- 1Department of Neurology, Yale University School of Medicine, New Haven, CT, United States
- 2Department of Neurology, University of Iowa Carver College of Medicine, Iowa City, IA, United States
- 3Department of Neurology, University of Utah School of Medicine, Salt Lake City, UT, United States
Endovascular thrombectomy (EVT) has changed the landscape of acute stroke therapy and has become the standard of care for selected patients presenting with anterior circulation large-vessel occlusion (LVO) stroke. Despite successful reperfusion, many patients with LVO stroke do not regain functional independence. Particularly, patients presenting with extremes of blood pressure (BP) or hemodynamic variability are found to have a worse clinical recovery, suggesting blood pressure optimization as a potential neuroprotective strategy. Current guidelines acknowledge the lack of randomized trials to evaluate the optimal hemodynamic management during the immediate post-stroke period. Following reperfusion, lower blood pressure targets may be warranted to prevent reperfusion injury and promote penumbral recovery, but adequate BP targets adjusted to individual patient factors such as degree of reperfusion, infarct size, and overall hemodynamic status remain undefined. This narrative review outlines the physiological mechanisms of BP control after EVT and summarizes key observational studies and clinical trials evaluating post-EVT BP targets. It also discusses novel treatment strategies and areas of future research that could aid in the determination of the optimal post-EVT blood pressure.
Introduction
Large vessel occlusions (LVO) account for approximately one third of ischemic strokes but contribute to more than half of all stroke-related mortality and severe disability (1–3). Over the last several years, LVO strokes are increasingly recognized as unique entities with distinct cerebrovascular pathophysiology and treatment strategies. Acute stroke interventions have traditionally focused on the rapid recanalization of the occluded vessel to restore blood flow to the ischemic tissue. This may be achieved, in a small percentage, by administering intravenous alteplase or, much more effectively, with the use of endovascular thrombectomy (EVT) (4–6). Despite successful reperfusion, LVO stroke patients may continue to have infarct growth, and half do not regain their functional independence, evidencing a need for adjunctive therapies to further improve outcomes (7, 8). Recent evidence suggests that hemodynamic management may play an important role in post-EVT care. While optimal BP targets remain unknown, clinicians must balance the need for sufficient post-thrombectomy blood flow to prevent further infarct growth with the risk of reperfusion injury and hemorrhagic transformation.
This narrative review aims to describe the physiological mechanisms of how BP influences outcome after EVT, provide an overview of observational studies, and discuss completed and ongoing clinical trials of BP management after EVT. We will also discuss recent advances in personalized blood pressure management approaches and suggest potential areas of future research.
Cerebral Autoregulation and Physiologic Considerations of BP Management After EVT
To understand the importance and relevance of blood pressure in acute ischemic stroke, it is helpful to review normal brain vascular physiology and how it can become impaired during ischemia. In a healthy brain, cerebral blood flow (CBF) depends on the pressure gradient between the cerebral arteries and veins. The cerebral arterial pressure is equivalent to the systemic arterial blood pressure (ABP), while the cerebral venous pressure is equivalent to the intracranial pressure (ICP) (9, 10). Cerebral autoregulation is the process in which the brain is able to maintain a steady CBF despite fluctuations in ABP and ICP (9, 11). The mechanisms of autoregulation are not entirely understood but have been shown to involve changes in arteriolar diameter, which directly affects cerebral vascular resistance (CVR) (11). CBF is directly related to ABP and inversely related to CVR, such that CBF = ABP/CVR (12, 13).
Following vessel occlusion, there is a reduction in CBF to the dependent vascular territory. Below a critical threshold of ~10 ml/100 g brain tissue/min, disruption of ion homeostasis and membrane depolarization lead to a loss of neuronal electrical activity (14, 15). Unless blood flow is rapidly restored, the damage becomes irreversible, and cell death occurs. Surrounding the infarct core, there is a zone of hypoperfused, functionally impaired but still viable tissue known as the “ischemic penumbra” (14, 16). The low perfusion pressure in the penumbra creates a pressure gradient that promotes retrograde flow to the ischemic territory (17). The rate of ischemic core growth depends on the duration of ischemia and the degree of collateral blood flow (18).
Ischemia-induced vasoparalysis in the penumbra leads to loss of intrinsic autoregulatory function (19, 20). The decrease in downstream perfusion pressure distal to the occluded vessel, with compensatory dilation of brain arterioles, further impairs autoregulation and causes the CBF to become passively dependent on the ABP (21). The loss of autoregulation after LVO can persist even after revascularization is achieved, rendering the brain vulnerable to fluctuations in perfusion pressure (22, 23). Low ABP can exacerbate cerebral hypoperfusion and cause further ischemic core growth, particularly among patients with incomplete reperfusion. In contrast, elevated ABP may cause excessive flow, cerebral edema, and hemorrhagic conversion (22, 23). Advanced age, greater stroke severity, hyperglycemia, large infarct volume, treatment with alteplase, and poor collateral status have been associated with the development of hemorrhagic transformation and may further increase the risk of reperfusion injury with elevated BP (24, 25). After thrombectomy, the optimal BP requires a careful balance of these opposing concerns to provide the ideal environment for penumbral recovery while avoiding secondary injury from hypo- or hyperperfusion.
Targeted BP therapies aim to maintain BP below or above a fixed threshold. However, BP is one of the most dynamic physiologic variables with significant changes during the early phase after LVO stroke. Sustained increases in BP variability (BPV) may reflect alterations in the mechanisms responsible for cardiovascular homeostasis and represent a potentially modifiable contributor to end-organ damage and poor neurological recovery after LVO stroke (26–30). Although the exact mechanism by which BPV may exert a negative influence remains unknown, direct exposure of the vulnerable oligemic brain tissue to perfusion fluctuations created by elevated systemic BPV may play a critical role. Short-term BP fluctuations can occur due to various internal and external factors, including central sympathetic drive, arterial tone, cardiopulmonary reflexes, humeral mechanisms, blood viscosity, volume status, and medications (31).
Relevant Observational Studies
Current guidelines by the American Heart Association released in 2019 recommend maintaining a BP goal of ≤ 180/105 for the first 24 h after mechanical thrombectomy (32). These guidelines are based on limited evidence and do not account for individual patient factors such as reperfusion status or infarct volume. Sustained elevations of systolic blood pressure (SBP) have been associated with higher rates of symptomatic intracerebral hemorrhage (sICH) and poor functional outcomes (33–36). The Blood pressure After Endovascular Stroke Therapy (BEST) multicenter prospective cohort study of 485 patients found that a peak post EVT SBP of >158 mmHg best discriminated between favorable (mRS 0–2) from unfavorable (mRS 3–6) (37), confirming the results of a smaller, previously published study (33). To further characterize BP trajectories during the first 72 h after thrombectomy, Kodali et al. used a person-centered modeling approach to identify subgroups of patients with similar BP patterns (38). Patients with high and high-to-moderate SBP trajectories had significantly greater odds of an unfavorable outcome compared to those with persistently low SBP post-EVT.
Several studies have suggested that the optimal BP target may depend on the degree of recanalization (35, 39, 40). Mistry et al. retrospectively evaluated a group of 228 patients with LVO stroke and found that higher peak values of systolic blood pressure in the first 24 h after EVT independently correlated with worse 90-day outcomes (41). Interestingly, hemorrhagic complications, a marker of reperfusion injury, were observed at lower mean peak SBP levels among recanalized patients compared to non-recanalized patients (41). However, others consistently found that the best outcomes in patients with successful recanalization occurred at lower blood pressures (36, 39, 40, 42, 43). Recanalized patients (TICI 2b-3) demonstrate a significant spontaneous decrease in SBP over 24 h after EVT (36). The relationship between post-EVT BP and functional outcomes becomes linear, with the most favorable outcomes at an SBP of 110 mmHg (35, 36). In contrast, non-recanalized patients (TICI 0-2a) show a diminished decline in post-EVT BP, and the relationship with functional outcomes is U or J-shaped. Both high and low average post EVT SBP are associated with worse outcomes.
Whether the association between lower post-EVT BP and better functional outcome in patients with successful recanalization is causal or just a reflection of other stroke-related factors, such as better collaterals, remains unclear. However, physiological consideration and the results mentioned above have led many stroke centers to adopt a tiered approach to BP management after thrombectomy stratified by reperfusion status. An online survey regarding BP management post EVT performed across StrokeNet sites in the United States found that most institutions (39%) used a target SBP of 120–139 mmHg on recanalized patients and permissive hypertension on non-recanalized patients (44). However, the complexity of location of vessel occlusion, degree of recanalization, and final infarct volume suggest that a more nuanced approach may be necessary.
Several observational studies have investigated the effect of differing BP treatment protocols after thrombectomy on radiographic and clinical outcomes. Goyal et al. separated patients into three groups based on post-EVT achieved SBPs of <140, <160, and <180 mmHg. Actual BP targets varied and depended on the treating physician's preference and the patient's clinical status. The investigators found that achieving an SBP goal of <160 mmHg during the first 24 h post-EVT was associated with lower odds of mortality than permissive hypertension (34). This study did not find any significant functional outcome differences at 3 months, but results may have been underpowered due to the small sample size. A more recent large multicenter study by Anadani et al. showed that SBP targets of <140 mmHg after successful reperfusion were associated with lower odds of unfavorable outcome and need for hemicraniectomy than SBP targets of <180 mmHg (36, 42).
Besides BP targets, BPV has emerged as an important aspect of post-EVT hemodynamic management. Studies found that BPV is elevated in patients with larger strokes and associated with higher rates of death and disability in stroke patients that did not undergo EVT (30, 45). Furthermore, antihypertensive drug classes have differing effects on interindividual variation in blood pressure. Calcium-channel blockers such as amlodipine (oral) and nicardipine (intravenous) have been shown to reduce BPV, while b-blocker may increase it (46, 47). Several recent studies have focused on the relationship between BPV and outcome in patients receiving EVT. While there was significant heterogeneity in the frequency and duration of measurements as well as BPV parameters, high BPV was consistently associated with a reduced likelihood of neurological recovery (29, 30, 48).
The effect of reperfusion status on the relationship between BPV and outcome is more controversial. After incomplete reperfusion, higher infarct volumes with surrounding ischemic tissue may make patients more vulnerable to BP fluctuations. Conversely, successful recanalization exposes friable brain tissue to changes in perfusion pressure, potentially increasing the risk of reperfusion injury. While one of the early studies showed the association between BPV and unfavorable outcome appeared strongest among patients with incomplete recanalization (29), others found the opposite effect (49–51). In a post-hoc analysis of the BEST study, patients were stratified by recanalization status (TICI 0-2a, 2b, and 3), and high BPV was associated with poor outcomes for patients with TICI 3 exclusively (37). Similarly, another study showed that the effects of higher BPV on outcomes were more pronounced among patients with better reperfusion status (52). A link between higher BP variability and an increased risk of reperfusion injury is supported by Kim et al. They found that high BP variability in the first 24 h following successful EVT was associated with an increased risk of symptomatic intracerebral hemorrhage (53). However, other investigators could not confirm this association (51).
Whether the relationship between high BPV and outcome is causative or an epiphenomenon of stroke-related factors and associated physiologic stressors remains unknown. Chang et al. demonstrated an inverse relationship between BPV parameters and the degree of recanalization (28). They hypothesized that lower recanalization success might increase the chances of insular and adjacent tissue destruction resulting in sympathetic overactivity. However, despite solid conceptual reasons to support a link between BPV and infarct location, other investigators found no association between BPV and injury to the structures of the central autonomic network, arguing against a central origin (51).
While evidence suggests that maintaining a stable BP with low variability after successful thrombectomy may be as important as controlling mean peak BP values, there has not been a clinical trial dedicated to reducing BPV in stroke patients. A summary of observational trials can be found in Table 1.
Clinical Trials Evidence
The recently published Blood Pressure Target in Acute Stroke to Reduce hemorrhaGe After Endovascular Therapy (BP TARGET) was a prospective, randomized, multicenter, controlled, open-label trial aimed to evaluate if BP control with a goal of 100–129 mmHg could reduce the incidence of sICH and improve functional outcomes compared to a goal of 130–185 mmHg in successfully recanalized post-EVT patients (49). A total of 324 patients were enrolled and randomly assigned to either group within 1 h after EVT. Target BP range was maintained for 24 h after EVT. The choice of the antihypertensive agent was at the discretion of the medical provider. To assess the occurrence of sICH, a CT scan was obtained within 24–36 h of EVT. The study did not show any difference in functional outcome or reduction in the rates of sICH with more intensive BP control. Mazighi et al. (50) However, adherence to the assigned BP target was suboptimal, with only 50% of patients in the intense target group achieving the BP goal of 100–129 at 3 h. Moreover, the median percentage of time in range was only 61% for the 130–185 mmHg group and 66% for the 100–129 mmHg group. The study protocol required a time within the assigned BP range of at least 80%. These results indicate that despite setting SBP targets, post-EVT blood pressure may be difficult to control in clinical practice. Furthermore, the frequent administration of antihypertensive medication with more intensive BP control could have induced higher BPV, potentially offsetting the benefits of BP lowering.
Several ongoing prospective randomized control trials are further evaluating the impact of BP after successful EVT. The Blood pressure After Endovascular Stroke Therapy (BEST-II, NCT04116112) is a phase 2 clinical trial that randomly assigns patients with TICI 2b-3 to BP targets of ≤140, ≤160, and ≤180 mmHg. These BPs will be maintained for 24-h post-EVT, and the outcomes measures will be final infarct volume at 36-h and 3-month mRS. The Outcome in patients Treated with Intraarterial Thrombectomy- Optimal Blood Pressure Control (OPTIMAL_BP; NCT04205305) is a clinical trial evaluating the impact of SBP targets <140 vs. <180 mmHg on 36-h sICH, 90-day mRS, and mortality on patients with successful reperfusion after EVT. Similarly, the Second Enhanced Control of Hypertension and Thrombectomy Stroke Study (ENCHANTED 2; NCT04140110) trial will assign patients to an SBP target of <120 vs. 140–180 mmHg during the first 72 h after EVT to evaluate the effect on 90-day mRS. Finally, the Invasive Control of Blood Pressure in Acute Ischemic Stroke After Endovascular Therapy on Clinical Outcomes (CRISIS I; NCT04775147) trial will compare SBP targets of <140 and <120 mmHg on 90-day sICH and mRS. All ongoing clinical trials will evaluate patients with successful EVT with TICI 2b-3, which is achieved in over 80% of patients with modern EVT techniques. The results of these trials will provide much-needed high-quality evidence and inform blood pressure management after thrombectomy. A summary of completed and ongoing prospective studies can be found in Table 2.
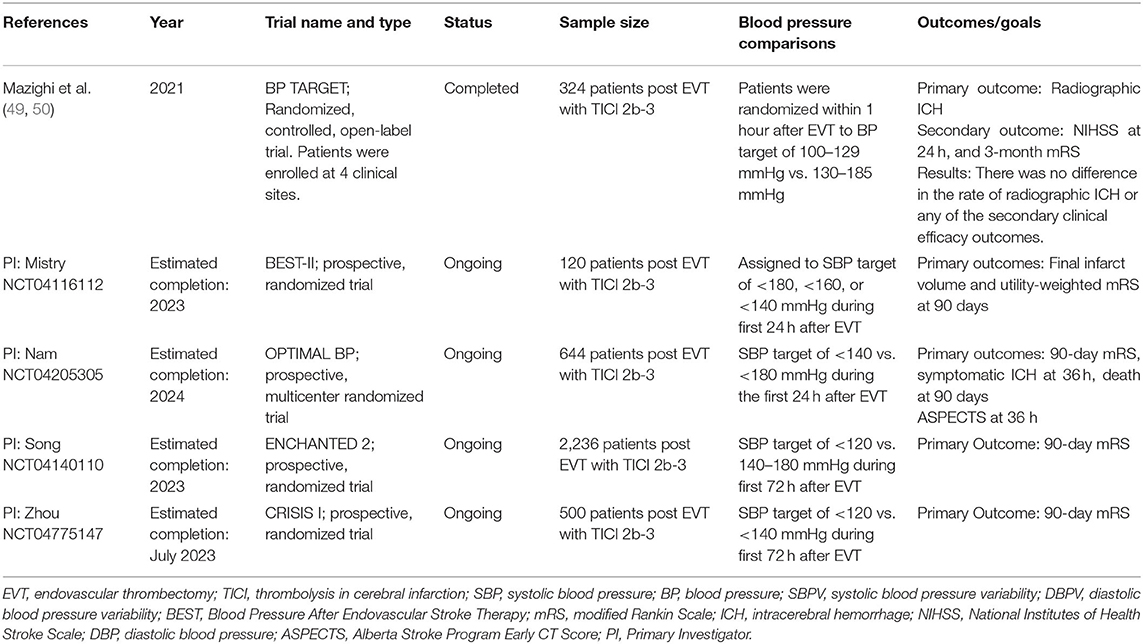
Table 2. Summary of completed and ongoing prospective studies evaluating post-EVT static BP and dynamic BP (BPV).
Individualized BP Targets
Despite the consistent findings of better outcomes with lower post-thrombectomy BP, reducing BP to the same fixed target may be an oversimplification of the complex physiology. The neutral results of the BP TARGET trial suggest that stratification by reperfusion status may be inadequate for the heterogenous EVT patient population. A one-size-fits-all approach does not account for individual patient factors such a collateral status, infarct size, or history of hypertension. For example, a patient with chronic hypertension may have a rightward shifted autoregulatory curve (58), and aggressive BP lowering could result in cerebral hypoperfusion and infarct expansion. Similarly, a patient with TICI 2b reperfusion may still have significant residual tissue at risk and thus require different BP targets post-EVT than a patient with TICI 3 reperfusion. In contrast, a patient with a large infarct despite successful EVT who also received intravenous thrombolytics is at increased risk of reperfusion injury related to hyperperfusion of vascular beds with failed autoregulation. This patient may require strict BP control. In clinical practice, not all thrombectomy patients are treated equally, and survey data indicate that physicians often select BP parameters on a per-patient basis (48). While it may be reasonable to aim for a lower BP, particularly after successful reperfusion, the optimal target remains unknown.
Besides maintaining BP below a fixed, predetermined value, there is a strong rationale for a more personalized BP management. We recently compared fixed vs. personalized autoregulation-oriented BP thresholds after EVT (59). Near-infrared spectroscopy-derived tissue oxygenation (NIRS) was used as a surrogate of CBF and compared to changes in mean arterial pressure (MAP) to calculate the autoregulatory index and the BP range at which autoregulation is most preserved. This study showed that exceeding this blood pressure range was associated with an increased risk of hemorrhagic transformation and worse functional outcomes. Using the same dataset, the association was not seen when applying fixed blood pressure thresholds. Currently, there are no clinical trials to support autoregulation-guided BP management as a potential post-EVT neuroprotective strategy. An impediment to testing autoregulation-guided blood pressure management has been the complex task of acquiring, integrating, and real-time processing of high-frequency physiologic data. Commercially available software and hardware solutions are emerging, which may help to implement a personalized approach to BP management in the clinical setting. A phase II clinical trial of autoregulation-oriented BP management after severe TBI has recently been completed, and the results are expected soon. The study will provide further data regarding the feasibility of a personalized BP management approach that would also apply to other forms of acute brain injury such as ischemic stroke.
Another easily implementable and generalizable approach to personalized BP management is currently being tested in the Effect of Individualized vs. Standard Blood Pressure Management during Mechanical Thrombectomy for Anterior Ischemic Stroke Trial (DETERMINE; NCT04352296). The study will compare maintaining the BP within 10% of the first MAP measured in the angiography suite vs. a fixed blood pressure goal of 140–180 mmHg. Selecting BP targets based on an individual patients' baseline BP could represent a valid method to incorporate patient-specific factors without the need for advanced monitoring. A similar approach could potentially also be implemented in the post-EVT setting.
Future Directions
Management of hemodynamics in the post-EVT patient is complex and requires careful BP control to ensure adequate CBF to supply the ischemic penumbra while avoiding reperfusion injury. Several clinical trials are underway to evaluate different fixed BP thresholds after EVT with successful recanalization. These studies will provide insight and guidance on the optimal post-EVT BP target. Still, future post-hoc analyses of these trials will be necessary to assess if stroke or patient characteristics have any effect on outcome.
Patients with high SBP trajectories during the first 24 h after mechanical thrombectomy are at increased risk of an unfavorable outcome. Future research should focus on the early identification of patients with high-risk trajectories that are most likely to benefit from intense post-EVT BP management. Autoregulation-guided blood pressure management may present an elegant alternative over the classical approach of maintaining blood pressure below a fixed, predetermined value. Clinical trials are needed to test autoregulation-based treatment strategies, including tailored pharmacologic blood pressure augmentation and lowering therapies based on patients' real-time autoregulatory status. Parallel lines of inquiry have begun in Europe targeting autoregulation in traumatic brain injury, further suggesting that this approach is timely and feasible in a multicenter clinical trial.
Other approaches to post-EVT BP management include the reduction of potentially harmful BP fluctuations. There is ample evidence showing that higher BPV is associated with worse functional outcomes and increased ICH risk. Although it is unclear if this is causative or an epiphenomenon related to the stroke itself, studies aiming to decrease BPV should be pursued (28, 29, 51, 53). The main challenge of assessing and manipulating BPV is the lack of bedside techniques to measure BPV in real-time and determine ideal candidates for clinical trials. Short-term BP variability has traditionally been measured as minute-to-minute or hour-to-hour oscillations. It requires a minimum 24-h period, which limits its usefulness for bedside clinical decision making (29, 51–53). One promising approach that warrants further investigation is the use of spectral analysis of beat-to-beat blood pressure to detect harmful patterns of BP variability within minutes (60). Ferreira et al. performed 5-min BP recordings recorded using non-invasive finger plethysmography immediately following TICI 2b-3 revascularization and found that a high-frequency BPV was correlated with lower rates of early neurological recovery and worse functional outcomes (60).
Cerebral perfusion optimization in the immediate post-EVT period is a research priority in acute stroke. Current research is focused on elucidating the best hemodynamic biomarker for defining and monitoring the ideal BP target after stroke. Results from ongoing and future clinical trials will be critical to reduce reperfusion injury and maximize neurological recovery for all stroke patients undergoing endovascular therapy.
Author Contributions
NP conceived the review. TP drafted the manuscript. SO-G, AH, and NP made critical revisions to the manuscript. All authors contributed to the article and approved the submitted version.
Funding
NP was supported by the NIH (K23NS110980).
Conflict of Interest
The authors declare that the research was conducted in the absence of any commercial or financial relationships that could be construed as a potential conflict of interest.
Publisher's Note
All claims expressed in this article are solely those of the authors and do not necessarily represent those of their affiliated organizations, or those of the publisher, the editors and the reviewers. Any product that may be evaluated in this article, or claim that may be made by its manufacturer, is not guaranteed or endorsed by the publisher.
References
1. Malhotra K, Gornbein J, Saver JL. Ischemic strokes due to large-vessel occlusions contribute disproportionately to stroke-related dependence and death: a review. Front Neurol. (2017) 8:651. doi: 10.3389/fneur.2017.00651
2. Smith WS, Lev MH, English JD, Camargo EC, Chou M, Johnston SC, et al. Significance of large vessel intracranial occlusion causing acute ischemic stroke and TIA. Stroke. (2009) 40:3834–40. doi: 10.1161/STROKEAHA.109.561787
3. Chen CJ, Ding D, Starke RM, Mehndiratta P, Crowley RW, Liu KC, et al. Endovascular vs medical management of acute ischemic stroke. Neurology. (2015) 85:1980–90. doi: 10.1212/WNL.0000000000002176
4. Jovin TG, Chamorro A, Cobo E, de Miquel MA, Molina CA, Rovira A, et al. Thrombectomy within 8 hours after symptom onset in ischemic stroke. N Engl J Med. (2015) 372:2296–306. doi: 10.1056/NEJMoa1503780
5. Saver JL, Goyal M, Bonafe A, Diener HC, Levy EI, Pereira VM, et al. Stent-retriever thrombectomy after intravenous t-PA vs. t-PA alone in stroke. N Engl J Med. (2015) 372:2285–95. doi: 10.1056/NEJMoa1415061
6. Bracard S, Ducrocq X, Mas JL, Soudant M, Oppenheim C, Moulin T, et al. Mechanical thrombectomy after intravenous alteplase versus alteplase alone after stroke (THRACE): a randomised controlled trial. Lancet Neurol. (2016) 15:1138–47. doi: 10.1016/S1474-4422(16)30177-6
7. Goyal M, Menon BK, van Zwam WH, Dippel DW, Mitchell PJ, Demchuk AM, et al. Endovascular thrombectomy after large-vessel ischaemic stroke: a meta-analysis of individual patient data from five randomised trials. Lancet. (2016) 387:1723–31. doi: 10.1016/S0140-6736(16)00163-X
8. Regenhardt RW, Etherton MR, Das AS, Schirmer MD, Hirsch JA, Stapleton CJ, et al. Infarct growth despite endovascular thrombectomy recanalization in large vessel occlusive stroke. J Neuroimaging. (2021) 31:155–64. doi: 10.1111/jon.12796
10. Ursino M, Lodi CA. A simple mathematical model of the interaction between intracranial pressure and cerebral hemodynamics. J Appl Physiol. (1997) 82:1256–69. doi: 10.1152/jappl.1997.82.4.1256
11. Somers VK, Mark AL, Zavala DC, Abboud FM. Contrasting effects of hypoxia and hypercapnia on ventilation and sympathetic activity in humans. J Appl Physiol. (1985) (1989) 67:2101–6. doi: 10.1152/jappl.1989.67.5.2101
12. Rosner MJ. Introduction to cerebral perfusion pressure management. Neurosurg Clin N Am. (1995) 6:761–73.
13. Silverman A, Petersen NH. Physiology Cerebral Autoregulation. Treasure Island, FL: StatPearls (2021).
14. Astrup J, Siesjo BK, Symon L. Thresholds in cerebral ischemia - the ischemic penumbra. Stroke. (1981) 12:723–5. doi: 10.1161/01.STR.12.6.723
15. Baron JC. Perfusion thresholds in human cerebral ischemia: historical perspective and therapeutic implications. Cerebrovasc Dis. (2001) 11:2–8. doi: 10.1159/000049119
16. Schaefer PW, Barak ER, Kamalian S, Gharai LR, Schwamm L, Gonzalez RG, et al. Quantitative assessment of core/penumbra mismatch in acute stroke: CT and MR perfusion imaging are strongly correlated when sufficient brain volume is imaged. Stroke. (2008) 39:2986–92. doi: 10.1161/STROKEAHA.107.513358
17. Regenhardt RW, Das AS, Stapleton CJ, Chandra RV, Rabinov JD, Patel AB, et al. Blood pressure and penumbral sustenance in stroke from large vessel occlusion. Front Neurol. (2017) 8:317. doi: 10.3389/fneur.2017.00317
18. Lin L, Yang J, Chen C, Tian H, Bivard A, Spratt NJ, et al. Association of collateral status and ischemic core growth in patients with acute ischemic stroke. Neurology. (2021) 96:e161–70. doi: 10.1212/WNL.0000000000011258
19. Olsen TS, Larsen B, Herning M, Skriver EB, Lassen NA. Blood flow and vascular reactivity in collaterally perfused brain tissue. Evidence of an ischemic penumbra in patients with acute stroke. Stroke. (1983) 14:332–41.
20. Dohmen C, Bosche B, Graf R, Reithmeier T, Ernestus RI, Brinker G, et al. Identification and clinical impact of impaired cerebrovascular autoregulation in patients with malignant middle cerebral artery infarction. Stroke. (2007) 38:56–61. doi: 10.1161/01.STR.0000251642.18522.b6
21. Eames PJ, Blake MJ, Dawson SL, Panerai RB, Potter JF. Dynamic cerebral autoregulation and beat to beat blood pressure control are impaired in acute ischaemic stroke. J Neurol Neurosurg Psychiatry. (2002) 72:467–72. doi: 10.1136/jnnp.72.4.467
22. Petersen NH, Ortega-Gutierrez S, Reccius A, Masurkar A, Huang A, Marshall RS. Dynamic cerebral autoregulation is transiently impaired for one week after large-vessel acute ischemic stroke. Cerebrovasc Dis. (2015) 39:144–50. doi: 10.1159/000368595
23. Xiong L, Liu X, Shang T, Smielewski P, Donnelly J, Guo ZN, et al. Impaired cerebral autoregulation: measurement and application to stroke. J Neurol Neurosurg Psychiatry. (2017) 88:520–31. doi: 10.1136/jnnp-2016-314385
24. Nogueira RG, Gupta R, Jovin TG, Levy EI, Liebeskind DS, Zaidat OO, et al. Predictors and clinical relevance of hemorrhagic transformation after endovascular therapy for anterior circulation large vessel occlusion strokes: a multicenter retrospective analysis of 1122 patients. J Neurointerv Surg. (2015) 7:16–21. doi: 10.1136/neurintsurg-2013-010743
25. Charbonnier G, Bonnet L, Biondi A, Moulin T. Intracranial bleeding after reperfusion therapy in acute ischemic stroke. Front Neurol. (2020) 11:629920. doi: 10.3389/fneur.2020.629920
26. Stevens SL, Wood S, Koshiaris C, Law K, Glasziou P, Stevens RJ, et al. Blood pressure variability and cardiovascular disease: systematic review and meta-analysis. BMJ. (2016) 354:i4098. doi: 10.1136/bmj.i4098
27. Rothwell PM. Limitations of the usual blood-pressure hypothesis and importance of variability, instability, episodic hypertension. Lancet. (2010) 375:938–48. doi: 10.1016/S0140-6736(10)60309-1
28. Chang JY, Jeon SB, Lee JH, Kwon OK, Han MK. The relationship between blood pressure variability, recanalization degree, and clinical outcome in large vessel occlusive stroke after an intra-arterial thrombectomy. Cerebrovasc Dis. (2018) 46:279–86. doi: 10.1159/000495300
29. Bennett AE, Wilder MJ, McNally JS, Wold JJ, Stoddard GJ, Majersik JJ, et al. Increased blood pressure variability after endovascular thrombectomy for acute stroke is associated with worse clinical outcome. J Neurointerv Surg. (2018) 10:823–27. doi: 10.1136/neurintsurg-2017-013473
30. Manning LS, Rothwell PM, Potter JF, Robinson TG. Prognostic significance of short-term blood pressure variability in acute stroke: systematic review. Stroke. (2015) 46:2482–90. doi: 10.1161/STROKEAHA.115.010075
31. Parati G, Torlasco C, Pengo M, Bilo G, Ochoa JE. Blood pressure variability: its relevance for cardiovascular homeostasis and cardiovascular diseases. Hypertens Res. (2020) 43:609–20. doi: 10.1038/s41440-020-0421-5
32. Powers WJ, Rabinstein AA, Ackerson T, Adeoye OM, Bambakidis NC, Becker K, et al. Guidelines for the early management of patients with acute ischemic stroke: 2019 update to the 2018 guidelines for the early management of acute ischemic stroke: a guideline for healthcare professionals from the American Heart Association/American Stroke Association. Stroke. (2019) 50:e344–418. doi: 10.1161/STR.0000000000000211
33. Maier IL, Tsogkas I, Behme D, Bahr M, Knauth M, Psychogios MN, et al. High systolic blood pressure after successful endovascular treatment affects early functional outcome in acute ischemic stroke. Cerebrovasc Dis. (2018) 45:18–25. doi: 10.1159/000484720
34. Goyal N, Tsivgoulis G, Pandhi A, Chang JJ, Dillard K, Ishfaq MF, et al. Blood pressure levels post mechanical thrombectomy and outcomes in large vessel occlusion strokes. Neurology. (2017) 89:540–7. doi: 10.1212/WNL.0000000000004184
35. Martins AI, Sargento-Freitas J, Silva F, Jesus-Ribeiro J, Correia I, Gomes JP, et al. Recanalization modulates association between blood pressure and functional outcome in acute ischemic stroke. Stroke. (2016) 47:1571–6. doi: 10.1161/STROKEAHA.115.012544
36. Matusevicius M, Cooray C, Bottai M, Mazya M, Tsivgoulis G, Nunes AP, et al. Blood pressure after endovascular thrombectomy: modeling for outcomes based on recanalization status. Stroke. (2020) 51:519–25. doi: 10.1161/STROKEAHA.119.026914
37. Mistry EA, Mehta T, Mistry A, Arora N, Starosciak AK, De Los Rios La Rosa F, et al. Blood pressure variability and neurologic outcome after endovascular thrombectomy: a secondary analysis of the BEST study. Stroke. (2020) 51:511–8. doi: 10.1161/STROKEAHA.19.027549
38. Kodali S, Meng C, Nguyen CK, Peshwe K, Silverman A, Strander S, et al. ESO-WSO 2020 Joint meeting abstracts- multi-trajectory modeling of blood pressure after thrombectomy: a multicenter analysis of individual patient blood pressure data. Int J Stroke. (2020) 15:3–752. doi: 10.1177/1747493020963387
39. McCarthy DJ, Ayodele M, Luther E, Sheinberg D, Bryant JP, Elwardany O, et al. Prolonged heightened blood pressure following mechanical thrombectomy for acute stroke is associated with worse outcomes. Neurocrit Care. (2020) 32:198–205. doi: 10.1007/s12028-019-00803-7
40. Chang JY, Han MK. Postthrombectomy systolic blood pressure and clinical outcome among patients with successful recanalization. Eur Neurol. (2019) 81:216–22. doi: 10.1159/000502519
41. Mistry EA, Mistry AM, Nakawah MO, Khattar NK, Fortuny EM, Cruz AS, et al. Systolic blood pressure within 24 hours after thrombectomy for acute ischemic stroke correlates with outcome. J Am Heart Assoc. (2017) 6:e006167. doi: 10.1161/JAHA.117.006167
42. Anadani M, Orabi MY, Alawieh A, Goyal N, Alexandrov AV, Petersen N, et al. Blood pressure and outcome after mechanical thrombectomy with successful revascularization. Stroke. (2019) 50:2448–54. doi: 10.1161/STROKEAHA.118.024687
43. Choi KH, Kim JM, Kim JH, Kim JT, Park MS, Choi SM, et al. Optimal blood pressure after reperfusion therapy in patients with acute ischemic stroke. Sci Rep. (2019) 9:5681. doi: 10.1038/s41598-019-42240-8
44. Mistry EA, Mayer SA, Khatri P. Blood pressure management after mechanical thrombectomy for acute ischemic stroke: a survey of the strokenet sites. J Stroke Cerebrovasc Dis. (2018) 27:2474–8. doi: 10.1016/j.jstrokecerebrovasdis.2018.05.003
45. de Havenon, Bennett A, Stoddard GJ, Smith G, Chung L, O'Donnell S, et al. Determinants of the impact of blood pressure variability on neurological outcome after acute ischaemic stroke. Stroke Vasc Neurol. (2017) 2:1–6. doi: 10.1136/svn-2016-000057
46. Webb AJ, Fischer U, Mehta Z, Rothwell PM. Effects of antihypertensive-drug class on interindividual variation in blood pressure and risk of stroke: a systematic review and meta-analysis. Lancet. (2010) 375:906–15. doi: 10.1016/S0140-6736(10)60235-8
47. Webb AJ, Rothwell PM. Effect of dose and combination of antihypertensives on interindividual blood pressure variability: a systematic review. Stroke. (2011) 42:2860–5. doi: 10.1161/STROKEAHA.110.611566
48. Vitt JR, Trillanes M, Hemphill JC. Management of blood pressure during and after recanalization therapy for acute ischemic stroke. Front Neurol. (2019) 10:138. doi: 10.3389/fneur.2019.00138
49. Mazighi M, Labreuche J, Richard S, Gory B, Lapergue B, Sibon I, et al. Blood pressure target in acute stroke to reduce hemorrhage after endovascular therapy: the randomized BP TARGET study protocol. Front Neurol. (2020) 11:480. doi: 10.3389/fneur.2020.00480
50. Mazighi M, Richard S, Lapergue B, Sibon I, Gory B, Berge J, et al. Safety and efficacy of intensive blood pressure lowering after successful endovascular therapy in acute ischaemic stroke (BP-TARGET): a multicentre, open-label, randomised controlled trial. Lancet Neurol. (2021) 20:265–74. doi: 10.1016/S1474-4422(20)30483-X
51. Cho BH, Kim JT, Lee JS, Park MS, Kang KW, Choi KH, et al. Associations of various blood pressure parameters with functional outcomes after endovascular thrombectomy in acute ischaemic stroke. Eur J Neurol. (2019) 26:1019–27. doi: 10.1111/ene.13951
52. Huang X, Guo H, Yuan L, Cai Q, Zhang M, Zhang Y, et al. Blood pressure variability and outcomes after mechanical thrombectomy based on the recanalization and collateral status. Ther Adv Neurol Disord. (2021) 14:1756286421997383. doi: 10.1177/1756286421997383
53. Kim TJ, Park HK, Kim JM, Lee JS, Park SH, Jeong HB, et al. Blood pressure variability and hemorrhagic transformation in patients with successful recanalization after endovascular recanalization therapy: A retrospective observational study. Ann Neurol. (2019) 85:574–81. doi: 10.1002/ana.25434
54. Goyal N, Tsivgoulis G, Pandhi A, Dillard K, Alsbrook D, Chang JJ, et al. Blood pressure levels post mechanical thrombectomy and outcomes in non-recanalized large vessel occlusion patients. J Neurointerv Surg. (2018) 10:925–31. doi: 10.1136/neurintsurg-2017-013581
55. Anadani M, Orabi Y, Alawieh A, Chatterjee A, Lena J, Al Kasab S, et al. Blood pressure and outcome post mechanical thrombectomy. J Clin Neurosci. (2019) 62:94–9. doi: 10.1016/j.jocn.2018.12.011
56. Mistry EA, Sucharew H, Mistry AM, Mehta T, Arora N, Starosciak AK, et al. Blood pressure after endovascular therapy for ischemic stroke (BEST): a multicenter prospective cohort study. Stroke. (2019) 50:3449–55. doi: 10.1161/STROKEAHA.119.026889
57. Anadani M, Arthur AS, Tsivgoulis G, Simpson KN, Alawieh A, Orabi Y, et al. Blood pressure goals and clinical outcomes after successful endovascular therapy: a multicenter study. Ann Neurol. (2020) 87:830–9. doi: 10.1002/ana.25716
58. Strandgaard S. Autoregulation of cerebral blood flow in hypertensive patients. The modifying influence of prolonged antihypertensive treatment on the tolerance to acute, drug-induced hypotension. Circulation. (1976) 53:720–7.
59. Petersen NH, Silverman A, Strander SM, Kodali S, Wang A, Sansing LH, et al. Fixed compared with autoregulation-oriented blood pressure thresholds after mechanical thrombectomy for ischemic stroke. Stroke. (2020) 51:914–21. doi: 10.1161/STROKEAHA.119.026596
Keywords: blood pressure, thrombectomy, stroke, cerebral autoregulation, neurocritical care
Citation: Peng TJ, Ortega-Gutiérrez S, de Havenon A and Petersen NH (2021) Blood Pressure Management After Endovascular Thrombectomy. Front. Neurol. 12:723461. doi: 10.3389/fneur.2021.723461
Received: 10 June 2021; Accepted: 12 August 2021;
Published: 03 September 2021.
Edited by:
Roland Faigle, Johns Hopkins University, United StatesReviewed by:
Robert William Regenhardt, Massachusetts General Hospital and Harvard Medical School, United StatesFeifei Ma, Vall d'Hebron Research Institute (VHIR), Spain
Copyright © 2021 Peng, Ortega-Gutiérrez, de Havenon and Petersen. This is an open-access article distributed under the terms of the Creative Commons Attribution License (CC BY). The use, distribution or reproduction in other forums is permitted, provided the original author(s) and the copyright owner(s) are credited and that the original publication in this journal is cited, in accordance with accepted academic practice. No use, distribution or reproduction is permitted which does not comply with these terms.
*Correspondence: Nils H. Petersen, nils.petersen@yale.edu