- 1Program in Neuroengineering, Edmond and Lily Safra International Institute of Neuroscience, Santos Dumont Institute, Macaíba, Brazil
- 2Department of Physical Education, Federal University of Rio Grande do Norte, Natal, Brazil
- 3Department of Biomedical Engineering, The City College of New York, New York, NY, United States
- 4Neuroscience and Aging Laboratory, Federal University of Paraíba, João Pessoa, Brazil
- 5Center for Mathematics, Computing and Cognition, Federal University of ABC, São Bernardo do Campo, Brazil
- 6Rehabilitation Center, Anita Garibaldi Center for Education and Health, Santos Dumont Institute, Macaíba, Brazil
- 7Department of Biomedical Sciences, State University of Rio Grande do Norte, Mossoró, Brazil
- 8Neuron—Care Unit in Neurosurgery, Hospital Rio Grande, Natal, Brazil
- 9Program in Rehabilitation Science, Program in Health Science, Federal University of Rio Grande do Norte, Natal, Brazil
Background: Clinical impact of transcranial direct current stimulation (tDCS) alone for Parkinson's disease (PD) is still a challenge. Thus, there is a need to synthesize available results, analyze methodologically and statistically, and provide evidence to guide tDCS in PD.
Objective: Investigate isolated tDCS effect in different brain areas and number of stimulated targets on PD motor symptoms.
Methods: A systematic review was carried out up to February 2021, in databases: Cochrane Library, EMBASE, PubMed/MEDLINE, Scopus, and Web of science. Full text articles evaluating effect of active tDCS (anodic or cathodic) vs. sham or control on motor symptoms of PD were included.
Results: Ten studies (n = 236) were included in meta-analysis and 25 studies (n = 405) in qualitative synthesis. The most frequently stimulated targets were dorsolateral prefrontal cortex and primary motor cortex. No significant effect was found among single targets on motor outcomes: Unified Parkinson's Disease Rating Scale (UPDRS) III – motor aspects (MD = −0.98%, 95% CI = −10.03 to 8.07, p = 0.83, I2 = 0%), UPDRS IV – dyskinesias (MD = −0.89%, CI 95% = −3.82 to 2.03, p = 0.55, I2 = 0%) and motor fluctuations (MD = −0.67%, CI 95% = −2.45 to 1.11, p = 0.46, I2 = 0%), timed up and go – gait (MD = 0.14%, CI 95% = −0.72 to 0.99, p = 0.75, I2 = 0%), Berg Balance Scale – balance (MD = 0.73%, CI 95% = −1.01 to 2.47, p = 0.41, I2 = 0%). There was no significant effect of single vs. multiple targets in: UPDRS III – motor aspects (MD = 2.05%, CI 95% = −1.96 to 6.06, p = 0.32, I2 = 0%) and gait (SMD = −0.05%, 95% CI = −0.28 to 0.17, p = 0.64, I2 = 0%). Simple univariate meta-regression analysis between treatment dosage and effect size revealed that number of sessions (estimate = −1.7, SE = 1.51, z-score = −1.18, p = 0.2, IC = −4.75 to 1.17) and cumulative time (estimate = −0.07, SE = 0.07, z-score = −0.99, p = 0.31, IC = −0.21 to 0.07) had no significant association.
Conclusion: There was no significant tDCS alone short-term effect on motor function, balance, gait, dyskinesias or motor fluctuations in Parkinson's disease, regardless of brain area or targets stimulated.
Introduction
Parkinson's disease (PD) is a chronic, multisystemic, neurodegenerative disorder with various mechanisms underlying its neuropathology (1). PD is standing out as a leading cause of disability-adjusted life year (DALY) globally (increasing 148% between 1990 and 2016), the most growing neurological disorder according to the Global Burden of Disease 2016 (2), and affecting 6.1 million people (3). As an aggravating factor, the forecast predicts that this number will double in the next generation (3).
Parkinson's disease is characterized by a triad of cardinal symptoms (bradykinesia, tremor, and rigidity). Bradykinesia or slowness of movement is the most characteristic motor symptom (4), covering many motor manifestations (5). Tremor initially appears unilateral and progresses to bilateral, worsening in stressful circumstances or cognitive tasks, and can be attenuated during sleep or movement (6). Rigidity causes constant or oscillating resistance to passive joint movement and can be increased by tasks demanding attention (7).
Among the current treatments available, drug administration is the most common option. However, a significant decrease in response to a drug occurs ~5 years after initial treatment, worsening motor fluctuations, dyskinesia, dystonia, incoordination, and arthralgia (9). Neurosurgical procedures involving deep brain stimulation are another option, but this method presents high cost (9), surgical risk (8), and possibility of worsening of verbal fluency and axial motor symptoms (8, 9). Appropriate interventions present little or no adverse effects, improve functionality and well-being, and delay the progression of the disease (9). Thus, new therapeutic approaches are necessary to provide a better quality of life and to reduce the financial burden for society and health systems.
Transcranial direct current stimulation (tDCS) has gained prominence for being a non-invasive, safe, low-cost neuromodulatory modality, with minimal or no adverse effect (10, 11). Its mechanisms of action go far beyond the elementary reasoning that anodic (a-tDCS) and cathodic (c-tDCS) stimulation increases or decreases, respectively, somatic polarity, excitability, and neuronal plasticity (12). Considering the complex functioning of the brain, the neurophysiology underlying tDCS is much more heterogeneous. It can encompass the following: complex forms of plasticity, involving distinct presynaptic and postsynaptic mechanisms (long-term potentiation and depression), soma polarization, dendrites, and synaptic terminals, axonal growth, network effects (amplifications and oscillations), and functions of interneurons, endothelial cells, and glia (13). Given the pathophysiological complexity of PD and the variability of its symptoms, multiple brain regions can modulate motor recovery, and consequently, the methods of applying tDCS can be diverging.
Previous reviews investigated tDCS and associated therapies (14–19), but tDCS alone is still a challenge to determine its clinical effect on PD (15). Thus, this systematic review and meta-analysis investigated the use of tDCS on PD based on the PICOS model: population (P): adult patients with PD; intervention (I): tDCS alone in different brain areas and number of stimulated nominal targets; comparison (C): control condition, placebo or sham; outcomes (O): PD motor symptoms; types of studies (S): clinical trials randomized or not with crossover or parallel design and open-label studies.
Methods
Protocol and Registration
A systematic review with meta-analysis and meta-regression was performed according to the Cochrane group (20), including review mechanisms, inclusion or exclusion criteria, search and selection of articles, analysis of the methodological quality of included studies, data extraction, and meta-analysis of results. The Preferred Reporting Items for Systematic Reviews and Meta-Analyses (PRISMA) guidelines were adopted (21). The selection of studies was performed by two independent reviewers (PCAO and TABA) according to the previously structured eligibility criteria. Disagreements between reviewers were resolved by a third reviewer (DGSM). The current review protocol was registered in the International Prospective Register of Systematic Reviews – PROSPERO–(https://www.crd.york.ac.uk/prospero/) under the publicly available registry number CRD42020188010 (https://www.crd.york.ac.uk/prospero/display_record.php?ID=CRD42020188010).
Search Strategy
The following databases were used for this review's literature survey: Cochrane Library, EMBASE, PubMed/MEDLINE, Scopus, and Web of science, and considered the literature until February 2021. The terms MeSh and operators Booleans were as follows: “Parkinson's disease” OR “Parkinson's disease” AND “transcranial direct current stimulation” OR “tDCS” OR “transcranial electrical stimulation” OR “non-invasive brain stimulation” OR “neuromodulation.” In addition, the reference lists of selected articles and literature reviews on the subject were checked to retrieve articles that were not covered by the database searches.
Eligibility Criteria
The search was carried out for full text articles, peer-reviewed, published in scientific journals without language restriction. However, only studies in English were found. To be included, studies should (a) include adults (over 18 years of age) with a clinical diagnosis guided by the Movement Disorder Society diagnostic criteria for PD (5), all types and levels of severity or by a clinical definition; (b) apply a-tDCS or c-tDCS; (c) report motor outcome data only from individuals with PD; (d) report data on motor outcomes only from the intervention with tDCS alone (for studies involving multiple interventions); (e) provide quantitative data for at least one of the outcome measures (in the manuscript or upon request); (f) have randomized and non-randomized clinical trials with parallel, crossover, or open-label design; and (g) have a sham or control condition. Studies involving research on animals, in vitro or computational models, were excluded. The agreement between reviewers for the screening of studies was analyzed using the Kappa (K) statistic, and the results revealed an “excellent” agreement (K = 0.969; p < 0.0001). The percentage of agreement between the reviewers was 99.9%, and the third reviewer's tie was 0.1%.
Study Quality Assessment
The evaluation of the internal validity and presentation of necessary statistical information of the studies was performed by two independent reviewers (PCAO and TABA), who used the classification scale of the Physiotherapy Evidence Database (PEDro) (22). The PEDro scale consists of 11 items that assess the followings: (1) eligibility criteria, (2) randomness of groups, (3) secret allocation, (4) homogeneity between groups, (5) blinding of participants, (6) blinding of therapists, (7) evaluator blinding, (8) key outcome in more than 85% of subjects, (9) intention-to-treat analysis, (10) statistical comparison between groups, and (11) precision measure and variability measures. The PEDro scale is one of the most used instruments in rehabilitation to assess the methodological quality of clinical trials (23, 24). Thus, it is a measure with sufficient validity to be used in systematic reviews of clinical trials and clinical practice guidelines (22). The classification of the PEDro score was as follows: scores from 0 to 4 = low quality; 4 to 5 = acceptable quality; 6 to 8 = good quality, and 9 to 10 = excellent quality (25).
The risk of bias was evaluated using the Cochrane risk of bias assessment (26), which assesses the followings: (a) random sequence generation, (b) allocation concealment, (c) blinding of participants and personnel, (d) blinding of outcome assessment, (e) incomplete outcome data, (f) selective reporting, and (g) other biases. Each item was classified as “low risk of bias” (“+”), “high risk of bias” (“–”) or “uncertain risk of bias” (“?”). Disagreements were resolved by a third reviewer (DGSM).
Data Extraction
Data extraction included sample size (number of individuals involved), participant characteristics [age, gender, time since PD diagnosis, Unified Parkinson's Disease Rating Scale (UPDRS) at baseline, medication, most affected hemibody, stage Hoehn and Yahr (HY)], intervention protocol (number of sessions, location of electrodes, anodic or cathodic, intensity, density, and duration of stimulation), and outcome measures (gait, motor function, motor aspects of daily life, dyskinesia, motor fluctuations, bradykinesia, manual dexterity, upper limb function, balance, postural stability, and freezing of gait) from all included studies. Missing article data were requested by email, and those who did not respond after three attempts or did not provide data for any reason were excluded from the meta-analysis. Thus, we excluded 15 articles from the quantitative synthesis, 11 for lack of response (27–37) and 4 for not having or not providing the data (38–41).
Quantitative Analysis
Quantitative synthesis was performed by combining individual studies into meta-analyses. We performed analyses comparing the effect of tDCS alone on motor symptoms according to the nominal stimulated target and compared the effect on single or multiple targets. To estimate the effect, we used continuous post-intervention mean and standard deviation data. We calculated the mean difference (MD) or the standardized mean difference (SMD), if the studies assessed the same outcome using different scales, confidence interval (CI) of 95% for each comparison, weighted by the inverse variance method using an effects model random or fixed-effects model, when applicable. Heterogeneity was assessed using chi-square (p < 0.1 = statistically significant), I2 (I2 > 75% = significant) and visual inspection of forest plots. If considerable heterogeneity was identified (chi-square p < 0.10; I2 > 75%), only a qualitative synthesis would be presented. Review Manager v.5.3 software (Copenhagen: Nordic Cochrane Center) was used for all data analysis, except for the meta-regression, performed in Python. The univariate meta-regression model used a sensitivity analysis to investigate possible effect moderators related to treatment characteristics (number of sessions and cumulative time). One predictor variable was analyzed at a time, and values of p < 0.05 were considered significant.
Results
Overview
This review comprises the range from 1984 to February 2021. The PRISMA flow diagram summarizes steps in the study identification procedures (Figure 1). The literature search identified 6,386 studies, and Mendeley software excluded 146 duplicates. No study was included based on verifying the reference lists of selected articles or literature reviews on the subject. Forty studies were eligible for full-text reading after evaluating titles and abstracts. The two most frequent causes of exclusion were an absence of a comparator and tDCS as a combined therapy. Another four studies were excluded after the analysis of abstracts. Studies that investigated non-motor outcomes after tDCS were also checked for the existence of motor outcomes for inclusion in the meta-analytic analysis. Finally, 25 studies involving 405 participants met our criteria and were included in the qualitative synthesis. Of those, 10 were included in the meta-analysis, covering a total of 236 participants.
Characteristics of Included Studies
Table 1 summarizes information of included studies, which investigated the effect of tDCS alone on the motor symptoms of PD. According to this table, 20 (80%) studies were randomized (27–29, 32, 34, 36–40, 42–51), four (16%) did not mention this information (31, 33, 35, 41) and one (4%) used pseudo-randomization (30). Twenty studies (80%) had a crossover design (28–37, 40, 41, 43, 44, 46–51) and five (20%) parallel (27, 38, 39, 42, 45). One (4%) study did not contain information about blinding (39), three (12%) trials had single-blind experiments (33, 44, 50), 20 (80%) double-blind experiments (27–32, 34, 36–38, 40–43, 45–51) and one (4%) double blind in only one of the experiments (35). Regarding the comparator group, 24 studies (96%) had a sham group (27–38, 40–51) and one (4%) had a control group, which did not undergo any type of therapy (39).
Characteristics of Participants
In total, 25 studies included 405 individuals with PD, and the mean sample size was 17.64 ± 7.40 (ranging from 7 to 26 participants), aged between 58 and 74 years. HY obtained a minimum score of 1.3 and a maximum of 2.8, indicating early to almost moderate stages of PD. The UPDRS II achieved a minimum score of 1.1 and a maximum of 11.6, a UPDRS III minimum of 13 and a maximum of 39.7, and a minimum of 16 and a maximum of 74.2 on UPDRS's total score. The duration of PD had a minimum of 4.3 and a maximum of 12.3 years whereas the dose of the medication had a minimum of 292.8 mg and a maximum of 1287.7 mg. Twenty-two (88%) studies performed the experiment in the ON state of the medication (27–35, 37–39, 41, 43–51), two (8%) in the OFF state (36, 40) and one (4%) in both states (42). Details of the participants of each study are shown in Table 2.
tDCS Protocols
Three (12%) studies stimulated multiple targets, and 22 studies (88%) stimulated single nominal target with dorsolateral prefrontal cortex (DLPFC) and primary motor cortex (M1) as the most common montages (Figure 2). In addition, most studies performed single tDCS session (66.7%), and others used 2 (11.1%), 4 (3.7%), 5 (11.1%), 8 (3.7%), and 10 sessions (3.7%). Twenty-two trials (88%) applied anodal tDCS (27, 28, 31–34, 36–51), whereas three (12%) performed anodal tDCS and cathodal tDCS (29, 30, 35). The minimum current intensity was 1 mA, the maximum was 4 mA, and the mean duration time of stimulation per session was 19.28 ± 4.47 min (minimum of 7 min and maximum of 30 min). Finally, 18 (72%) studies performed pre- and postintervention assessments (27–31, 33–35, 40, 41, 43–46, 48–51), whereas seven (28%) also performed follow-up evaluations (range from 15 min to 3 months) (32, 36–39, 42, 47).
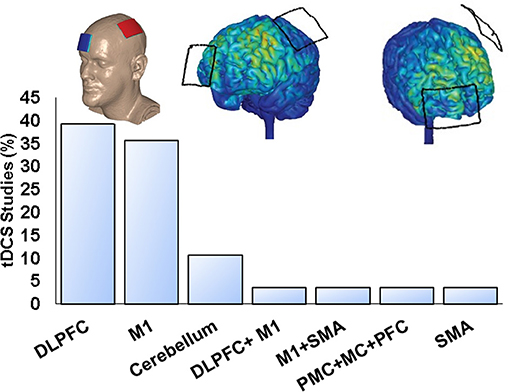
Figure 2. Quantity of tDCS studies on PD using single nominal targets, and example of M1 anodal electrode on C3.
Motor Outcome Result Measures
Gait was analyzed in 17 (68%) of those studies (31–34, 37, 38, 40–46, 48–51) and which was evaluated by the timed up and go (TUG) test, 10-m walk test, video analysis and pressure platform, six-min walk test, stand–walk–sit test, 25-foot walk test, and Dynamic Gait Index. Thirteen studies (52%) also investigated the effect of tDCS alone on UPDRS scores (28–30, 35–40, 42, 45, 47, 50), on bradykinesia, manual dexterity, and upper limb function (27, 29, 30, 35, 38, 42). Balance or postural stability was analyzed in five (20%) studies (28, 41, 48, 49, 51) and freezing of gait (FOG) in other three (12%) studies (37, 40, 47).
Quality of Included Studies
Internal validity and necessary statistical information were evaluated using the PEDro scale and obtained a mean score of 8.28 ± 1.24, which reveals a good methodological quality of the studies (25). Details of the scores for each study are shown in Table 3.
The results of risk of bias indicate a low or unclear risk for most studies except for allocation concealment that was considered high. Details of risk of bias of each study are shown in Figure 3.
Meta-Analysis Results
Single Nominal Targets on Motor Symptoms
UPDRS III–Motor Aspects
This analysis included one study (47) and two experiments divided by nominal target, namely M1 and cerebellum. A total of nine participants were involved and randomly assigned to one stimulation protocol at once. There was no significant effect of tDCS on motor aspects measured by the UPDRS III. Analyzing the combined effect of these areas (MD = −0.98%, 95% CI = −10.03 to 8.07, p = 0.83, I2 = 0%, without significant heterogeneity and fixed-effects model), there was no significant effect about isolated areas (Figure 4A).
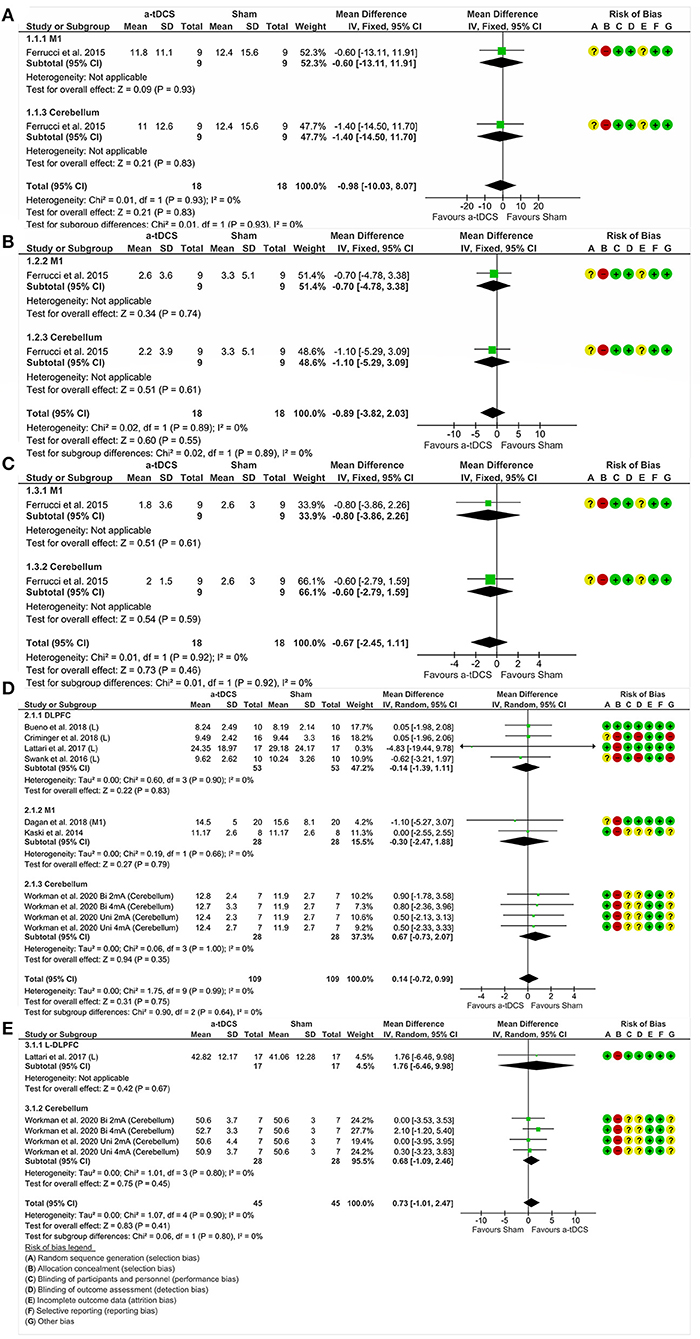
Figure 4. Forest plot showing mean difference from the comparison between single targets in motor function—UPDRS III (A) and dyskinesias—UPDRS IV (B) and motor fluctuations—UPDRS IV (C) and the gait—TUG (D) and balance—BBS (E). Risk of bias was deemed as “low risk of bias” (“+”), “high risk of bias” (“–”), or “unclear risk of bias” (“?”).
UPDRS IV—Dyskinesias
One study (47) enrolled 09 participants and conducted two experiments in which nominal targets, M1 and cerebellum, were stimulated. There was no significant effect between tDCS and dyskinesias assessed by the UPDRS IV. Furthermore, analyzing the combined effect of these areas (MD = −0.89%, CI 95% = −3.82 to 2.03, p = 0.55, I2 = 0%, without significant heterogeneity and fixed-effects model), there was also no significant effect in the analysis of isolated areas (Figure 4B).
UPDRS IV—Motor Fluctuations
Two experiments divided by nominal targets included M1 (47) and cerebellum (47). A total of 09 participants were involved and randomly assigned to M1, cerebellum or sham stimulation. There was no significant effect of tDCS in relation to motor fluctuations, measured by the UPDRS IV. Analyzing the combined effect of these areas (MD = −0.67%, CI 95% = −2.45 to 1.11, p = 0.46, I2 = 0%, without significant heterogeneity and fixed-effects model), there was also no significant effect in the analysis of isolated areas (Figure 4C).
TUG—Gait
We analyzed 98 participants distributed in seven studies, grouped by areas of stimulation, namely DLPFC (43, 44, 49, 50), M1 (46, 48) and cerebellum (51). There was no significant effect of tDCS in relation to gait, measured by TUG. Analyzing the combined effect of these areas (MD = 0.14%, CI 95% = −0.72 to 0.99, p = 0.75, I2 = 0%, without significant heterogeneity and random effects model), there was also no significant effect in the analysis of isolated areas (Figure 4D).
Berg Balance Scale—Balance
We compared 24 participants and protocol stimulations distributed in two studies, divided according to the areas of DLPFC (49) and cerebellum (51). There was no significant effect of tDCS related to balance, measured by the BBS. Analyzing the combined effect of these areas (MD = 0.73%, CI 95% = −1.01 to 2.47, p = 0.41, I2 = 0%, without significant heterogeneity and random effects model), there was no significant effect in the analysis of isolated areas (Figure 4E).
Single and Multiple Nominal Targets on Motor Symptoms
UPDRS III—Motor Aspects
We analyzed three studies and four experiments, with 51 participants, grouped according to the number of stimulation areas: single target (47) and multiple targets (42, 45). There was no significant effect of tDCS in relation to the motor aspects assessed by the UPDRS III. Analyzing the combined effect of these areas (MD = 2.05%, CI 95% = −1.96 to 6.06, p = 0.32, I2 = 0%, no heterogeneity and random effects model), there was also no significant effect in the analysis of isolated areas (Figure 5A).
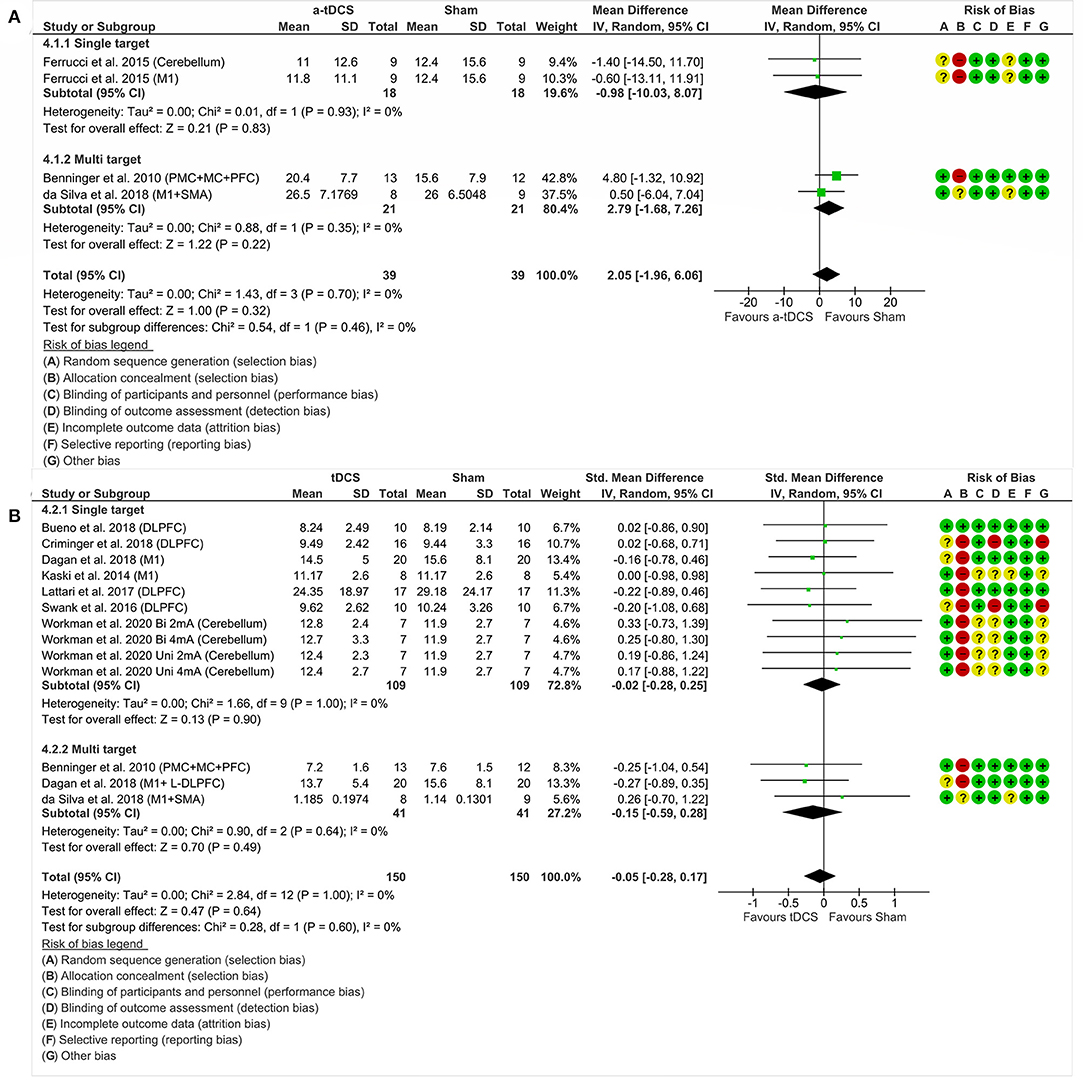
Figure 5. Forest plot showing mean difference from the comparison between single targets vs. multitarget in motor aspects—UPDRS III (A) and standardized mean difference in the gait (B). Risk of bias was deemed as “low risk of bias” (“+”), “high risk of bias” (“–”), or “unclear risk of bias” (“?”).
Gait
In this analysis, we included 10 studies, with 98 participants, grouped by the amount of stimulation areas: single target (43, 44, 46, 48–51) and multiple targets (42, 45, 46). The investigation did not show a significant effect of tDCS, regardless of the number of nominal targets stimulated, in relation to gait. Analyzing the combined effect of these areas (SMD = −0.05%, 95% CI = −0.28 to 0.17, p = 0.64, I2 = 0%, without significant heterogeneity and random effects model), there was also no significant effect in the analysis of isolated areas (Figure 5B).
Meta-Regression
Simple univariate meta-regression analysis was performed by a blinded investigator (ACRN) using Python “Pymare” library to investigate the association between effect size and treatment dosage considered as the number of sessions and cumulative time. Analysis revealed that the number of sessions was not significantly associated with effect size (estimate = −1.7, SE = 1.51, z-score = −1.18, p = 0.2, CI = −4.75 to 1.17). The analysis also revealed that cumulative time was also not significantly associated with effect size (estimate = −0.07, SE = 0.07, z-score = −0.99, p = 0.31, CI = −0.21 to 0.07).
Discussion
This systematic review with meta-analysis and meta-regression includes 25 studies with 405 participants and investigated the effect of tDCS on the motor symptoms of PD. Our results demonstrated that there was no significant effect of tDCS on short-term motor symptoms of PD, regardless of brain area, number of stimulated nominal targets, or treatment dosage. The regions most covered by the included studies were DLPFC and M1.
The DLPFC is a brain region commonly studied in tDCS research to observe its effect on non-motor symptoms of PD, but it has also been widely investigated in motor symptoms. The justification includes several explanations: (a) the non-motor symptoms influence the motor symptoms because cognitive functions are needed to perform motor tasks and are partly modulated by the DLPFC (31). An example of this relationship is the execution of the gait, where the individual needs the ability to perform a dual task (43); (b) DLPFC appears to interfere with balance, through the attribution of the prefrontal cortex to spatial orientation (52) in addition to its activation during gait in several challenging conditions (53, 54). Thus, the hypothesis suggests that modulating DLPFC can improve visuospatial processing that improves the balance of individuals with PD (49). However, the literature shows divergent results related to the stimulation of this area to improve motor functions. Previous research (30, 38, 39, 43, 44, 50) found no significant effect of tDCS on DLPFC for motor function, simple reaction time, aspects of isolated and dual task gait, quality of life, or motor aspects of daily life. In contrast, other studies (31, 33, 34, 49) found a beneficial effect for walking alone and with dual task, FOG, functional mobility, or balance. Finally, responses in DLPFC can activate distinct networks of motor areas, such as M1, supplementary motor area, and premotor area, which exert direct control over motor aspects (55, 56). However, the possibility of cortical functioning through a matrix cannot be excluded, as in pain processing (12, 57).
In turn, the M1 area is also a widely investigated target for treatment of motor symptoms of PD, due to its primordial role in motor control and learning (58). In summary, the disturbance in the functioning of the basal ganglia causes cortical dysfunction and promotes the motor symptoms of PD. Thus, the hypothesis is that the modulation of cortical areas can drive changes in the cortical–subcortical pathway, positively influencing the basal ganglia, to correct such dysfunction and reduce symptoms (30). However, the literature about tDCS in M1 shows divergences. According to previous studies (29, 30, 35–37, 41, 47), tDCS in M1 showed a significant effect on hand motor performance, dyskinesia, gait, FOG, motor function, and simple reaction time, respectively. However, other studies (46, 48) did not obtain a significant effect on gait and balance.
It is important to note that despite the inaccuracies in the clinical effect, there is evidence that tDCS stimulates both the target area and beyond (12). Neurophysiological mechanisms may include changes in neuronal excitability, plasticity, neuronal oscillations, and connectivity (12). Numerous studies using electroencephalography (59–62), functional magnetic resonance (57, 63–65), combination of transcranial magnetic stimulation with electroencephalography (66, 67), and functional near-infrared spectroscopy (68) have shown brain changes after tDCS in M1 with modulation of this neural network.
According to the meta-analysis, it is still not possible to determine the number of nominal targets to be stimulated in tDCS protocols to reduce motor symptoms in PD. Considering pathophysiological mechanisms, chronic evolution, multisystem repercussions, and varied symptoms, it is necessary to note the importance of functional rehabilitation combined with additional approaches. The potential of tDCS at disease onset is also relevant as most motor treatment is provided in the early phase (during the 1st week and month) (69, 70). Here, we provide some evidences that tDCS can improve motor function in early-stage patients to some extent. In previous studies, early stimulation of tDCS reduced cadence (45), upper limb bradykinesia (29), FOG (46), and improved levodopa-induced dyskinesia (47).
There is little evidence regarding the mechanisms of action of tDCS in the pathophysiology of PD. However, our hypothesis is that multiple sessions of tDCS associated with rehabilitation training can activate brain regions by the task-related activity and, therefore, make them more sensitive to modulation by tDCS (13). Different hypotheses can explain our results: a) few studies involving PD, neuromodulation, and motor symptoms aimed to assess the isolated effect of tDCS. Furthermore, studies that proposed to investigate such aspects, an even smaller number presented essential numerical data for a meta-analytic evaluation; b) our meta-regression showed that the number and cumulative time of sessions were not associated with tDCS effect size, which may suggest insufficient corticospinal changes to increase motor performance and such insufficiency may be associated with other factors, including long interval of hours between applications and longer application time, which can inhibit overstimulation through neuronal counter-regulation, among others (71); (c) the sample size of the included trials may have been limited to provide an adequate effect size; and d) there is a lack of evidence on the non-motor aspects of PD, which may influence the effectiveness of tDCS on motor outcomes. Thus, it is likely that cognitive processing is supported by several brain regions and neural networks, which makes it challenging to identify specific nominal targets to stimulate. Furthermore, our results cannot be applicable to individuals in the OFF state of medication, as most studies (88%) performed their research only in the ON state.
This systematic review with meta-analysis and meta-regression aimed to fill the gaps in the literature related to the effect of tDCS on the motor symptoms of individuals with PD. Based on the evidence from previous meta-analyses, our study (a) provided a direct comparison between the effect sizes of studies that used motor and non-motor cortical targets, (b) compared the effects of single montages target vs. multitarget in motor function, (c) included a larger set of important studies (27, 28, 32, 34, 36, 40, 51) that bring relevant approaches to the field under investigation and that were published after previous reviews were carried out, and (d) analyzed the association of certain therapeutic variables with tDCS effect size. The recent evidence-based guidelines for neuromodulation target sites for the treatment of motor function in PD concludes that anodal tDCS over motor, premotor, and supplementary motor area is likely to be effective (level C), whereas on the prefrontal cortex, there is possibly no efficacy (level B) (15). Thus, considering the gaps that still exist in the literature and seeking clarification in future recommendations, further studies should include secret allocation, adequate blinding, homogeneous comparison group, sufficient sample size, application of tDCS in single and multiple brain regions, shorter interval of hours between sessions, and evaluation of the long-term effect on simple and complex motor tasks. Finally, future studies could go beyond the target area and investigate patterns of cortical activation at baseline and during treatment to infer possible predictors of response to therapy. A deeper look at the neurophysiological correlates in patients with PD is needed, particularly to provide neurophysiological evidence that cholinergic dysfunction may be an important and early contributor to motor and cognitive dysfunction in PD.
Conclusion
In summary, this systematic review with meta-analysis and meta-regression found no significant short-term effect of tDCS alone on motor function, balance, gait, dyskinesia, and motor fluctuations, regardless of brain area or number of stimulated nominal targets in patients with PD. We also found no relationship between the effect of tDCS alone and the number of sessions or cumulative treatment time.
Data Availability Statement
The data supporting the conclusions of this review article will be made available by the corresponding author on request to qualified researcher.
Ethics Statement
Ethical review and approval was not required for the study on human participants in accordance with the local legislation and institutional requirements. Written informed consent for participation was not required for this study in accordance with the national legislation and the institutional requirements.
Author Contributions
PO and TA contributed with preliminary databases' searches, selection of studies, screening of research results and eligibility criteria, data extraction, risk of bias, quality assessment, data analysis, and manuscript writing. DM supervised the systematic review of studies, reviewed, and edited the manuscript. AR contributed to the meta-regression analysis. MB, SA, AO, HS, RP, and EM reviewed and edited the manuscript. All authors listed above have made a substantial, direct and intellectual contribution to this work, contributed to manuscript revision, read, and approved the submitted version.
Funding
This study had neither direct nor specific funding, but was financed in part by: Live Without Limits Brazilian Programme for Disabilities/Ministry of Health (Brazil)—Portaria N 2.164/GM/MS: funds the operation and pays professionals' salaries of the Rehabilitation Center/Anita Garibaldi Center for Education and Health/Santos Dumont Institute. Coordenação de Aperfeiçoamento de Pessoal de Nível Superior—Brazil (CAPES)/Ministry of Education (Brazil)—Finance Code 001: funds postgraduate scholarships in neuromodulation at Edmond and Lily Safra International Institute of Neuroscience/Santos Dumont Institute. AHO financial support from CNPq (487361/2013-0 and 479000/2012-3), FAPESP (13/10187-0 and 14/101347). EM and AHO financial support from FAPESP/CEPID/BRAINN (13/07559-3). Santos Dumont Institute—Management Contract by Ministry of Education (Brazil): funds the operation and pays Researcher's salaries of Santos Dumont Institute.
Conflict of Interest
The City University of New York holds patents on brain stimulation with MB as inventor. MB has equity in Soterix Medical Inc. MB consults, received grants, assigned inventions, and/or serves on the SAB of SafeToddles, Boston Scientific, GlaxoSmithKline, Biovisics, Mecta, Lumenis, Halo Neuroscience, Google-X, i-Lumen, Humm, Allergan (Abbvie), Apple. HS served as a consultant for Abbott, LivaNova, and Boston Scientific in the previous 5 years.
The remaining authors declare that the research was conducted in the absence of any commercial or financial relationships that could be a potential conflict of interest.
Publisher's Note
All claims expressed in this article are solely those of the authors and do not necessarily represent those of their affiliated organizations, or those of the publisher, the editors and the reviewers. Any product that may be evaluated in this article, or claim that may be made by its manufacturer, is not guaranteed or endorsed by the publisher.
Acknowledgments
We are grateful to the authors of original studies who provided the data, making this meta-analysis possible.
Abbreviations
PD, Parkinson's disease; tDCS, transcranial direct current stimulation; a-tDCS, anodic transcranial direct current stimulation; c-tDCS, cathodic transcranial direct current stimulation; DALY, Disability-adjusted life year; PRISMA, Preferred Reporting Items for Systematic Reviews and Meta-Analyses; PEDro, Physiotherapy Evidence Database; UPDRS, Unified Parkinson's Disease Rating Scale; HY, Hoehn and Yahr; FOG, Freezing of gait; MD, Mean difference; SMD, Standardized mean difference; CI, Confidence interval; DLPFC, Dorsolateral prefrontal cortex; M1, Primary motor cortex; TUG, Timed up and go; BBS, Berg Balance Scale.
References
1. Rousseaux MWC, Shulman JM, Jankovic J. Progress toward an integrated understanding of Parkinson's disease. F1000Research. (2017) 6:1121. doi: 10.12688/f1000research.11820.1
2. Feigin VL, Vos T. Global, regional, and national burden of neurological disorders during 1990-2016: a systematic analysis for the Global Burden of Disease Study 2016. Lancet Neurol. (2019) 18:459–80. doi: 10.1016/S1474-4422(18)30499-X
3. Dorsey ER, Elbaz A, Nichols E, Abd-Allah F, Abdelalim A, Adsuar JC, et al. Global, regional, and national burden of Parkinson's disease, 1990-2016: a systematic analysis for the Global Burden of Disease Study 2016. Lancet Neurol. (2018) 17:939–53. doi: 10.1016/S1474-4422(18)30295-3
4. Berg D, Adler CH, Bloem BR, Chan P, Gasser T, Goetz CG, et al. Movement disorder society criteria for clinically established early Parkinson's disease. Move Disord. (2018) 33:1643–6. doi: 10.1002/mds.27431
5. Postuma RB, Berg D, Stern M, Poewe W, Olanow CW, Oertel W, et al. MDS clinical diagnostic criteria for Parkinson's disease. Move Disord. (2015) 30:1591–601. doi: 10.1002/mds.26424
6. DeMaagd G, Philip A. Parkinson's disease and its management: part 1: disease entity, risk factors, pathophysiology, clinical presentation, and diagnosis. P T. (2015) 40:504–32.
7. Hallett M, Hess C. The phenomenology of Parkinson's disease. Semin Neurol. (2017) 37:109–17. doi: 10.1055/s-0037-1601869
8. Munhoz RP, Picillo M, Fox SH, Bruno V, Panisset M, Honey CR, et al. Eligibility criteria for deep brain stimulation in Parkinson's disease, tremor, and dystonia. Canad J Neurol Sci. (2016) 43:462–71. doi: 10.1017/cjn.2016.35
9. Capato TT, da C. Versão em português da Diretriz Européia de Fisioterapia para a Doença de Parkinson: informações para pessoas com a doença de Parkinson. 1st ed. Vol. 1. São Paulo: Editora e Eventos Omnifarma (2016).
10. Bikson M, Grossman P, Thomas C, Zannou AL, Jiang J, Adnan T, et al. Safety of transcranial direct current stimulation: evidence based update 2016. Brain Stimulation. (2016) 9:641–61. doi: 10.1016/j.brs.2016.06.004
11. Woods AJ, Antal A, Bikson M, Boggio PS, Brunoni AR, Celnik P, et al. A technical guide to tDCS, and related non-invasive brain stimulation tools. Clin Neurophysiol. (2016) 127:1031–48. doi: 10.1016/j.clinph.2015.11.012
12. Morya E, Monte-Silva K, Bikson M, Esmaeilpour Z, Biazoli CE Jr, Fonseca A, et al. Beyond the target area: an integrative view of tDCS-induced motor cortex modulation in patients and athletes. J NeuroEng Rehabilit. (2019) 16:581. doi: 10.1186/s12984-019-0581-1
13. Jackson MP, Rahman A, Lafon B, Kronberg G, Ling D, Parra LC, et al. Animal models of transcranial direct current stimulation: Methods and mechanisms. Clin Neurophysiol. (2016) 127:3425–54. doi: 10.1016/j.clinph.2016.08.016
14. Elsner B, Kugler J, Pohl M, Mehrholz J. Transcranial direct current stimulation (tDCS) for idiopathic Parkinson's disease. Cochrane Database Syst Rev. (2016) 7:CD010916. doi: 10.1002/14651858.CD010916.pub2
15. Fregni F, El-Hagrassy MM, Pacheco-Barrios K, Carvalho S, Leite J, Simis M, et al. Evidence-based guidelines and secondary meta-analysis for the use of transcranial direct current stimulation in neurological and psychiatric disorders. Int J Neuropsychopharmacol. (2020) 24:256–313. doi: 10.1093/ijnp/pyaa051
16. Goodwill AM, Lum JAG, Hendy AM, Muthalib M, Johnson L, Albein-Urios N, et al. Using non-invasive transcranial stimulation to improve motor and cognitive function in Parkinson's disease: a systematic review and meta-analysis. Sci Rep. (2017) 7:13260. doi: 10.1038/s41598-017-13260-z
17. Lee HK, Ahn SJ, Shin YM, Kang N, Cauraugh JH. Does transcranial direct current stimulation improve functional locomotion in people with Parkinson's disease? A systematic review and meta-analysis. J NeuroEng Rehabilitation. (2019) 16:562. doi: 10.1186/s12984-019-0562-4
18. Orrù G, Baroni M, Cesari V, Conversano C, Hitchcott PK, Gemignani A. The effect of single and repeated tDCS sessions on motor symptoms in Parkinson's disease: a systematic review. Arch Italiennes Biol. (2019) 2:89–101. doi: 10.12871/00039829201925
19. Simpson MW, Mak M. The effect of transcranial direct current stimulation on upper limb motor performance in Parkinson's disease: a systematic review. J Neurol. (2019) 267:3479–88. doi: 10.1007/s00415-019-09385-y
21. Liberati A, Altman DG, Tetzlaff J, Mulrow C, Gotzsche PC, Ioannidis JPA, et al. The PRISMA statement for reporting systematic reviews and meta-analyses of studies that evaluate healthcare interventions: explanation and elaboration. BMJ. (2009) 339:b2700. doi: 10.1136/bmj.b2700
22. Maher CG, Sherrington C, Herbert RD, Moseley AM, Elkins M. Reliability of the PEDro scale for rating quality of randomized controlled trials. Phys Ther. (2003) 83:713–21. doi: 10.1093/ptj/83.8.713
23. Sampaio R, Mancini M. Estudos de revisão sistemática: um guia para síntese criteriosa da evidência científica. Revista Brasileira de Fisioterapia. (2007) 11:83–9. doi: 10.1590/S1413-35552007000100013
24. de Morton NA. The PEDro scale is a valid measure of the methodological quality of clinical trials: a demographic study. Austr J Physiother. (2009) 55:129–33. doi: 10.1016/S0004-9514(09)70043-1
25. Cashin AG, Mcauley JH. Clinimetrics: physiotherapy evidence database (PEDro) scale. J Physiother. (2020) 66:59. doi: 10.1016/j.jphys.2019.08.005
26. Lundh A, Gøtzsche PC. Recommendations by cochrane review groups for assessment of the risk of bias in studies. BMC Med Res Methodol. (2008) 8:22. doi: 10.1186/1471-2288-8-22
27. Lima de Albuquerque L, Pantovic M, Clingo M, Fischer K, Jalene S, Landers M, et al. An acute application of cerebellar transcranial direct current stimulation does not improve motor performance in Parkinson's disease. Brain Sci. (2020) 10:735. doi: 10.3390/brainsci10100735
28. Beretta VS, Vitório R, Nóbrega-Sousa P, Conceição NR, Orcioli-Silva D, Pereira MP, et al. Effect of different intensities of transcranial direct current stimulation on postural response to external perturbation in patients with Parkinson's disease. Neurorehabilitation Neural Repair. (2020) 34:1009–19. doi: 10.1177/1545968320962513
29. Cosentino G, Valentino F, Todisco M, Alfonsi E, Davì R, Savettieri G, et al. Effects of more-affected vs. less-affected motor cortex tDCS in Parkinson's disease. Front Human Neurosci. (2017) 11:309. doi: 10.3389/fnhum.2017.00309
30. Fregni F, Boggio PS, Santos MC, Lima M, Vieira AL, Rigonatti SP, et al. Noninvasive cortical stimulation with transcranial direct current stimulation in Parkinson's disease. Move Disord. (2006) 21:1693–702. doi: 10.1002/mds.21012
31. Manenti R, Brambilla M, Rosini S, Orizio I, Ferrari C, Borroni B, et al. Time up and go task performance improves after transcranial direct current stimulation in patient affected by Parkinson's disease. Neurosci Lett. (2014) 580:74–7. doi: 10.1016/j.neulet.2014.07.052
32. Mishra R kinker, Thrasher AT. Transcranial direct current stimulation of dorsolateral prefrontal cortex improves dual-task gait performance in patients with Parkinson's disease: A double blind, sham-controlled study. Gait Posture. (2021) 84:11–6. doi: 10.1016/j.gaitpost.2020.11.012
33. Putzolu M, Pelosin E, Ogliastro C, Lagravinese G, Bonassi G, Ravaschio A, et al. Anodal tDCS over prefrontal cortex improves dual-task walking in Parkinsonian patients with freezing. Move Disord. (2018) 33:1972–3. doi: 10.1002/mds.27533
34. Putzolu M, Ogliastro C, Lagravinese G, Bonassi G, Trompetto C, Marchese R, et al. Investigating the effects of transcranial direct current stimulation on obstacle negotiation performance in Parkinson disease with freezing of gait: A pilot study. Brain Stimulation. (2019) 12:1583–5. doi: 10.1016/j.brs.2019.07.006
35. Salimpour Y, Mari ZK, Shadmehr R. Altering effort costs in Parkinson's disease with noninvasive cortical stimulation. J Neurosci. (2015) 35:12287–302. doi: 10.1523/JNEUROSCI.1827-15.2015
36. Schoellmann A, Scholten M, Wasserka B, Govindan RB, Krüger R, Gharabaghi A, et al. Anodal tDCS modulates cortical activity and synchronization in Parkinson's disease depending on motor processing. NeuroImage: Clinical. (2019) 22:101689. doi: 10.1016/j.nicl.2019.101689
37. Valentino F, Cosentino G, Brighina F, Pozzi NG, Sandrini G, Fierro B, et al. Transcranial direct current stimulation for treatment of freezing of gait: A cross-over study. Move Disord. (2014) 29:1064–9. doi: 10.1002/mds.25897
38. Doruk D, Gray Z, Bravo GL, Pascual-Leone A, Fregni F. Effects of tDCS on executive function in Parkinson's disease. Neurosci Lett. (2014) 582:27–31. doi: 10.1016/j.neulet.2014.08.043
39. Lawrence BJ, Gasson N, Johnson AR, Booth L, Loftus AM. Cognitive training and transcranial direct current stimulation for mild cognitive impairment in Parkinson's disease: a randomized controlled trial. Parkinson's Dis. (2018) 2018:1–12. doi: 10.1155/2018/4318475
40. Lu C, Huffmaster SLA, Tuite PJ, Mackinnon CD. The effects of anodal tDCS over the supplementary motor area on gait initiation in Parkinson's disease with freezing of gait: a pilot study. J Neurol. (2018) 265:2023–32. doi: 10.1007/s00415-018-8953-1
41. Verheyden G, Purdey J, Burnett M, Cole J, Ashburn A. Immediate effect of transcranial direct current stimulation on postural stability and functional mobility in Parkinson's disease. Move Disord. (2013) 28:2040–1. doi: 10.1002/mds.25640
42. Benninger DH, Lomarev M, Lopez G, Wassermann EM, Li X, Considine E, et al. Transcranial direct current stimulation for the treatment of Parkinson's disease. J Neurol Neurosurg Psychiatry. (2010) 81:1105–11. doi: 10.1136/jnnp.2009.202556
43. Bueno MEB, Neto LIDN, Terra MB, Barboza NM, Okano AH, Smaili SM. Effectiveness of acute transcranial direct current stimulation on non-motor and motor symptoms in Parkinson's disease. Neurosci Lett. (2019) 696:46–51. doi: 10.1016/j.neulet.2018.12.017
44. Criminger C, Swank C, Almutairi S, Mehta J. Transcranial direct current stimulation plus concurrent activity may influence task prioritization during walking in people with Parkinson's disease - initial findings. J Parkinson Restless Legs Syndr. (2018) 8:25–32. doi: 10.2147/JPRLS.S161740
45. da Silva DCL, Lemos T, de Sá Ferreira A, Horsczaruk CHR, Pedron CA, de Carvalho Rodrigues E, et al. Effects of acute transcranial direct current stimulation on gait kinematics of individuals with Parkinson's disease. Topics Geriatric Rehabilitation. (2018) 34:262–8. doi: 10.1097/TGR.0000000000000203
46. Dagan M, Herman T, Harrison R, Zhou J, Giladi N, Ruffini G, et al. Multitarget transcranial direct current stimulation for freezing of gait in Parkinson's disease. Move Disord. (2018) 33:642–6. doi: 10.1002/mds.27300
47. Ferrucci R, Cortese F, Bianchi M, Pittera D, Turrone R, Bocci T, et al. Cerebellar and motor cortical transcranial stimulation decrease levodopa-induced dyskinesias in Parkinson's Disease. Cerebellum. (2015) 15:43–7. doi: 10.1007/s12311-015-0737-x
48. Kaski D, Dominguez R, Allum J, Islam A, Bronstein A. Combining physical training with transcranial direct current stimulation to improve gait in Parkinson's disease: a pilot randomized controlled study. Clin Rehabilitation. (2014) 28:1115–24. doi: 10.1177/0269215514534277
49. Lattari E, Costa SS, Campos C, Oliveira AJD, Machado S, Neto GAM. Can transcranial direct current stimulation on the dorsolateral prefrontal cortex improves balance and functional mobility in Parkinson's disease? Neurosci Lett. (2017) 636:165–9. doi: 10.1016/j.neulet.2016.11.019
50. Swank C, Mehta J, Criminger C. Transcranial direct current stimulation lessens dual task cost in people with Parkinson's disease. Neurosci Lett. (2016) 626:1–5. doi: 10.1016/j.neulet.2016.05.010
51. Workman CD, Fietsam AC, Uc EY, Rudroff T. Cerebellar transcranial direct current stimulation in people with Parkinson's disease: a pilot study. Brain Sci. (2020) 10:96. doi: 10.3390/brainsci10020096
52. Huang D, Mao Y, Chen P, Li L. Virtual reality training improves balance function. Neural Regen Res. (2014) 9:1628. doi: 10.4103/1673-5374.141795
53. Hamacher D, Herold F, Wiegel P, Hamacher D, Schega L. Brain activity during walking: A systematic review. Neurosci Biobehav Rev. (2015) 57:310–27. doi: 10.1016/j.neubiorev.2015.08.002
54. Maidan I, Nieuwhof F, Bernad-Elazari H, Reelick MF, Bloem BR, Giladi N, et al. The role of the frontal lobe in complex walking among patients with Parkinson's disease and healthy older adults. Neurorehabilitation Neural Repair. (2016) 30:963–71. doi: 10.1177/1545968316650426
55. Kim YK, Park E, Lee A, Im C-H, Kim Y-H. Changes in network connectivity during motor imagery and execution. PLoS ONE. (2018) 13:e0190715. doi: 10.1371/journal.pone.0190715
56. Stuart S, Alcock L, Rochester L, Vitorio R, Pantall A. Monitoring multiple cortical regions during walking in young and older adults: Dual-task response and comparison challenges. Int J Psychophysiol. (2019) 135:63–72. doi: 10.1016/j.ijpsycho.2018.11.006
57. Sankarasubramanian V, Cunningham DA, Potter-Baker KA, Beall EB, Roelle SM, Varnerin NM, et al. Transcranial direct current stimulation targeting primary motor versus dorsolateral prefrontal cortices: proof-of-concept study investigating functional connectivity of thalamocortical networks specific to sensory-affective information processing. Brain Connectivity. (2017) 7:182–96. doi: 10.1089/brain.2016.0440
58. Papale AE, Hooks BM. Circuit changes in motor cortex during motor skill learning. Neuroscience. (2018) 368:283–97. doi: 10.1016/j.neuroscience.2017.09.010
59. Polanía R, Nitsche MA, Paulus W. Modulating functional connectivity patterns and topological functional organization of the human brain with transcranial direct current stimulation. Human Brain Mapping. (2010) 32:1236–49. doi: 10.1002/hbm.21104
60. Hordacre B, Moezzi B, Ridding MC. Neuroplasticity and network connectivity of the motor cortex following stroke: A transcranial direct current stimulation study. Human Brain Mapping. (2018) 39:3326–39. doi: 10.1002/hbm.24079
61. Baxter BS, Edelman BJ, Sohrabpour A, He B. Anodal transcranial direct current stimulation increases bilateral directed brain connectivity during motor-imagery based brain-computer interface control. Front Neurosci. (2017) 11:691. doi: 10.3389/fnins.2017.00691
62. Gaxiola-Tirado JA, Rodríguez-Ugarte M, Iáñez E, Ortiz M, Gutiérrez D, Azorín JM. The effect of tDCS on EEG-based functional connectivity in gait motor imagery. Understanding Brain Funct Emotions. (2019) 2019:3–10. doi: 10.1007/978-3-030-19591-5_1
63. Lefebvre S, Dricot L, Laloux P, Desfontaines P, Evrard F, Peeters A, et al. Increased functional connectivity one week after motor learning and tDCS in stroke patients. Neuroscience. (2017) 340:424–35. doi: 10.1016/j.neuroscience.2016.10.066
64. Chen JL, Schlaug G. Erratum: Increased resting state connectivity between ipsilesional motor cortex and contralesional premotor cortex after transcranial direct current stimulation with physical therapy. Sci Rep. (2016) 6:24960. doi: 10.1038/srep24960
65. Sehm B, Kipping J, Schäfer A, Villringer A, Ragert P. A comparison between uni- and bilateral tDCS effects on functional connectivity of the human motor cortex. Front Human Neurosci. (2013) 7:183. doi: 10.3389/fnhum.2013.00183
66. Farzan F, Vernet M, Shafi MMD, Rotenberg A, Daskalakis ZJ, Pascual-Leone A. Characterizing and modulating brain circuitry through transcranial magnetic stimulation combined with electroencephalography. Front Neural Circuits. (2016) 10:73. doi: 10.3389/fncir.2016.00073
67. Hill AT, Fitzgerald PB, Hoy KE. Effects of anodal transcranial direct current stimulation on working memory: a systematic review and meta-analysis of findings from healthy and neuropsychiatric populations. Brain Stimulation. (2016) 9:197–208. doi: 10.1016/j.brs.2015.10.006
68. Yan J, Wei Y, Wang Y, Xu G, Li Z, Li X. Use of functional near-infrared spectroscopy to evaluate the effects of anodal transcranial direct current stimulation on brain connectivity in motor-related cortex. J Biomed Optics. (2015) 20:046007. doi: 10.1117/1.JBO.20.4.046007
69. Emamzadeh FN, Surguchov A. Parkinson's disease: biomarkers, treatment, and risk factors. Front Neurosci. (2018) 12:612. doi: 10.3389/fnins.2018.00612
70. Kaasinen V. Upregulation of putaminal dopamine D2 receptors in early Parkinson's disease: a comparative PET study with [11C] raclopride and [11C] N-methylspiperone. J Nuclear Med. (2000) 41:65–70.
Keywords: transcranial direct current stimulation (tDCS), Parkinson's disease, neuromodulation, motor symptoms, meta-analysis
Citation: Oliveira PCA, Araújo TAB, Machado DGS, Rodrigues AC, Bikson M, Andrade SM, Okano AH, Simplicio H, Pegado R and Morya E (2022) Transcranial Direct Current Stimulation on Parkinson's Disease: Systematic Review and Meta-Analysis. Front. Neurol. 12:794784. doi: 10.3389/fneur.2021.794784
Received: 14 October 2021; Accepted: 30 November 2021;
Published: 10 January 2022.
Edited by:
Fabio Godinho, University of São Paulo, BrazilReviewed by:
Francesco Di Lorenzo, Santa Lucia Foundation (IRCCS), ItalyBernardo Assumpção de Monaco, University of São Paulo, Brazil
Murilo Marinho, Federal University of São Paulo, Brazil
Clarice Listik, University of São Paulo, Brazil
Copyright © 2022 Oliveira, Araújo, Machado, Rodrigues, Bikson, Andrade, Okano, Simplicio, Pegado and Morya. This is an open-access article distributed under the terms of the Creative Commons Attribution License (CC BY). The use, distribution or reproduction in other forums is permitted, provided the original author(s) and the copyright owner(s) are credited and that the original publication in this journal is cited, in accordance with accepted academic practice. No use, distribution or reproduction is permitted which does not comply with these terms.
*Correspondence: Edgard Morya, ZWRnYXJkLm1vcnlhQGlzZC5vcmcuYnI=
†These authors have contributed equally to this work and share first authorship
‡These authors have contributed equally to this work and share senior authorship