdis.2010.02.011
78. Kaneko Y, Hajek VE, Zivanovic V, Raboud J, Bradley TD. Relationship of sleep apnea to functional capacity and length of hospitalization following stroke. Sleep. (2003) 26:293–7. doi: 10.1093/sleep/26.3.293
79. Boulos MI, Dharmakulaseelan L, Brown DL, Swartz RH. Trials in sleep apnea and stroke: learning from the past to direct future approaches. Stroke. (2021) 52:366–72. doi: 10.1161/STROKEAHA.120.031709
80. Ryan CM, Bayley M, Green R, Murray BJ, Bradley TD. Influence of continuous positive airway pressure on outcomes of rehabilitation in stroke patients with obstructive sleep apnea. Stroke. (2011) 42:1062–7. doi: 10.1161/STROKEAHA.110.597468
81. Gupta A, Shukla G, Afsar M, Poornima S, Pandey RM, Goyal V, et al. Role of positive airway pressure therapy for obstructive sleep apnea in patients with stroke: a randomized controlled trial. J Clin Sleep Med JCSM. (2018) 14:511–21. doi: 10.5664/jcsm.7034
82. Minnerup J, Ritter MA, Wersching H, Kemmling A, Okegwo A, Schmidt A, et al. Continuous positive airway pressure ventilation for acute ischemic stroke: a randomized feasibility study. Stroke. (2012) 43:1137–9. doi: 10.1161/STROKEAHA.111.637611
83. Bravata DM, Sico J, Vaz Fragoso CA, Miech EJ, Matthias MS, Lampert R, et al. Diagnosing and treating sleep apnea in patients with acute cerebrovascular disease. J Am Heart Assoc. (2018) 7:e008841. doi: 10.1161/JAHA.118.008841
84. Bravata DM, Concato J, Fried T, Ranjbar N, Sadarangani T, McClain V, et al. Auto-titrating continuous positive airway pressure for patients with acute transient ischemic attack: a randomized feasibility trial. Stroke. (2010) 41:1464–70. doi: 10.1161/STROKEAHA.109.566745
85. McEvoy RD, Antic NA, Heeley E, Luo Y, Ou Q, Zhang X, et al. CPAP for prevention of cardiovascular events in obstructive sleep apnea. N Engl J Med. (2016) 375:919–31. doi: 10.1056/NEJMoa1606599
86. Sandberg O, Franklin KA, Bucht G, Eriksson S, Gustafson Y. Nasal continuous positive airway pressure in stroke patients with sleep apnoea: a randomized treatment study. Eur Respir J. (2001) 18:630–4. doi: 10.1183/09031936.01.00070301
87. Aaronson JA, Hofman WF, van Bennekom CA, van Bezeij T, van den Aardweg JG, Groet E, et al. Effects of continuous positive airway pressure on cognitive and functional outcome of stroke patients with obstructive sleep apnea: a randomized controlled trial. J Clin Sleep Med. (2016) 12:533–41. doi: 10.5664/jcsm.5684
88. Gottlieb DJ, Punjabi NM, Mehra R, Patel SR, Quan SF, Babineau DC, et al. CPAP versus oxygen in obstructive sleep apnea. N Engl J Med. (2014) 370:2276–85. doi: 10.1056/NEJMoa1306766
89. Phillips CL, Yee BJ, Marshall NS, Liu PY, Sullivan DR, Grunstein RR. Continuous positive airway pressure reduces postprandial lipidemia in obstructive sleep apnea: a randomized, placebo-controlled crossover trial. Am J Respir Crit Care Med. (2011) 184:355–61. doi: 10.1164/rccm.201102-0316OC
90. Bravata DM, Ferguson J, Miech EJ, Agarwal R, McClain V, Austin C, et al. Diagnosis and Treatment of Sleep Apnea in patients' homes: the rationale and methods of the “GoToSleep” randomized-controlled trial. J Clin Sleep Med JCSM. (2012) 8:27–35. doi: 10.5664/jcsm.1654
91. Chan W, Coutts SB, Hanly P. Sleep apnea in patients with transient ischemic attack and minor stroke: opportunity for risk reduction of recurrent stroke? Stroke. (2010) 41:2973–5. doi: 10.1161/STROKEAHA.110.596759
92. Arzt M, Young T, Peppard PE, Finn L, Ryan CM, Bayley M, et al. Dissociation of obstructive sleep apnea from hypersomnolence and obesity in patients with stroke. Stroke. (2010) 41:e129–134. doi: 10.1161/STROKEAHA.109.566463
93. Srijithesh PR, Shukla G, Srivastav A, Goyal V, Singh S, Behari M. Validity of the Berlin Questionnaire in identifying obstructive sleep apnea syndrome when administered to the informants of stroke patients. J Clin Neurosci. (2011) 18:340–3. doi: 10.1016/j.jocn.2010.04.048
94. Rotenberg B, George C, Sullivan K, Wong E. Wait times for sleep apnea care in Ontario: a multidisciplinary assessment. Can Respir J. (2010) 17:170–4. doi: 10.1155/2010/420275
95. El Shayeb M, Topfer LA, Stafinski T, Pawluk L, Menon D. Diagnostic accuracy of level 3 portable sleep tests versus level 1 polysomnography for sleep-disordered breathing: a systematic review and meta-analysis. CMAJ. (2014) 186:E25–51. doi: 10.1503/cmaj.130952
96. Chernyshev OY, McCarty DE, Moul DE, Liendo C, Caldito GC, Munjampalli SK, et al. A pilot study: portable out-of-center sleep testing as an early sleep apnea screening tool in acute ischemic stroke. Nat Sci Sleep. (2015) 7:127–38. doi: 10.2147/NSS.S85780
97. Boulos MI, Kamra M, Colelli DR, Kirolos N, Gladstone DJ, Boyle K, et al. SLEAP SMART (sleep apnea screening using mobile ambulatory recorders after TIA/Stroke): a randomized controlled trial. Stroke. (2022) 53:710–8. doi: 10.1161/STROKEAHA.120.033753
98. Alzheimer's and Dementia in Canada - Alzheimer's Association (2018). Available online at: https://www.alz.org/ca/dementia-alzheimers-canada.asp (accessed November 14, 2018).
99. Scheltens P, Blennow K, Breteler MM, de Strooper B, Frisoni GB, Salloway S, et al. Alzheimer's disease. Lancet. (2016) 388:505–17. doi: 10.1016/S0140-6736(15)01124-1
100. Moorhouse P, Rockwood K. Vascular cognitive impairment: current concepts and clinical developments. Lancet Neurol. (2008) 7:246–55. doi: 10.1016/S1474-4422(08)70040-1
101. Smith EE. Clinical presentations and epidemiology of vascular dementia. Clin Sci (Lond). (2017) 131:1059–68. doi: 10.1042/CS20160607
102. Sonnen JA, Larson EB, Crane PK, Haneuse S, Li G, Schellenberg GD, et al. Pathological correlates of dementia in a longitudinal, population-based sample of aging. Ann Neurol. (2007) 62:406–13. doi: 10.1002/ana.21208
103. Neuropathology Group. Medical Research Council Cognitive F, Aging S. Pathological correlates of late-onset dementia in a multicentre, community-based population in England and Wales. Neuropathology Group of the Medical Research Council Cognitive Function and Ageing Study (MRC CFAS). Lancet. (2001) 357:169–175. doi: 10.1016/S0140-6736(00)03589-3
104. Gagnon K, Baril AA, Gagnon JF, Fortin M, Decary A, Lafond C, et al. Cognitive impairment in obstructive sleep apnea. Pathol Biol. (2014) 62:233–40. doi: 10.1016/j.patbio.2014.05.015
105. Landmann N, Kuhn M, Piosczyk H, Feige B, Baglioni C, Spiegelhalder K, et al. The reorganisation of memory during sleep. Sleep Med Rev. (2014) 18:531–41. doi: 10.1016/j.smrv.2014.03.005
106. Berezuk C, Ramirez J, Gao F, Scott CJ, Huroy M, Swartz RH, et al. Virchow-Robin spaces: correlations with polysomnography-derived sleep parameters. Sleep. (2015) 38:853–8. doi: 10.5665/sleep.4726
107. Xie L, Kang H, Xu Q, Chen MJ, Liao Y, Thiyagarajan M, et al. Sleep drives metabolite clearance from the adult brain. Science. (2013) 342:373–7. doi: 10.1126/science.1241224
108. Tarasoff-Conway JM, Carare RO, Osorio RS, Glodzik L, Butler T, Fieremans E, et al. Clearance systems in the brain-implications for Alzheimer disease. Nat Rev Neurol. (2015) 11:457–70. doi: 10.1038/nrneurol.2015.119
109. Kim H, Yun CH, Thomas RJ, Lee SH, Seo HS, Cho ER, et al. Obstructive sleep apnea as a risk factor for cerebral white matter change in a middle-aged and older general population. Sleep. (2013) 36:709–715B. doi: 10.5665/sleep.2632
110. Yaffe K, Laffan AM, Harrison SL, Redline S, Spira AP, Ensrud KE, et al. Sleep-disordered breathing, hypoxia, and risk of mild cognitive impairment and dementia in older women. JAMA. (2011) 306:613–9. doi: 10.1001/jama.2011.1115
111. Leng Y, McEvoy CT, Allen IE, Yaffe K. Association of sleep-disordered breathing with cognitive function and risk of cognitive impairment: a systematic review and meta-analysis. JAMA Neurol. (2017) 74:1237–45. doi: 10.1001/jamaneurol.2017.2180
112. Guarnieri B, Adorni F, Musicco M, Appollonio I, Bonanni E, Caffarra P, et al. Prevalence of sleep disturbances in mild cognitive impairment and dementing disorders: a multicenter Italian clinical cross-sectional study on 431 patients. Dement Geriatr Cogn Disord. (2012) 33:50–8. doi: 10.1159/000335363
113. Jorge C, Benitez I, Torres G, Dakterzada F, Minguez O, Huerto R, et al. The STOP-Bang and Berlin questionnaires to identify obstructive sleep apnoea in Alzheimer's disease patients. Sleep Med. (2019) 57:15–20. doi: 10.1016/j.sleep.2019.01.033
114. Bucks RS, Olaithe M, Eastwood P. Neurocognitive function in obstructive sleep apnoea: a meta-review. Respirology. (2013) 18:61–70. doi: 10.1111/j.1440-1843.2012.02255.x
115. Shieu MM, Zaheed A, Shannon C, Chervin RD, Conceicao A, Paulson HL, et al. Positive airway pressure and cognitive disorders in adults with obstructive sleep apnea: a systematic review of the literature. Neurology. (2022). doi: 10.1212/WNL.0000000000200383
116. Troussiere AC, Charley CM, Salleron J, Richard F, Delbeuck X, Derambure P, et al. Treatment of sleep apnoea syndrome decreases cognitive decline in patients with Alzheimer's disease. J Neurol Neurosurg Psychiatry. (2014) 85:1405–8. doi: 10.1136/jnnp-2013-307544
117. Swartz RH, Bayley M, Lanctot KL, Murray BJ, Cayley ML, Lien K, et al. Post-stroke depression, obstructive sleep apnea, and cognitive impairment: Rationale for, and barriers to, routine screening. Int J Stroke. (2016) 11:509–18. doi: 10.1177/1747493016641968
118. Sadeh A. The role and validity of actigraphy in sleep medicine: an update. Sleep Med Rev. (2011) 15:259–67. doi: 10.1016/j.smrv.2010.10.001
119. Colelli DR, Black SE, Masellis M, Lam B, Lim ASP, Boulos MI. Feasibility of unattended home sleep apnea testing in a cognitively impaired clinic population. J Clin Sleep Med. (2021) 17:435–44. doi: 10.5664/jcsm.8918
120. Ambrosino N, Carpene N, Gherardi M. Chronic respiratory care for neuromuscular diseases in adults. Eur Resp J. (2009) 34:444–51. doi: 10.1183/09031936.00182208
121. Tabachnik E, Muller NL, Bryan AC, Levison H. Changes in ventilation and chest wall mechanics during sleep in normal adolescents. J Appl Physiol Respir Environ Exerc Physiol. (1981) 51:557–64. doi: 10.1152/jappl.1981.51.3.557
122. Berthon-Jones M, Sullivan CE. Ventilation and arousal responses to hypercapnia in normal sleeping humans. J Appl Physiol Respir Environ Exerc Physiol. (1984) 57:59–67. doi: 10.1152/jappl.1984.57.1.59
123. Hudgel DW, Martin RJ, Johnson B, Hill P. Mechanics of the respiratory system and breathing pattern during sleep in normal humans. J Appl Physiol Respir Environ Exerc Physiol. (1984) 56:133–7. doi: 10.1152/jappl.1984.56.1.133
124. Labanowski M, Schmidt-Nowara W, Guilleminault C. Sleep and neuromuscular disease: frequency of sleep-disordered breathing in a neuromuscular disease clinic population. Neurology. (1996) 47:1173–80. doi: 10.1212/WNL.47.5.1173
125. Johnson DC, Kazemi H. Central control of ventilation in neuromuscular disease. Clin Chest Med. (1994) 15:607–17. doi: 10.1016/S0272-5231(21)00956-4
126. White JE, Drinnan MJ, Smithson AJ, Griffiths CJ, Gibson GJ. Respiratory muscle activity and oxygenation during sleep in patients with muscle weakness. Eur Respir J. (1995) 8:807–14.
127. Bauman KA, Kurili A, Schmidt SL, Rodriguez GM, Chiodo AE, Sitrin RG. Home-based overnight transcutaneous capnography/pulse oximetry for diagnosing nocturnal hypoventilation associated with neuromuscular disorders. Arch Phys Med Rehabil. (2013) 94:46–52. doi: 10.1016/j.apmr.2012.08.215
128. Bye PT, Ellis ER, Issa FG, Donnelly PM, Sullivan CE. Respiratory failure and sleep in neuromuscular disease. Thorax. (1990) 45:241–7. doi: 10.1136/thx.45.4.241
129. Berlowitz DJ, Howard ME, Fiore JF Jr, Vander Hoorn S, O'Donoghue FJ, Westlake J, et al. Identifying who will benefit from non-invasive ventilation in amyotrophic lateral sclerosis/motor neurone disease in a clinical cohort. J Neurol Neurosurg Psychiatry. (2016) 87:280–6. doi: 10.1136/jnnp-2014-310055
130. Bourke SC, Tomlinson M, Williams TL, Bullock RE, Shaw PJ, Gibson GJ. Effects of non-invasive ventilation on survival and quality of life in patients with amyotrophic lateral sclerosis: a randomised controlled trial. Lancet Neurol. (2006) 5:140–7. doi: 10.1016/S1474-4422(05)70326-4
131. Jacobs TL, Brown DL, Baek J, Migda EM, Funckes T, Gruis KL. Trial of early noninvasive ventilation for ALS: a pilot placebo-controlled study. Neurology. (2016) 87:1878–83. doi: 10.1212/WNL.0000000000003158
132. Sancho J, Martinez D, Bures E, Diaz JL, Ponz A, Servera E. Bulbar impairment score and survival of stable amyotrophic lateral sclerosis patients after noninvasive ventilation initiation. ERJ Open Res. (2018) 4:00159–2017. doi: 10.1183/23120541.00159-2017
133. Vitacca M, Montini A, Lunetta C, Banfi P, Bertella E, De Mattia E, et al. Impact of an early respiratory care programme with non-invasive ventilation adaptation in patients with amyotrophic lateral sclerosis. Eur J Neurol. (2018) 25:556–e533. doi: 10.1111/ene.13547
134. Ackrivo J, Hsu JY, Hansen-Flaschen J, Elman L, Kawut SM. Noninvasive ventilation use is associated with better survival in amyotrophic lateral sclerosis. Ann Am Thorac Soc. (2021) 18:486–94. doi: 10.1513/AnnalsATS.202002-169OC
135. Simonds AK. Home ventilation. Eur Respir J Suppl. (2003) 47:38s−46s. doi: 10.1183/09031936.03.00029803
136. Kaslow JA, Soslow JH, Burnette WB, Raucci FJ, Hills TJ, Ibach MG, et al. Improving access and guideline adherence in pulmonary care in patients with Duchenne muscular dystrophy. Respir Care. (2022) 67:347–52. doi: 10.4187/respcare.09502
137. Fauroux B, Griffon L, Amaddeo A, Stremler N, Mazenq J, Khirani S, et al. Respiratory management of children with spinal muscular atrophy (SMA). Arch Pediatr. (2020) 27:7S29–27S34. doi: 10.1016/S0929-693X(20)30274-8
138. Andersen PM, Abrahams S, Borasio GD, de Carvalho M, Chio A, Van Damme P, et al. EFNS guidelines on the clinical management of amyotrophic lateral sclerosis (MALS)—revised report of an EFNS task force. Eur J Neurol. (2012) 19:360–75. doi: 10.1111/j.1468-1331.2011.03501.x
139. Ward S, Chatwin M, Heather S, Simonds AK. Randomised controlled trial of non-invasive ventilation (NIV) for nocturnal hypoventilation in neuromuscular and chest wall disease patients with daytime normocapnia. Thorax. (2005) 60:1019–24. doi: 10.1136/thx.2004.037424
140. Kapur VK, Auckley DH, Chowdhuri S, Kuhlmann DC, Mehra R, Ramar K, et al. Clinical practice guideline for diagnostic testing for adult obstructive sleep apnea: an American Academy of sleep medicine clinical practice guideline. J Clin Sleep Med. (2017) 13:479–504. doi: 10.5664/jcsm.6506
141. Goldberg A. Clinical indications for noninvasive positive pressure ventilation in chronic respiratory failure due to restrictive lung disease, COPD, and nocturnal hypoventilation—a consensus conference report. Chest. (1999) 116:521–34. doi: 10.1378/chest.116.2.521
142. Hannan LM, Rautela L, Berlowitz DJ, McDonald CF, Cori JM, Sheers N, et al. Randomised controlled trial of polysomnographic titration of noninvasive ventilation. Eur Resp J. (2019) 53:1802118. doi: 10.1183/13993003.02118-2018
143. Fanfulla F, Delmastro M, Berardinelli A, Lupo ND, Nava S. Effects of different ventilator settings on sleep and inspiratory effort in patients with neuromuscular disease. Am J Respir Crit Care Med. (2005) 172:619–24. doi: 10.1164/rccm.200406-694OC
144. Berry RB, Chediak A, Brown LK, Finder J, Gozal D, Iber C, et al. Best clinical practices for the sleep center adjustment of noninvasive positive pressure ventilation (NPPV) in stable chronic alveolar hypoventilation syndromes. J Clin Sleep Med JCSM. (2010) 6:491–509. doi: 10.5664/jcsm.27941
145. Lopes de. Almeida JP, Pinto A, Pinto S, Ohana B, de Carvalho M. Economic cost of home-telemonitoring care for BiPAP-assisted ALS individuals. Amyotroph Lateral Scler. (2012) 13:533–7. doi: 10.3109/17482968.2012.703675
146. Pinto A, Almeida JP, Pinto S, Pereira J, Oliveira AG, de Carvalho M. Home telemonitoring of non-invasive ventilation decreases healthcare utilisation in a prospective controlled trial of patients with amyotrophic lateral sclerosis. J Neurol Neurosurg Psychiatry. (2010) 81:1238–42. doi: 10.1136/jnnp.2010.206680
Keywords: stroke, neuromuscular conditions, ambulatory sleep testing, home sleep apnea test (HSAT), screening, mobile sleep medicine, sleep-disordered breathing, cognitive impairment
Citation: Boulos MI, Chi L and Chernyshev OY (2022) The mobile sleep medicine model in neurologic practice: Rationale and application. Front. Neurol. 13:1032463. doi: 10.3389/fneur.2022.1032463
Received: 30 August 2022; Accepted: 22 September 2022;
Published: 28 October 2022.
Edited by:
Nina Christmann, Central Institute of Mental Health, GermanyReviewed by:
George Tze Ping Tay, Queensland Health, AustraliaYaroslav Winter, University Mainz, Germany
Copyright © 2022 Boulos, Chi and Chernyshev. This is an open-access article distributed under the terms of the Creative Commons Attribution License (CC BY). The use, distribution or reproduction in other forums is permitted, provided the original author(s) and the copyright owner(s) are credited and that the original publication in this journal is cited, in accordance with accepted academic practice. No use, distribution or reproduction is permitted which does not comply with these terms.
*Correspondence: Oleg Y. Chernyshev, oleg.chernyshev@lsuhs.edu
†These authors have contributed equally to this work