- 1Department of Adult Neurology, University Clinical Center, Medical University of Gdańsk, Gdańsk, Poland
- 2II Department of Radiology, Medical University of Gdańsk, Department of Radiology, University Clinical Centre, Gdańsk, Poland
Background: The main and well-defined complication of intravenous administration of recombinant tissue plasminogen activator (tPA) in patients with acute ischemic stroke (AIS) is symptomatic intracranial hemorrhage (sICH). However, rtPA might also be connected with the formation of cerebral microbleeds (CMBs), located remotely from the ischemic lesions, that may remain clinically silent. This association might be important because the load of CMBs has been associated with cognitive impairment. We investigated whether administration of rtPA in AIS results in the appearance of new CMBs and if the initial load of CMBs is associated with hemorrhagic transformation.
Methods: A total of fifty-nine consecutive patients with AIS treated with rtPA underwent MRI including T2*-weighted Echo Planar Imaging (T2*-EPI) shortly before and 7–9 days after rtPA administration. We calculated the load of new CMBs located outside the MR diffusion restriction area in the follow-up imaging and assessed hemorrhagic transformation with ECASS-II scoring.
Results: A total of forty-nine patients were included for the final analysis. On initial T2*-EPI-GRE, 37 baseline microbleeds (CMBs) were observed in 14 patients (28.6%). On follow-up T2*-EPI-GRE amount of CMBs increased to a total number of 103. New CMBs were found in 5 (14.3%) of 35 patients without and in 9 (64.3%) of 14 with any baseline CMBs. Multiple logistic regression analysis indicated that presence of baseline CMBs (risk ratio [RR] 5.95, 95% CI 2.69–13.20, p < 0.001) and lower platelets level (risk ratio [RR] 0.992, 95% CI 0.986–0.998, p = 0.007) were independently associated with new CMBs. The baseline load of CMBs was not associated with the risk of hemorrhagic transformation.
Conclusion: In this study, new CMBs were found in nearly 30% of patients with AIS on the 7–9 days after rtPA treatment. Baseline CMBs correlated with a higher risk of new CMBs appearing after the rtPA treatment, independently of other factors. At the same time, in our sample, baseline CMBs did not correlate with an increased risk of hemorrhagic transformation. Since the associations between the CMBs load and cognitive impairment have already been proved, further studies are warranted to investigate possible associations between the thrombolytic treatment of patients with AIS, mainly those with baseline CMBs, and the risk of earlier cognitive decline.
Introduction
Intravenous thrombolysis with recombinant tissue plasminogen activator (tPA) is the mainstay therapeutic method of acute ischemic stroke (AIS) with proven clinical benefit (1) and is recommended up to 4.5 h after stroke onset (2). In addition, recent randomized controlled trials and meta-analyses enabled the extension of the time window in selected cases or administration of rtPA in some subjects with unknown onset of stroke (3–5).
The most common complication of rtPA treatment is the hemorrhagic transformation of the ischemic lesion. This transformation might be of various severity that has been classified with ECASS-II score (Table 1) (6). The severity of hemorrhagic transformation is strictly connected with clinical significance and the symptomatic intracerebral hemorrhage (sICH), which occurs in 3–7% of rtPA treated patients, is of the highest clinical interest since it is associated with poor functional outcomes. Symptomatic intracerebral hemorrhage has been well-characterized in the multiple studies (2, 7–9).
Administration of rtPA might also result in another hemorrhagic complication—cerebral microhemorrhages or microbleeds (CMBs). CMBs are small, rounded signal loss lesions surrounded by brain tissue with a diameter up to 5 mm. CMBs might appear remotely from the ischemic lesion in an isolated or diffuse pattern across the brain. The CMBs neuroimaging characteristics are listed in Table 2 (10–13). The prevalence of CMBs is significantly higher in the elderly population with multiple comorbidities and high-total cardiovascular risk, especially hypertension with subsequent hypertensive arteriopathy (13, 14). Usually, CMBs are clinically silent in the terms of acute stroke care but might have a cumulative impact on patients in the following years, mainly because of the associations between the load of CMBs and cognitive impairment (15–20).
Cerebral microbleeds have also been under study because of their possible pathophysiological connection with hemorrhagic transformation as CMBs are commonly treated as markers of increased vascular vulnerability due to severe small vessel disease (21–23). This connection seems plausible also in light of the recent analyses that have revealed that CMBs burden might be a useful single marker of the risk of sICH in patients, after stroke or TIA, receiving oral anticoagulants (OACs) (24, 25). In these reports, CMBs burden was more predictive of sICH than other tools, including HAS-BLED (26).
The problem of rtPA-associated CMBs has already been addressed in a few studies (27–32). However, only four of them were planned to assess true baseline CMBs load with MRI preceding the rtPA administration (28–31). The problem to quantify and compare CMBs before and after the thrombolytic treatment is related to the fact that their proper assessment requires the use of MRI. The long duration of standard MRI testing interferes with the urge of administering the rtPA and thus precludes regular and easy use of this neuroimaging technique in thrombolysed patients.
In the presented study, we investigated whether administration of rtPA in AIS results in the appearance of new CMBs and whether the baseline CMBs load increases the risk of new CMBs and hemorrhagic transformation after the rtPA treatment. The assessment was performed using pre- and post-thrombolysis special MRI protocols.
Methods
Patients and Study Protocol
A total of fifty-nine consecutive patients with AIS treated with intravenous thrombolysis between March 20, 2019 and October 19, 2020 were prospectively enrolled into the study. The study protocol included the following:
(1) head MRI that includes T2*-weighted Echo Planar Imaging (T2*-EPI-GRE) in the protocol before rtPA administration (to exclude ICH);
(2) intravenous thrombolysis, as the main therapy (without mechanical thrombectomy) with standard dosage of rtPA administered either within 4.5 h after stroke onset (the time when a patient was last known to be without symptoms), according to standard dosing protocol approved and recommended for thrombolysis in patients with AIS (33) or with unknown time of onset based on WAKE-UP trial protocol (3) with DWI-FLAIR MRI mismatch (five patients).
(3) Follow-up MRI that includes T2*-EPI-GRE in the protocol, performed on 7–9 days after stroke onset (for therapeutic reasons, in eight cases, follow-up MRI was performed out of the target time frame).
The aforementioned steps constituted the eligibility criteria—patients who could not follow one or more of the steps were not included in the assessments. In addition, the exclusion criterion was the poor quality of obtained neuroimaging data. Forty-nine patients (26 women and 23 men, mean age - 66 years) were included in the final analysis. Three of them had been treated with the non-vitamin K antagonist oral anticoagulant (NOAC) before stroke onset because of atrial fibrillation. However, their laboratory-assessed anticoagulant activity on admission was low (<20 ng/ml) and did not constitute a contraindication for thrombolysis. None of the patients involved in the analysis received any kind of anticoagulation before the follow-up MRI.
All the patients provided informed consent for the involvement in the study. The clinical characteristics of the study group are presented in Table 3.
MRI Protocol and Image Analysis
All MRI examinations were performed with a 1.5-T MRI scanner (Magnetom Aera, Siemens, Erlangen, Germany) with 20 channel head/neck coil in AutoCoil selection mode. Baseline MRI—the “Go-Brain” protocol—designed for the fast imaging in the acute phase of stroke (34), consisted of sagittal T1-weighted gradient recalled echo (GRE), axial T2-weighted turbo spin-echo (TSE), axial T2-weighted TSE fluid-attenuation inversion recovery (FLAIR), axial diffusion-weighted (DWI) single-shot echo-planar imaging (EPI), and axial T2*-weighted EPI-GRE. The high-diagnostic value of ultrafast sequences was presented by Prakkamakul et al. (35). The “Go-Brain” protocol included the “AutoAlign” mode, which uses anatomical landmarks for automated alignment for slice position and prevents erroneous double counting in the follow-up assessment.
The follow-up MRI protocol included axial T2*-weighted EPI-GRE sequences that were used to assess the number of CMBs. Next, where needed the susceptibility-weighted imaging (SWI) was used to confirm CMBs detected on the follow-up T2*-weighted EPI-GRE images. DWI sequence was also used to evaluate baseline (before rtPA administration) infarct volume. Axial T2 FLAIR sequence was used to assess the leukoaraiosis severity in the Fazekas scale (36). Detailed MRI parameters are listed in Table 4.
Cerebral microbleeds neuroimaging characteristics are listed in Table 2 (10–13). CMBs observed in the region of the ischemic lesion were not included in the analysis. CMBs in T2*-weighted EPI-GRE sequences were manually assessed by three observers—a neurologist (BJ) and a radiologist working together with a physicist (ESz, DO). Discrepancies were resolved by consensus and by independent decisions of an experienced stroke neurologist (BK).
Hemorrhagic transformation was graded by the ECASS-II (European Cooperative Acute Stroke Study) classification (6) (Table 1). Symptomatic intracerebral hemorrhage was defined according to SITS-MOST (The Safe Implementation of Thrombolysis in Stroke- Monitoring Study) criteria (37): a type 2 parenchymal hemorrhage (PH2) with deterioration in the National Institutes of Health Stroke Scale (NIHSS) score of 4 points or more, or death.
Clinical Assessment
All the patients enrolled were clinically assessed by an experienced stroke neurologist and classified according to the Trial of Org 10172 in Acute Stroke Treatment (TOAST) classification (38).
Statistics
Interrater agreement for the MRI reading was evaluated using the weighted Cohen's kappa with linear weights and interpreted in the following way: 0.01–0.20 as poor agreement, 0.21–0.40 as fair agreement, 0.41–0.60 as moderate agreement, 0.61–0.80 as substantial agreement, and above 0.80 as almost perfect agreement.
The characteristics of the patients were presented according to mean and standard deviation (SD) for normally distributed continuous data, whereas non-normally distributed variables were described as quartiles; categorical variables were reported as counts and percentages. The between-group differences were evaluated using t-test or Mann-Whitney test for continuous data and the chi-square test or Fisher's exact test for categorical variables. The two-tailed tests were carried out at a significance level of p ≤ 0.05. A generalized linear model assuming Poisson distribution with a log-link function was used to identify risk factors for the incidence of new CMBs. A stepwise forward selection was applied to build up a model with the lowest score of the Akaike information criterion. The final model was recalculated taking into account a robust error variance. Regression coefficients were expressed as adjusted relative risk (aRR) with a 95% CI. All the statistical analyses were performed using the R statistical package (version 3.6.3.).
Results
On initial T2*-EPI-GRE we identified 37 baselines CMBs in 14 patients (28.6%). A total of thirty-four (91.9%) of CMBs were localized in the cortical/subcortical area and only 3 (8.1%) in the deep brain structures. In all but one patient, the number of baseline CMBs did not exceed 3. Interobserver agreement was high and kappa values are presented in Table 5. On follow-up T2*-EPI-GRE, the total amount of CMBs increased up to an absolute number of 103 (all patients combined). New CMBs were found in 5 (14.3%) of 35 patients without baseline CMBs (Figure 1) and in 9 (64.3%) of 14 with CMBs detected in baseline MRI. Among a total of 66 new CMBs, 48 (72.7%) were localized in the cortical/subcortical area, 18 (27.3%) in deep brain structures, and 35 (53.0%) in the ipsilateral hemisphere. Hemorrhagic transformation (ECASS) was observed in 10 (20.4%) patients - 6 HI1, 2 HI2, 1 PH1, and 1 PH2. Baseline CMBs did not correlate significantly with hemorrhagic transformation (p = 0.647).
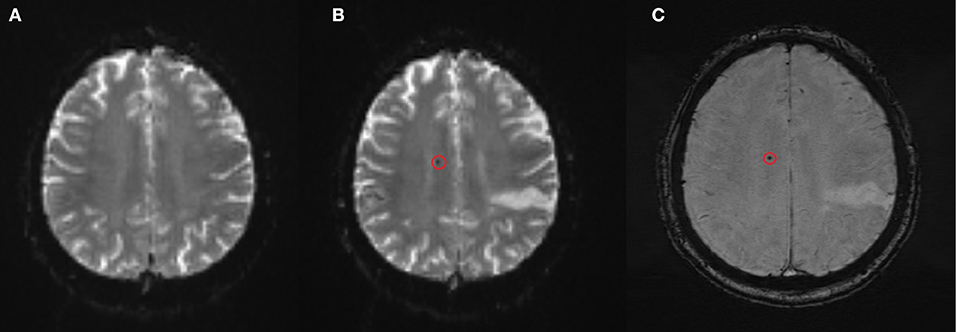
Figure 1. New CMB found on the follow-up MRI on day 7–9 after rtPA treatment (A) baseline T2*-weighted EPI-GRE sequence, (B) follow-up T2*-weighted EPI-GRE, (C) susceptibility-weighted imaging confirmatory slice.
Patients with new CMBs were older (median 72.5, interquartile range (IQR) 66.5, 84.0 vs. 62.0, IQR 46.0, 75.5; p = 0.026), had higher creatinine level (median 1.0, IQR 0.9,1.1 vs. 0.8, IQR 0.7, 0.9; p = 0.035) and more often had higher counts of baseline CMBs: 9 (64.3%) vs. 5 (14.3%), p < 0.001. Other parameters were not statistically significantly different between the groups including pre-existing hypertension, the National Institutes of Health Stroke Scale (NIHSS) admission score, stroke subtype according to TOAST classification, the severity of leukoaraiosis, and baseline DWI lesion volume.
Multiple logistic regression analysis (Table 6) indicated that presence of baseline CMBs (risk ratio 5.95, 95% confidence interval CI 2.69–13.20, p < 0.001) and lower platelets level (risk ratio 0.992, 95% CI 0.986–0.998, p = 0.007) were independently associated with new CMBs.
Discussion
In our patient sample, administration of rtPA resulted in the appearance of new CMBs in nearly 30% of the subjects with AIS, and the baseline CMBs were associated with the higher risk of the new ones appearing after the treatment. However, in this cohort, the baseline CMB load was not related to the increased risk of hemorrhagic transformation, unlike in many of the previous reports (21, 23, 32, 39). This finding seems not to be in line with the pathophysiological connections of CMBs and various vascular pathologies, notably small vessel disease, which in turn is related to increased vascular vulnerability and thus with hemorrhagic transformation—these correlations have been extensively discussed elsewhere (21–23). The reason for this discrepancy might be that these associations may be influenced by many confounding factors connected with the individual characteristics of a patient. CMBs are to be found in “healthy” populations, however, the insight in the available reports prove susceptibility of these populations for numerous future health risks that are additionally modulated by many coexisting factors such as hypertension, smoking, Apo E homozygosity, aspirin intake, white matter lesions or cerebral amyloid angiopathy (40). In addition, it has been reported that the correlation between CMBs and hemorrhagic transformation is not linear and that there is a threshold of baseline CMBs that, only when exceeded, results in higher risks for the transformation, especially for the sICH. The most commonly reported threshold is the > 10 CMBs (41) and the definite majority of our patients presented with not more than 3 baseline CMBs.
One of the future health issues of patients with CMBs is cognitive decline. The available studies suggest strong correlations between CMBs and cognitive impairment (15, 16). The substantial correlation between rtPA treatment and new CMBs found in our study, may have a major implication for the cognitive health of patients with AIS, and thus, longitudinal long-term studies in rt-PA treated patients with AIS are warranted for the need of powerful assessment of potential relations between thrombolytic treatment, CMBs, and cognitive function. This seems to be specifically important in patients with minor and/or lacunar stroke, where thrombolytic treatment does not have that much scientific data, as large vessel occlusion strokes, to prove favorable outcomes (42).
The study was not controlled with a placebo sample and therefore the direct correlation of new CMBs with rtPA treatment cannot be concluded. Additionally, the acute cerebral infarction itself is suggested to promote the development of CMBs (43). However, taking into account other studies on CMBs in acute stroke, and the known rate of the hemorrhagic infarction unrelated to rtPA administration, it is unlikely that this burden value would be as high as about 30% with no connection to rtPA. Furthermore, a strong indication of rtPA-CMBs correlation has been presented in the recent study by Miwa et al., where new CMBs were found only in patients who received rtPA (31). The high percentage of new CMBs in our study is much higher in comparison with other studies that revealed rates in the range of 4–13% (27–31). We believe that it is probably connected with a small sample size that resulted in incidental recruitment of more predisposed patients, as we discussed earlier.
This study has several methodological limitations. Importantly, as discussed earlier, we do not have a control group of non-thrombolysis patients to compare with. Another limitation of this study was the use of a 1.5-T field MR machine which is inferior to a 3-T in detecting CMBs, and the T2*EPI-GRE imaging sequence—much shorter but less sensitive than SWI for CMBs detection (44, 45). To support the lower sensitivity of T2*EPI-GRE sequences, SWI sequences were additionally used to confirm the CMB assessments. Finally, our study encompassed a relatively small sample of subjects, which is, however, similar to many of the reported cohorts. The main reason for the small number of patients is the conflict of interest between the optimal timing of rtPA treatment and the demanding circumstances of MRI testing that is necessary for proper CMBs assessment.
In conclusion, in this study, we present that baseline CMBs burden in patients with AIS is associated with a higher risk of new CMBs after rtPA treatment, but not with the risk of hemorrhagic transformation. New CMBs alone or combined with other selected characteristics (e.g., age, platelet count, or creatine level as indicated in this study) may become a useful predictor of long-term CMBs-related complications of thrombolytic treatment of stroke.
Data Availability Statement
The original contributions presented in the study are included in the article, further inquiries can be directed to the corresponding author.
Ethics Statement
The studies involving human participants were reviewed and approved by the Independent Bioethics Committee for Scientific Research at Medical University of Gdańsk, Poland (Approval No. NKBBN/76/19). The participants provided their written informed consent to participate in this study. Written informed consent was obtained from the individuals for the publication of any potentially identifiable images or data included in this article.
Author Contributions
BJ co-conceptualization of the study, patient recruitment, data assessment and analysis, combined analysis of all data, and writing. AG-G patient recruitment, data assessment and analysis, writing, and editing. DO neuroimaging data collection and assessment and writing. ES neuroimaging data assessment. AW statistical analysis and writing. BR clinical data assessment. BK conceptualization and funding receipt (a major project), writing, review, editing, and coordination. All authors contributed to the article and approved the submitted version.
Funding
Neuroimaging data were part of our project funded by Siemens Heathineers (research grant for the Medical University of Gdansk, PI – BK, 2018; Contract Number C00229230). The authors declare that this study received funding from Siemens Heathineers. The funder was not involved in the study design, collection, analysis, interpretation of data, the writing of this article or the decision to submit it for publication.
Conflict of Interest
The authors declare that the research was conducted in the absence of any commercial or financial relationships that could be construed as a potential conflict of interest.
Publisher's Note
All claims expressed in this article are solely those of the authors and do not necessarily represent those of their affiliated organizations, or those of the publisher, the editors and the reviewers. Any product that may be evaluated in this article, or claim that may be made by its manufacturer, is not guaranteed or endorsed by the publisher.
Acknowledgments
This was a fully independent (–university-designed) project granted from the commercial institution, following the extensive review process. All the procedures within the study were carried out by independent academic researchers and clinicians.
References
1. Emberson J, Lees KR, Lyden P, Blackwell L, Albers G, Bluhmki E, et al. Effect of treatment delay, age, and stroke severity on the effects of intravenous thrombolysis with alteplase for acute ischaemic stroke: a meta-analysis of individual patient data from randomised trials. Lancet. (2014) 384:1929–35. doi: 10.1016/S0140-6736(14)60584-5
2. Hacke W, Kaste M, Bluhmki E, Brozman M, Dávalos A, Guidetti D, et al. Thrombolysis with alteplase 3 to 4.5 hours after acute ischemic stroke. N Engl J Med. (2008) 359:1317–29. doi: 10.1056/NEJMoa0804656
3. Thomalla G, Simonsen CZ, Boutitie F, Andersen G, Berthezene Y, Cheng B, et al. MRI-guided thrombolysis for stroke with unknown time of onset. N Engl J Med. (2018) 379:611–22. doi: 10.1056/NEJMoa1804355
4. Thomalla G, Boutitie F, Ma H, Koga M, Ringleb P, Schwamm LH, et al. Intravenous alteplase for stroke with unknown time of onset guided by advanced imaging: systematic review and meta-analysis of individual patient data. Lancet. (2020) 396:1574–84. doi: 10.1016/S0140-6736(20)32163-2
5. Campbell BCV, Ma H, Ringleb PA, Parsons MW, Churilov L, Bendszus M, et al. Extending thrombolysis to 4·5-9 h and wake-up stroke using perfusion imaging: a systematic review and meta-analysis of individual patient data. Lancet. (2019) 394:139–47. doi: 10.1016/S0140-6736(19)31053-0
6. Larrue V, von Kummer R R, Müller A, Bluhmki E. Risk factors for severe hemorrhagic transformation in ischemic stroke patients treated with recombinant tissue plasminogen activator: a secondary analysis of the European-Australasian Acute Stroke Study (ECASS II). Stroke. (2001) 32:438–41. doi: 10.1161/01.STR.32.2.438
7. IST-3 collaborative group, Sandercock P, Wardlaw JM, Lindley RI, Dennis M, Cohen G, et al. The benefits and harms of intravenous thrombolysis with recombinant tissue plasminogen activator within 6 h of acute ischaemic stroke (the third international stroke trial [IST-3]): a randomised controlled trial. Lancet. (2012) 379:2352–63. doi: 10.1016/S0140-6736(12)60768-5
8. Karaszewski B, Houlden H, Smith EE, Markus HS, Charidimou A, Levi C, et al. What causes intracerebral bleeding after thrombolysis for acute ischaemic stroke? Recent insights into mechanisms and potential biomarkers. J Neurol Neurosurg Psychiatry. (2015) 86:1127–36. doi: 10.1136/jnnp-2014-309705
9. Whiteley WN, Emberson J, Lees KR, Blackwell L, Albers G, Bluhmki E, et al. Risk of intracerebral haemorrhage with alteplase after acute ischaemic stroke: a secondary analysis of an individual patient data meta-analysis. Lancet Neurol. (2016) 15:925–33. doi: 10.1016/S1474-4422(16)30076-X
10. Charidimou A, Werring D. Cerebral microbleeds: detection, mechanisms and clinical challenges. Future Neurol. (2011) 6:587–611. doi: 10.2217/fnl.11.42
11. Greenberg SM, Vernooij MW, Cordonnier C, Viswanathan A, Al-Shahi Salman R, Warach S, et al. Cerebral microbleeds: a guide to detection and interpretation. Lancet Neurol. (2009) 8:165–74. doi: 10.1016/S1474-4422(09)70013-4
12. Wardlaw JM, Smith EE, Biessels GJ, Cordonnier C, Fazekas F, Frayne R, et al. Neuroimaging standards for research into small vessel disease and its contribution to ageing and neurodegeneration. Lancet Neurol. (2013) 12:822–38. doi: 10.1016/S1474-4422(13)70124-8
13. Lee J, Sohn EH, Oh E, Lee AY. Characteristics of cerebral microbleeds. Dement Neurocogn Disord. (2018) 17:73–82. doi: 10.12779/dnd.2018.17.3.73
14. Poels MM, Vernooij MW, Ikram MA, Hofman A, Krestin GP, van der Lugt A, et al. Prevalence and risk factors of cerebral microbleeds: an update of the Rotterdam scan study. Stroke. (2010) 41:S103–6. doi: 10.1161/STROKEAHA.110.595181
15. Yatawara C, Guevarra AC, Ng KP, Chander R, Lam BYK, Wong A, et al. The role of cerebral microbleeds in the incidence of post-stroke dementia. J Neurol Sci. (2020) 412:116736. doi: 10.1016/j.jns.2020.116736
16. Paradise M, Seruga A, Crawford JD, Chaganti J, Thalamuthu A, Kochan NA, et al. The relationship of cerebral microbleeds to cognition and incident dementia in non-demented older individuals. Brain Imaging Behav. (2019) 13:750–761. doi: 10.1007/s11682-018-9883-3
17. Li L, Wu DH, Li HQ, Tan L, Xu W, Dong Q, et al. Association of cerebral microbleeds with cognitive decline: a longitudinal study. J Alzheimers Dis. (2020) 75:571–9. doi: 10.3233/JAD-191257
18. Nakamori M, Hosomi N, Tachiyama K, Kamimura T, Matsushima H, Hayashi Y, et al. Lobar microbleeds are associated with cognitive impairment in patients with lacunar infarction. Sci Rep. (2020) 10:16410. doi: 10.1038/s41598-020-73404-6
19. Haller S, Bartsch A, Nguyen D, Rodriguez C, Emch J, Gold G, et al. Cerebral microhemorrhage and iron deposition in mild cognitive impairment: susceptibility-weighted MR imaging assessment. Radiology. (2010) 257:764–73. doi: 10.1148/radiol.10100612
20. Poels MM, Ikram MA, van der Lugt A, Hofman A, Niessen WJ, Krestin GP, et al. Cerebral microbleeds are associated with worse cognitive function: the Rotterdam Scan Study. Neurology. (2012) 78:326–33. doi: 10.1212/WNL.0b013e3182452928
21. Charidimou A, Shoamanesh A, Wilson D, Gang Q, Fox Z, Jäger HR, et al. Cerebral microbleeds and postthrombolysis intracerebral hemorrhage risk Updated meta-analysis. Neurology. (2015) 85:927–4. doi: 10.1212/WNL.0000000000001923
22. Wilson D, Charidimou A, Ambler G, Fox ZV, Gregoire S, Rayson P, et al. Recurrent stroke risk and cerebral microbleed burden in ischemic stroke and TIA: a meta-analysis. Neurology. (2016) 87:1501. doi: 10.1212/WNL.0000000000003183
23. Zand R, Tsivgoulis G, Singh M, McCormack M, Goyal N, Ishfaq MF, et al. Cerebral microbleeds and risk of intracerebral hemorrhage post intravenous thrombolysis. J Stroke Cerebrovasc Dis. (2017) 26:538–44. doi: 10.1016/j.jstrokecerebrovasdis.2016.11.127
24. Wilson D, Ambler G, Lee KJ, Lim JS, Shiozawa M, Koga M, et al. Cerebral microbleeds and stroke risk after ischaemic stroke or transient ischaemic attack: a pooled analysis of individual patient data from cohort studies. Lancet Neurol. (2019) 18:653–65. doi: 10.1016/S1474-4422(19)30197-8
25. Best JG, Ambler G, Wilson D, Lee KJ, Lim JS, Shiozawa M, et al. Development of imaging-based risk scores for prediction of intracranial haemorrhage and ischaemic stroke in patients taking antithrombotic therapy after ischaemic stroke or transient ischaemic attack: a pooled analysis of individual patient data from cohort studies. Lancet Neurol. (2021) 20:294–303. doi: 10.1016/S1474-4422(21)00024-7
26. Pisters R, Lane DA, Nieuwlaat R, de Vos CB, Crijns HJ, Lip GY, et al. novel user-friendly score (HAS-BLED) to assess 1-year risk of major bleeding in patients with atrial fibrillation: the Euro Heart Survey. Chest. (2010) 138:1093–100. doi: 10.1378/chest.10-0134
27. Jeon SB, Kwon SU, Cho AH, Yun SC, Kim JS, Kang DW. Rapid appearance of new cerebral microbleeds after acute ischemic stroke. Neurology. (2009) 73:1638–44. doi: 10.1212/WNL.0b013e3181bd110f
28. Kimura K, Aoki J, Shibazaki K, Saji N, Uemura J, Sakamoto Y. New appearance of extraischemic microbleeds on T2*-weighted magnetic resonance imaging 24 hours after tissue-type plasminogen activator administration. Stroke. (2013) 44:2776–81. doi: 10.1161/STROKEAHA.113.001778
29. Yan S, Chen Y, Zhang X, Liebeskind DS, Lou M. New microbleeds after thrombolysis: contiguous thin-slice 3T MRI. Medicine. (2014) 93:e99. doi: 10.1097/MD.0000000000000099
30. Braemswig TB, Villringer K, Turc G, Erdur H, Fiebach JB, Audebert HJ, et al. Predictors of new remote cerebral microbleeds after IV thrombolysis for ischemic stroke. Neurology. (2019) 92:e630–8. doi: 10.1212/WNL.0000000000006915
31. Miwa K, Koga M, Inoue M, Yoshimura S, Sasaki M, Yakushiji Y, et al. Cerebral microbleeds development after stroke thrombolysis: A secondary analysis of the THAWS randomized clinical trial. Int J Stroke. (2021) 3:17474930211035023. doi: 10.1177/17474930211035023
32. Capuana ML, Lorenzano S, Caselli MC, Paciaroni M, Toni D. Hemorrhagic risk after intravenous thrombolysis for ischemic stroke in patients with cerebral microbleeds and white matter disease. Neurol Sci. (2021) 42:1969–76. doi: 10.1007/s10072-020-04720-y
33. Powers WJ, Rabinstein AA, Ackerson T, Adeoye OM, Bambakidis NC, et al. Guidelines for the Early Management of Patients With Acute Ischemic Stroke: 2019 Update to the 2018 Guidelines for the Early Management of Acute Ischemic Stroke: A Guideline for Healthcare Professionals From the American Heart Association/American Stroke Association. Stroke. (2019) 50:e344–e418 doi: 10.1161/STR.0000000000000211
34. Rapalino O, Heberlein M, Heberlein K. New strategies for protocol optimization for clinical MRI : rapid examinations and improved patient care. Siemens Magnetom. (2016) 2:22–25.
35. Prakkamakul S, Witzel T, Huang S, Boulter D, Borja MJ, Schaefer P, et al. Ultrafast brain MRI: clinical deployment and comparison to conventional brain MRI at 3T. J Neuroimaging. (2016) 26:503–10. doi: 10.1111/jon.12365
36. Fazekas F, Chawluk JB, Alavi A, Hurtig HI, Zimmerman RA. MR signal abnormalities at 1.5 T in Alzheimer's dementia and normal aging. AJR Am J Roentgenol. (1987) 149:351–6. doi: 10.2214/ajr.149.2.351
37. Wahlgren N, Ahmed N, Dávalos A, Ford GA, Grond M, Hacke W, et al. Thrombolysis with alteplase for acute ischaemic stroke in the Safe Implementation of Thrombolysis in Stroke-Monitoring Study (SITS-MOST): an observational study. Lancet. (2007) 369:275–82. doi: 10.1016/S0140-6736(07)60149-4
38. Adams HP, Bendixen BH, Kappelle LJ, Biller J, Love BB, Gordon DL, et al. Classification of subtype of acute ischemic stroke. Definitions for use in a multicenter clinical trial. TOAST. Trial of Org 10172 in Acute Stroke Treatment. Stroke. (1993) 24:35–41. doi: 10.1161/01.STR.24.1.35
39. Charidimou A, Turc G, Oppenheim C, Yan S, Scheitz JF, Erdur H, et al. Microbleeds, cerebral hemorrhage, and functional outcome after stroke thrombolysis. Stroke. (2017) 48:2084–90. doi: 10.1161/STROKEAHA.116.012992
40. Das AS, Regenhardt RW, Vernooij MW, Blacker D, Charidimou A, Viswanathan A. Asymptomatic cerebral small vessel disease: insights from population-based studies. J Stroke. (2019) 21:121–38. doi: 10.5853/jos.2018.03608
41. Chen J, Duris K, Yang X. Effect of cerebral microbleeds on hemorrhagic transformation and functional prognosis after intravenous thrombolysis of cerebral infarction. Brain Hemorrhages. (2021). doi: 10.1016/j.hest.2021.05.004
42. Karaszewski B, Wyszomirski A, Jabłoński B, Werring DJ, Tomaka D. Efficacy and safety of intravenous rtPA in ischemic strokes due to small-vessel occlusion: systematic review and meta-analysis. Transl Stroke Res. (2021) 12:406–15. doi: 10.1007/s12975-021-00890-9
43. Charidimou A, Kakar P, Fox Z, Werring DJ. Cerebral microbleeds and recurrent stroke risk: systematic review and meta-analysis of prospective ischemic stroke and transient ischemic attack cohorts. Stroke. (2013) 44:995–1001. doi: 10.1161/STROKEAHA.111.000038
44. Cheng AL, Batool S, McCreary CR, Lauzon ML, Frayne R, Goyal M, et al. Susceptibility-weighted imaging is more reliable than T2*-weighted gradient-recalled echo MRI for detecting microbleeds. Stroke. (2013) 44:2782–6. doi: 10.1161/STROKEAHA.113.002267
Keywords: cerebral microbleeds, acute ischemic stroke, thrombolysis, hemorrhagic transformation, neuroimaging, MRI
Citation: Jabłoński B, Gójska-Grymajło A, Ossowska D, Szurowska E, Wyszomirski A, Rojek B and Karaszewski B (2022) New Remote Cerebral Microbleeds on T2*-Weighted Echo Planar MRI After Intravenous Thrombolysis for Acute Ischemic Stroke. Front. Neurol. 12:744701. doi: 10.3389/fneur.2021.744701
Received: 20 July 2021; Accepted: 16 December 2021;
Published: 15 February 2022.
Edited by:
Marios Psychogios, University Hospital of Basel, SwitzerlandReviewed by:
Ozge Altintas Kadirhan, Kirklareli University, TurkeySheng Zhang, Zhejiang Provincial People's Hospital, China
Copyright © 2022 Jabłoński, Gójska-Grymajło, Ossowska, Szurowska, Wyszomirski, Rojek and Karaszewski. This is an open-access article distributed under the terms of the Creative Commons Attribution License (CC BY). The use, distribution or reproduction in other forums is permitted, provided the original author(s) and the copyright owner(s) are credited and that the original publication in this journal is cited, in accordance with accepted academic practice. No use, distribution or reproduction is permitted which does not comply with these terms.
*Correspondence: Bartosz Karaszewski, YmFydG9zeiYjeDAwMDQwO2thcmFzemV3c2tpLm9yZw==