- 1Department of Emergency Medicine, Chungbuk National University Hospital, Cheongju, Republic of Korea
- 2Laboratory of Emergency Medical Services, Seoul National University Hospital Biomedical Research Institute, Seoul, Republic of Korea
- 3Department of Emergency Medicine, Seoul National University Hospital, Seoul, Republic of Korea
- 4Department of Emergency Medicine, Seoul National University College of Medicine, Seoul, Republic of Korea
- 5Department of Emergency Medicine, Seoul National University Boramae Medical Center, Seoul, Republic of Korea
- 6Department of Emergency Medicine, Chonnam National University Hospital, Gwangju, Republic of Korea
- 7Department of Emergency Medicine, School of Medicine Kyungpook National University and Kyungpook National University Hospital, Daegu, Republic of Korea
Background: Traumatic brain injury (TBI) is a major public health problem with high mortality and disability. Vitamin E, one of the antioxidants for treatment of TBI, has not been sufficiently evaluated for predicting prognosis of TBI. This study aimed to evaluate the prognostic value of vitamin E on functional outcomes of TBI patients with intracranial injury.
Methods: A multi-center prospective cohort study was conducted in five university hospitals between 2018 and 2020. Adult TBI patients who visited the emergency department (ED) with intracranial hemorrhage or diffuse axonal injury confirmed by radiological examination were eligible. Serum vitamin E levels (mg/dL) were categorized into 4 groups: low (0.0–5.4), low-normal (5.5–10.9), high-normal (11.0–16.9), and high (17.0–). Study outcomes were set as 1- and 6-month disability (Glasgow outcome scale (GOS) 1–4). Multilevel logistic regression analysis was conducted to calculate the adjusted odds ratios (AORs) of vitamin E for related outcomes.
Results: Among 550 eligible TBI patients with intracranial injury, the median (IQR) of serum vitamin E was 10.0 (8.0–12.3) mg/dL; 204/550 (37.1%) had 1-month disability and 197/544 (36.1%) had 6-month disability of GOS 1–4. Compared with the high-normal group, the odds of 1-month disability and 6-month disability increased in the low and low-normal group (AORs (95% CIs): 3.66 (1.62–8.27) and 2.60 (1.15–5.85) for the low group and 1.63 (1.08–2.48) and 1.60 (1.04–2.43) for the low-normal group, respectively).
Conclusion: Low serum vitamin E level was associated with poor prognosis at 1 and 6 months after TBI with intracranial injury.
Introduction
Traumatic brain injury (TBI) is a serious public health burden worldwide, with more than 10 million people worldwide hospitalized or dead annually from TBI (1). TBI occurs frequently in younger age, which results in high mortality or leaving a permanent impairment (2). In addition, the disease burden of TBI is expected to increase further due to aging of the population as well as improvement in immediate life-saving treatment (3, 4).
Vitamin E is a lipid-soluble antioxidant that reduces reactive oxygen species. Vitamin E deficiency causes degeneration of neurons, particularly peripheral axons and posterior column neurons, which results in peripheral neuropathy, ataxia, and skeletal myopathy (5, 6). In the pathophysiology of TBI, increased production of free radicals and reactive oxygen species after injury leads to oxidative stress and secondary neurotoxicity (7). In cases where there is a deficiency of various antioxidants, involved in recovery of brain tissue post injury, treatment with antioxidants may be theoretically effective in preventing propagation of tissue damage as well as in improving short- and long-term survival/functional outcomes (8–12). Several animal studies have evaluated that vitamin E supplementation before TBI reduced oxidative stress and improved learning and memory (13, 14). Vitamin E administration after TBI also reduced microscopic brain damage, promoted nerve regeneration, and improved cognitive function in animal models (15–17). In clinical settings, early antioxidant supplementation including vitamin E in critically ill patients, including TBI patients, was associated with decrease in organ failure and hospital stay (18, 19). Vitamin E supplementation showed a significant reduction in mortality and improvement of long-term functional outcomes of TBI patients (20).
Given the evidence of the beneficial effects of vitamin E, it was hypothesized that vitamin E deficiency would be associated with poor survival outcomes and functional recovery after TBI with intracranial injury, and that serum vitamin E levels could be utilized as a nutritional biomarker for clinical outcomes after TBI. This study aimed to determine the association between serum vitamin E levels and functional/survival outcomes among TBI patients with intracranial injury.
Methods
Study design, setting, data source
This was a multi-center prospective cohort study conducted in five participating university hospitals in Korea based on the Pan-Asian Trauma Outcome Study (PATOS) registry (21). The PATOS-TBI study is a collaborative research network that started in 2018 throughout Korea for in-depth research on TBI (ClinicalTrials.gov, ID: NCT04718935). The objectives of PATOS-TBI study are to identify nutritional and metabolic biomarkers related to prognosis of TBI with intracranial injury, and to develop a prognostic predictive model of long-term prognosis that applies them to select high-risk populations funded by the National Research Foundation of Korea (22).
The inclusion criteria were TBI patients with intracranial injury confirmed by a brain radiological examination. Intracranial injury was defined as intracranial hemorrhage or diffuse axial injury (ICD-10 S06.1–06.9). Patients with TBI over 18 years of age who visited participating hospitals' ED using emergency medical services (EMS) within 72 h of injury were enrolled. Patients with penetrating brain injury, history of psychiatric or neurological disorders, terminal cancer, pregnant women, and those transferred after surgery from other hospitals were excluded from the study.
The PATOS-TBI study registry comprises of several variables including the patient's demographics, injury-related information, emergency medical service (EMS) records, clinical findings, laboratory test results, brain imaging findings, diagnoses and medical treatment in hospitals, and patient outcomes at time of hospital discharge and follow-up.
Upon confirmation of intracranial injury on brain computer tomography (CT) and magnetic resonance imaging (MRI), an emergency physician obtained informed consent for enrollment and registered the patients to the PATOS-TBI study during the ED treatment process and blood samples were obtained for biomarker analysis. Based on the informed consent from the patient or guardians, primary surveillance data were collected by an emergency physician. A trained research coordinator from each participating hospital collected and entered the registry through interviews and electronic medical records review. Serum biomarker levels were not reported to physicians, and patient management was not altered by the study. Follow-up data at 1- and 6-months after the injury were captured via telephone surveys. All research coordinators were required to receive education and training prior to participation in the study and periodically during the study period. Data were collected using a standardized data collection protocol, a case report form, and a web-based data collection system. The Quality Management Committee (QMC) reviewed the data monthly and provided regular feedback for quality assurance (22, 23).
Study population
The study population included all adult TBI patients who visited the participating EDs between 2018 and 2020 with intracranial injury (intracranial hemorrhage or diffuse axonal injury, ICD-10 S06.1–06.9) confirmed by brain CT or MRI. Patients with unknown information regarding vitamin E levels and 1-month GOS score were excluded.
Main outcomes
The primary outcome was 1-month disability after injury, which was defined as Glasgow Outcome Scale (GOS) score of 1 to 4. GOS was scored from 0 to 5 as follows: 1 (dead), 2 (vegetative state), 3 (severe disability), 4 (moderate disability), and 5 (good recovery) (24). The secondary outcome was 1-month mortality and the tertiary outcomes were 6-month disability and 6-month mortality.
Analysis of serum biomarkers
Upon confirmation of intracranial injury on brain CT or MRI and patients' consent for study enrollment, 24 mL of blood was drawn via venipuncture in the ED. Centrifugation was performed at 3,000 rpm for 10 min at room temperature within an h of blood sampling. Levels of serum α-tocopherol, the most biologically active form of vitamin E, were analyzed. Vitamin E is a collective term that refers to eight fat-soluble forms with antioxidant activities. The liver preferentially re-secretes only α-tocopherol via the α-tocopherol transport protein and degrades all other forms of vitamin E. Therefore, α-tocopherol is the most recognized form of vitamin E in human body, and most research on vitamin E is performed using α-tocopherol level (25).
Serum samples were kept frozen at −20°C and were analyzed within 7 days for serum biomarkers. After dispensing 500 μL of calibrator and dispensing the sample into a 15 mL conical tube, six calibration standard solutions were prepared for each standard concentration. The solutions were adjusted to the total volume of 500 μL, with addition of 1 mL of ethanol for deproteinization. Next, 3 mL of N-Hexane was added, then centrifuging at 3,000 rpm for 5 min. After transferring 2 mL of the supernatant to the test tube and evaporating with nitrogen gas, 150 μL of methanol was added to the residue and transferred to a vial. These pretreated samples were analyzed after standard testing with High-performance liquid chromatography (HPLC).
Variables and measurements
Considering the normal range of vitamin E level in adults, the plasma vitamin E level was categorized into four groups: low (0.0–5.4 mg/dL), low-normal (5.5–10.9 mg/dL), high-normal (11.0–16.9 mg/dL), and high group (17.0– mg/dL) (26).
Information were collected including the patients' demographics (age, sex, education, comorbidities, body mass index, and pre-injury disability), injury characteristics (mechanism of injury (road traffic injury, fall, blunt trauma, and others), date and time of injury, and alcohol intake at injury, ED (Glasgow coma scale score at arrival to the ED, transfer from other hospitals, time interval from injury to ED, type of intracranial injury on brain CT or MRI, injury severity, and length of stay in ED), and outcomes (ED disposition and hospital outcome, 1-month and 6-month follow up outcomes).
Statistical analysis
Descriptive analysis was conducted to compare the characteristics of study population according to serum vitamin E levels. Categorical variables were reported as counts and proportions and continuous variables were reported as medians and interquartile ranges. Differences between groups were compared using Pearson's chi-square test and Wilcoxon rank sum test.
Adjusted odds ratios (AORs) with 95% confidence intervals (CIs) were calculated using multilevel multivariable logistic regression analysis to examine associations between serum vitamin E levels and study outcomes in TBI patients after adjusting for hospital clustering. Potential confounders were selected based on directed acyclic graph (DAG) models and included age, sex, obesity (body mass index ≥25), high education (college and more), comorbidities (hypertension and diabetes mellitus), pre-injury disability (mRS score 3–5), and injury severity (AIS score of TBI ≥3). Variables were adjusted in the model as potential confounders if they block all back-door paths from the main exposure to the outcome in DAG models (Supplementary Figure 1); affect the outcome; and are not mediators in a causal pathway (27, 28). Akaike information criterion (AIC) and Bayesian information criterion (BIC) values were calculated to evaluate a goodness-of-fit of the model.
Receiver operating characteristic (ROC) analysis was performed to evaluate the performance of serum vitamin E levels and Youden's Index was used to find the best cut-off point of serum vitamin E levels in predicting study outcomes.
A two-sided P < 0.05 was defined as significant. All statistical analyses were performed using SAS software, version 9.4 (SAS Institute Inc., Cary, NC, USA).
Ethics statement
The study was approved by the Institutional Review Board of all participating hospitals (IRB no.: SNUH-1806-078-951; CNUH-2018-297; KNUH-2018-10-014-007; CBNUH-2018-09-018; BMC-30-2018-85). Informed consent was obtained from the patients or family members/legal representatives of unconscious patients. Patient information was anonymized prior to analysis.
Results
A total 550 adult TBI patients out of 606 patients were included in this study, excluding 56 patients for whom information on 1-month GOS was unknown.
Table 1 presents the demographics of TBI patients according to the vitamin E levels. The median (IQR) of serum vitamin E was 10.0 (8.0–12.3) mg/dL and vitamin E deficiency was observed in 35/550 (6.4%) of the study population. The proportion of 1-month disability after injury was 37.1% (204/550) in study population, and 1-month mortality was 18.7% (103/550). Among 544 patients who were followed up to 6-months after injury, 36.1% (197/544) had disability of GOS 1–4 and 20.5% (112/544) had mortality. The proportions of 1-month disability after injury were 65.7% (23/35) for the low group, 40.5% (123/304) for the low-normal group, 27.3% (50/183) for the high-normal group, and 28.6% (8/28) for the high group, respectively (p < 0.001).
Table 2 shows the characteristics of TBI patients by 1-month disability. The medians (IQR) of serum vitamin E levels were 9.2 (7.3–11.4) mg/dL for 204 patients who had GOS 1–4 at 1-month after injury and 10.5 (8.6–12.5) mg/dL for 346 patients with GOS 5 (p < 0.001).
Table 3 presents the associations between serum vitamin E levels and study outcomes. In the multilevel logistic regression analysis, patients with low vitamin E had higher odds of disability and mortality. The adjusted odds for 1-month disability increased in the low-normal and the low groups when compared with the high-normal group (adjusted ORs (95% CIs): 3.66 (1.62–8.27) for the low group and 1.63 (1.08–2.48) for the low-normal group). Regarding 1-month mortality, 6-month disability, and 6-month mortality, the trends were similar (adjusted ORs (95% CIs): 4.77 (1.96–11.62) and 2.31 (1.28–4.15) for 1-month mortality, 2.60 (1.15–5.85) and 1.60 (1.04–2.43) for 6-month disability, and 4.91 (1.98–12.17) and 2.36 (1.34–4.15) for 6-month mortality, respectively).
In the ROC analysis, the area under the curve (AUC) of serum vitamin E was 0.63 (95% CIs, 0.58–0.68) (Figure 1). The optimal cut-off value of vitamin E was 8.4 mg/dL, corresponding to a sensitivity of 44.0% and a specificity of 76.9%. Serum vitamin E levels < 8.4 mg/dL were significantly associated with poorer outcomes (adjusted ORs (95% CIs): 2.69 (1.82–3.97) for 1-month disability, 2.49 (1.53–4.07) for 1-month mortality, 2.37 (1.57–3.57) for 6-month disability, and 2.54 (1.57–4.10) for 6-month mortality, respectively) (Table 4).
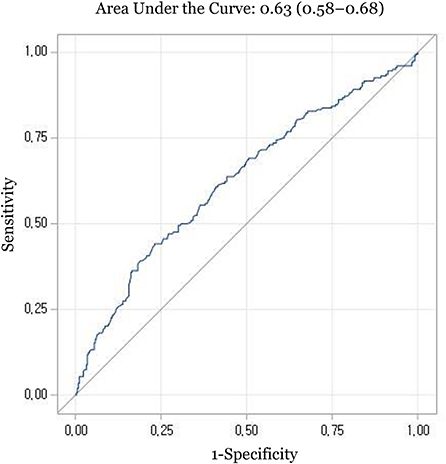
Figure 1. Receiver operating characteristic curve for prediction of 1-month disability with serum vitamin E levels.
Discussion
This multi-center prospective cohort study evaluated the prognostic value of serum vitamin E levels on functional/survival outcomes for TBI patients with intracranial injury. There was an association between serum vitamin E levels and 1-month disability (adjusted ORs (95% CIs): 3.66 (1.62–8.27) for the low group and 1.63 (1.08–2.48) for the low-normal group, when compared with the high-normal group). Regarding survival outcomes, there was an association between serum vitamin E levels and 1-month mortality (adjusted ORs (95% CIs): 4.77 (1.96–11.62) for the low group and 2.31 (1.28–4.15) for the low-normal group). These results suggest serum vitamin E as a potential biomarker related to functional and survival outcomes of TBI with intracranial injury.
Reactive oxygen species-induced oxidative damage has been identified as a major contributor to secondary injury from TBI, which has resulted in further damage to neuronal tissue and vasculature. Therefore, various antioxidants such as vitamin B, C, D, zinc, and magnesium have been used before and after TBI to prevent further brain damage (8, 10). Among them, vitamin E has been highlighted because it can easily be implemented in emergency care. It is an inexpensive, safe, and ubiquitous dietary supplement. It is also well tolerated and easily administered via enteral feeding (12, 29). Some animal studies have shown the efficacy of vitamin E in exerting a neuroprotective effect by decreasing reactive oxygen species. Vitamin E supplements before and after TBI reduced oxidative stress and showed significant beneficial effects on cognition and mortality (13, 16, 17). Vitamin E is also known as one of the factors inhibiting genetically programmed cell death, and has been used in studies to treat degenerative brain diseases (30). Vitamin E depletion is one of the major factors that increase vulnerability to TBI (8, 9).
The preclinical data supporting vitamin E in TBI are strong. However, only a select few studies have been conducted in vivo. Some studies reported that plasma vitamin E levels were rapidly decreased following TBI, which showed early vitamin E depletion and the subsequent need for further supplementation (31, 32). The results of this study also showed similar results, that lower serum levels of vitamin E were associated with poorer outcomes after TBI with intracranial injury. The low and low-normal vitamin E level groups showed significantly poorer survival and functional outcomes. The relative decrease in antioxidant capacity may correlate with the severity of brain injury and suggests a dose-response relationship between vitamin E deficiency and poor neurological outcomes.
Two randomized trials for vitamin E administration in critically ill patients showed only short-term benefits such as reduction of rates of organ failure, inflammatory responses, and a shorter length of stay in the intensive care unit (18, 19). Since the above studies were conducted for critically ill patients, including TBI patients, the interpretation of the results may be limited. The only randomized clinical trial conducted in TBI patients reported that vitamin E administration has showed a beneficial effect in significantly reducing mortality rates and improving functional outcomes at discharge and follow-up (20).
This study is worthwhile in that it prospectively constructed a cohort of TBI patients and identified a relationship between serum vitamin E levels and short-/long-term clinical outcomes after TBI. Currently used diagnostic imaging techniques for TBI are well known to have high initial diagnostic accuracy for intracranial injury, but the clinical role of these on predicting long-term cognitive and physical impairment is limited (33, 34). Based on the results of this study, it can be expected that high-risk groups with poor prognosis will be screened, and the disability and burden of TBI will be reduced through early vitamin E correction and treatment.
This study has several limitations. First, blood samples in this study were collected only at the ED. Serial measurements of vitamin E during hospitalization and understanding the kinetics of vitamin E over time after TBI would be needed to better interpret the findings of this study. Second, potential confounders were selected based on the DAG model. Using the rules of DAG models, the variables in Model 3 were chosen as a minimally sufficient conditioning set to estimate the unbiased effect of serum vitamin E levels. However, there is a possibility that there is some back-door path which can bias the vitamin E levels effects on study outcomes. There may also be unmeasured potential effect modifiers which may violate the assumption of independent effect of vitamin E levels on the study outcome, such as brain volume and anatomical location affected by intracranial injury, and patients' other nutritional status. Third, there were no available data on the history of vitamin E levels before injury, diet diary, and nutritional status, which would have been related to serum vitamin E levels at the ED. Moreover, there were no information about whether vitamin E was supplemented before/after TBI. Fourth, selection bias may arise because the TBI patients with unknown 1-month disability were excluded. Finally, there would be unmeasured and uncontrolled bias when considering the complex pathophysiological processes after TBI. Caution should be taken when interpreting the study results of this study given the significant limitations.
In conclusion, serum vitamin E level is associated with long- and short-term disability and mortality for TBI patients with intracranial hemorrhage and diffuse axonal injury. This study suggests that serum vitamin E may be a potential biomarker in predicting the long- and short-term prognoses of TBI patients with intracranial injury. Further research is needed to evaluate the benefit of vitamin E supplementation in improving the long-term outcomes of TBI patients.
Data availability statement
The raw data supporting the conclusions of this article will be made available by the authors, without undue reservation.
Ethics statement
The studies involving human participants were reviewed and approved by of all participating hospitals (Seoul National Univesity Hospital, Seoul National University Boramae Medical Center, Chungbuk National University Hospital, Kyungpook National University Hospital, and Chonnam National University Hospital). Written informed consent to participate in this study was provided by the participants' legal guardian/next of kin.
Author contributions
Conceptualization, had full access to all of the data in the study, and take responsibility for the integrity of the data as well as the accuracy of the data analysis: GP and YR. Data curation: GP, HY, SL, EJ, and SM. Formal analysis and software, funding acquisition, and writing–original draft: GP. Investigation: YR, HY, SL, EJ, and SM. Methodology: GP, YR, SK, and SS. Supervision: YR, SK, and SS. Validation: HY, SL, EJ, and SM. Visualization: GP and SK. Writing—review and editing: YR and SS. Approval of final manuscript: all authors.
Funding
This work was supported by the National Research Foundation of Korea (NRF) grant funded by the Korean government (MSIT) (Grant no.: NRF-2018R1C1B6007625 and NRF-2021R1A2C4002898). This funding source had no role in the study design, execution, analyses, interpretation of the data, or decision to submit results.
Conflict of interest
The authors declare that the research was conducted in the absence of any commercial or financial relationships that could be construed as a potential conflict of interest.
Publisher's note
All claims expressed in this article are solely those of the authors and do not necessarily represent those of their affiliated organizations, or those of the publisher, the editors and the reviewers. Any product that may be evaluated in this article, or claim that may be made by its manufacturer, is not guaranteed or endorsed by the publisher.
Supplementary material
The Supplementary Material for this article can be found online at: https://www.frontiersin.org/articles/10.3389/fneur.2022.1008717/full#supplementary-material
References
1. Hyder AA, Wunderlich CA, Puvanachandra P, Gururaj G, Kobusingye OC. The impact of traumatic brain injuries: a global perspective. NeuroRehabilitation. (2007) 22:341–53. doi: 10.3233/NRE-2007-22502
2. Stocchetti N, Zanier ER. Chronic impact of traumatic brain injury on outcome and quality of life: a narrative review. Crit Care. (2016) 20:148. doi: 10.1186/s13054-016-1318-1
3. Maas AIR, Menon DK, Adelson PD, Andelic N, Bell MJ, Belli A, et al. Traumatic brain injury: integrated approaches to improve prevention, clinical care, and research. Lancet Neurol. (2017) 16:987–1048. doi: 10.1016/S1474-4422(17)30371-X
4. Gardner RC, Dams-O'Connor K, Morrissey MR, Manley GT. Geriatric traumatic brain injury: epidemiology, outcomes, knowledge gaps, and future directions. J Neurotrauma. (2018) 35:889–906. doi: 10.1089/neu.2017.5371
5. Herrera E, Barbas C. Vitamin E: action, metabolism and perspectives. J Physiol Biochem. (2001) 57:43–56. doi: 10.1007/BF03179812
6. Medicine Io. Dietary Reference Intakes for Vitamin C, Vitamin E, Selenium, and Carotenoids. Washington, DC: The National Academies Press. (2000) p 528.
7. Gilgun-Sherki Y, Rosenbaum Z, Melamed E, Offen D. Antioxidant therapy in acute central nervous system injury: current state. Pharmacol Rev. (2002) 54:271–84. doi: 10.1124/pr.54.2.271
8. Vonder Haar C, Peterson TC, Martens KM, Hoane MR. Vitamins and nutrients as primary treatments in experimental brain injury: Clinical implications for nutraceutical therapies. Brain Res. (2016) 1640:114–29. doi: 10.1016/j.brainres.2015.12.030
9. Hall ED, Vaishnav RA, Mustafa AG. Antioxidant therapies for traumatic brain injury. Neurotherapeutics. (2010) 7:51–61. doi: 10.1016/j.nurt.2009.10.021
10. Shen Q, Hiebert JB, Hartwell J, Thimmesch AR, Pierce JD. Systematic review of traumatic brain injury and the impact of antioxidant therapy on clinical outcomes. Worldviews Evid Based Nurs. (2016) 13:380–9. doi: 10.1111/wvn.12167
11. Horn SD, Kinikini M, Moore LW, Hammond FM, Brandstater ME, Smout RJ, et al. Enteral nutrition for patients with traumatic brain injury in the rehabilitation setting: associations with patient preinjury and injury characteristics and outcomes. Arch Phys Med Rehabil. (2015) 96:S245–55. doi: 10.1016/j.apmr.2014.06.024
12. Institute of Medicine Committee on Nutrition T, the B. In: Erdman J, Oria M, Pillsbury L, editors. Nutrition and Traumatic Brain Injury: Improving Acute and Subacute Health Outcomes in Military Personnel. Washington (DC): National Academies Press (US). (2011).
13. Aiguo W, Zhe Y, Gomez-Pinilla F. Vitamin E protects against oxidative damage and learning disability after mild traumatic brain injury in rats. Neurorehabil Neural Repair. (2010) 24:290–8. doi: 10.1177/1545968309348318
14. Inci S, Ozcan OE, Kilinç K. Time-level relationship for lipid peroxidation and the protective effect of alpha-tocopherol in experimental mild and severe brain injury. Neurosurgery. (1998) 43:330–5. doi: 10.1097/00006123-199808000-00095
15. Conte V, Uryu K, Fujimoto S, Yao Y, Rokach J, Longhi L, et al. Vitamin E reduces amyloidosis and improves cognitive function in Tg2576 mice following repetitive concussive brain injury. J Neurochem. (2004) 90:758–64. doi: 10.1111/j.1471-4159.2004.02560.x
16. Yang J, Han Y, Ye W, Liu F, Zhuang K, Wu G. Alpha tocopherol treatment reduces the expression of Nogo-A and NgR in rat brain after traumatic brain injury. J Surg Res. (2013) 182:e69–77. doi: 10.1016/j.jss.2012.11.010
17. Ishaq GM, Saidu Y, Bilbis LS, Muhammad SA, Jinjir N, Shehu BB. Effects of α-tocopherol and ascorbic acid in the severity and management of traumatic brain injury in albino rats. J Neurosci Rural Pract. (2013) 4:292–7. doi: 10.4103/0976-3147.118784
18. Nathens AB, Neff MJ, Jurkovich GJ, Klotz P, Farver K, Ruzinski JT, et al. Randomized, prospective trial of antioxidant supplementation in critically ill surgical patients. Ann Surg. (2002) 236:814–22. doi: 10.1097/00000658-200212000-00014
19. Berger MM, Soguel L, Shenkin A, Revelly JP, Pinget C, Baines M, et al. Influence of early antioxidant supplements on clinical evolution and organ function in critically ill cardiac surgery, major trauma, and subarachnoid hemorrhage patients. Crit Care. (2008) 12:R101. doi: 10.1186/cc6981
20. Razmkon A, Sadidi A, Sherafat-Kazemzadeh E, Mehrafshan A, Jamali M, Malekpour B, et al. Administration of vitamin C and vitamin E in severe head injury: a randomized double-blind controlled trial. Clin Neurosurg. (2011) 58:133–7. doi: 10.1227/NEU.0b013e3182279a8f
21. Kong SY, Shin SD, Tanaka H, Kimura A, Song KJ, Shaun GE, et al. Pan-Asian Trauma Outcomes Study (PATOS): rationale and methodology of an international and multicenter trauma registry. Prehosp Emerg Care. (2018) 22:58–83. doi: 10.1080/10903127.2017.1347224
22. Jung E, Ro YS, Park JH, Moon SB, Lee SGW, Park GJ, et al. Vitamin D deficiency and prognosis after traumatic brain injury with intracranial injury: a multicenter observational study. J Neurotrauma. (2022). doi: 10.1089/neu.2022.0053
23. Lee SGW, Ro YS, Jung E, Moon SB, Park GJ, Yoon H, et al. Serum acylcarnitine and long-term functional prognosis after traumatic brain injury with intracranial injury: a multicenter prospective study. J Neurotrauma. (2022). doi: 10.1089/neu.2022.0096
24. Maas AI, Stocchetti N, Bullock R. Moderate and severe traumatic brain injury in adults. Lancet Neurol. (2008) 7:728–41. doi: 10.1016/S1474-4422(08)70164-9
25. Blaner WS. Vitamin E: the enigmatic one! J Lipid Res. (2013) 54:2293–4. doi: 10.1194/jlr.E042267
26. Traber MG. Vitamin E: Metabolism and Requirements. In: Caballero B, editor. Encyclopedia of Human Nutrition (Third Edition). Waltham: Academic Press. (2013) p. 383–9. doi: 10.1016/B978-0-12-375083-9.00278-6
27. Rothman KJ, Greenland S, Lash TL. Modern Epidemiology. 3rd ed. Philadelphia, PA: Lippincott, Williams & Wilkins. (2012).
28. Greenland S, Pearl J, Robins JM. Causal diagrams for epidemiologic research. Epidemiology. (1999) 10:37–48. doi: 10.1097/00001648-199901000-00008
29. Diplock AT. Safety of antioxidant vitamins and beta-carotene. Am J Clin Nutr. (1995) 62:1510s−6s. doi: 10.1093/ajcn/62.6.1510S
30. Xiao R, Wang S, Guo J, Liu S, Ding A, Wang G, et al. Ferroptosis-related gene NOX4, CHAC1 and HIF1A are valid biomarkers for stomach adenocarcinoma. J Cell Mol Med. (2022) 26:1183–93. doi: 10.1111/jcmm.17171
31. Paolin A, Nardin L, Gaetani P, Rodriguez YBR, Pansarasa O, Marzatico F. Oxidative damage after severe head injury and its relationship to neurological outcome. Neurosurgery. (2002) 51:949–54. doi: 10.1227/00006123-200210000-00018
32. Hans P, Franssen C, Pincemail J, Bertrand Y, Hannique G, Damas P, et al. Plasma myeloperoxidase and vitamin E levels in head injury: preliminary results related to outcome. J Neurosurg Anesthesiol. (1992) 4:26–30. doi: 10.1097/00008506-199201000-00005
33. Currie S, Saleem N, Straiton JA, Macmullen-Price J, Warren DJ, Craven IJ. Imaging assessment of traumatic brain injury. Postgrad Med J. (2016) 92:41–50. doi: 10.1136/postgradmedj-2014-133211
Keywords: vitamin E, prognosis, biomarker, traumatic brain injury, trauma
Citation: Park GJ, Ro YS, Yoon H, Lee SGW, Jung E, Moon SB, Kim SC, Shin SD and Pan-Asia Trauma Outcomes Study for Traumatic Brain Injury (PATOS-TBI) research network (2022) Serum vitamin E level and functional prognosis after traumatic brain injury with intracranial injury: A multicenter prospective study. Front. Neurol. 13:1008717. doi: 10.3389/fneur.2022.1008717
Received: 01 August 2022; Accepted: 23 September 2022;
Published: 19 October 2022.
Edited by:
Lutz Schomburg, Charité Universitätsmedizin Berlin, GermanyReviewed by:
Patrick Haubruck, University of Sydney, AustraliaRaban Heller, Heidelberg University, Germany
Copyright © 2022 Park, Ro, Yoon, Lee, Jung, Moon, Kim, Shin and Pan-Asia Trauma Outcomes Study for Traumatic Brain Injury (PATOS-TBI) research network. This is an open-access article distributed under the terms of the Creative Commons Attribution License (CC BY). The use, distribution or reproduction in other forums is permitted, provided the original author(s) and the copyright owner(s) are credited and that the original publication in this journal is cited, in accordance with accepted academic practice. No use, distribution or reproduction is permitted which does not comply with these terms.
*Correspondence: Young Sun Ro, Ro.youngsun@gmail.com