- 1The National Clinical Research Center for Mental Disorders & Beijing Key Laboratory of Mental Disorders, Beijing Anding Hospital, Capital Medical University, Beijing, China
- 2Advanced Innovation Center for Human Brain Protection, Capital Medical University, Beijing, China
- 3Department of Acupuncture and Moxibustion, Guang'anmen Hospital, China Academy of Chinese Medical Sciences, Beijing, China
Introduction: Non-invasive brain stimulation (NIBS) techniques have been widely used for the purpose of improving clinical symptoms of schizophrenia. However, the ambiguous stimulation targets may limit the efficacy of NIBS for schizophrenia. Exploring effective stimulation targets may improve the clinical efficacy of NIBS in schizophrenia.
Methods: We first conducted a neurosynth-based meta-analysis of 715 functional magnetic resonance imaging studies to identify schizophrenia-related brain regions as regions of interest. Then, we performed the resting-state functional connectivity analysis in 32 patients with first-episode schizophrenia to find brain surface regions correlated with the regions of interest in three pipelines. Finally, the 10–20 system coordinates corresponding to the brain surface regions were considered as potential targets for NIBS.
Results: We identified several potential targets of NIBS, including the bilateral dorsal lateral prefrontal cortex, supplementary motor area, bilateral inferior parietal lobule, temporal pole, medial prefrontal cortex, precuneus, superior and middle temporal gyrus, and superior and middle occipital gyrus. Notably, the 10-20 system location of the bilateral dorsal lateral prefrontal cortex was posterior to F3 (F4), not F3 (F4).
Conclusion: Conclusively, our findings suggested that the stimulation locations corresponding to these potential targets might help clinicians optimize the application of NIBS therapy in individuals with schizophrenia.
Introduction
Schizophrenia (SCZ) is a complex psychiatric disorder associated with disturbances in social interaction and communication (1). Despite centuries of research, the pathophysiological cause of SCZ remains elusive. Around 18.8 to 20.8% of the patients with SCZ are thought to be non-responders to antipsychotic drugs (2). It is considered that the efficacious management also requires non-pharmacotherapies to treat SCZ patients in clinic.
Notably, non-invasive brain stimulation (NIBS) techniques have been widely used to improve the clinical symptoms of SCZ. The most commonly used NIBS technique is repetitive transcranial magnetic stimulation (rTMS). Low-frequency (≤1 Hz) rTMS reduces cortical excitability, whereas high-frequency (5–20 Hz) rTMS does the opposite (3). It has been demonstrated that rTMS on the left temporo-parietal region effectively reduced auditory hallucinations than bilateral or sham stimulation (4). Another frequent NIBS application used in treating SCZ is transcranial direct current stimulation (tDCS), which produces polarity-dependent cortical excitability changes (3). The tDCS appeared to be effective not only for ambulatory and higher-functioning patients but also for patients with ultra-treatment resistant SCZ (5). Specifically, NIBS exerts a small transdiagnostic effect on working memory (6). Nonetheless, a meta-analysis indicated that NIBS was not associated with a reliable improvement in working memory for individuals with SCZ (7). A possible reason limiting the efficacy of NIBS for SCZ is the ambiguous stimulation site (8). Indeed, there are several sites reported in studies, such as dorsolateral prefrontal cortex (dlPFC) (9–11), temporoparietal cortex (TPC) (12, 13), and superior temporal gyrus (STG) (14, 15). The sites used in NIBS research were empirical. Thus, it is necessary to identify viable stimulation sites before using NIBS techniques.
Novel stimulation sites for depression (16), mild cognitive impairment (17), and autism (18) were identified by combining meta-analysis and functional connectivity (FC) analysis from three pipelines. The pipelines contain brain surfaces from (1) meta-analysis, (2) FC analysis results from disease network, and (3) FC analysis results from each disease-associated region of interest (ROI). This method combines the preponderance of meta-analysis and the FC analysis. Researchers optimized the sites of NIBS for treating neuropsychiatric disorders, suggesting the worth of a connectivity-based targeting strategy for NIBS techniques. However, there have been no studies using this method to find potential sites for SCZ. In the present study, we performed a meta-analysis and resting-state FC analysis to identify brain surface regions associated with SCZ-related ROIs to investigate potential targets of NIBS treatment in SCZ.
Patients and Methods
Patients
A total of 32 right-handed first episode SCZ patients (13 males and 19 females) were included in the FC analysis. The age of these patients was 23.625 ± 7.404, 17 ~ 42 (M ± SD, Min ~ Max) years old. All examinations were carried out under the guidance of the Declaration of Helsinki. The present study was approved by the Ethics Committee of Beijing Anding Hospital, Capital medical university, China. All the subjects were Chinese Han people. Diagnoses were given by two trained psychiatrists using the Mini-International Neuropsychiatric Interview (M.I.N.I.) (19) under DSM-IV criteria. Psychiatric symptomatology was evaluated by using the Positive and Negative Syndrome Scale (PANSS) (20). Participants were excluded if they (a) were < 16 years old, (b) had current comorbid substance-use disorder (daily consumption of substances for at least one year), (c) had a history of neurological disorders or family history of hereditary neurological disorders, (d) had gross morphological anomalies as evidenced by brain MRI scans, and (e) had any electronic or metal implants.
MRI Data Acquisition
Resting-state functional magnetic resonance imaging (RS-fMRI) data were acquired with a 3.0 Tesla MRI scanner (Prisma 3.0; Siemens, Germany) in the Beijing Anding Hospital, Capital medical university, China. RS-fMRI were acquired with a single-shot, gradient-recalled echo-planar imaging sequence with the following parameters: repetition time = 2000 ms, echo time = 30 ms, flip angle = 90°, matrix = 64 × 64, field of view = 200 mm × 200 mm, slice thickness = 3.5 mm, gap = 1 mm, 33 axial sections, and 240 volumes.
High-resolution brain structural images were acquired with a T1-weighted three-dimensional (3D) multi-echo magnetization-prepared rapid gradient-echo (MPRAGE) sequence [echo time: 3.39 ms, repetition time: 2,530 ms, slice thickness 1.3 mm, voxel size: 1.3 × 1 × 1 mm3, field of view (FOV): 256 × 256 mm2, and volume number: 128].
Before scanning, all participants were asked to rest for 30 min and were instructed to stay still, keep their eyes closed, and not fall asleep during scanning. Foam head holders were immobilized to minimize head movements during scanning.
Image Processing
Firstly, the initial five volumes of the RS-fMRI data were removed. Secondly, the subjects whose head motion evaluated by the mean relative root mean square (RMS) exceeded 0.2 mm or whose maximum head motion exceeded 3 mm were excluded from the analysis. The two steps were conducted by Data Processing and Analysis for Brain Imaging (DPABI) version 5.1 (http://rfmri.org/dpabi) (21). Finally, the remaining subjects' images were preprocessed and analyzed in Conn version 18a (https://sites.google.com/view/conn/) (22) and SPM12 (using Conn's default preprocessing pipeline).
Conn's default preprocessing pipelines included both functional images' and structural images' preprocessing steps. Functional images were slice-timing corrected, realigned, normalized [3 × 3 × 3 mm3 in Montreal Neurological Institute (MNI) space], and smoothed (6 × 6 × 6 mm3). The outliers (>3 SD and >0.5 mm) for subsequent scrubbing regression were detected by the Artifact Detection Tool (www.nitrc.org/projects/artifact_detect/). The structural images were segmented into gray matter, white matter (WM), and cerebral spinal fluid (CSF) and normalized (3 × 3 × 3 mm3) to MNI space. Then, linear regression using WM and CSF signals (CompCor; 10 components for WM and five components for CSF), linear trend, subject motion (six rotation/translation motion parameters and six first-order temporal derivatives), and outliers (scrubbing) was conducted to remove confounding effects. After that, the residual blood oxygen level dependent (BOLD) time series was band-pass filtered (0.01–0.1 Hz).
Identifying SCZ-Associated ROIs From Meta-Analysis
In order to identify SCZ-associated ROIs, we conducted a meta-analysis including 715 fMRI studies under the “schizophrenia” term via Neurosynth platform (https://neurosynth.org/;accessed 13 August 2020) (23). The complete list of studies can be found in Supplementary Table S1. Neurosynth platform provides two types of meta-analysis results: the uniformity test maps and association test maps. The uniformity test map was used to identify the SCZ-associated brain regions since the uniformity test maps provide information about the consistency of activation for a given process. Association test maps provided information about the relative selectivity with which regions activate in a particular process (23). A false discovery rate (FDR) adjusted p-value of 0.01 was applied to produce the uniformity test map. Next, the coordinates with peak z-scores with all clusters larger than 50 voxels were identified by the xjview toolbox (http://www.alivelearn.net/xjview/). Finally, the 6-mm radius spherical masks centered on the specified peak coordinates were exacted by MarsBaR (http://marsbar.sourceforge.net/, version 0.44). Finally, the masks from MarsBaR and the original uniformity test map from Neurosynth were taken the overlap by xjview. The final ROIs only included the voxels from the original uniformity test map.
FC Analysis
To explore potential brain surface regions of SCZ, we conducted a seed-to-voxel FC analysis by Conn. At the subject level, the residual BOLD time course was extracted from the ROIs, and Pearson's correlation coefficients were computed between ROIs and all other brain voxels. Then, the coefficients were subsequently transformed into z-scores to increase normality. All subject-level seed maps of seed-to-voxel connectivity were included in a one sample t-test to get a group-level correlation map.
Exploring Potential NIBS Locations for SCZ
As the NIBS technique could not access whole brain regions, we used a brain surface mask created in previous studies (16, 18). The mask included the following brain regions: the bilateral pre and post-central gyrus; superior and middle frontal gyrus; superior, inferior, and middle occipital gyrus; superior and inferior parietal lobule; supramarginal gyrus; angular gyrus; superior temporal gyrus; superior temporal pole; middle temporal gyrus (MTG); middle temporal pole; inferior temporal gyrus; opercular inferior frontal gyrus (IFG); Rolandic operculum; triangular IFG; superior medial frontal gyrus; calcarine sulcus; orbital middle, superior and inferior frontal gyri; orbital medial frontal gyrus; supplementary motor area (SMA); paracentral lobule; precuneus; and cuneus. To explore the potential NIBS locations for SCZ, we picked brain surfaces from three different pipelines (Figure 1): (1) meta-analysis; (2) FC analysis results of SCZ network; (3) binary masks combined from each SCZ ROI FC analysis results. In pipelines 2 and 3, a voxel-wise level threshold of p < 0.001 and a cluster level family-wise error (FWE) of p < 0.05 were applied to obtain group-level correlation maps of ROIs.
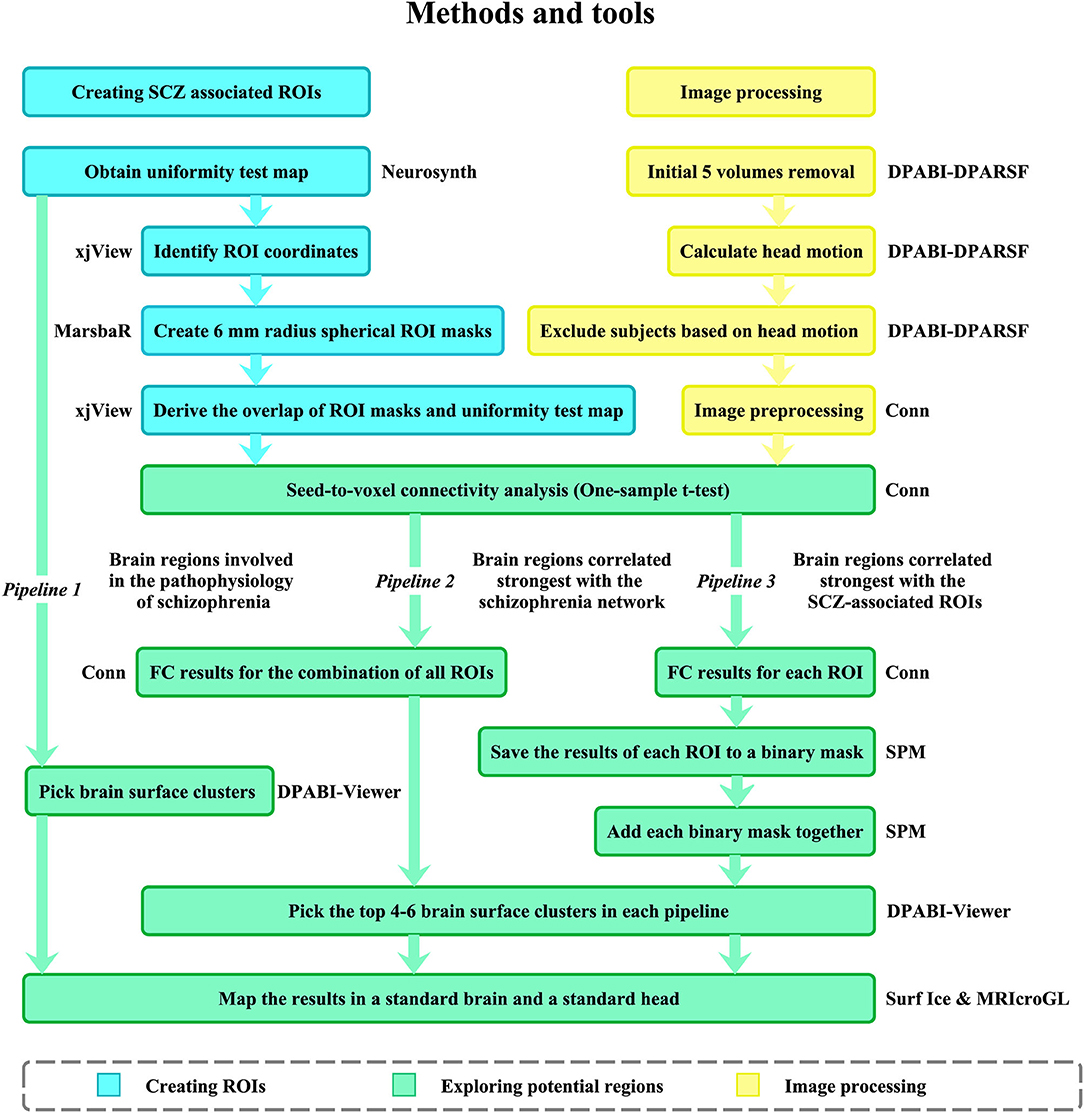
Figure 1. Data processing procedure. SCZ associated ROIs were identified from meta-analysis conducted by Neurosynth. The ROIs were used for FC analysis in 32 SCZ patients. Then, three pipelines were applied to explore potential targets for NIBS in SCZ. ROI, region of interest; FC, functional connectivity; RMS, root mean square; SCZ, schizophrenia.
Pipeline 1 Meta-Analysis
The brain surface clusters were directly picked from the Neurosynth meta-analysis (the uniformity test map) since the brain regions may be directly involved in the pathophysiology of SCZ.
Pipeline 2 FC Analysis Results of SCZ Network
The SCZ-associated ROIs were formed into an SCZ network, which was regarded as an ROI for FC analysis in CONN. Next, we excluded the clusters smaller than 20 voxels on the group-level correction map. Finally, four to six surface clusters with the largest peak z-scores were picked, with positive and negative correlation maps, respectively. These clusters represent the brain surface regions possessing the strongest correlations with the SCZ network.
Pipeline 3 Combined Binary Masks From FC Analysis Results of Each SCZ-Associated ROI
The group-level correlation map of each SCZ-associated ROI was saved to a binary mask. The binary masks of all ROIs formed a third-level map (positive and negative correlation maps, respectively). The intensity of each voxel in the third-level map represents the number of SCZ–ROIs correlated with the voxel. Finally, we identified four to six surface clusters as potential regions with the largest peak z-scores among all clusters larger than 20 voxels. These clusters represented the brain surface regions which were correlated with the largest number of SCZ-ROIs. The results of the three pipelines were mapped onto a standard brain and a standard head with the international 10–20 system in MNI space (24) using Surf Ice (www.nitrc.org/projects/surfice/) and MRIcroGL (www.mccauslandcenter.sc.edu/mricrogl/).
Results
SCZ-Associated ROIs Identified From Meta-Analysis
Fifteen clusters with peak coordinates were identified from the meta-analysis (Table 1). The included studies are listed in the (Supplementary Table S1). These coordinates were used to create 6 mm radius spherical masks, including the bilateral amygdala, insula, thalamus, caudate, and the left caudate, precentral, precuneus, supplementary motor area (SMA), inferior parietal lobule, and the right middle frontal cortex. We took the overlap of these masks and the original meta-analysis map (Supplementary Figure S2). Finally, the refined masks were used for seed-to-voxel connectivity analysis.
Potential NIBS Locations for SCZ
Thirty-two SCZ patients were included in this meta-analysis. The results of these three pipelines mapped on a standard brain and a standard head in the MNI space were shown in Table 2 and Figure 2. The original results of each pipeline were in (Supplementary Figures S3–S7).
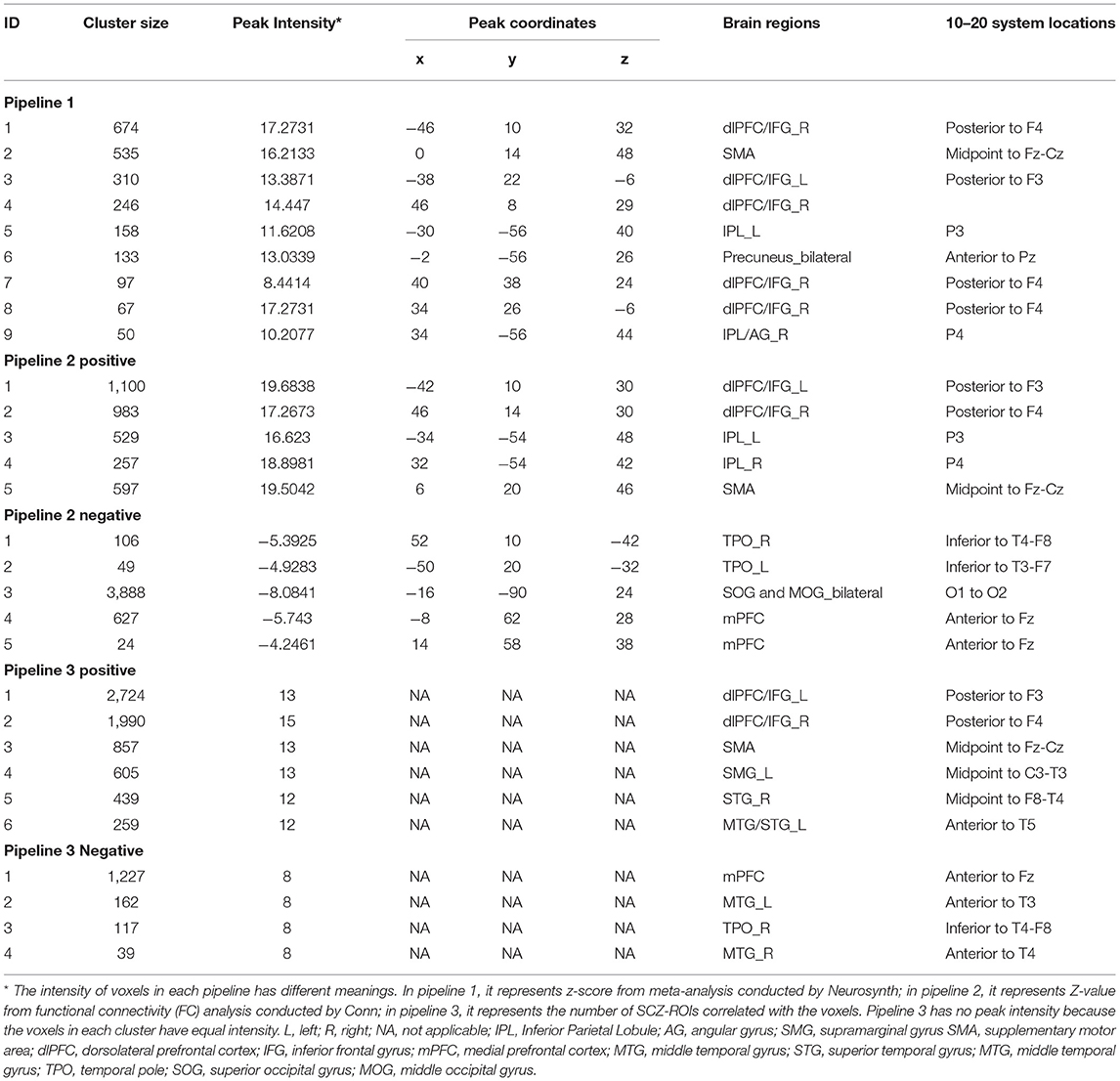
Table 2. Potential locations for non-invasive brain stimulation (NIBS) techniques in SCZ from the three pipelines.
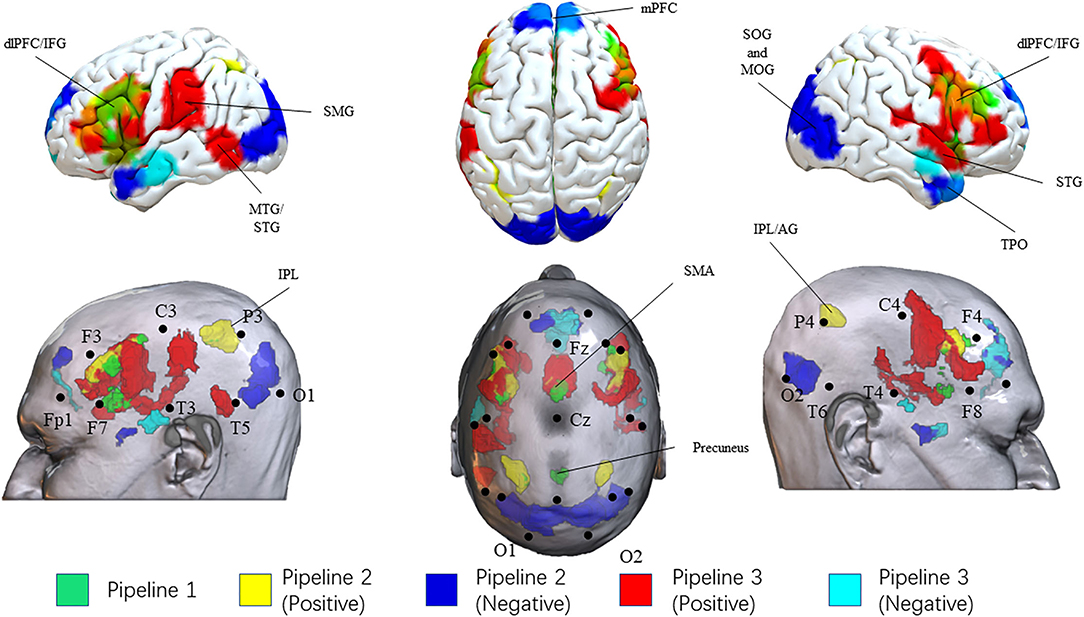
Figure 2. Results of three pipelines. Brain surface regions identified from the three pipelines were presented on the top. Scalp locations corresponding to the brain surface regions were presented on the bottom. Results from pipeline 1, pipeline 2 (positive correlation), pipeline 2 (negative correlation), pipeline 3 (positive correlation), pipeline 3 (negative correlation) were presented as green, yellow, blue, red, and cyan, respectively. L, left; R, right; IPL, Inferior Parietal Lobule; AG, angular gyrus; SMG, supramarginal gyrus SMA, supplementary motor area; dlPFC, dorsolateral prefrontal cortex; IFG, inferior frontal gyrus; mPFC, medial prefrontal cortex; MTG, middle temporal gyrus; STG, superior temporal gyrus; MTG, middle temporal gyrus; TPO, temporal pole; SOG, superior occipital gyrus; MOG, middle occipital gyrus.
In pipeline 1, we directly picked up the brain surface regions from the meta-analysis. Finally, the bilateral dlPFC/IFG, SMA, inferior parietal lobule, and precuneus were identified as potential brain surface regions. The 10–20 system coordinates corresponding to the center of these regions were located approximately posterior to F3(F4), midpoint to Fz-Cz, P3(P4), and anterior to Pz. These brain regions may be directly involved in the pathophysiology of SCZ.
In pipeline 2, group-level FC analysis results (SCZ-network as seed) were mapped onto the head surface. Finally, the bilateral dlPFC/IFG (approximately posterior to F3/F4), inferior parietal lobule [IPL (P3/P4)], and SMA (midpoint to Fz-Cz) were found to be positively correlated with the SCZ network. The bilateral temporal pole, superior and middle occipital gyrus, and medial prefrontal cortex were showed overlap negatively correlated with the SCZ network. These regions were located approximately inferior to T4-F8 (T3-F7), midpoint to O1-O2, and anterior to Fz. These brain regions possessed the strongest correlations with the SCZ network.
In pipeline 3, the largest number of brain surface regions from the third-level FC analysis were picked. Finally, we found that the bilateral dlPFC/IFG, SMA, the right STG, the left MTG/STS, and the left supramarginal gyrus were positively correlated with SCZ-associated ROIs, located approximately posterior to F3(F4), midpoint to Fz-Cz, midpoint to F8-T4, anterior to T5, and midpoint to C3-T3. The bilateral MTG, medial prefrontal cortex, and the right temporal pole were potential brain surface regions positively correlated with SCZ-associated ROIs. The 10–20 system coordinates corresponding to the center of these regions were located approximately anterior to T3(T4), anterior to Fz, and inferior to T4-F8. These brain surface regions correlated with the largest number of SCZ-ROIs.
Previous NIBS Studies in SCZ
We summarized the targets used in the previous NIBS studies in SCZ from several systematic reviews (8, 25–27) in Table 3. The left dlPFC and the left TPJ were the most frequency targets used in the rTMS and tDCS.
Discussion
In the present study, we attempt to explore potential brain regions and their corresponding scalp locations for NIBS techniques in treating SCZ. We have detected several potential brain regions by combining meta-analysis and FC analysis, which may contribute to improve the clinical efficacy of NIBS in SCZ.
The bilateral dlPFC/IFG and SMA are the most frequent targets for NIBS treatment in SCZ. Previous meta-analyses have demonstrated that high frequency rTMS and tDCS did not have a reliable improvement on treating SCZ by modulating the dlPFC (7, 70, 71). Contrary to previous clinical trials, our results showed that the location of dlPFC was posterior to F3 (F4) rather than exactly F3 (F4). The brain stimulation experiments in healthy subjects have illustrated that posterior to F3 was the optimal location for stimulating the dlPFC (72). Particularly, the Brodmann Area 9 located on the posterior to F4 in the dlPFC was remarkably associated with negative symptom severity (73). These findings suggest that stimulating the posterior to the F3 (F4) rather than the exact F3 (F4) may improve NIBS efficacy for negative symptoms.
In addition, the resting-state hyperperfusion of the SMA was considered as a marker of current catatonia in SCZ (74). Furthermore, the altered gray matter (74) and white matter volume (75) in the SMA were associated with disturbed motor behavior in SCZ. In our results, the SMA was identified in three pipelines suggesting that the SMA could serve as a considerable NIBS stimulation location for treating SCZ patients, especially those with motor abnormalities. The SMA is easily accessible using NIBS, while it is still virtually left to explore in SCZ. After reviewing the literature and registered trials, we have found just one published trial (76) and one ongoing trial — the Overcoming Psychomotor Slowing in Psychosis trial (OCoPS-P, NCT03921450) — for motor abnormalities in patients with SCZ over the SMA. The published trial, conducted by the Sebastian Walther group, has reported that inhibitory stimulation of the SMA might ameliorate psychomotor slowing in psychosis and major depression patients (76). Our data corroborated the ideas of Sebastian Walther, who suggested that NIBS stimulation of the cortical motor areas could be a practical methodology for improving and restoring motor impairment in SCZ (77).
There are other brain regions identified in our study, involving the bilateral IPL, the right temporal pole (TPO), and the medial prefrontal cortex (mPFC). IPL is one of the structures in the sense of agency and the dysconnectivity of the agency network (78), considered as a center of multisensory integration (79). The bilateral IPL, especially the angular gyrus (AG), may be directly involved in the pathophysiology of SCZ and extremely correlate with the SCZ network. A prior study has implicated that the reduction of IPL might be specific for long-term antipsychotic treatment (80). Our findings further supported that IPL could be a possible target for medication development in the future. Moreover, our results provided functional image evidence for the alteration of mPFC and TPO. The altered dopaminergic and GABAergic modulation in the mPFC is involved in SCZ progression (81). Previous meta-analysis including 4,474 individuals with SCZ has reported that only TPO thickness was negatively correlated with age, and cortical volumes at illness onset and progressive volume were declined in the temporal pole in SCZ (82). The right TPO and mPFC may strongly correlate with the SCZ network and correlate with the largest number of SCZ-associated ROIs. Despite the importance of mPFC and TPO, there remains a paucity of evidence for NIBS techniques treating SCZ over mPFC or TPO.
Interestingly, the dlPFC, SMA, and MTG are the components of the task positive network (TPN), which associates with externally oriented attention (83, 84). The mPFC, IPL, and precuneus play an important role in the default mode network (DMN) related to introspectively oriented cognitive processes, such as self-referential and reflective activity. Consistent with another study (85), we observed that TPN and DMN networks were anti-correlated. Here, our study provided the evidence to support that the anti-correlated networks were relevant to SCZ. Balancing the TPN and DMN network may have a beneficious effect in treating SCZ by NIBS to regulate neural circuits (3, 86).
Some limitations are needed to pay attention in the present study. First, excitatory or inhibitory brain regions in our study are not to be discriminated, which is essential to some NIBS techniques such as TMS. Second, the parameters used in each NIBS technique have not been studied, which may affect the efficacy. Finally, neurosynth-based meta-analysis is not flawless – potential error could occur – although several supporting analyses have been conducted to confirm the validity and sensitivity.
Conclusion
Combining meta-analysis and FC analysis from three pipelines, we identified several potential NIBS targets on the brain surface (with dlPFC and SMA to be the most promising regions) and locations on the scalp for treating SCZ patients. Specifically, the location of dlPFC was suggested to be posterior to F3 (F4) and not F3 (F4). Besides, we also identified that balancing the TPN and DMN might be a potential strategy to treat SCZ. These identified targets could contribute to improving the efficacy of NIBS in treating SCZ patients.
Data Availability Statement
The original contributions presented in the study are included in the article/Supplementary Material, further inquiries can be directed to the corresponding author/s.
Ethics Statement
The studies involving human participants were reviewed and approved by the Ethics Committee of Beijing Anding Hospital. The patients/participants provided their written informed consent to participate in this study.
Author Contributions
HJ provided his expertise in schizophrenia, managed the data collection, and contributed to the writing of the manuscript. BZ conceived the idea and methodology for the study, designed the study, and contributed to the writing of the manuscript. YN and SZ managed data analyses and wrote the manuscript. SF was contributed to the writing of the manuscript and the graph display. All authors contributed to the article and approved the submitted version.
Funding
This study was supported by Beijing Natural Science Foundation (Grant No. 7212050), Capital's Funds for Health Improvement and Research (Grant Nos. 2018-1-2122, 2020-4-2126), Beijing Hospitals Authority Clinical Medicine Development of Special Funding (Grant No. ZYLX202129), Beijing Hospitals Authority's Ascent Plan (Grant No. DFL20191901), Beijing Hospitals Authority Youth Program (Grant No. QML20201901), Talents Training Fund of Beijing (Grant No. 2018000021469G292), China Academy of Chinese Medical Sciences Fund for Excellent Young Scholars (Grant No. ZZ14-YQ-017), and China Academy of Chinese Medical Sciences Innovation Fund (Grant No. CI2021A03316).
Conflict of Interest
The authors declare that the research was conducted in the absence of any commercial or financial relationships that could be construed as a potential conflict of interest.
The handling editor declared a past collaboration with one of the authors BZ.
Publisher's Note
All claims expressed in this article are solely those of the authors and do not necessarily represent those of their affiliated organizations, or those of the publisher, the editors and the reviewers. Any product that may be evaluated in this article, or claim that may be made by its manufacturer, is not guaranteed or endorsed by the publisher.
Supplementary Material
The Supplementary Material for this article can be found online at: https://www.frontiersin.org/articles/10.3389/fneur.2021.766736/full#supplementary-material
References
1. Owen MJ, Sawa A, Mortensen PB. Schizophrenia. Lancet. (2016) 388:86–97. doi: 10.1016/S0140-6736(15)01121-6
2. Samara MT, Nikolakopoulou A, Salanti G, Leucht S. How many patients with schizophrenia do not respond to antipsychotic drugs in the short term? An analysis based on individual patient data from randomized controlled trials. Schizophr Bull. (2019) 45:639–46. doi: 10.1093/schbul/sby095
3. Cirillo G, Di Pino G, Capone F, Ranieri F, Florio L, Todisco V, et al. Neurobiological after-effects of non-invasive brain stimulation. Brain Stimul. (2017) 10:1–18. doi: 10.1016/j.brs.2016.11.009
4. Vercammen A, Knegtering H, Bruggeman R, Westenbroek HM, Jenner JA, Slooff CJ, et al. Effects of bilateral repetitive transcranial magnetic stimulation on treatment resistant auditory-verbal hallucinations in schizophrenia: a randomized controlled trial. Schizophr Res. (2009) 114:172–9. doi: 10.1016/j.schres.2009.07.013
5. Lindenmayer JP, Kulsa MKC, Sultana T, Kaur A, Yang R, Ljuri I, et al. Transcranial direct-current stimulation in ultra-treatment-resistant schizophrenia. Brain Stimul. (2019) 12:54–61. doi: 10.1016/j.brs.2018.10.002
6. Begemann MJ, Brand BA, Curčić-Blake B, Aleman A, Sommer IE. Efficacy of non-invasive brain stimulation on cognitive functioning in brain disorders: a meta-analysis. Psychol Med. (2020) 50:2465–86. doi: 10.1017/S0033291720003670
7. Sloan NP, Byrne LK, Enticott PG, Lum JG. Non-invasive brain stimulation does not improve working memory in schizophrenia: a meta-analysis of randomised controlled trials. Neuropsychol Rev. (2021) 31:115–38. doi: 10.1007/s11065-020-09454-4
8. Marzouk T, Winkelbeiner S, Azizi H, Malhotra AK, Homan P. Transcranial magnetic stimulation for positive symptoms in schizophrenia: a systematic review. Neuropsychobiology. (2020) 79:384–96. doi: 10.1159/000502148
9. Brunoni AR, Vanderhasselt M-A. Working memory improvement with non-invasive brain stimulation of the dorsolateral prefrontal cortex: a systematic review and meta-analysis. Brain Cogn. (2014) 86:1–9. doi: 10.1016/j.bandc.2014.01.008
10. Smith RC, Boules S, Mattiuz S, Youssef M, Tobe RH, Sershen H, et al. Effects of transcranial direct current stimulation (tDCS) on cognition, symptoms, and smoking in schizophrenia: a randomized controlled study. Schizophr Res. (2015) 168:260–6. doi: 10.1016/j.schres.2015.06.011
11. Sreeraj VS, Bose A, Chhabra H, Shivakumar V, Agarwal SM, Narayanaswamy JC, et al. Working memory performance with online-tDCS in schizophrenia: a randomized, double-blinded, sham-controlled, partial cross-over proof-of-concept study. Asian J Psychiatr. (2020) 50:101946. doi: 10.1016/j.ajp.2020.101946
12. Vercammen A, Knegtering H, Liemburg EJ, Den Boer JA, Aleman A. Functional connectivity of the temporo-parietal region in schizophrenia: effects of rTMS treatment of auditory hallucinations. J Psychiatr Res. (2010) 44:725–31. doi: 10.1016/j.jpsychires.2009.12.011
13. Blumberger DM, Christensen BK, Zipursky RB, Moller B, Chen R, Fitzgerald PB, et al. MRI-targeted repetitive transcranial magnetic stimulation of Heschl's gyrus for refractory auditory hallucinations. Brain Stimul. (2012) 5:577–85. doi: 10.1016/j.brs.2011.12.002
14. Schönfeldt-Lecuona C, Grön G, Walter H, Büchler N, Wunderlich A, Spitzer M, et al. Stereotaxic rTMS for the treatment of auditory hallucinations in schizophrenia. Neuroreport. (2004) 15:1669–73. doi: 10.1097/01.wnr.0000126504.89983.ec
15. Paillère-Martinot ML, Galinowski A, Plaze M, Andoh J, Bartrés-Faz D, Bellivier F, et al. Active and placebo transcranial magnetic stimulation effects on external and internal auditory hallucinations of schizophrenia. Acta Psychiatr Scand. (2017) 135:228–38. doi: 10.1111/acps.12680
16. Zhang B, Liu J, Bao T, Wilson G, Park J, Zhao B, et al. Locations for noninvasive brain stimulation in treating depressive disorders: A combination of meta-analysis and resting-state functional connectivity analysis. Austr New Zeal J Psychiatr. (2020) 54:582–90. doi: 10.1177/0004867420920372
17. Liu J, Zhang B, Wilson G, Kong J, Weiner MW, Aisen P, et al. New perspective for non-invasive brain stimulation site selection in mild cognitive impairment: based on meta- and functional connectivity analyses. Front Aging Neurosci. (2019) 11:228. doi: 10.3389/fnagi.2019.00228
18. Huang Y, Zhang B, Cao J, Yu S, Wilson G, Park J, et al. Potential locations for noninvasive brain stimulation in treating autism spectrum disorders—a functional connectivity study. Front Psychiatry. (2020) 11. doi: 10.3389/fpsyt.2020.00388
19. Sheehan DV, Lecrubier Y, Sheehan KH, Amorim P, Janavs J, Weiller E, et al. The Mini-International Neuropsychiatric Interview (M.I.N.I.): the development and validation of a structured diagnostic psychiatric interview for DSM-IV and ICD-10. J Clin Psychiatry. (1998) 59:22–33. doi: 10.1037/t18597-000
20. Kay SR, Fiszbein A, Opler LA. The positive and negative syndrome scale (PANSS) for schizophrenia. Schizophr Bull. (1987) 13:261–76. doi: 10.1093/schbul/13.2.261
21. Yan C-G, Wang X-D, Zuo X-N, Zang Y-F. DPABI: data processing and analysis for (resting-state) brain imaging. Neuroinformatics. (2016) 14:339–51. doi: 10.1007/s12021-016-9299-4
22. Whitfield-Gabrieli S, Nieto-Castanon A. Conn: a functional connectivity toolbox for correlated and anticorrelated brain networks. Brain Connect. (2012) 2:125–41. doi: 10.1089/brain.2012.0073
23. Yarkoni T, Poldrack RA, Nichols TE, Van Essen DC, Wager TD. Large-scale automated synthesis of human functional neuroimaging data. Nat Methods. (2011) 8:665–70. doi: 10.1038/nmeth.1635
24. Cutini S, Scatturin P, Zorzi M. A new method based on ICBM152 head surface for probe placement in multichannel fNIRS. Neuroimage. (2011) 54:919–27. doi: 10.1016/j.neuroimage.2010.09.030
25. Osoegawa C, Gomes JS, Grigolon RB, Brietzke E, Gadelha A, Lacerda ALT, et al. Non-invasive brain stimulation for negative symptoms in schizophrenia: an updated systematic review and meta-analysis. Schizophr Res. (2018) 197:34–44. doi: 10.1016/j.schres.2018.01.010
26. Mehta UM, Naik SS, Thanki MV, Thirthalli J. Investigational and therapeutic applications of transcranial magnetic stimulation in schizophrenia. Curr Psychiatry Rep. (2019) 21:89. doi: 10.1007/s11920-019-1076-2
27. Cheng PWC, Louie LLC, Wong YL, Wong SMC, Leung WY, Nitsche MA, et al. The effects of transcranial direct current stimulation (tDCS) on clinical symptoms in schizophrenia: a systematic review and meta-analysis. Asian J Psychiatr. (2020) 53:102392. doi: 10.1016/j.ajp.2020.102392
28. Mcintosh AM, Semple D, Tasker K, Harrison LK, Owens DG, Johnstone EC, et al. Transcranial magnetic stimulation for auditory hallucinations in schizophrenia. Psychiatry Res. (2004) 127:9–17. doi: 10.1016/j.psychres.2004.03.005
29. Fitzgerald PB, Benitez J, Daskalakis JZ, Brown TL, Marston NA, De Castella A, et al. A double-blind sham-controlled trial of repetitive transcranial magnetic stimulation in the treatment of refractory auditory hallucinations. J Clin Psychopharmacol. (2005) 25:358–62. doi: 10.1097/01.jcp.0000168487.22140.7f
30. Jandl M, Steyer J, Weber M, Linden DE, Rothmeier J, Maurer K, et al. Treating auditory hallucinations by transcranial magnetic stimulation: a randomized controlled cross-over trial. Neuropsychobiology. (2006) 53:63–9. doi: 10.1159/000091721
31. Saba G, Verdon CM, Kalalou K, Rocamora JF, Dumortier G, Benadhira R, et al. Transcranial magnetic stimulation in the treatment of schizophrenic symptoms: a double blind sham controlled study. J Psychiatr Res. (2006) 40:147–52. doi: 10.1016/j.jpsychires.2005.02.008
32. Rosa MO, Gattaz WF, Rosa MA, Rumi DO, Tavares H, Myczkowski M, et al. Effects of repetitive transcranial magnetic stimulation on auditory hallucinations refractory to clozapine. J Clin Psychiatry. (2007) 68:1528–32. doi: 10.4088/JCP.v68n1009
33. Slotema CW, Blom JD, De Weijer AD, Diederen KM, Goekoop R, Looijestijn J, et al. Can low-frequency repetitive transcranial magnetic stimulation really relieve medication-resistant auditory verbal hallucinations? Negative results from a large randomized controlled trial. Biol Psychiatry. (2011) 69:450–6. doi: 10.1016/j.biopsych.2010.09.051
34. Hoffman RE, Boutros NN, Berman RM, Roessler E, Belger A, Krystal JH, et al. Transcranial magnetic stimulation of left temporoparietal cortex in three patients reporting hallucinated “voices”. Biol Psychiatry. (1999) 46:130–2. doi: 10.1016/S0006-3223(98)00358-8
35. Hoffman RE, Boutros NN, Hu S, Berman RM, Krystal JH, Charney DS. Transcranial magnetic stimulation and auditory hallucinations in schizophrenia. Lancet. (2000) 355:1073–5. doi: 10.1016/S0140-6736(00)02043-2
36. Hoffman RE, Hawkins KA, Gueorguieva R, Boutros NN, Rachid F, Carroll K, et al. Transcranial magnetic stimulation of left temporoparietal cortex and medication-resistant auditory hallucinations. Arch Gen Psychiatry. (2003) 60:49–56. doi: 10.1001/archpsyc.60.1.49
37. Chibbaro G, Daniele M, Alagona G, Di Pasquale C, Cannavò M, Rapisarda V, et al. Repetitive transcranial magnetic stimulation in schizophrenic patients reporting auditory hallucinations. Neurosci Lett. (2005) 383:54–7. doi: 10.1016/j.neulet.2005.03.052
38. Hoffman RE, Gueorguieva R, Hawkins KA, Varanko M, Boutros NN, Wu YT, et al. Temporoparietal transcranial magnetic stimulation for auditory hallucinations: safety, efficacy and moderators in a fifty patient sample. Biol Psychiatry. (2005) 58:97–104. doi: 10.1016/j.biopsych.2005.03.041
39. Lee SH, Kim W, Chung YC, Jung KH, Bahk WM, Jun TY, et al. A double blind study showing that two weeks of daily repetitive TMS over the left or right temporoparietal cortex reduces symptoms in patients with schizophrenia who are having treatment-refractory auditory hallucinations. Neurosci Lett. (2005) 376:177–81. doi: 10.1016/j.neulet.2004.11.048
40. Bagati D, Nizamie SH, Prakash R. Effect of augmentatory repetitive transcranial magnetic stimulation on auditory hallucinations in schizophrenia: randomized controlled study. Aust N Z J Psychiatry. (2009) 43:386–92. doi: 10.1080/00048670802653315
41. Loo CK, Sainsbury K, Mitchell P, Hadzi-Pavlovic D, Sachdev PS. A sham-controlled trial of left and right temporal rTMS for the treatment of auditory hallucinations. Psychol Med. (2010) 40:541–6. doi: 10.1017/S0033291709990900
42. Klein E, Kolsky Y, Puyerovsky M, Koren D, Chistyakov A, Feinsod M. Right prefrontal slow repetitive transcranial magnetic stimulation in schizophrenia: a double-blind sham-controlled pilot study. Biol Psychiatry. (1999) 46:1451–4. doi: 10.1016/S0006-3223(99)00182-1
43. Hajak G, Marienhagen J, Langguth B, Werner S, Binder H, Eichhammer P. High-frequency repetitive transcranial magnetic stimulation in schizophrenia: a combined treatment and neuroimaging study. Psychol Med. (2004) 34:1157–63. doi: 10.1017/S0033291704002338
44. Holi MM, Eronen M, Toivonen K, Toivonen P, Marttunen M, Naukkarinen H. Left prefrontal repetitive transcranial magnetic stimulation in schizophrenia. Schizophr Bull. (2004) 30:429–34. doi: 10.1093/oxfordjournals.schbul.a007089
45. Cordes J, Thünker J, Agelink MW, Arends M, Mobascher A, Wobrock T, et al. Effects of 10 Hz repetitive transcranial magnetic stimulation (rTMS) on clinical global impression in chronic schizophrenia. Psychiatry Res. (2010) 177:32–6. doi: 10.1016/j.psychres.2009.01.014
46. Rollnik JD, Huber TJ, Mogk H, Siggelkow S, Kropp S, Dengler R, et al. High frequency repetitive transcranial magnetic stimulation (rTMS) of the dorsolateral prefrontal cortex in schizophrenic patients. Neuroreport. (2000) 11:4013–5. doi: 10.1097/00001756-200012180-00022
47. Jin Y, Potkin SG, Kemp AS, Huerta ST, Alva G, Thai TM, et al. Therapeutic effects of individualized alpha frequency transcranial magnetic stimulation (alphaTMS) on the negative symptoms of schizophrenia. Schizophr Bull. (2006) 32:556–61. doi: 10.1093/schbul/sbj020
48. Schneider AL, Schneider TL, Stark H. Repetitive transcranial magnetic stimulation (rTMS) as an augmentation treatment for the negative symptoms of schizophrenia: a 4-week randomized placebo controlled study. Brain Stimul. (2008) 1:106–11. doi: 10.1016/j.brs.2008.01.001
49. Prikryl R, Ustohal L, Prikrylova Kucerova H, Kasparek T, Venclikova S, Vrzalova M, et al. A detailed analysis of the effect of repetitive transcranial magnetic stimulation on negative symptoms of schizophrenia: a double-blind trial. Schizophr Res. (2013) 149:167–73. doi: 10.1016/j.schres.2013.06.015
50. Prikryl R, Ustohal L, Kucerova HP, Kasparek T, Jarkovsky J, Hublova V, et al. Repetitive transcranial magnetic stimulation reduces cigarette consumption in schizophrenia patients. Prog Neuropsychopharmacol Biol Psychiatry. (2014) 49:30–5. doi: 10.1016/j.pnpbp.2013.10.019
51. Wölwer W, Lowe A, Brinkmeyer J, Streit M, Habakuck M, Agelink MW, et al. Repetitive transcranial magnetic stimulation (rTMS) improves facial affect recognition in schizophrenia. Brain Stimul. (2014) 7:559–63. doi: 10.1016/j.brs.2014.04.011
52. Li Z, Yin M, Lyu X-L, Zhang L-L, Du X-D, Hung GC-L. Delayed effect of repetitive transcranial magnetic stimulation (rTMS) on negative symptoms of schizophrenia: Findings from a randomized controlled trial. Psychiatry Res. (2016) 240:333–5. doi: 10.1016/j.psychres.2016.04.046
53. Zhou DD, Wang W, Wang GM, Li DQ, Kuang L. An updated meta-analysis: Short-term therapeutic effects of repeated transcranial magnetic stimulation in treating obsessive-compulsive disorder. J Affect Disord. (2017) 215:187–96. doi: 10.1016/j.jad.2017.03.033
54. Fitzgerald PB, Herring S, Hoy K, Mcqueen S, Segrave R, Kulkarni J, et al. A study of the effectiveness of bilateral transcranial magnetic stimulation in the treatment of the negative symptoms of schizophrenia. Brain Stimul. (2008) 1:27–32. doi: 10.1016/j.brs.2007.08.001
55. Barr MS, Farzan F, Tran LC, Fitzgerald PB, Daskalakis ZJ. A randomized controlled trial of sequentially bilateral prefrontal cortex repetitive transcranial magnetic stimulation in the treatment of negative symptoms in schizophrenia. Brain Stimul. (2012) 5:337–46. doi: 10.1016/j.brs.2011.06.003
56. Wobrock T, Guse B, Cordes J, Wölwer W, Winterer G, Gaebel W, et al. Left prefrontal high-frequency repetitive transcranial magnetic stimulation for the treatment of schizophrenia with predominant negative symptoms: a sham-controlled, randomized multicenter trial. Biol Psychiatry. (2015) 77:979–88. doi: 10.1016/j.biopsych.2014.10.009
57. Fitzgerald PB, Mcqueen S, Daskalakis ZJ, Hoy KE. A negative pilot study of daily bimodal transcranial direct current stimulation in schizophrenia. Brain Stimul. (2014) 7:813–6. doi: 10.1016/j.brs.2014.08.002
58. Fröhlich F, Burrello TN, Mellin JM, Cordle AL, Lustenberger CM, Gilmore JH, et al. Exploratory study of once-daily transcranial direct current stimulation (tDCS) as a treatment for auditory hallucinations in schizophrenia. Eur Psychiatry. (2016) 33:54–60. doi: 10.1016/j.eurpsy.2015.11.005
59. Bose A, Shivakumar V, Agarwal SM, Kalmady SV, Shenoy S, Sreeraj VS, et al. Efficacy of fronto-temporal transcranial direct current stimulation for refractory auditory verbal hallucinations in schizophrenia: a randomized, double-blind, sham-controlled study. Schizophr Res. (2018) 195:475–80. doi: 10.1016/j.schres.2017.08.047
60. Koops S, Blom JD, Bouachmir O, Slot MI, Neggers B, Sommer IE. Treating auditory hallucinations with transcranial direct current stimulation in a double-blind, randomized trial. Schizophr Res. (2018) 201:329–36. doi: 10.1016/j.schres.2018.06.010
61. Chang CC, Kao YC, Chao CY, Chang HA. Enhancement of cognitive insight and higher-order neurocognitive function by fronto-temporal transcranial direct current stimulation (tDCS) in patients with schizophrenia. Schizophr Res. (2019) 208:430–8. doi: 10.1016/j.schres.2018.12.052
62. Kantrowitz JT, Sehatpour P, Avissar M, Horga G, Gwak A, Hoptman MJ, et al. Significant improvement in treatment resistant auditory verbal hallucinations after 5 days of double-blind, randomized, sham controlled, fronto-temporal, transcranial direct current stimulation (tDCS): A replication/extension study. Brain Stimul. (2019) 12:981–91. doi: 10.1016/j.brs.2019.03.003
63. Brunelin J, Mondino M, Gassab L, Haesebaert F, Gaha L, Suaud-Chagny MF, et al. Examining transcranial direct-current stimulation (tDCS) as a treatment for hallucinations in schizophrenia. Am J Psychiatry. (2012) 169:719–24. doi: 10.1176/appi.ajp.2012.11071091
64. Mondino M, Jardri R, Suaud-Chagny MF, Saoud M, Poulet E, Brunelin J. Effects of fronto-temporal transcranial direct current stimulation on auditory verbal hallucinations and resting-state functional connectivity of the left temporo-parietal junction in patients with schizophrenia. Schizophr Bull. (2016) 42:318–26. doi: 10.1093/schbul/sbv114
65. Valiengo L, Goerigk S, Gordon PC, Padberg F, Serpa MH, Koebe S, et al. Efficacy and safety of transcranial direct current stimulation for treating negative symptoms in schizophrenia: a randomized clinical trial. JAMA Psychiatry. (2020) 77:121–9. doi: 10.1001/jamapsychiatry.2019.3199
66. Palm U, Keeser D, Hasan A, Kupka MJ, Blautzik J, Sarubin N, et al. Prefrontal transcranial direct current stimulation for treatment of schizophrenia with predominant negative symptoms: a double-blind, sham-controlled proof-of-concept study. Schizophr Bull. (2016) 42:1253–61. doi: 10.1093/schbul/sbw041
67. Chang CC, Kao YC, Chao CY, Tzeng NS, Chang HA. Examining bi-anodal transcranial direct current stimulation (tDCS) over bilateral dorsolateral prefrontal cortex coupled with bilateral extracephalic references as a treatment for negative symptoms in non-acute schizophrenia patients: a randomized, double-blind, sham-controlled trial. Prog Neuropsychopharmacol Biol Psychiatry. (2020) 96:109715. doi: 10.1016/j.pnpbp.2019.109715
68. Gomes JS, Trevizol AP, Ducos DV, Gadelha A, Ortiz BB, Fonseca AO, et al. Effects of transcranial direct current stimulation on working memory and negative symptoms in schizophrenia: a phase II randomized sham-controlled trial. Schizophr Res Cogn. (2018) 12:20–8. doi: 10.1016/j.scog.2018.02.003
69. Jeon DW, Jung DU, Kim SJ, Shim JC, Moon JJ, Seo YS, et al. Adjunct transcranial direct current stimulation improves cognitive function in patients with schizophrenia: a double-blind 12-week study. Schizophr Res. (2018) 197:378–85. doi: 10.1016/j.schres.2017.12.009
70. Lefaucheur J-P, André-Obadia N, Antal A, Ayache SS, Baeken C, Benninger DH, et al. Evidence-based guidelines on the therapeutic use of repetitive transcranial magnetic stimulation (rTMS). Clin Neurophysiol. (2014) 125:2150–206. doi: 10.1016/j.clinph.2014.05.021
71. Pondé PH, De Sena EP, Camprodon JA, de Araújo AN, Neto MF, DiBiasi M, et al. Use of transcranial direct current stimulation for the treatment of auditory hallucinations of schizophrenia - a systematic review. Neuropsychiatric Dis Treatment. (2017) 13:347–55. doi: 10.2147/NDT.S122016
72. Fitzgerald PB, Maller JJ, Hoy KE, Thomson R, Daskalakis ZJ. Exploring the optimal site for the localization of dorsolateral prefrontal cortex in brain stimulation experiments. Brain Stimul. (2009) 2:234–7. doi: 10.1016/j.brs.2009.03.002
73. Brady RO, Gonsalvez I, Lee I, Öngür D, Seidman LJ, Schmahmann JD, et al. Breakdown of functional connectivity in cerebellar-prefrontal network underlies negative symptoms in schizophrenia. Am J Psychiatry. (2019) 176:512–20. doi: 10.1176/appi.ajp.2018.18040429
74. Stegmayer K, Horn H, Federspiel A, Razavi N, Bracht T, Laimböck K, et al. Supplementary motor area (SMA) volume is associated with psychotic aberrant motor behaviour of patients with schizophrenia. Psychiatry Res. (2014) 223:49–51. doi: 10.1016/j.pscychresns.2014.05.002
75. Walther S, Federspiel A, Horn H, Razavi N, Wiest R, Dierks T, et al. Alterations of white matter integrity related to motor activity in schizophrenia. Neurobiol Dis. (2011) 42:276–83. doi: 10.1016/j.nbd.2011.01.017
76. Walther S, Alexaki D, Schoretsanitis G, Weiss F, Vladimirova I, Stegmayer K, et al. Inhibitory repetitive transcranial magnetic stimulation to treat psychomotor slowing: a transdiagnostic, mechanism-based randomized double-blind controlled trial. Schizophrenia Bull Open. (2020) 1:sgaa020. doi: 10.1093/schizbullopen/sgaa020
77. Lefebvre S, Pavlidou A, Walther S. What is the potential of neurostimulation in the treatment of motor symptoms in schizophrenia? Expert Rev Neurother. (2020) 20:697–706. doi: 10.1080/14737175.2020.1775586
78. Koreki A, Maeda T, Okimura T, Terasawa Y, Kikuchi T, Umeda S, et al. Dysconnectivity of the agency network in schizophrenia: a functional magnetic resonance imaging study. Front Psychiatry. (2019) 10:171. doi: 10.3389/fpsyt.2019.00171
79. Smiley JF, Konnova K, Bleiwas C. Cortical thickness, neuron density and size in the inferior parietal lobe in schizophrenia. Schizophr Res. (2012) 136:43–50. doi: 10.1016/j.schres.2012.01.006
80. Liu N, Xiao Y, Zhang W, Tang B, Zeng J, Hu N, et al. Characteristics of gray matter alterations in never-treated and treated chronic schizophrenia patients. Transl Psychiatry. (2020) 10:136. doi: 10.1038/s41398-020-0828-4
81. Xu P, Chen A, Li Y, Xing X, Lu H. Medial prefrontal cortex in neurological diseases. Physiol Genomics. (2019) 51:432–42. doi: 10.1152/physiolgenomics.00006.2019
82. Van Erp TGM, Walton E, Hibar DP, Schmaal L, Jiang W, Glahn DC, et al. Cortical brain abnormalities in 4474 individuals with schizophrenia and 5098 control subjects via the enhancing neuro imaging genetics through meta analysis (ENIGMA) consortium. Biol Psychiatry. (2018) 84:644–54. doi: 10.1016/j.biopsych.2018.04.023
83. Williamson P. Are anticorrelated networks in the brain relevant to schizophrenia? Schizophr Bull. (2007) 33:994–1003. doi: 10.1093/schbul/sbm043
84. Liu H, Kaneko Y, Ouyang X, Li L, Hao Y, Chen EYH, et al. Schizophrenic patients and their unaffected siblings share increased resting-state connectivity in the task-negative network but not its anticorrelated task-positive network. Schizophr Bull. (2012) 38:285–94. doi: 10.1093/schbul/sbq074
85. Hu M-L, Zong X-F, Mann JJ, Zheng J-J, Liao Y-H, Li Z-C, et al. A review of the functional and anatomical default mode network in schizophrenia. Neurosci Bull. (2017) 33:73–84. doi: 10.1007/s12264-016-0090-1
Keywords: schizophrenia, non-invasive brain stimulation, functional magnetic resonance imaging, functional connectivity, DLPFC (dorsolateral prefrontal cortex)
Citation: Ning Y, Zheng S, Feng S, Zhang B and Jia H (2021) Potential Locations for Non-Invasive Brain Stimulation in Treating Schizophrenia: A Resting-State Functional Connectivity Analysis. Front. Neurol. 12:766736. doi: 10.3389/fneur.2021.766736
Received: 30 August 2021; Accepted: 09 November 2021;
Published: 15 December 2021.
Edited by:
Jian Kong, Massachusetts General Hospital and Harvard Medical School, United StatesReviewed by:
Jordi A. Matias-Guiu, Hospital Clínico San Carlos, SpainYann Quidé, University of New South Wales, Australia
Copyright © 2021 Ning, Zheng, Feng, Zhang and Jia. This is an open-access article distributed under the terms of the Creative Commons Attribution License (CC BY). The use, distribution or reproduction in other forums is permitted, provided the original author(s) and the copyright owner(s) are credited and that the original publication in this journal is cited, in accordance with accepted academic practice. No use, distribution or reproduction is permitted which does not comply with these terms.
*Correspondence: Binlong Zhang, YmlubG9uZ3poYW5nQGJ1Y20uZWR1LmNu; Hongxiao Jia, amh4bGpAY2NtdS5lZHUuY24=
†These authors have contributed equally to this work