- 1Department of Neurology, Xuanwu Hospital, Capital Medical University, Beijing, China
- 2Laboratory of Brain Disorders, Beijing Institute of Brain Disorders, Capital Medical University, Beijing, China
- 3Department of Neurosurgery, Wayne State University, Detroit, MI, United States
- 4Department of Neurosurgery, Munson Medical Center, Traverse City, MI, United States
- 5Department of Radiology, Xuanwu Hospital, Capital Medical University, Beijing, China
- 6Beijing Key Laboratory of Hypoxic Conditioning Translational Medicine, Xuanwu Hospital, Capital Medical University, Beijing, China
- 7Department of Emergency, Xuanwu Hospital, Capital Medical University, Beijing, China
Background and Purpose: Moyamoya disease (MMD) is a complicated cerebrovascular disease with recurrent ischemic or hemorrhagic events. This study aimed to prove the safety and efficacy of remote ischemic conditioning (RIC) on MMD.
Methods: In total, 34 patients with MMD participated in this pilot, prospective randomized controlled study for 1 year. 18 patients were allocated into the RIC group, and 16 patients accepted routine medical treatment only. RIC-related adverse events were recorded. The primary outcome was the improvement ratio of mean cerebral blood flow (mCBF) in middle cerebral artery territory measured by multidelay pseudocontinuous arterial spin labeling, and the secondary outcomes were the cumulative incidence of major adverse cerebrovascular events (MACEs), the prevalence of stenotic-occlusive progression, and periventricular anastomosis at 1-year follow-up.
Results: In total, 30 of the 34 patients with MMD completed the final follow-up (17 in the RIC group and 13 in the control group). No adverse events of RIC were observed. The mCBF improvement ratio of the RIC group was distinctively higher compared with the control group (mCBF−whole-brain: 0.16 ± 0.15 vs. −0.03 ± 0.13, p = 0.001). Stenotic-occlusive progression occurred in 11.8% hemispheres in the RIC group and 38.5% in the control group (p = 0.021). The incidence of MACE was 5.9% in the RIC group and 30.8% in the control group (hazard ratio with RIC, 0.174; 95% CI, 0.019–1.557; p = 0.118). No statistical difference was documented in the periventricular anastomosis between the two groups after treatment.
Conclusions: Remote ischemic conditioning has the potential to be a safe and effective adjunctive therapy for patients with MMD largely due to improving cerebral blood flow and slowing the arterial progression of the stenotic-occlusive lesions. These findings warrant future studies in larger trials.
Introduction
Moyamoya disease (MMD) is a complicated cerebrovascular disease, mainly involving children or young adults characterized by stenosis or occlusion at the bifurcation of the circle of Willis and proliferation of basal collaterals (1). Chronic cerebral hypoperfusion can make patients with MMD suffer from ischemic stroke, transient ischemic attack (TIA), cognitive impairment, and even intracranial hemorrhage (2).
The mechanism of MMD has not been fully elucidated, thereby there is no specific medication for MMD. Antiplatelet therapy is utilized to prevent ischemic stroke and TIA, however, it is controversial for patients with MMD, because antiplatelet agents may increase the risk of intracranial hemorrhage in patients with MMD (3). Revascularization surgery has been shown to improve cerebral perfusion and reduce cerebrovascular events for patients with MMD (4–6), nevertheless, complications like hyperperfusion syndrome, postoperative stroke can occur and may lead to neurological deterioration (7).
Remote ischemic conditioning (RIC) is a non-invasive approach protecting the brain by repeated ischemia-reperfusion on the upper limbs. Neuroprotective factors were produced by the stimulus of RIC and these factors conferred a protective effect on remote target organs (8). It has been confirmed that RIC can improve cerebral perfusion by promoting angiogenesis and arteriogenesis in ischemic animal brains (9–11). In addition, daily RIC is a promising technique to ameliorate injury caused by chronic cerebrovascular diseases such as intracranial atherosclerotic stenosis (ICAS), small-vessel disease (12, 13). Meng et al. revealed that RIC could reduce recurrent stroke in patients with symptomatic ICAS by promoting cerebral perfusion (12). Wang et al. reported that daily RIC for 1 year could improve cognitive function and reduce the volume of white matter hyperintensities of small vessel disease (13). Recently, a single-arm study has reported that RIC relieved ischemic events and improved perfusion in ischemic patients with MMD (14). However, the efficacy of RIC for patients with MMD is still unknown in a randomized controlled setting. As far as we know, two randomized clinical trials about the efficacy of RIC for pediatric patients with MMD are in progress (NCT03546309 and NCT03821181) (15) and thus, we conducted a pilot randomized controlled study to explore the safety and efficacy of RIC treating adult patients with MMD to guide further trials.
Methods
Study Design
This was a single-center, open-label, prospective, parallel randomized study from 2019 July to 2021 February in patients with MMD with 1-year treatment at Xuanwu Hospital, Capital Medical University. This study was registered at clinicaltrials.gov with the unique identifier NCT04012268. This protocol was approved by the Ethics Committee of Xuanwu Hospital, Capital Medical University. All the subjects have provided written informed consent.
Inclusion Criteria
Patients who participated in this study met all of the inclusion criteria: (1) Patients aged from 18 to 60 years; (2) All of the patients underwent digital subtraction angiography (DSA) and met the current diagnostic criteria recommended by the Research Committee on MMD of the Ministry of Health and Welfare of Japan in 2012; (3) Modified Rankin Scale (mRs) score ≤ 3; and (4) Informed consent obtained from the patient or acceptable surrogate patient.
Exclusion Criteria
Subjects who met any of the exclusion criteria were excluded from this study: (1) patients with acute ischemic or hemorrhagic stroke within 3 months; (2) severe hepatic or renal dysfunction; (3) severe hemostatic disorder or severe coagulation dysfunction; (4) severe cardiac diseases; (5) patients with severe existing neurological or psychiatric disease; (6) patients with moyamoya syndrome caused by autoimmune disease, Down syndrome, neurofibromatosis, leptospiral infection, or previous skull-base radiation therapy; and (7) patients with completed or planned revascularization surgery.
Randomization and Masking
The subjects diagnosed as MMD by DSA who had not been accepted for revascularization surgery were recruited. After baseline assessment, eligible patients signed informed consent. They were randomized in 1:1 ratio to accept either RIC plus routine medical treatment or routine medical treatment only with a computer-generated randomization code. The code was put into an opaque envelope. The investigators would number the eligible patients and open the envelope to determine the treatment plan. Investigators who assessed outcomes were blinded to the allocation.
Sample Size
This was a pilot randomized controlled study. There were no parameters referred to estimate the sample size, and Hertzog (16) has shown that 10–20 subjects per group are adequate to evaluate the feasibility in a pilot study. In addition, Dobkin (17) has suggested that 15 subjects in each group are enough to evaluate whether a larger multicenter trial should be implemented. Thus, we aimed to recruit 15 patients per group, and finally, we recruited a total of 34 patients in this study.
Procedures
Patients in the RIC group accepted bilateral upper limbs RIC performed by an autocontrol device (patent no.: ZL200820123637.X, China), including five cycles of inflating and deflating for 5 min alternately twice daily for 1 year. The inflating pressure was 200 mm Hg. The investigators performed telephone follow-ups every month to assure patients insisting on this treatment. Considering the effect of single-time RIC could sustain 4 days (18), the compliance of 1 month would be considered substandard if the patient discontinued RIC treatment for consecutive 4 days of the month. Both groups accepted routine medical treatment that includes antiplatelet therapy, lipid-lowering therapy, controlling of vascular risk factors, and adjunct drug butylphthalide. Antiplatelet therapy was not available for asymptomatic and hemorrhagic patients in this study.
Outcome Assessment
The primary outcome was the mean cerebral blood flow (mCBF) improvement ratio after 1-year treatment. Secondary outcomes were the progression of stenotic-occlusive lesion, the change of periventricular anastomosis, and the incidence of major adverse cerebrovascular events (MACEs). The outcomes of safety were the incidence of adverse events related to RIC and the change of hematologic indexes. Fasting blood of patients was collected and hematologic indexes were examined including white blood cell count (WBC), red blood cell count (RBC), hemoglobin (HGB), and platelet count (PLT). Alanine transaminase (ALT) and aspartate aminotransferase (AST) were examined to reflect hepatic function, creatinine (CREA), and urea nitrogen (UREA) were obtained to assess renal function. Creatine kinase (CK), a biomarker for reflecting skeletal muscle, was also tracked to monitor for injury. Multidelay pseudocontinuous arterial spin-labeling MR (PCASL-MR), time-of-flight MR angiography (TOF-MRA), and hematologic indexes collection were performed within 1 week before and 1 year after the treatment.
Baseline and Follow-Up Evaluations
Demographic characteristics, clinical history including ischemic stroke-related risk factors, symptoms, concomitant diseases, and routine medical treatments were recorded. All participants underwent 3T black-blood T1-weighted intracranial vessel wall imaging to exclude moyamoya syndrome (such as ICAS, vasculitis, and so on).
Cerebral Blood Flow Evaluation
Neuroradiologic outcomes were assessed by two experienced radiologists who were blinded to the allocation, the result would be obtained when a consensus was achieved. The images of cerebral blood flow (CBF) were acquired from PCASL-MR which were performed at 12 ± 0.5 months from baseline. A 3T imaging system (Verio, Siemens Healthcare, Erlangen, Germany) with a 32-channel head coil was applied. The PCASL images were obtained by an acquisition protocol with an acquisition time of 5 min and 40 s, post-labeling delay of 1,500/2,000/2,500/3,000 ms, echo time of 22.67 ms, 26 scan slices, slice thickness of 5 mm, and a 64 × 64 matrix resolution. Data of PCASL in DICOM form were converted to perfusion maps. The CBF map was processed by OsiriX (University of California, Los Angeles, USA). In each hemisphere, the middle cerebral artery (MCA) territory was divided into 10 regions according to Albert Stroke Program Early CT score (ASPECTS). Regions of interest (ROI) were drawn manually in each region of the MCA to determine the absolute CBF values in slices of the corona radiata and the basal ganglia (detailed ROIs were depicted in Supplementary Figure I).
Mean CBF was calculated by formula: mCBF−hemisphere = CBF (M1 + M2 + M3 + M4 + M5 + M6 + insula + internal capsule + lentiform nucleus + caudate)/10; mean CBF of the whole brain (mCBF−whole-brain) was defined as the average value of mCBF in the left and right hemisphere of the patient. mCBF−cortex was the average value of CBF values in bilateral M1-M6 and insula. The mCBF−basal−ganglia was defined as the mean value of CBF values in bilateral internal capsule, lentiform and caudate. The improvement ratio of corresponding mCBF was calculated by the formula: the mCBF improvement ratio = (mCBF at 1 year–mCBF at baseline)/mCBF at baseline.
Evaluation of Periventricular Anastomosis and Arterial Lesion Progression
The periventricular anastomosis and arterial lesion progression were evaluated by 3T 3D TOF-MRA. All the subjects were examined on a 3-Tesla system (Magnetom Verio; Siemens Healthineers, Erlangen, Germany), a 32-channel head coil was used for signal reception at 3T. The parameters of 3D TOF-MRA included: axial plane, flip angle 20°, TR/TE = 22/3.99 ms, FOV = 180 × 180 mm2, slice thickness = 0.8 mm, and matrix = 320 × 320).
The periventricular anastomosis is a connection beginning at the perforating arteries ending at the medullary artery at the lateral corner of the frontal horn or body of the lateral ventricle. The periventricular anastomosis was classified into three types: lenticulostriate anastomosis, thalamic anastomosis, and choroidal anastomosis (19). Each type of anastomosis was recorded as “presence” for “positive” and “absence” for “negative” from TOF-MRA images at sagittal orientation.
Arterial progression could be confirmed according to following conditions: (1) compared to baseline, the stenotic or occlusive lesion extended to another segment of Willis circle (for example, lesion extended from MCA M1 segment to M2 segment); (2) lesion extended from proximal portion to distal portion of one segment (such as extending from proximal portion to distal portion of MCA M1 segment); (3) stenotic lesion developed as the occlusive lesion. Houkin's MRA score (20) was used to evaluate the status of the arterial lesion in the Willis circle at baseline and 1 year follow-up.
Major Adverse Cerebrovascular Events Evaluation
Major adverse cerebrovascular events include hospital admissions with the TIA, ischemic stroke, and hemorrhagic stroke in this study. Patients were required to record the adverse events and were telephoned by two experienced neurologists every 3 months.
Statistical Analysis
To compare the characteristics at baseline or follow-up of the RIC group with the control group, continuous variables described as mean ± SD or medians (IQRs) were analyzed by the independent Student's t-test or the Mann–Whitney U test; categorical variables described as proportions were analyzed by the χ2 test or the Fisher exact test using SPSS Statistics Version 23 (IBM Incorporation, Armonk, New York, USA). To further evaluate the effect of RIC on the progression of stenotic-occlusive lesion, the binary logistic regression analysis was used to determine the odds ratio (OR) with RIC. For comparing the cumulative incidence of MACEs at 12 months, the Cox proportional-hazards models were used. Interrater agreements of CBF measurements were evaluated by intraclass correlation coefficients (ICC). Consistency between two radiologists is considered good when the ICC value ≥ 0.75. p < 0.05 was considered to be statistically significant for all the tests. All the tests were 2-sided.
Results
After screening 114 patients diagnosed as adult MMD, 34 patients were recruited. In total, 18 patients were allocated to the RIC group and 16 patients were in the control group. A total of 17 patients in the RIC group completed the 1-year follow-up, while 13 patients in the control group completed the follow-up (Figure 1). Thereby, a total of 30 patients (60 hemispheres) were included in this per-protocol analysis. In the RIC group, all the patients complied ≥80% of the months.
Baseline Characteristics
There were no significant differences between the two groups in demographic characteristics, symptoms, concomitant disease, medical treatment (Table 1), and hematologic indexes (Table 2). mCBF of the whole brain (RIC vs. control: 43.03 ± 8.70 vs. 47.06 ± 8.44 ml/100 g per min, p = 0.213), cortex (RIC vs. control: 45.07 ± 9.40 vs. 49.11 ± 10.23 ml/100 g per min, p = 0.278), basal ganglia (RIC vs. control: 38.28 ± 8.36 vs. 42.28 ± 10.30 ml/100 g per min, p = 0.243) were all not significantly different between the two groups at baseline (Table 2). In addition, no significant difference was shown in any type of periventricular anastomosis between the two groups (detailed characteristics were listed in Table 3).
Safety Outcome
No severe adverse events related to RIC occurred in 17 patients of the RIC group during the 1-year treatment. The hematologic indexes reflecting hepatic, renal function, and muscle injury remained normal and no statistical difference was documented (Table 2).
Efficacy Outcome
Neuroradiologic Outcomes
The inter-rater agreement was good in evaluating CBF by two radiologists. Intraclass correlation coefficients was 0.942 (95% CI, 0.882–0.972), 0.911 (95% CI, 0.821–0.956), 0.930 (95% CI, 0.858–0.966) for improvement ratio of mCBF−whole−brain, mCBF−cortex, and mCBF−basal−ganglia, respectively. The improvement ratio of mCBF−whole−brain in the RIC group was significantly higher than that in the control group (0.16 ± 0.15 vs. −0.03 ± 0.13, p = 0.001). The mCBF of the cortex and the basal ganglia in the RIC group were improved by ratios of 0.16 ± 0.18, 0.15 ± 0.18, respectively, which were significantly higher than those in the control group (p < 0.01). The mCBF improvement ratios of the cortex and the basal ganglia in the control group were −0.01 ± 0.13, −0.08 ± 0.16, respectively. In addition, there was no significant difference in the improvement ratio between cortex and basal ganglia in the RIC group (p = 0.830). Detailed information is shown in Table 2; Figure 2.
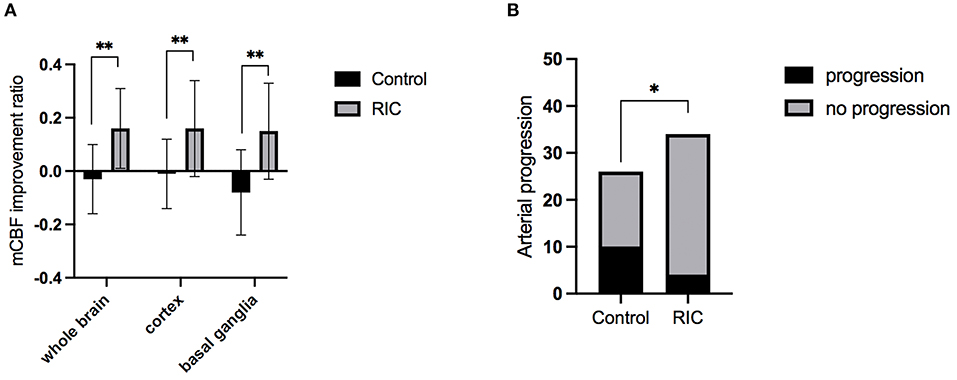
Figure 2. The mCBF improvement ratio and stenotic-occlusive progression of two groups. (A) mCBF improvement ratio of whole brain, cortex, and basal ganglia between the two groups at 1-year treatment; (B) the number of hemispheres with arterial progression in two groups at 1-year follow-up; mCBF, mean cerebral blood flow; *p < 0.05; **p < 0.01.
The progression of the stenotic or occlusive lesion in the Willis circle was seen in 10 (38.5%) hemispheres in the control group, while four (11.8%) in the RIC group (OR with RIC, 0.21; 95% CI, 0.06–0.79, p = 0.021). In the control group, five hemispheres suffered lesion progression from segment M1 to other segments [four to M2 and one to anterior cerebral artery (ACA)]; three progressed from proximal to distal M1 segment; one progressed from stenotic lesion to occlusive lesion at M1 segment and one progressed from ACA A1 to A2 segment. In the RIC group, three hemispheres suffered progression from segment M1 to other segments (two to M2, one to ACA) and one progressed from proximal to distal M1 segment. The improvement of CBF and stenotic-occlusive lesion of two subjects were shown in Figure 3. No significant difference was documented in MRA score at baseline and 1-year follow-up between the two groups (Table 3). But, we found MRA score of the control group significantly increased after 1-year medical treatment [3.5 (2–5) vs. 4 (3–5), p = 0.002], while the MRA score of the RIC group kept stable [3 (3–4) vs. 3 (3–4), p = 0.739].
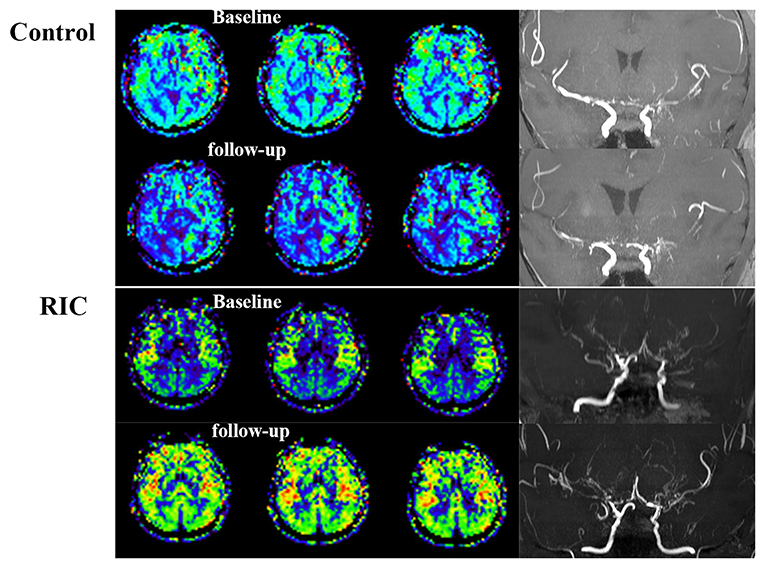
Figure 3. Cerebral blood flow and stenotic-occlusive lesion at baseline and follow-up. ASL images of the patient in the control group showed deterioration of CBF mainly at the right side, which could be reflected by the progression of stenotic-occlusive lesion at right middle cerebral artery (MCA). arterial spin labeling (ASL) images of the remote ischemic conditioning (RIC) group demonstrated improved CBF and more abundant collaterals after RIC treatment in both the sides.
At 1-year follow-up, there were still no differences in three types of periventricular anastomosis between the two groups (p > 0.05).
Clinical Outcomes
Major adverse cerebrovascular events occurred in 30.8% of patients in the control group, one suffered TIA, two suffered an ischemic stroke, and one suffered a hemorrhagic stroke. In the RIC group, only one patient (5.9%) suffered TIA and no patients suffered an ischemic or hemorrhagic stroke. However, there was no evidence of a significant difference in cumulative incidence of MACE between the control group and RIC group (hazard ratio with RIC, 0.17; 95% CI, 0.019–1.56, p = 0.118) (Figure 4). In addition, there was no distinct difference in any components of MACE containing TIA, ischemic stroke, and hemorrhagic stroke (Supplementary Table I).
Discussion
Previous studies have shown that daily RIC was well-tolerated and safe in patients with symptomatic ICAS and small vessel disease (12, 13). The present study showed that no patient in the RIC group suffered any RIC-related adverse events and routine blood tests, renal, or hepatic function were normal during 1-year treatment.
Efficacy evaluation indicated that RIC could significantly improve the CBF and alleviate the progression of the stenotic-occlusive lesion in patients with MMD compared with the control group. Besides, the presence of periventricular anastomosis was not statistically different between the two groups. Although non-significantly, there existed a tendency that patients in the RIC group had a lower incidence of MACEs.
Patients with MMD with misery cerebral perfusion have a higher risk of subsequent ischemic stroke (21–23). Recent studies also indicated insufficient perfusion may cause future hemorrhagic events (24, 25). Direct surgery has been generally performed to improve the CBF of MCA territory immediately (26). In addition to improving outcomes of ischemic patients with MMD, a Japan Adult Moyamoya (JAM) trial was conducted to reveal that direct surgery could also reduce rebleeding attacks for hemorrhagic patients (6). The improved perfusion at MCA territory supplied by the external carotid artery system can reduce the hemodynamic burden of choroidal or thalamic collaterals, and then prevents subsequent rupture. Other retrospective studies also indicated a lower risk of cerebral infarction or hemorrhage in patients with MMD after revascularization (27–29). Hence, both cerebrovascular events and improved perfusion are major outcomes that need to be evaluated after revascularization surgery (26).
Thus, it is crucial for patients with MMD to improve and maintain cerebral perfusion of MCA territory. RIC has been shown to improve CBF of patients with chronic circulation insufficiency like ICAS by promoting angiogenesis or arteriogenesis (9–11, 30, 31). A recent single-arm study also observed an improvement of cerebral perfusion in patients with MMD by RIC treatment (14). This study showed that mCBF of the MCA region was improved in the RIC group. The control group conversely tends to have a reduced CBF at 1 year from baseline. Several studies reported that revascularization surgery mainly improved cortical perfusion compared with central regions (32). Our results showed no difference in the CBF improvement ratio between cortex and basal ganglia, which demonstrated that the CBF was increased evenly by RIC treatment. The detailed pathway of improving CBF in patients with MMD by RIC is still not clearly understood. Considering unchanged periventricular anastomosis of basal collaterals after RIC treatment in this study, we assumed that the ischemia tissue may be improved by angiogenesis or arteriogenesis from the leptomeningeal system or external carotid artery system and more stable status of Willis circle. Further studies need to be constructed to validate the assumption by DSA.
The absolute CBF was measured by ASL in this study, which has become a validated technique to monitor CBF changes after revascularization surgery without a contrast agent (26). There is a concern that ASL will underestimate the CBF value in patients with MMD, thus multidelay PCASL-MR that can alleviate the underestimation was utilized (33, 34).
Progressive stenosis or occlusion at Willis circle is one of the characteristics of MMD, which can induce a series of symptoms (35, 36). We evaluated the progression of the stenotic-occlusive lesion and found that patients in the RIC group had a lower risk of arterial progression within 1 year, which may be related to the effects of RIC on improving endothelial function (37), ameliorating inflammation (38), and promotion of arteriogenesis or angiogenesis (9, 10). A recent study showed that RIC could ameliorate vascular remodeling in conduit artery and small resistance artery of hypertensive rats by suppressing deposition of the extracellular matrix and hypertrophy of smooth muscle cells (39). It indicated that RIC may prevent arterial progression through ameliorating vascular remodeling in patients with MMD. But the pattern of vascular remodeling is different between MMD and hypertension, the definite mechanism still needs to be further explored.
The regression of dilated and extended anterior choroidal arteries was seen in patients with MMD after revascularization surgery (40, 41), but it was not found after RIC treatment. The method to evaluate choroidal arteries on non-invasive imaging, relatively short-time follow-up, and small sample size in this study may contribute to the result.
The MACE rate of the RIC group was lower than that in the control group, but it was not statistical. In this study, subjects were mostly composed of the ischemic-onset patients with MMD, and the recurrence rate of ischemic events was reported 3–10% which was relatively low (42, 43). A previous retrospective study with a large sample size also could not get a significant difference in the incidence of recurrent stroke between the surgery group and conservative group until 10-year follow-up (44). Long-term follow-up (3–5 years and even more than 10 years) were commonly used in previous studies observing the efficacy of revascularization surgery (6, 44, 45). Thus, we thought that 1-year follow-up in this study with a small sample size was not sufficient to observe a significant difference in MACE rate between the two groups, and long-term follow-up should be implemented in further research.
Although this study could not determine the effect of RIC on the prevention of cerebrovascular events, the improved CBF of patients with MMD which could be an indicator of better long-term outcome was confirmed. Thus, daily RIC could be an alternative therapy for patients with MMD in the future and will need further study.
This study has some limitations. Small sample size may bring selection bias for this study. There existed a discrepancy in the sample size between the two groups which may be due to the demand for further treatment of patients in the control group without a placebo. The mechanism of RIC for MMD needs further studies to reveal.
Our findings demonstrated that daily RIC treatment is safe and well-tolerated for adult MMD patients. RIC can improve the CBF and halt the progression of stenotic-occlusive lesions. Subjects in the RIC group tended to have a lower risk of MACEs. Thus, RIC seems to be a potential treatment approach for MMD.
Data Availability Statement
The raw data supporting the conclusions of this article will be made available by the authors, without undue reservation.
Ethics Statement
The studies involving human participants were reviewed and approved by Ethics Committee of Xuanwu Hospital, Capital Medical University. The patients/participants provided their written informed consent to participate in this study.
Author Contributions
XJ, SL, QZ, and JX contributed to conceptualization. JX and QZ contributed to writing the original draft. JX, BZ, and WG contributed to the investigation. QY and FW contributed to the methodology. BZ, XX, and WG contributed to data curation. SL, YD, and GR contributed to writing, reviewing, and editing. WZ contributed to the formal analysis. FW contributed to the visualization. JX and SL contributed to project administration. XJ contributed to supervision. SL and XJ contributed to funding acquisition. All authors have read and agreed to the published version of the manuscript.
Funding
This study was supported by the National Natural Science Foundation of China (No. 81801313) and the Beijing Municipal Administration of Hospitals Incubating Program (No. PX2019028).
Conflict of Interest
XJ is one of the inventors of the electric autocontrol device that has been patented in China (ZL200820123637.X, China).
The remaining authors declare that the research was conducted in the absence of any commercial or financial relationships that could be construed as a potential conflict of interest.
Publisher's Note
All claims expressed in this article are solely those of the authors and do not necessarily represent those of their affiliated organizations, or those of the publisher, the editors and the reviewers. Any product that may be evaluated in this article, or claim that may be made by its manufacturer, is not guaranteed or endorsed by the publisher.
Supplementary Material
The Supplementary Material for this article can be found online at: https://www.frontiersin.org/articles/10.3389/fneur.2021.811854/full#supplementary-material
References
1. Scott RM, Smith ER. Moyamoya disease and moyamoya syndrome. N Engl J Med. (2009) 360:1226–37. doi: 10.1056/NEJMra0804622
2. Kuroda S, Houkin K. Moyamoya disease: current concepts and future perspectives. Lancet Neurol. (2008) 7:1056–66. doi: 10.1016/S1474-4422(08)70240-0
3. Guidelines for diagnosis and treatment of moyamoya disease (spontaneous occlusion of the circle of Willis). Neurol Med Chir. (2012) 52:245–66. doi: 10.2176/nmc.52.245
4. Jeon JP, Kim JE, Cho WS, Bang JS, Son YJ, Oh CW. Meta-analysis of the surgical outcomes of symptomatic moyamoya disease in adults. J Neurosurg. (2018) 128:793–9. doi: 10.3171/2016.11.JNS161688
5. Lee SU, Oh CW, Kwon OK, Bang JS, Ban SP, Byoun HS, et al. Surgical Treatment of Adult Moyamoya Disease. Curr Treat Options Neurol. (2018) 20:22. doi: 10.1007/s11940-018-0511-8
6. Miyamoto S, Yoshimoto T, Hashimoto N, Okada Y, Tsuji I, Tominaga T, et al. Effects of extracranial-intracranial bypass for patients with hemorrhagic moyamoya disease: results of the Japan Adult Moyamoya Trial. Stroke. (2014) 45:1415–21. doi: 10.1161/STROKEAHA.113.004386
7. Hazra DN, Ghosh AK. Complications following Encephalo-Duro-Arterio-Myo-Synangiosis in a case of Moyamoya disease. Brain Circ. (2020) 6:57–9. doi: 10.4103/bc.bc_27_19
8. Hausenloy DJ, Yellon DM. Remote ischaemic preconditioning: underlying mechanisms and clinical application. Cardiovasc Res. (2008)79: 377–86. doi: 10.1093/cvr/cvn114
9. Ren C, Li S, Wang B, Han R, Li N, Gao J, et al. Limb remote ischemic conditioning increases Notch signaling activity and promotes arteriogenesis in the ischemic rat brain. Behav Brain Res. (2018) 340:87–93. doi: 10.1016/j.bbr.2016.10.036
10. Ren C, Li N, Li S, Han R, Huang Q, Hu J, et al. Limb ischemic conditioning improved cognitive deficits via eNOS-dependent augmentation of angiogenesis after chronic cerebral hypoperfusion in rats. Aging Dis. (2018) 9:869–79. doi: 10.14336/AD.2017.1106
11. Khan MB, Hafez S, Hoda MN, Baban B, Wagner J, Awad ME, et al. Chronic remote ischemic conditioning is cerebroprotective and induces vascular remodeling in a VCID model. Transl Stroke Res. (2018) 9:51–63. doi: 10.1007/s12975-017-0555-1
12. Meng R, Asmaro K, Meng L, Liu Y, Ma C, Xi C, et al. Upper limb ischemic preconditioning prevents recurrent stroke in intracranial arterial stenosis. Neurology. (2012) 79:1853–61. doi: 10.1212/WNL.0b013e318271f76a
13. Wang Y, Meng R, Song H, Liu G, Hua Y, Cui D, et al. Remote ischemic conditioning may improve outcomes of patients with cerebral small-vessel disease. Stroke. (2017) 48:3064–72. doi: 10.1161/STROKEAHA.117.017691
14. Ding JY, Shang SL, Sun ZS, Asmaro K, Li WL, Yang Q, et al. Remote ischemic conditioning for the treatment of ischemic moyamoya disease. CNS Neurosci Ther. (2020) 26:549–57. doi: 10.1111/cns.13279
15. Li S, Zhao W, Han C, Rajah GB, Ren C, Xu J, et al. Safety and efficacy of remote ischemic conditioning in pediatric moyamoya disease patients treated with revascularization therapy. Brain Circ. (2017) 3:213–8. doi: 10.4103/bc.bc_30_17
16. Hertzog MA. Considerations in determining sample size for pilot studies. Res Nurs health. (2008) 31:180–91. doi: 10.1002/nur.20247
17. Dobkin BH. Progressive Staging of Pilot Studies to Improve Phase III Trials for Motor Interventions. Neurorehabil Neural Repair. (2009) 23:197–206. doi: 10.1177/1545968309331863
18. Yellon DM, Downey JM. Preconditioning the myocardium: from cellular physiology to clinical cardiology. Physiol rev. (2003) 83:1113–51. doi: 10.1152/physrev.00009.2003
19. Funaki T, Takahashi JC, Yoshida K, Takagi Y, Fushimi Y, Kikuchi T, et al. Periventricular anastomosis in moyamoya disease: detecting fragile collateral vessels with MR angiography. J Neurosurg. (2016) 124:1766–72. doi: 10.3171/2015.6.JNS15845
20. Deng X, Zhang Z, Zhang Y, Zhang D, Wang R, Ye X, et al. Comparison of 70- and 30-T MRI and MRA in ischemic-type moyamoya disease: preliminary experience. J Neurosurg. (2016) 124:1716–25. doi: 10.3171/2015.5.JNS15767
21. Noh HJ, Kim SJ, Kim JS, Hong SC, Kim KH, Jun P, et al. Long term outcome and predictors of ischemic stroke recurrence in adult moyamoya disease. J Neurol Sci. (2015) 359:381–8. doi: 10.1016/j.jns.2015.11.018
22. Nam KW, Cho WS, Kwon HM, Kim JE, Lee YS, Park SW, et al. Ivy sign predicts ischemic stroke recurrence in adult moyamoya patients without revascularization surgery. Cerebrovas Dis. (2019) 47:223–30. doi: 10.1159/000500610
23. Hervé D, Ibos-Augé N, Calvière L, Rogan C, Labeyrie MA, Guichard JP, et al. Predictors of clinical or cerebral lesion progression in adult moyamoya angiopathy. Neurology. (2019) 93:e388–97. doi: 10.1212/WNL.0000000000007819
24. Takahashi JC, Funaki T, Houkin K, Kuroda S, Fujimura M, Tomata Y, et al. Impact of cortical hemodynamic failure on both subsequent hemorrhagic stroke and effect of bypass surgery in hemorrhagic moyamoya disease: a supplementary analysis of the Japan Adult Moyamoya Trial. J Neurosurg. (2020) 134:1–6. doi: 10.3171/2020.1.JNS192392
25. Jo KI, Kim MS, Yeon JY, Kim JS, Hong SC. Recurrent bleeding in hemorrhagic moyamoya disease: prognostic implications of the perfusion status. J Korean Neurosurg Soc. (2016) 59:117–21. doi: 10.3340/jkns.2016.59.2.117
26. Lee S, Yun TJ, Yoo RE, Yoon BW, Kang KM, Choi SH, et al. Monitoring cerebral perfusion changes after revascularization in patients with moyamoya disease by using arterial spin-labeling MR imaging. Radiology. (2018) 288:565–72. doi: 10.1148/radiol.2018170509
27. Deng X, Gao F, Zhang D, Zhang Y, Wang R, Wang S, et al. Direct versus indirect bypasses for adult ischemic-type moyamoya disease: a propensity score-matched analysis. J Neurosurg. (2018) 128:1785–91. doi: 10.3171/2017.2.JNS162405
28. Abhinav K, Furtado SV, Nielsen TH, Lyer A, Gooderham PA, Teo M, et al. Functional outcomes after revascularization procedures in patients with hemorrhagic moyamoya disease. Neurosurgery. (2020) 86:257–65. doi: 10.1093/neuros/nyz074
29. Park SE, Kim JS, Park EK, Shim KW, Kim DS. Direct versus indirect revascularization in the treatment of moyamoya disease. J Neurosurg. (2018) 129:480–9. doi: 10.3171/2017.5.JNS17353
30. You J, Feng L, Bao L, Xin M, Ma D, Feng J. Potential Applications of remote limb ischemic conditioning for chronic cerebral circulation insufficiency. Front Neurol. (2019) 10:467. doi: 10.3389/fneur.2019.00467
31. Wang L, Ren C, Li Y, Gao C, Li N, Li H, et al. Remote ischemic conditioning enhances oxygen supply to ischemic brain tissue in a mouse model of stroke: Role of elevated 2,3-biphosphoglycerate in erythrocytes. J Cereb Blood Flow Metab. (2021) 41:1277–90. doi: 10.1177/0271678X20952264
32. Schubert GA, Czabanka M, Seiz M, Horn P, Vajkoczy P, Thomé C. Perfusion characteristics of Moyamoya disease: an anatomically and clinically oriented analysis and comparison. Stroke. (2014) 45:101–6. doi: 10.1161/STROKEAHA.113.003370
33. Wang R, Yu S, Alger JR, Zuo Z, Chen J, Wang R, et al. Multi-delay arterial spin labeling perfusion MRI in moyamoya disease–comparison with CT perfusion imaging. Eur Radiol. (2014) 24:1135–44. doi: 10.1007/s00330-014-3098-9
34. Fan AP, Guo J, Khalighi MM, Gulaka PK, Shen B, Park JH, et al. Long-delay arterial spin labeling provides more accurate cerebral blood flow measurements in moyamoya patients: a simultaneous positron emission tomography/MRI study. Stroke. (2017) 48:2441–9. doi: 10.1161/STROKEAHA.117.017773
35. Funaki T, Takahashi JC, Takagi Y, Kikuchi T, Yoshida K, Mitsuhara T, et al. Unstable moyamoya disease: clinical features and impact on perioperative ischemic complications. J Neurosurg. (2015) 122:400–7. doi: 10.3171/2014.10.JNS14231
36. Yu L, Ma L, Huang Z, Shi Z, Wang R, Zhao Y, et al. Revascularization surgery in patients with ischemic-type moyamoya disease: predictors for postoperative stroke and long-term outcomes. World Neurosurg. (2019) 128:e582–96. doi: 10.1016/j.wneu.2019.04.214
37. Jones H, Hopkins N, Bailey TG, Green DJ, Cable NT, Thijssen DH. Seven-day remote ischemic preconditioning improves local and systemic endothelial function and microcirculation in healthy humans. Am J Hypertens. (2014) 27:918–25. doi: 10.1093/ajh/hpu004
38. Khan MB, Hoda MN, Vaibhav K, Giri S, Wang P, Waller JL, et al. Remote ischemic postconditioning: harnessing endogenous protection in a murine model of vascular cognitive impairment. Transl stroke Res. (2015) 6:69–77. doi: 10.1007/s12975-014-0374-6
39. Gao Y, Ren C, Li X, Yu W, Li S, Li H, et al. Ischemic conditioning ameliorated hypertension and vascular remodeling of spontaneously hypertensive rat via inflammatory regulation. Aging Dis. (2021)12:116–31. doi: 10.14336/AD.2020.0320
40. Wang QN, Bao XY, Zhang Y, Zhang Q, Li DS, Duan L, et al. Encephaloduroarteriosynangiosis for hemorrhagic moyamoya disease: long-term outcome of a consecutive series of 95 adult patients from a single center. J Neurosurg. (2019) 130:1898–905. doi: 10.3171/2017.12.JNS172246
41. Kang K, Lu J, Ju Y, Ji R, Wang D, Shen Y, et al. Clinical and radiological outcomes after revascularization of hemorrhagic moyamoya disease. Front Neurol. (2020) 11:382. doi: 10.3389/fneur.2020.00382
42. Miyoshi K, Chida K, Kobayashi M, Kubo Y, Yoshida K, Terasaki K, et al. Two-year clinical, cerebral hemodynamic, and cognitive outcomes of adult patients undergoing medication alone for symptomatically ischemic moyamoya disease without cerebral misery perfusion: a prospective cohort study. Neurosurgery. (2019) 84:1233–41. doi: 10.1093/neuros/nyy234
43. Cho WS, Chung YS, Kim JE, Jeon JP, Son YJ, Bang JS, et al. The natural clinical course of hemodynamically stable adult moyamoya disease. J Neurosurg. (2015) 122:82–9. doi: 10.3171/2014.9.JNS132281
44. Kim T, Oh CW, Kwon OK, Hwang G, Kim JE, Kang HS, et al. Stroke prevention by direct revascularization for patients with adult-onset moyamoya disease presenting with ischemia. J Neurosurg. (2016) 124:1788–93. doi: 10.3171/2015.6.JNS151105
Keywords: moyamoya disease, stroke, remote ischemic conditioning, cerebral blood flow, arterial spin labeling (ASL)
Citation: Xu J, Zhang Q, Rajah GB, Zhao W, Wu F, Ding Y, Zhang B, Guo W, Yang Q, Xing X, Li S and Ji X (2022) Daily Remote Ischemic Conditioning Can Improve Cerebral Perfusion and Slow Arterial Progression of Adult Moyamoya Disease—A Randomized Controlled Study. Front. Neurol. 12:811854. doi: 10.3389/fneur.2021.811854
Received: 09 November 2021; Accepted: 20 December 2021;
Published: 03 February 2022.
Edited by:
Francisco Purroy, Lleida Institute for Biomedical Research (IRBLleida), SpainReviewed by:
Zhen-Ni Guo, First Affiliated Hospital of Jilin University, ChinaWon-Sang Cho, Seoul National University Hospital, South Korea
Copyright © 2022 Xu, Zhang, Rajah, Zhao, Wu, Ding, Zhang, Guo, Yang, Xing, Li and Ji. This is an open-access article distributed under the terms of the Creative Commons Attribution License (CC BY). The use, distribution or reproduction in other forums is permitted, provided the original author(s) and the copyright owner(s) are credited and that the original publication in this journal is cited, in accordance with accepted academic practice. No use, distribution or reproduction is permitted which does not comply with these terms.
*Correspondence: Sijie Li, bGlzaWppZUB4d2guY2NtdS5lZHUuY24=; Xunming Ji, aml4bUBjY211LmVkdS5jbg==
†These authors have contributed equally to this work