- 1Department of Neurology, Affiliated Jinling Hospital, Medical School of Nanjing University, Nanjing, China
- 2Department of Neurology, Jinling Hospital, Medical School of Southeast University, Nanjing, China
- 3Department of Neurology, Jinling Hospital, The First School of Clinical Medicine, Southern Medical University, Nanjing, China
Objective: To determine the clinical significance of post-procedural abnormal liver function test (ALFT) on the functional outcomes at 90 days in acute ischemic stroke (AIS) treated with mechanical thrombectomy (MT).
Methods: In this retrospective observational study, patients with AIS undergoing MT were enrolled from the Nanjing Stroke Registry Program and the multicenter Captor trial. A favorable outcome was defined as a modified Rankin Scale score 0–2 at 90 days. Predictive models were established by multivariable logistic regression. Improved predictive value of models was assessed by continuous net reclassification improvement (NRI) and integrated discrimination improvement (IDI). In addition, multivariable logistic regression and restricted cubic spline were used to analyze dose–response correlations between the severity of ALFT and prognosis.
Results: Among 420 patients enrolled, 234 (55.7%) patients were diagnosed as post-procedural ALFT after MT. Patients with post-procedural ALFT had higher National Institute of Health Stroke Scale score on admission (median, 18 vs. 15, p < 0.001) and more pneumonia (65.4 vs. 38.2%, p < 0.001) than those without post-procedural ALFT. Post-procedural ALFT, rather than preprocedural ALFT, was independently associated with favorable outcome (adjusted odds ratio, 0.48; 95% CI 0.28–0.81; p = 0.006). The improvement of predictive model after adding post-procedural ALFT was significant [continuous NRI (value, 0.401; p < 0.001), IDI (value, 0.013; p < 0.001)]. However, the restricted cubic spline indicated no evidence of a dose–response relationship between the severity of post-procedural ALFT and prognosis.
Conclusions: In AIS patients treated by MT, post-procedural ALFT was associated with more severe stroke and served as an independent predictor of worse prognosis at 90 days.
Introduction
Mechanical thrombectomy (MT) is a well-established treatment for acute ischemic stroke (AIS) caused by large vessel occlusions (LVO) (1). However, regardless of the proven validity of MT, only approximately 40% of patients undergoing MT could achieve functional independence at 90 days (1, 2). Despite the growing attention, factors affecting clinical outcomes have not been fully recognized yet. Conventional factors including age, baseline disability, history of hypertension, and hyperglycemia were known to be associated with worse prognosis in AIS patients treated with MT (3). Besides, radiological markers of chronic brain damage, such as cortical microinfarcts (4), leukoaraiosis (5), brain atrophy (6), and cerebral microbleeds (7), have recently been identified as risk factors of poor clinical outcomes. Comorbidities and complications such as malignant brain edema (8), pneumonia (9), and renal dysfunction (10) were reported as potential risk factors for worse prognosis, too.
Liver is a vital organ with essential biosynthetic and metabolic functions. It was reported that abnormal liver function test (ALFT) was associated with higher mortality risk in critically ill patients (11–14). ALFTs are extremely common in AIS patients, with an incidence of about 40% (15–18). A prospective study showed more severe stroke and worse outcomes in AIS patients with non-alcoholic fatty liver disease (15). However, there are scarce data regarding the association between ALFT and clinical outcomes in AIS-LVO patients undergoing MT.
The aims of this study were to (1) determine the association between periprocedural ALFT and the clinical outcomes, and (2) assess the potential dose–response relationship between liver function test levels and prognosis of AIS-LVO patients undergoing MT.
Methods
Data Resources
Patients were screened from the Nanjing Stroke Registry Program (NSRP, between January 2014 and December 2019) and the Captor trial (a multi-center clinical trial between March 2018 and July 2019, register code: ChiCTR1900025256). Detailed descriptions of NSRP had been published elsewhere (19). The Captor trial was aimed to compare the Captor stent retriever with the Solitaire FR device for rapid flow restoration in AIS-LVO. The non-inferiority of the Captor retrievable stent was proved after enrolling 245 patients from 16 comprehensive stroke centers in China.
Inclusion and Exclusion Criteria
The key inclusion criteria were as follows: (1) age ≥18 years; (2) National Institute of Health Stroke Scale (NIHSS) score on admission ≥6; (3) occlusion of the intracranial large vessel (defined as diameter ≥2 mm) proved by CT angiography, magnetic resonance angiography, or digital subtraction angiography; (4) completion of groin-puncture within 8 h since stroke onset.
The key exclusion criteria were as follows: (1) active hemorrhage or hemorrhagic tendency; (2) severe organic diseases such as heart, lung, liver, and kidney failure; (3) occlusion attributed to arterial dissection or arteritis. The Ethics Committee of Jinling Hospital and each participating center approved this study according to the Declaration of Helsinki. The requirement for written informed consent was waived because of its retrospective nature.
Data Collections
Baseline demographic parameters, medical histories, laboratory tests, the NIHSS scores, and radiographic evaluation were obtained. The treatment profiles were also gathered, including intravenous thrombolysis (IVT), number of retrieval attempts, final reperfusion status, and procedural time. IVT was administrated within 4.5 h of stroke onset after excluding contraindications. Retrieval attempts were recommended no more than three times, but it was at the discretion of the interventionalists. Reperfusion was evaluated with the modified Thrombolysis In Cerebral Ischemia scale (mTICI) (20). Successful reperfusion was defined as the mTICI scale score of 2b or 3.
Post-procedural NIHSS scores of all patients were assessed by attending neurologists. Follow-up imaging examinations were performed within 24 ± 6 h after the procedure. Symptomatic intracranial hemorrhage (SICH) was defined as imaging evidence of intracranial hemorrhage (ICH) with an increase of ≥4 points on the NIHSS within 24 h. On day 7 ± 2 or hospital discharge if earlier, assessments of neurological severity were performed on the subjects again.
Post-procedural laboratory tests were carried out routinely in the acute phase (7 ± 2 days after MT) and/or in case of clinical deterioration. Liver function was assessed according to tests of serum aspartate aminotransferase [upper limit of normal (ULN): 40 U/L], alanine aminotransferase (ULN: 40 U/L), total bilirubin (ULN: 21 mmol/L), and direct bilirubin (ULN: 10 mmol/L). ALFT was defined as any elevated value above the ULN (21). Multiplemax was defined as the maximum multiple of ULN in the four variables (aspartate aminotransferase, alanine aminotransferase, total bilirubin, and direct bilirubin) during hospitalization after MT.
Follow-up was performed by outpatient clinical visits or telephone contacts to record the modified Rankin Scale (mRS) score at 90 ± 14 days. A favorable outcome was defined as mRS 0–2 at 90 days, and an excellent outcome was referred to as mRS 0–1 at 90 days.
Statistical Analysis
Median imputation was performed to account for missing values [white blood cell: 6 (1.4%); creatinine: 9 (2.1%); glucose: 11 (2.6%); activated partial thromboplastin time: 17 (4.0%); partial thromboplastin time: 13 (3.1%); international normalized ratio: 13 (3.1%)].
Frequency (percentage) was used for categorical variables. Mean (SD) was adopted for continuous variables with normal distribution and median (interquartile range, IQR) for non-normal variables.
Univariate analysis was conducted with Student t-test, Mann–Whitney U, χ2 test, or Fisher exact test as appropriate. Confounding factors and other variables with a statistical trend (p ≤ 0.1) in the univariate analysis were included in multivariate logistic regression analysis (forward selection) to develop a basic model for the favorable prognosis. Composite model was established by factors of basic model and IVT, ASITN/SIR, recanalization, and SICH. Besides, the predictive value of adding preprocedural or post-procedural ALFT into the basic model was estimated by the area under curve (AUC). The improvements on the basic model were assessed by the continuous net reclassification index (NRI) and integrated discrimination improvement (IDI) of which values above 0 were regarded as significant (22). Multiplemax was categorized based on quartiles to evaluate the association between Multiplemax and prognosis. The pattern of the potential dose–response relationship between Multiplemax and prognosis was revealed using a restricted cubic spline with 3 knots after adjusting for confounders. Odds ratio (OR) was reported with a 95% CI.
Subgroup analyses were performed to detect the heterogeneity in the effect of post-procedural ALFT on prognosis. Sensitivity analyses were conducted to explore the effect of ALFTs at different periods on prognosis. A two-tailed p ≤ 0.05 was considered statistically significant. Statistical analyses were performed with the SPSS software package, version 26 (IBM, Armonk, NY) and R statistical software 3.6.3 [R Core Team (2020). R: A language and environment for statistical computing. R Foundation for Statistical Computing, Vienna, Austria. URL https://www.R-project.org/].
Results
Clinical Characteristics
The flowchart of this study is illustrated in Supplementary Figure 1. The demographic characteristics of all participants are displayed in Table 1. Of the 420 patients enrolled in this study, men accounted for 60.7% and the median age was 68 years. In total, 234 (55.7%) subjects presented as post-procedural ALFT, and 186 (44.3%) had normal liver function after MT.
Patients were divided into two groups (the ALFT group and the non-ALFT group) according to the post-procedural ALFT. Medical histories were balanced between the two groups. The ALFT group had a significantly larger proportion of preprocedural ALFT (40.2 vs. 16.1%, p < 0.001). Higher NIHSS score on admission (median, 18 vs. 15, p < 0.001), higher white blood cell count (p = 0.019), higher glucose level (p = 0.035), more pneumonia (65.4 vs. 38.2%, p < 0.001), and more SICH (12.0 vs. 3.8%, p < 0.001) were detected in the ALFT group than the non-ALFT group. The ratio of IVT pretreatment (34.2 vs. 28.5%, p = 0.213) was similar between patients with normal and abnormal liver function. The comparisons between the two groups on median NIHSS scores at 24 h (19 in the ALFT group vs. 11 in the non-ALFT group, p < 0.001) and 7 days (14 in the ALFT group vs. 6 in the non-ALFT group, p < 0.001) showed similar trends with that of the admission NIHSS score (Table 1).
Clinical Outcomes
The mortality rate at 7 days was comparable between the two groups (p = 0.190). More deaths occurred in the ALFT group at 90 days (29.1 vs. 15.6%, p = 0.001, Table 1).
In total, 168 (40.0%) patients ranked 0–2 on mRS score at 90 days after MT, and the proportion of excellent prognosis was 26.9%. Compared with the non-ALFT subjects, the ALFT group had a lower percentage of favorable prognosis (26.5 vs. 57.0%, p < 0.001) and excellent prognosis (15.4 vs. 41.4%, p < 0.001) at 90 days (Figure 1). The density plot of log-transformed Multiplemax distribution for dichotomous prognosis is shown in Supplementary Figure 2. Smaller Multiplemax was detected in patients achieving better prognosis.
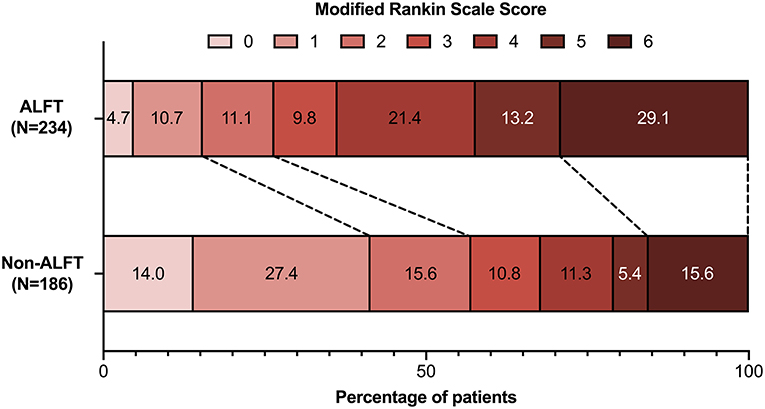
Figure 1. Comparisons of modified Rankin scale scores at 90 days between the ALFT group and the non-ALFT group. ALFT, abnormal liver function test.
Multivariable Analyses
The basic model for predicting favorable prognosis was developed after adjusting for confounding factors and variables with a statistical trend (p ≤ 0.1) in the univariate analysis. Finally, age [OR (95% CI): 0.96 (0.94–0.98), p < 0.001], the NIHSS score at 24 h [OR (95% CI): 0.85 (0.82–0.88), p < 0.001], pneumonia [OR (95% CI): 0.43 (0.25–0.72), p = 0.002], and glucose level [OR (95% CI): 0.81 (0.71–0.92), p = 0.001] on admission were included in the basic model (Table 2).
An improved model was established after adding ALFT into the basic model. As shown in the improved model, post-procedural ALFT had a significant correlation [OR (95% CI): 0.48 (0.28–0.81), p = 0.006] with mRS 0–2 at 90 days after adjusting for the factors involved in the basic model (Table 2). Compared with the basic model, the predictive value of the improved model on favorable prognosis showed a significant improvement when assessed by AUC (basic model: 0.875; improved model: 0.881, p = 0.007), continuous NRI (value: 0.401, p < 0.001), and IDI (value: 0.013, p < 0.001), as shown in Table 3. In addition, preprocedural ALFT was not a statistically significant predictor of clinical prognosis (all p-values > 0.05).

Table 3. Comparison of basic models and models adding ALFT for predicting favorable prognosis and excellent prognosis.
The role of Multiplemax in predicting favorable prognosis was also assessed in multivariable analysis. After adjusting for age, pneumonia, glucose level, and median NIHSS score at 24 h, Multiplemax remained an independent predictive factor of favorable prognosis (p = 0.019, Table 4). To explore potential dose–response association, the restricted cubic spline was drawn with three knots after adjusting for covariates mentioned previously. Interestingly, increasing Multiplemax was no longer lower odds of favorable prognosis (Figure 2) further when Multiplemax was more than 2.
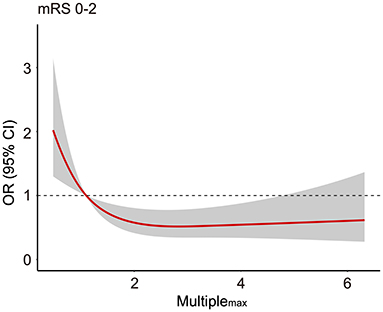
Figure 2. The association between Multiplemax and the odds ratio for favorable prognosis (90-day modified Rankin scale scores 0–2). Adjusted for age, median NIHSS score at 24 h, pneumonia, and glucose level. mRS, modified Rankin scale; OR, odds ratio; NIHSS, National Institute of Health Stroke Scale.
Subgroup Analyses and Sensitivity Analyses
A dominant tendency toward a lower proportion of favorable prognosis in the ALFT group was elucidated in the subgroup analysis (all p-values < 0.01, except for subjects who had NIHSS score ≥15) (Figure 3). Of note, no significant interaction was detected between post-procedural ALFT and these clinical features on the favorable prognosis.
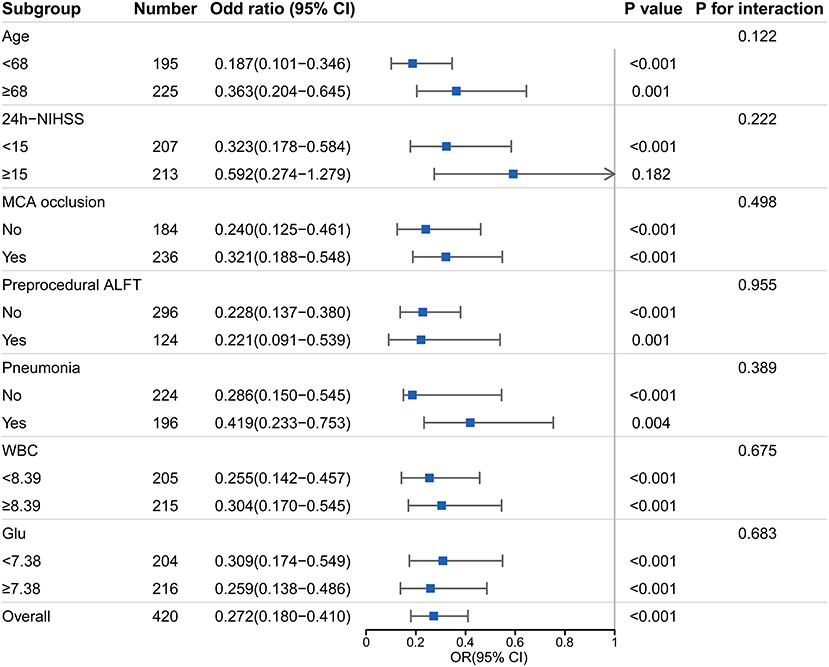
Figure 3. Subgroup analyses of the association between post-procedural ALFT and the favorable prognosis. This forest plot shows the odds ratio for favorable prognosis (90-day modified Rankin scale scores 0–2) of post-procedural ALFT in subgroups. NIHSS, National Institute Health Stroke Scale; MCA, middle cerebral artery; ALFT, abnormal liver function; WBC, white blood cell; mRS, modified Rankin scale; OR, odds ratio.
In sensitivity analyses, patients with post-procedural ALFTs alone had a lower proportion of favorable prognosis [OR (95% CI): 0.23 (0.14–0.38), p < 0.001] than patients with preprocedural ALFTs alone [OR (95% CI): 1.94 (0.84–4.52), p = 0.120] (Figure 4). They even had less favorable prognoses than patients with both pre- and post-procedural ALFTs [OR (95% CI): 0.23 (0.14–0.38) vs. 0.43 (0.25–0.73)].
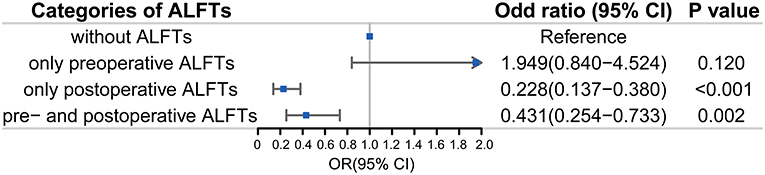
Figure 4. Association between ALFTs at different periods and the favorable prognosis in patients with AIS. ALFT, abnormal liver function; OR, odds ratio.
Discussion
In this multi-center retrospective study, 55.7% of all AIS patients undergoing MT were identified as post-procedural ALFT. Patients with post-procedural ALFT had more severe stroke, and higher WBC count and blood glucose values on admission. The presence of post-procedural ALFT, rather than the preprocedural ALFT, was independently associated with a decreased proportion of favorable outcomes at 90 days. However, there was no evidence of a dose–response relationship between the severity of post-procedural ALFT and functional prognosis.
The prevalence of post-procedural ALFT in patients with AIS in our study was 55.7%, higher than the previously reported frequency (about 40%) in AIS patients (15–18). Post-procedural ALFT may be a consequence of neuroendocrine dysregulation after stroke onset (23). Focal brain ischemia could induce systemic pathophysiologic reactions and contribute to hepatic inflammatory and apoptotic activation (24). Stroke-induced catecholamine surge promotes the endoplasmic reticulum stress and impairs hepatic insulin signaling (25). Moreover, elevated bilirubin and liver enzyme levels may also be attributed to the treatment measures of AIS, such as antibiotic drugs, statin, and endovascular therapy (21, 26). Therefore, metabolic imbalances and the reflection of treatment during hospitalization are conjectured to be major mechanisms of post-procedural ALFT.
This study demonstrated a significant correlation between post-procedural ALFT and the severity of stroke. Similar to the findings of previous researches, subjects with ALFT were associated with higher NIHSS scores during hospitalization, indicating more severe stroke (15, 18). Analogously, Muscari et al. elucidated that impaired metabolic homeostasis in the liver was associated with cerebral infarct volume in experimental stroke models (27).
Inspiringly, this study revealed the reliable prognostic value of post-procedural ALFT on predicting functional outcomes of AIS patients treated with MT. This finding was in line with previous reports on the prognostic role of ALFT in AIS (15, 18). Substantial evidence expounded that hepatic dysfunction served as a predictor of clinical outcomes in critically ill patients (11–14). In addition, a population-based research indicated abnormal liver tests were associated with increased all-cause mortality in elderly people (28). However, in our study, data regarding liver function tests before the procedure were limited, and no significant association between preprocedural ALFT and the prognosis was observed. There might exist bias regarding preprocedural ALFT, as patients with severe ALFTs might not receive MT. This might partly explain why the prognostic value of post-procedural ALFT was important than the preprocedural ALFT. Furthermore, results of post-procedural liver function tests may be more representative of the physiological state and restoration of metabolic homeostasis.
Despite unclear specific mechanisms, it is conceivable that ALFT affects functional outcomes since the liver is an important organ for metabolism and immunity (29). It is known that AIS patients suffer from potential immunodepression due to the post-stroke autonomic system activation (30, 31). Subsequent infections and impaired metabolic homeostasis contribute to organ abnormalities, ultimately resulting in the poor prognosis of AIS (23).
Several strengths of our study were noteworthy. Relatively intact data from multiple centers revealed the contemporary status of MT for AIS-LVO in China. Moreover, comprehensive analyses were performed to explore the relationship between ALFT and functional outcomes after MT. Most importantly, liver function tests serve as routine examinations during hospitalization. The availability of test results makes its predictive value more significant for clinical application.
Despite the advantages mentioned previously, our study also had some inevitable limitations. First, this study is subject to the inherent limitations of a retrospective study design. Second, the liver function tests containing only four liver chemistries. Incomprehensive assessments made limited contributions to revealing the detailed mechanism. Third, missing records of the adjuvant medicine, especially for liver protection drugs, was another limitation. Finally, only the Chinese population was enrolled in this study, which may limit the generalizability of the conclusions. Further researches based on different ethnic populations and larger sample sizes are warranted.
Conclusions
In this study, post-procedural ALFT occurred commonly in AIS patients treated with MT. It was associated with the severity of stroke and was an independent predictor for worse functional outcomes. More attention is needed for AIS patients who were diagnosed as ALFT after MT concerning the increased risk for poor prognosis.
Data Availability Statement
The raw data supporting the conclusions of this article will be made available by the authors, without undue reservation.
Ethics Statement
The studies involving human participants were reviewed and approved by The Ethics Committee of Jinling Hospital and each participating center. Written informed consent for participation was not required for this study in accordance with the national legislation and the institutional requirements.
Author Contributions
RL, KH, and XL: concept and design. KH, MZ, MW, and QY: acquisition of data. KH, MZ, RL, and LX: analysis and interpretation of the data. KH, MZ, LX, JG, and JD: drafting of the article. RL, KH, MZ, and XL: critical revision of the article for important intellectual content. All authors contributed to the article and approved the submitted version.
Funding
This work was supported by National Natural Science Foundation of China (81901218, 81870946, 81870946, and 81701299); National Key Research and Development Program of China (2017YFC1307900/2017YFC1307901).
Conflict of Interest
XL served as the principal investigator for the Captor clinical trial sponsored by Shanghai strokecare medical Co. LTD. RL served as a researcher for the Captor clinical trial and provided consultation to Shanghai strokecare medical Co. LTD.
The remaining authors declare that the research was conducted in the absence of any commercial or financial relationships that could be construed as a potential conflict of interest.
Publisher's Note
All claims expressed in this article are solely those of the authors and do not necessarily represent those of their affiliated organizations, or those of the publisher, the editors and the reviewers. Any product that may be evaluated in this article, or claim that may be made by its manufacturer, is not guaranteed or endorsed by the publisher.
Acknowledgments
The authors gratefully acknowledge all the participants and all staff of research cooperation institutions.
Supplementary Material
The Supplementary Material for this article can be found online at: https://www.frontiersin.org/articles/10.3389/fneur.2021.670387/full#supplementary-material
References
1. Badhiwala JH, Nassiri F, Alhazzani W, Selim MH, Farrokhyar F, Spears J, et al. Endovascular thrombectomy for acute ischemic stroke: a meta-analysis. Jama. (2015) 314:1832–43. doi: 10.1001/jama.2015.13767
2. Yang P, Zhang Y, Zhang L, Zhang Y, Treurniet KM, Chen W, et al. Endovascular thrombectomy with or without intravenous alteplase in acute stroke. N Engl J Med. (2020) 382:1981–93. doi: 10.1056/NEJMoa2001123
3. Rabinstein AA, Albers GW, Brinjikji W, Koch S. Factors that may contribute to poor outcome despite good reperfusion after acute endovascular stroke therapy. Int J Stroke. (2019) 14:23–31. doi: 10.1177/1747493018799979
4. Wei Y, Pu Y, Pan Y, Nie X, Duan W, Liu D, et al. Cortical microinfarcts associated with worse outcomes in patients with acute ischemic stroke receiving endovascular treatment. Stroke. (2020) 51:2742–51. doi: 10.1161/STROKEAHA.120.030895
5. Bracard S, Ducrocq X, Mas JL, Soudant M, Oppenheim C, Moulin T, et al. Mechanical thrombectomy after intravenous alteplase versus alteplase alone after stroke (THRACE): a randomised controlled trial. Lancet Neurol. (2016) 15:1138–47. doi: 10.1016/S1474-4422(16)30177-6
6. Pedraza MI, de Lera M, Bos D, Calleja AI, Cortijo E, Gómez-Vicente B, et al. Brain atrophy and the risk of futile endovascular reperfusion in acute ischemic stroke. Stroke. (2020) 51:1514–21. doi: 10.1161/STROKEAHA.119.028511
7. Choi K-H, Kim J-H, Kang K-W, Kim J-T, Choi S-M, Lee S-H, et al. Impact of microbleeds on outcome following recanalization in patients with acute ischemic stroke. Stroke. (2019) 50:127–34. doi: 10.1161/STROKEAHA.118.023084
8. Li J, Zhang P, Wu S, Wang Y, Zhou J, Yi X, et al. Stroke-related complications in large hemisphere infarction: incidence and influence on unfavorable outcome. Ther Adv Neurol Disord. (2019) 12:1756286419873264. doi: 10.1177/1756286419873264
9. de Montmollin E, Ruckly S, Schwebel C, Philippart F, Adrie C, Mariotte E, et al. Pneumonia in acute ischemic stroke patients requiring invasive ventilation: impact on short and long-term outcomes. J Infect. (2019) 79:220–7. doi: 10.1016/j.jinf.2019.06.012
10. Xiao L, Ma M, Gu M, Han Y, Wang H, Zi W, et al. Renal impairment on clinical outcomes following endovascular recanalization. Neurology. (2020) 94:e464–73. doi: 10.1212/WNL.0000000000008748
11. Kramer L, Jordan B, Druml W, Bauer P, Metnitz PG. Incidence and prognosis of early hepatic dysfunction in critically ill patients—a prospective multicenter study. Crit Care Med. (2007) 35:1099–e7. doi: 10.1097/01.CCM.0000259462.97164.A0
12. Fuhrmann V, Kneidinger N, Herkner H, Heinz G, Nikfardjam M, Bojic A, et al. Hypoxic hepatitis: underlying conditions and risk factors for mortality in critically ill patients. Intensive Care Med. (2009) 35:1397–405. doi: 10.1007/s00134-009-1508-2
13. Jäntti T, Tarvasmäki T, Harjola V-P, Parissis J, Pulkki K, Sionis A, et al. Frequency and prognostic significance of abnormal liver function tests in patients with cardiogenic shock. Am J Cardiol. (2017) 120:1090–7. doi: 10.1016/j.amjcard.2017.06.049
14. Jung C, Fuernau G, Eitel I, Desch S, Schuler G, Kelm M, et al. Incidence, laboratory detection and prognostic relevance of hypoxic hepatitis in cardiogenic shock. Clin Res Cardiol. (2017) 106:341–9. doi: 10.1007/s00392-016-1060-3
15. Abdeldyem SM, Goda T, Khodeir SA, Abou Saif S, Abd-Elsalam S. Nonalcoholic fatty liver disease in patients with acute ischemic stroke is associated with more severe stroke and worse outcome. J Clin Lipidol. (2017) 11:915–9. doi: 10.1016/j.jacl.2017.04.115
16. Moshayedi H, Ahrabi R, Mardani A, Sadigetegad S, Farhudi M. Association between non-alcoholic fatty liver disease and ischemic stroke. Iran J Neurol. (2014) 13:144.
17. Arsalan, Ismail M, Khattak MB, Khan F, Anwar MJ, Murtaza Z, et al. Prognostic significance of serum bilirubin in stroke. J Ayub Med Coll Abbottabad. (2011) 23:104–7.
18. Li H, Hu B, Wei L, Zhou L, Zhang L, Lin Y, et al. Non-alcoholic fatty liver disease is associated with stroke severity and progression of brainstem infarctions. Eur J Neurol. (2018) 25:577–e34. doi: 10.1111/ene.13556
19. Liu X, Xu G, Wu W, Zhang R, Yin Q, Zhu W. Subtypes and one-year survival of first-ever stroke in Chinese patients: the Nanjing stroke registry. Cerebrovasc Dis. (2006) 22:130–6. doi: 10.1159/000093241
20. Zaidat OO, Yoo AJ, Khatri P, Tomsick TA, von Kummer R, Saver JL, et al. Recommendations on angiographic revascularization grading standards for acute ischemic stroke: a consensus statement. Stroke. (2013) 44:2650–63. doi: 10.1161/STROKEAHA.113.001972
21. Kwo PY, Cohen SM, Lim JK. ACG clinical guideline: evaluation of abnormal liver chemistries. Am J Gastroenterol. (2017) 112:18–35. doi: 10.1038/ajg.2016.517
22. Pencina MJ, D'Agostino RB, Sr, D'Agostino RB, Jr, Vasan RS. Evaluating the added predictive ability of a new marker: from area under the ROC curve to reclassification and beyond. Stat Med. (2008) 27:157–72; discussion 207–12. doi: 10.1002/sim.2929
23. Balch MH, Nimjee SM, Rink C, Hannawi Y. Beyond the brain: the systemic pathophysiological response to acute ischemic stroke. J Stroke. (2020) 22:159–72. doi: 10.5853/jos.2019.02978
24. Ottani A, Giuliani D, Mioni C, Galantucci M, Minutoli L, Bitto A, et al. Vagus nerve mediates the protective effects of melanocortins against cerebral and systemic damage after ischemic stroke. J Cereb Blood Flow Metab. (2009) 29:512–23. doi: 10.1038/jcbfm.2008.140
25. Wang Y-Y, Lin S-Y, Chuang Y-H, Sheu WH-H, Tung K-C, Chen C-J. Activation of hepatic inflammatory pathways by catecholamines is associated with hepatic insulin resistance in male ischemic stroke rats. Endocrinology. (2014) 155:1235–46. doi: 10.1210/en.2013-1593
26. Adhyaru BB, Jacobson TA. Safety and efficacy of statin therapy. Nat Rev Cardiol. (2018) 15:757–69. doi: 10.1038/s41569-018-0098-5
27. Muscari A, Collini A, Fabbri E, Giovagnoli M, Napoli C, Rossi V, et al. Changes of liver enzymes and bilirubin during ischemic stroke: mechanisms and possible significance. BMC Neurol. (2014) 14:122. doi: 10.1186/1471-2377-14-122
28. Fleming KM, West J, Aithal G, Fletcher AE. Abnormal liver tests in people aged 75 and above: prevalence and association with mortality. Aliment Pharmacol Therap. (2011) 34:324–34. doi: 10.1111/j.1365-2036.2011.04718.x
29. Heymann F, Tacke F. Immunology in the liver—from homeostasis to disease. Nat Rev Gastroenterol Hepatol. (2016) 13:88. doi: 10.1038/nrgastro.2015.200
30. Meisel C, Schwab JM, Prass K, Meisel A, Dirnagl U. Central nervous system injury-induced immune deficiency syndrome. Nat Rev Neurosci. (2005) 6:775–86. doi: 10.1038/nrn1765
Keywords: liver function tests, ischemic stroke, thrombectomy, prognosis, observational study
Citation: Huang K, Zha M, Xiao L, Gao J, Du J, Wu M, Yang Q, Liu R and Liu X (2021) Prognostic Value of Abnormal Liver Function Tests After Mechanical Thrombectomy for Acute Ischemic Stroke. Front. Neurol. 12:670387. doi: 10.3389/fneur.2021.670387
Received: 21 February 2021; Accepted: 29 June 2021;
Published: 28 July 2021.
Edited by:
Robert G. Kowalski, University of Colorado, United StatesReviewed by:
Ning Ma, Capital Medical University, ChinaWenbo Zhao, Capital Medical University, China
Copyright © 2021 Huang, Zha, Xiao, Gao, Du, Wu, Yang, Liu and Liu. This is an open-access article distributed under the terms of the Creative Commons Attribution License (CC BY). The use, distribution or reproduction in other forums is permitted, provided the original author(s) and the copyright owner(s) are credited and that the original publication in this journal is cited, in accordance with accepted academic practice. No use, distribution or reproduction is permitted which does not comply with these terms.
*Correspondence: Xinfeng Liu, xfliu2@vip.163.com; Rui Liu, liurui8616@163.com
†These authors have contributed equally to this work