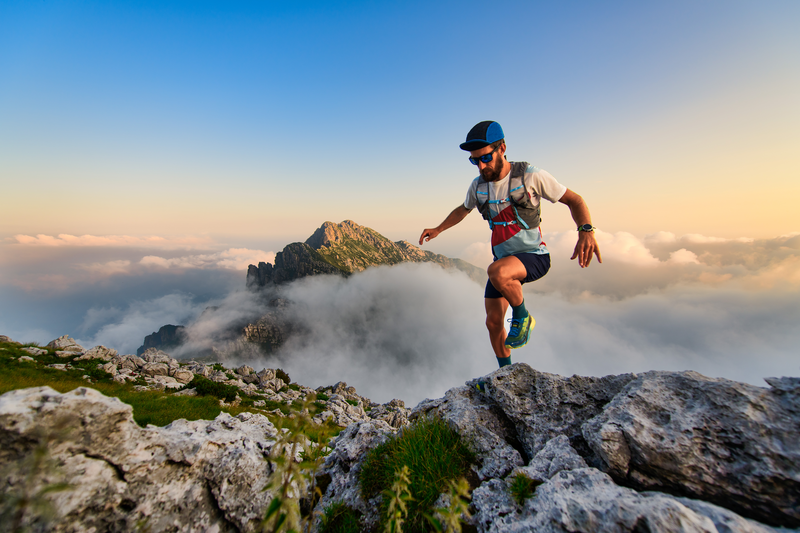
95% of researchers rate our articles as excellent or good
Learn more about the work of our research integrity team to safeguard the quality of each article we publish.
Find out more
ORIGINAL RESEARCH article
Front. Neurol. , 10 March 2021
Sec. Neuro-Oncology and Neurosurgical Oncology
Volume 12 - 2021 | https://doi.org/10.3389/fneur.2021.646075
This article is part of the Research Topic nTMS, Connectivity and Neuromodulation in Brain Tumor Patients View all 25 articles
Background: The simplistic approaches to language circuits are continuously challenged by new findings in brain structure and connectivity. The posterior middle frontal gyrus and area 55b (pFMG/area55b), in particular, has gained a renewed interest in the overall language network.
Methods: This is a retrospective single-center cohort study of patients who have undergone awake craniotomy for tumor resection. Navigated transcranial magnetic simulation (nTMS), tractography, and intraoperative findings were correlated with language outcomes.
Results: Sixty-five awake craniotomies were performed between 2012 and 2020, and 24 patients were included. nTMS elicited 42 positive responses, 76.2% in the inferior frontal gyrus (IFG), and hesitation was the most common error (71.4%). In the pMFG/area55b, there were seven positive errors (five hesitations and two phonemic errors). This area had the highest positive predictive value (43.0%), negative predictive value (98.3%), sensitivity (50.0%), and specificity (99.0%) among all the frontal gyri. Intraoperatively, there were 33 cortical positive responses—two (6.0%) in the superior frontal gyrus (SFG), 15 (45.5%) in the MFG, and 16 (48.5%) in the IFG. A total of 29 subcortical positive responses were elicited−21 in the deep IFG–MFG gyri and eight in the deep SFG–MFG gyri. The most common errors identified were speech arrest at the cortical level (20 responses−13 in the IFG and seven in the MFG) and anomia at the subcortical level (nine patients—eight in the deep IFG–MFG and one in the deep MFG–SFG). Moreover, 83.3% of patients had a transitory deterioration of language after surgery, mainly in the expressive component (p = 0.03). An increased number of gyri with intraoperative positive responses were related with better preoperative (p = 0.037) and worse postoperative (p = 0.029) outcomes. The involvement of the SFG–MFG subcortical area was related with worse language outcomes (p = 0.037). Positive nTMS mapping in the IFG was associated with a better preoperative language outcome (p = 0.017), relating to a better performance in the expressive component, while positive mapping in the MFG was related to a worse preoperative receptive component of language (p = 0.031).
Conclusion: This case series suggests that the posterior middle frontal gyrus, including area 55b, is an important integration cortical hub for both dorsal and ventral streams of language.
Previous models of parcellation of the cerebral cortex have been proposed based on cytoarchitectonic (1, 2), myeloarchitectonic (3, 4), or functional characteristics of the different cerebral cortical areas (5, 6).
More recently, a new mapping of the human cortex has been described, using a multi-modal gradient-based parcellation approach (7). One of the novelties of this approach has been the identification of new cortical areas with a distinctive myelo/cytoarchitectonic and functional profile. A particularly interesting region is the frontal area 55b. Initially noted by Hopf in 1956 (4), this area is located at the posterior aspect of the middle frontal gyrus (MFG) and is delimited by the frontal eye field (FEF) superiorly, the premotor eye field (PEF) inferiorly, the primary motor cortex and the ventral motor cortex posteriorly, and by the prefrontal areas anteriorly (7, 8). Area 55b appears to be lightly myelinated and lies between moderately myelinated areas (i.e., FEF above and PEF below) and anteriorly to heavily myelinated areas (i.e., primary motor cortex). It has been described to be involved in various language production tasks and fluency of speech (7, 9, 10). These findings are responsible for the renewed interest in the contribution of the posterior MFG to the overall language network.
Techniques of brain mapping that have evolved to increase the extent and safety of tumor resection in eloquent areas of the brain (11) have the unique advantage of testing different functions of specific cortical areas and networks at the individual level (12). Direct electrical stimulation (DES) at the cortical and subcortical levels is the gold standard for intraoperative mapping, defining the functional borders of resection in glioma surgery (13–15). In addition, navigated transcranial magnetic stimulation (nTMS) has emerged over the past decade as a useful adjunct for the preoperative mapping of motor (16–21) and language (22–27) areas of the brain.
In the present paper, we reviewed the results obtained by combining DES and nTMS in the functional assessment of the middle frontal gyrus and area 55b in a series of patients undergoing awake surgery for brain tumors. In addition, we evaluated the potential relationship between preoperative and intraoperative language mapping and between the assessment of language performed prior to and following surgery, with a view to assess the relative contribution of the MFG on the language outcome. The preoperative and intraoperative findings are reviewed, and the potential role of these areas as part of the language network is discussed.
This is a retrospective single-center cohort study of non-consecutive patients admitted with language eloquent tumors for surgical treatment from January 2012 to January 2020. The inclusion criteria for the current study were age above 18 years old, awake craniotomy with DES for language mapping, and a tumor located in the dominant frontal lobe. Hemispheric dominance was assessed with the Edinburgh Handedness Inventory scale. The exclusion criteria included failed awake craniotomy and awake craniotomy for non-language mapping purposes.
The preoperative and postoperative assessments were performed using the Sheffield Aphasia Screening Test for Acquired Language Disorder (SST) (28). This test was applied by the same speech and language therapist responsible for the intraoperative language testing. The patients were interviewed pre- and postoperatively to assess their communication abilities in conversational speech. Subtle subjective changes pertaining to comprehension, speech, reading, or writing abilities affecting daily living were evaluated. Where relevant, additional subtests were administered from the Mount Wilga Higher Level Language Test (29). The language errors were divided into speech arrest, hesitation, fluency disturbance, repetition disturbance, semantic paraphasia, and anomia.
An asleep–awake–asleep craniotomy was performed in all the included patients. Low-frequency intraoperative stimulation according to the Penfield technique (30) was performed. Then, 50-Hz biphasic square wave pulses of 1-ms duration were applied using a constant current stimulator (ISIS Neurostimulator; Inomed Medizintechnik GmbH). The current threshold used for brain mapping was the minimal current responsible for speech arrest during the counting task (two out of three attempts) or the highest current non-responsible for after-discharges. The exposed cortical area was mapped with one stimulation area every 2–3 cm at least three times per language task. The selection of the intraoperative tasks were performed according to the Dutch Linguistic Intra-operative Protocol (31) and the Verb and Noun Test for Peri-Operative Testing (32).
Positive and negative responses were recorded, indicating the presence and absence of language errors elicited by DES respectively. After a speech arrest was identified and the threshold was established, the entire exposed cortical area of the frontal lobe was mapped with object naming and action naming in both present and past tenses. At the subcortical level, repetition tasks were used for mapping the arcuate fasciculus (AF), sentence completion for the fronto-aslant tract (FAT), and semantic odd-one-out and object naming for the inferior fronto-occipital fasciculus (IFOF). The positive responses—two out of three attempts—with induced speech deficit in the absence of after-discharges on electrocorticography were documented with intraoperative pictures.
Preoperative mapping with nTMS has been used at our center since 2016 as an adjunct to intraoperative DES mapping. Whenever available, data from nTMS were included in the analysis.
Language mapping was performed following resting motor threshold determination (33). A set series of pre-designed images were presented to the patient for baseline assessment in two consecutive rounds: object naming, action naming in the present tense, and action naming in the past tense (32). The inter-picture interval was set to 2,500 ms, and the display time (DT) varied between 500 and 1,000 ms, which was dependent on a patient's ability. Images that were incorrectly described or with hesitation were excluded from the final exam. An offline analysis was performed, comparing the stimulation assessment to the baseline assessment and identifying any changes in language function during the exam. Language errors were classified into distinct categories (hesitation, expressive, semantic, anomia, arrest, other, and no errors).
The intraoperative pictures of positive stimulation sites collected during intraoperative mapping were included in the preoperative MRI studies (T1-weighted images after gadolinium injection) by comparing the anatomical landmarks (i.e., sulci and gyri) of the single pictures with the axial brain volumetric images and reformatted sagittal/coronal images (33). It was therefore possible to correlate the positive intraoperative sites with nTMS mapping, allowing for the calculation of TMS specificity, sensitivity, and positive and negative predictive values (PPV and NPV, respectively).
STATA 13.0 software was used for statistical analysis. Regression techniques were performed to compare the language outcomes—screening test for acquired language disorder (SST) and its receptive and expressive subdivisions—with the number of gyri and the main subcortical areas infiltrated by the tumor. A p-value < 0.05 was considered as significant.
Sixty-five awake craniotomies were performed between 2012 and 2020. There were 24 (36.9%) frontal tumors that constitute the object of the current study. The demographics showed an even distribution between males and females, with a mean age of 47 ± 13.8 years old (standard deviation). The majority of the included patients had left-sided tumors (22, 91.6%). Meanwhile, 19 (79.1%) patients presented with a new-onset seizure; two (8.3%) had a motor deficit, and two (8.3%) presented with a language deficit. The vast majority of patients (20, 83.4%) presented with a performance status of 0. High-grade gliomas were prevalent in this series [71% World Health Organization (WHO) grade III and IV]. Isocitrate dehydrogenase was positive in 10 (41.6%) tumors, whereas 1p/19q co-deletion was present in nine (37.5%) oligodendrogliomas. Figure 1 and Table 1 summarize the patients' characteristics.
Figure 1. Flow chart of the present study, detailing the inclusion and exclusion criteria. Positive responses indicate language errors elicited by direct electric stimulation. Negative responses indicate no language errors elicited by direct electric stimulation. Exposure indicates the specific frontal gyrus exposed during craniotomy.
Table 1. Demographics of the included subjects along with the classification of tumors based on histology, grade, and biomarkers.
The average preoperative SST was 18.9/20. The average receptive language skill score was 8.1/9, and the average expressive language skill score was 10.7/11 (seven patients were excluded due to incomplete assessments). From the SST, the most frequent receptive errors were auditory semantic differentiation and the ability to fully comprehend a paragraph-level narrative. Expressive errors were rare, but when present, the most frequent error was the ability to provide word definitions. The SST does not measure phonemic ability, but no patient displayed any marked phonemic errors in conversation preoperatively (Table 2).
Table 2. A comprehensive table detailing the navigated transcranial magnetic simulation language errors, intraoperative stimulation positive responses, a comparison of language pathway error, Sheffield Aphasia Screening Test (SST) for Acquired Language Disorder assessments pre- and postoperatively, changes in SST score, and a comprehensive speech and language therapy pre-, intra-, and postoperative assessment.
nTMS was performed in 14 (58.3%) patients with frontal tumors (1,844 stimulations distributed across the gyri as follows: SFG−172, MFG−428, IFG−1,244), and positive responses were elicited in 12 patients (85.7%), with a total of 42 positive responses: three responses in the SFG (one hesitation and two anomic errors); seven responses in the MFG (five hesitations and two phonemic errors); 32 responses in the IFG (24 hesitations, six phonemic, and two semantic errors). This shows a preferential distribution of the stimulations in the IFG, particularly given the likelihood of positive responses in the area of the frontal operculum.
Overall, the nTMS had a PPV of 31.0%, a NPV of 97.8%, sensitivity of 45.7%, and specificity of 98.3%. When the gyri were compared, the MFG had the highest PPV (43.0% vs. IFG−31.25% and SFG−0%), NPV (98.3% vs. IFG−97.8% and SFG−95.9%), sensitivity (50.0% vs. IFG−47.1%, and SFG−30.0%), and specificity (99.0% vs. IFG−98.2% and SFG−98.1%) (Table 2, Figure 2, and Supplementary Video 1).
Figure 2. Schematic representation of the positive navigated transcranial magnetic simulation responses, intraoperative cortical responses, and positive subcortical responses according to the different language errors. (A) Positive TMS responses. (B) Positive cortical intraoperative responses. (C) Positive subcortical intraoperative responses.
A preoperative structural connectome analysis performed in three patients with positive responses in the area 55b showed the strongest connectivity of this area not only with the primary motor cortex but as well as with the supplementary motor area, inferior frontal gyrus, and anterior aspect of the MFG (Figure 3). Positive nTMS mapping in the inferior frontal gyrus was related with a better preoperative overall SST score (p = 0.017) due to a better receptive component (p = 0.001). Positive nTMS mapping for the posterior MFG/area 55b was related with a worse receptive preoperative component of the SST (p = 0.031), but with no expression in the overall score (p = 0.059) (Table 3).
Figure 3. Qualitative analysis for region of interest-based tractography of the arcuate fasciculus (AF). The middle frontal gyrus region is in red, where the streamlines from the AF terminate. (A) Area 55b and whole-brain tractography. (B) Area 55b and ROI-based tractography of AF tract. (C) Whole-brain connectome. (D) Connectivity from the left Area 55b to the remaining brain areas. (E) Connectivity from the right Area 55b to the remaining brain areas.
Table 3. Correlation of navigated transcranial magnetic simulation responses per gyrus and the preoperative language assessment (Sheffield aphasia screening test for acquired language disorder).
The superior frontal gyrus (SFG) was exposed in 21 (87.5%), the middle frontal gyrus (MFG) in 23 (95.8%), and the inferior frontal gyrus (IFG) in 23 (95.8%) craniotomies. A total of 33 cortical positive responses for language were recorded. Two (6.0%) responses were recorded in the SFG, 15 (45.5%) in the MFG, and 16 (48.5%) in the IFG. Of relevance is the fact that all positive responses recorded in the MFG were demonstrated in area 55b. The following responses were identified: speech arrest in 20 patients (13 in IFG; seven in area 55b), hesitation in one patient (area 55b), decreased fluency in one patient (SFG), phonemic errors in seven patients (four in area 55b and three in IFG), semantic paraphasias in two patients (area 55b), and anomia in two patients (one in MFG and one in SFG). At the subcortical level, 29 positive responses were identified and divided into two main areas: 21 patients in the deep IFG–MFG area and eight patients in the deep MFG–SFG area. These positive responses were divided as follows: repetition disturbance in one patient (deep IFG), speech arrest in one patient (deep IFG), hesitation in four patients (deep MFG–SFG), decreased fluency in three patients (deep SFG), phonemic errors in four patients (deep IFG–MFG), semantic paraphasias in seven patients (deep IFG–MFG), and nine anomias (eight in deep IFG–MFG and one in deep MFG–SFG) (Figure 2).
Twenty (83.3%) patients had a transient deterioration of their language function after surgery (mean postoperative SST = 14.67 ± 0.76). Both the expressive (−2.875 ± 0.55) and the receptive (−1.36 ± 0.37) components of the SST deteriorated, with a statistically significant greater deterioration of the expressive component (p = 0.03). The single involvement of a particular gyrus (including area 55b) was not related per se with significant changes in language outcomes. The number of gyri with documented intraoperative positive language mapping was correlated with language outcomes: an increased number of gyri involvement was related with a better preoperative assessment (p = 0.037) and worse immediate language outcome (p = 0.029). This is mainly due to the changes in the expressive component of language (SST expressive preoperatively—p = 0.045) and SST expressive postoperatively−0.030). No significant changes were identified at the level of the receptive component of language. At the subcortical level, the involvement of the deep white matter of the SFG–MFG was related with worse expressive outcome postoperatively (p = 0.037), but no correlation was identified with the preoperative assessment (p = 0.780), and no overall impact was reflected in the overall SST assessment (SST preoperatively—p = 0.895; SST postoperatively−0.109). The preoperative nTMS mapping was not related with the language outcome (Table 4).
Table 4. Cortical and subcortical intraoperative involvement and pre- and postoperative language outcomes.
The inferior frontal gyrus and the “classical” Broca's area have been traditionally considered as the main language hub in the dominant frontal lobe. However, the findings from multiple intraoperative reports showed that the functional organization of the frontal lobe is more complex, with positive language sites described both at the level of the MFG and, to a lesser extent, in the SFG (34–39).
In recent years, there has been a renewed interest in the role of the MFG as part of the language network. This is particularly the case after the description of area 55b, located at the posterior aspect of the MFG (7). Previous studies have implicated the role of posterior MFG and area 55b in language (7, 9, 10). In the present series, we report a high rate of positive speech responses at the level of the MFG (45% of intraoperative errors).
Two points require further discussion: First, the high incidence of positive responses in the MFG were replicated with the use of preoperative nTMS in addition to intraoperative DES. In addition, the majority of positive language sites in the MFG were confined to the posterior aspect of the gyrus, covering the anatomical location of area 55b. We hypothesize that these results can be explained due to the involvement of area 55b when stimulating the posterior aspect of the MFG. This is consistent with previous descriptions of the intraoperative responses obtained at area 55b (9).
Second, the responses recorded in the MFG with the combined nTMS and DES were both phonological (hesitations and phonemic errors) and semantic (semantic paraphasias and anomias). The involvement of this area in both semantic processing (12) and speech articulation (40) has been well recognized. The results therefore show that the posterior MFG is likely implicated in both the “dorsal phonological” and the “ventral semantic” streams of language (36). The involvement of the posterior MFG area in both streams of language was also supported at the subcortical level, where again both phonological (speech arrest, hesitation, and fluency disturbance) and semantic disturbances (semantic paraphasia and anomia) were elicited while stimulating the white matter deep to the MFG.
The subcortical areas were divided into two main areas (IFG–MFG and MFG–SFG) as the included tumors all involved more than one subcortical area. The majority of the recorded errors at the subcortical level occurred in the IFG–MFG area (72.4%). Both the arcuate fasciculus (AF) and the inferior fronto-occipital fasciculus (IFOF) are known to cross deep to the IFG–MFG area, with terminations at the level of the posterior MFG. AF, the main dorsal stream fasciculus, has terminations in the MFG documented by anatomical cadaveric, diffusion imaging, and resting-state fMRI studies. It is reported that up to 56% of patients can have terminations of the AF in the MFG, particularly the long segment (41–44). From a ventral stream perspective, multiple components of the IFOF were proposed based on anatomical studies (45), DES (46), and diffusion imaging (47). These methods concur that this tract has a termination in the posterior aspect of the MFG and therefore may serve as a substrate for the semantic errors identified in this area (45). The errors detected at the level of the MFG–SFG could be related to the stimulation of the fronto-aslant tract (hesitation and decreased fluency). This tract has been recently involved in the dorsal stream functions of language, particularly the fluency and initiation of speech (48–50).
In addition, the original structural connectivity data presented also support a strong connectivity of the MFG with the adjacent cortical gyri (IFG and SFG), likely mediated via U-fiber short association fibers. These findings are similar to those of other connectivity studies reported in the literature (7, 8). In this context, the interaction with the FEF in the anterior/middle frontal gyrus raises the possibility of a potential integration of visual recognition processes with speech production (51).
Therefore, two hypotheses can be formulated to support the interaction of posterior MFG and area 55b with both streams of language: a direct connectivity via relay of some subcomponents of both AF and IFOF and an indirect connectivity via the stimulation of U-fibers to the adjacent gyri (IFG and SMA). In addition to previous imaging and dissection data, recent nTMS data support a strong connectivity of language positive sites via the U-fiber system, supporting the indirect connectivity theory (52). The strong connectivity to the primary motor cortex further supports the potential role for hand movement integration in language (43) and the involvement of this area in the articulation and praxis of speech (9).
The impact of positive responses in the area 55b on clinical outcomes is difficult to establish, as these are usually associated with positive responses in either the IFG or SFG. Generally, the overall SST score and each of its components deteriorated temporarily after resection. Thus, the direct involvement of the posterior middle frontal gyrus and area 55b was not related with language outcome. However, the involvement of an increased number of gyri was related with better preoperative SST but with worse postoperative SST scores, particularly due to the receptive component. We believe that neuroplasticity within the language network can be partially responsible for these findings. A higher number of involved gyri may imply the involvement of preoperative adaptive mechanisms to maintain a high level of language function. However, at the same time, they may represent an overall stretched network that has a limited ability to recover from the hit provided by surgical resection and therefore linked to worse language outcomes. This natural process of adaptation has been seen in other systems of the human brain, such as the motor system (53), and further studies are required to ascertain if a similar process may be involved in the language connectivity and network.
There is evidence for language network plasticity in patients, given the intrinsic changes in the intra- and interhemispheric inhibition mechanisms altered by pathological conditions (54). Furthermore, multiple preoperative and intraoperative studies have documented the presence of language network plasticity, particularly in tumors with a long course and natural history, such as low-grade gliomas (55–59).
Despite it being acknowledged that there are different degrees of plasticity potential for different functions of language (60), we hypothesize that an increased number of frontal lobe gyri involved in language may act as a surrogate for the degree of plasticity and adaptation of the language network already present before surgery.
To this regard, preoperative speech mapping with nTMS can play an important role in detecting the extent of involvement of the different frontal gyri in language function, thus providing a useful tool for preoperative counseling. It is crucial to take a patient-centred approach in neuro-oncology in order to meet patient expectations with surgical and oncological treatment (61).
This study has the general limitations of a retrospective cohort study. The most significant one is the incomplete preoperative data for some of the patients included, where the posterior middle frontal gyrus and the area 55b were mapped intraoperatively. However, it provides evidence for the added value of the integration of preoperative advanced mapping and intraoperative language mapping of area 55b and further establishes this area within the MFG as a potential relay for both ventral and dorsal streams of language.
This case series suggests that the posterior MFG, including area 55b, is an important integration cortical hub for both dorsal and ventral streams of language. It demonstrates this area as a cluster of positive responses in the MFG for both preoperative nTMS and intraoperative DES language mapping with a potential impact on language outcomes in dominant frontal lobe surgery.
The original contributions presented in the study are included in the article/Supplementary Material, further inquiries can be directed to the corresponding author/s.
Written informed consent was obtained from the individual(s) for the publication of any potentially identifiable images or data included in this article.
SH and MA: data collection, data analysis, literature review, and manuscript writing. JL: data collection, data analysis, literature review, manuscript writing, and statistical analysis. SP: data collection (nTMS, demographics), and written part of methodology. HW: reviewed speech and language data, written part of methodology. OL, CS, HI, AM, and SO: area 55b seed, connectivity data. JL, AK-V, RG, KA, and RB: reviewed manuscript. FV: principal manuscript reviewer, innovative concepts and ideas. All authors contributed to the article and approved the submitted version.
OL was funded by the EPSRC Research Council (EPSRC DTP EP/R513064/1). HI and CS were supported by the EPSRC-funded UCL Centre for Doctoral Training in Medical Imaging (EP/L016478/1).
The authors declare that the research was conducted in the absence of any commercial or financial relationships that could be construed as a potential conflict of interest.
The Supplementary Material for this article can be found online at: https://www.frontiersin.org/articles/10.3389/fneur.2021.646075/full#supplementary-material
Supplementary Material 1. Tractography. Diffusion and T1-weighted data were pre-processed using the framework described by Mancini et al. (62). Multi-fiber orientations were estimated using single-shell two-tissue constrained spherical deconvolution using order lmax = 8 (63). Probabilistic tractography was done using Second-order integration over Fiber Orientation Distributions (iFOD2) (63) and seeding randomly from the white-matter/grey-matter interface, both to reconstruct whole-brain (FOD amplitude cut-off = 0.05 and total_streamlines = 10 million) and region-of-interest (ROI) based tractography (FOD amplitude cut-off = 0.05 and total_streamlines = 1,000). ROI-based tractography relied on reconstructing the arcuate fasciculus (AF) tract from the left side of the brain based on similar pipeline introduced in (62). The posterior MFG was used to filter the reconstructed AF and its connectivity analysis. Additionally, qualitative results are shown in Figure 3. For surgical planning purposes, region of interest based tractography using subcortical anatomical areas was performed as described by Fekonja et al., in (64). The nTMS responses were overlayed over the dissected tracts considered for language network.
Supplementary Video 1. Preoperative language mapping (nTMS assessment) demonstrating language errors.
1. Brodmann K. Vergleichende Lokalisationslehre der Grosshirnrinde in ihren Prinzipien dargestellt auf Grund des Zellenbaues (English Translation by Garey, L.J.: Brodmann's Localization in the Cerebral Cortex; Smith Gordon, London, 1994), Barth (1909).
2. von Economo CF, Koskinas GN. Die cytoarchitektonik der hirnrinde des erwachsenen menschen. London: Springer (1925).
3. Vogt C, Vogt O. Allgemeinere Ergebnisse unserer Hirnforschung. J Psychol Neurol. (1919) 25:279–462.
4. Hopf A. Distribution of myeloarchitectonic marks in the frontal cerebral cortex in man. J Hirnforschung. (1956) 2:311–33.
5. Nieuwenhuys R. The myeloarchitectonic studies on the human cerebral cortex of the Vogt-Vogt school, and their significance for the interpretation of functional neuroimaging data. Brain Struct Func. (2013) 218:303–52. doi: 10.1007/s00429-012-0460-z
6. Van Essen DC, Glasser MF, Dierker DL, Harwell J, Coalson T. Parcellations and hemispheric asymmetries of human cerebral cortex analyzed on surface-based atlases. Cerebral Cortex. (2012) 22:2241–62. doi: 10.1093/cercor/bhr291
7. Glasser MF, Coalson TS, Robinson EC, Hacker CD, Harwell J, Yacoub E, et al. A multi-modal parcellation of human cerebral cortex. Nature. (2016) 536:171–8. doi: 10.1038/nature18933
8. Baker CM, Burks JD, Briggs RG, Sheets JR, Conner AK, Glenn CA, et al. A connectomic Atlas of the human cerebrum-chapter 3: the motor, premotor, and sensory cortices. Operat Neurosurg. (2018) 15 (suppl_1), S75–S121. doi: 10.1093/ons/opy256
9. Chang EF, Kurteff G, Andrews JP, Briggs RG, Conner AK, Battiste JD, et al. Pure apraxia of speech after resection based in the posterior middle frontal gyrus. Neurosurgery. (2020) 87:E383–9. doi: 10.1093/neuros/nyaa002
10. Donahue CJ, Glasser MF, Preuss TM, Rilling JK, Van Essen DC. Quantitative assessment of prefrontal cortex in humans relative to nonhuman primates. Proc Natl Acad Sci. (2018) 115:E5183–92. doi: 10.1073/pnas.1721653115
11. De Witt Hamer PC, Robles SG, Zwinderman AH, Duffau H, Berger MS. Impact of intraoperative stimulation brain mapping on glioma surgery outcome: a meta-analysis. J Clin Oncol. (2012) 30:2559–65. doi: 10.1200/JCO.2011.38.4818
12. Sarubbo S, Tate M, De Benedictis A, Merler S, Moritz-Gasser S, Herbet G, et al. Mapping critical cortical hubs and white matter pathways by direct electrical stimulation: an original functional atlas of the human brain. Neuroimage. (2020) 205:116237. doi: 10.1016/j.neuroimage.2019.116237
13. Mandonnet E, Winkler PA, Duffau H. Direct electrical stimulation as an input gate into brain functional networks: principles, advantages and limitations. Acta Neurochirurgica. (2010) 152:185–93. doi: 10.1007/s00701-009-0469-0
14. Borchers S, Himmelbach M, Logothetis N, Karnath HO. Direct electrical stimulation of human cortex - the gold standard for mapping brain functions? Nat Rev Neurosci. (2011) 13:63–70. doi: 10.1038/nrn3140
15. Pallud J, Zanello M, Kuchcinski G, Roux A, Muto J, Mellerio C, et al. Individual variability of the human cerebral cortex identified using intraoperative mapping. World Neurosurg. (2018) 109:e313–7. doi: 10.1016/j.wneu.2017.09.170
16. Forster M-T, Hattingen E, Senft C, Gasser T, Seifert V, Szelényi A. Navigated transcranial magnetic stimulation and functional magnetic resonance imaging: advanced adjuncts in preoperative planning for central region tumors. Neurosurgery. (2011) 68:1317–25. doi: 10.1227/NEU.0b013e31820b528c
17. Picht T, Schmidt S, Brandt S, Frey D, Hannula H, Neuvonen T, et al. Preoperative functional mapping for rolandic brain tumour surgery: comparison of navigated transcranial magnetic stimulation to direct cortical stimulation. Neurosurgery. (2011) 69:581–8. Discussion 588. doi: 10.1227/NEU.0b013e3182181b89
18. Picht T, Frey D, Thieme S, Kliesch S, Vajkoczy P. Presurgical navigated TMS motor cortex mapping improves outcome in glioblastoma surgery: a controlled observational study. J Neurooncol. (2016) 126:535–43. doi: 10.1007/s11060-015-1993-9
19. Krieg SM, Sabih J, Bulubasova L, Obermueller T, Negwer C, Janssen I, et al. Preoperative motor mapping by navigated transcranial magnetic brain stimulation improves outcome for motor eloquent lesions. Neurooncology. (2014) 16:1274–82. doi: 10.1093/neuonc/nou007
20. Raffa G, Scibilia A, Conti A, Ricciardo G, Rizzo V, Morelli A, et al. The role of navigated transcranial magnetic stimulation for surgery of motor-eloquent brain tumors: a systematic review and metaanalysis. Clin Neurol Neurosurg. (2019) 180:7–17. doi: 10.1016/j.clineuro.2019.03.003
21. Raffa G, Picht T, Scibilia A, Rösler J, Rein J, Conti A, et al. Surgical treatment of meningiomas located in the rolandic area: the role of navigated transcranial magnetic stimulation for preoperative planning, surgical strategy, and prediction of arachnoidal cleavage and motor outcome. J Neurosurg. (2019) 14:1–12. doi: 10.3171/2019.3.JNS183411
22. Sollmann N, Picht T, Mäkelä JP, Meyer B, Ringel F, Krieg SM. Navigated transcranial magnetic stimulation for preoperative language mapping in a patient with a left frontoopercular glioblastoma. J Neurosurg. (2013) 118:175–9. doi: 10.3171/2012.9.JNS121053
23. Krieg SM, Lioumis P, Mäkelä JP, Wilenius J, Karhu J, Hannula H, et al. Protocol for motor and language mapping by navigated TMS in patients and healthy volunteers; workshop report. Acta Neurochirurgica. (2017) 159:1187–95. doi: 10.1007/s00701-017-3187-z
24. Jeltema HR, Ohlerth AK, de Wit A, Wagemakers M, Rofes A, Bastiaanse R, et al. Comparing navigated transcranial magnetic stimulation mapping and “gold standard” direct cortical stimulation mapping in neurosurgery: a systematic review. Neurosurg Rev. (2020). doi: 10.1007/s10143-020-01397-x
25. Bährend I, Muench MR, Schneider H, Moshourab R, Dreyer FR, Vajkoczy P, et al. Incidence and linguistic quality of speech errors: a comparison of preoperative transcranial magnetic stimulation and intraoperative direct cortex stimulation. J Neurosurg. (2020) 29:1–10. doi: 10.3171/2020.3.JNS193085
26. Krieg SM, Tarapore PE, Picht T, Tanigawa N, Houde J, Sollmann N, et al. Optimal timing of pulse onset for language mapping with navigated repetitive transcranial magnetic stimulation. Neuroimage. (2014) 100:219–36. doi: 10.1016/j.neuroimage.2014.06.016
27. Raffa G, Quattropani MC, Germanò A. When imaging meets neurophysiology: the value of navigated transcranial magnetic stimulation for preoperative neurophysiological mapping prior to brain tumor surgery. Neurosurg Focus. (2019) 47:E10. doi: 10.3171/2019.9.FOCUS19640
28. Syder D, Body R, Parker M, Boddy M. Sheffield Screening Test for Acquired Language Disorders. Windsor: NFER-NELSON (1993).
29. Simpson F, Christie J, Mortensen L, Clark W. Mount Wilga High Level Language Test: Administration & Scoring Manual Plus Test Form with UK Adaptations and Large Print Additions. (2006). Available online at: http://nebula.wsimg.com/5dc06d53fe8a246679ba15f02e226ed0?AccessKeyId=5861B1733117182DC99B&disposition=0&alloworigin=1
30. Penfield W, Boldrey E. Somatic motor and sensory representation in the cerebral cortex of man as studied by electrical stimulation. Brain. (1937) 60:389–443. doi: 10.1093/brain/60.4.389
31. De E, Satoer D, Robert E, Colle H, Verheyen S, Visch-Brink E, et al. The Dutch linguistic intraoperative protocol: a valid linguistic approach to awake brain surgery. Brain Lang. (2015) 140:35–48. doi: 10.1016/j.bandl.2014.10.011
32. Ohlerth AK, Valentin A, Vergani F, Ashkan K, Bastiaanse R. The verb and noun test for peri-operative testing (VAN-POP): standardized language tests for navigated transcranial magnetic stimulation and direct electrical stimulation. Acta Neurochirurgica. (2020) 162:397–406. doi: 10.1007/s00701-019-04159-x
33. Jung J, Lavrador JP, Patel S, Giamouriadis A, Lam J, Bhangoo R, et al. First United Kingdom experience of navigated transcranial magnetic stimulation in preoperative mapping of brain tumors. World Neurosurg. (2019) 122:e1578–87. doi: 10.1016/j.wneu.2018.11.114
34. Sanai N, Mirzadeh Z, Berger MS. Functional outcome after language mapping for glioma resection. N Engl J Med. (2008) 358:18–27. doi: 10.1056/NEJMoa067819
35. Sarubbo S, De Benedictis A, Merler S, Mandonnet E, Balbi S, Granieri E, et al. Towards a functional atlas of human white matter. Hum Brain Map. (2015) 36:3117–36. doi: 10.1002/hbm.22832
36. Sarubbo S, De Benedictis A, Merler S, Mandonnet E, Barbareschi M, Dallabona M, et al. Structural and functional integration between dorsal and ventral language streams as revealed by blunt dissection and direct electrical stimulation. Hum Brain Map. (2016) 37:3858–72. doi: 10.1002/hbm.23281
37. Gonen T, Gazit T, Korn A, Kirschner A, Perry D, Hendler T, et al. Intra-operative multi-site stimulation: Expanding methodology for cortical brain mapping of language functions. PLoS ONE. (2017) 12:e0f180740. doi: 10.1371/journal.pone.0180740
38. Duffau H. The error of Broca: from the traditional localizationist concept to a connectomal anatomy of human brain. J Chem Neuroanat. (2017) 89:73–81. doi: 10.1016/j.jchemneu.2017.04.003
39. Rahimpour S, Haglund MM, Friedman AH, Duffau H. History of awake mapping and speech and language localization: from modules to networks. Neurosurg Focus. (2019) 47:E4. doi: 10.3171/2019.7.FOCUS19347
40. Duffau H, Gatignol P, Mandonnet E, Peruzzi P, Tzourio-Mazoyer N, Capelle L. New insights into the anatomo-functional connectivity of the semantic system: a study using cortico-subcortical electrostimulations. Brain J Neurol. (2005) 128 (Pt 4):797–810. doi: 10.1093/brain/awh423
41. Catani M, Jones DK, Ffytche DH. Perisylvian language networks of the human brain. Ann Neurol. (2005) 57:8–16. doi: 10.1002/ana.20319
42. Martino J, De Witt Hamer PC, Berger MS, Lawton MT, Arnold CM, de Lucas EM, et al. Analysis of the subcomponents and cortical terminations of the perisylvian superior longitudinal fasciculus: a fiber dissection and DTI tractography study. Brain Struct Funct. (2013) 218:105–21. doi: 10.1007/s00429-012-0386-5
43. Barbeau EB, Descoteaux M, Petrides M. Dissociating the white matter tracts connecting the temporo-parietal cortical region with frontal cortex using diffusion tractography. Sci Rep. (2020) 10:8186. doi: 10.1038/s41598-020-64124-y
44. Yagmurlu K, Middlebrooks EH, Tanriover N, Rhoton AL Jr. Fiber tracts of the dorsal language stream in the human brain. J Neurosurg. (2016) 124:1396–405. doi: 10.3171/2015.5.JNS15455
45. Sarubbo S, De Benedictis A, Maldonado IL, Basso G, Duffau H. Frontal terminations for the inferior fronto-occipital fascicle: anatomical dissection, DTI study and functional considerations on a multi-component bundle. Brain Struct Funct. (2013) 218:21–37. doi: 10.1007/s00429-011-0372-3
46. Martino J, Brogna C, Robles SG, Vergani F, Duffau H. Anatomic dissection of the inferior fronto-occipital fasciculus revisited in the lights of brain stimulation data. Cortex. (2010) 46:691–9. doi: 10.1016/j.cortex.2009.07.015
47. Wu Y, Sun D, Wang Y, Wang Y. Subcomponents and connectivity of the inferior fronto-occipital fasciculus revealed by diffusion spectrum imaging fiber tracking. Front Neuroanat. (2016) 10:88. doi: 10.3389/fnana.2016.00088
48. Catani M, Mesulam MM, Jakobsen E, Malik F, Martersteck A, Wieneke C, et al. A novel frontal pathway underlies verbal fluency in primary progressive aphasia. Brain J Neurol. (2013). 136 (Pt 8):2619–28. doi: 10.1093/brain/awt163
49. Vergani F, Lacerda L, Martino J, Attems J, Morris C, Mitchell P, et al. White matter connections of the supplementary motor area in humans. J Neurol Neurosurg Psychiatry. (2014) 85:1377–85. doi: 10.1136/jnnp-2013-307492
50. Kinoshita M, de Champfleur NM, Deverdun J, Moritz-Gasser S, Herbet G, Duffau H. Role of fronto-striatal tract and frontal aslant tract in movement and speech: an axonal mapping study. Brain Struct Funct. (2015) 220:3399–412. doi: 10.1007/s00429-014-0863-0
51. Genon S, Reid A, Li H, Fan L, Müller VI, Cieslik EC, et al. Preoperative transcranial magnetic stimulation for picture naming is reliable in mapping segments of the arcuate fasciculus. Brain Commun. (2020) 2:fcaa158. doi: 10.1093/braincomms/fcaa158
52. Zhang H, Schramm S, Schröder A, Zimmer C, Meyer B, Krieg SM, et al. Function-based tractography of the language network correlates with aphasia in patients with language-eloquent glioblastoma. Brain Sci. (2020) 10:412. doi: 10.3390/brainsci10070412
53. Zdunczyk A, Schwarzer V, Mikhailov M, Bagley B, Rosenstock T, Picht T, et al. The corticospinal reserve capacity: reorganization of motor area and excitability as a novel pathophysiological concept in cervical myelopathy. Neurosurgery. (2018) 83:810–8. doi: 10.1093/neuros/nyx437
54. Tzourio-Mazoyer N, Perrone-Bertolotti M, Jobard G, Mazoyer B, Baciu M. Multi-factorial modulation of hemispheric specialization and plasticity for language in healthy and pathological conditions: a review. Cortex. (2017) 86:314–39. doi: 10.1016/j.cortex.2016.05.013
55. Raffa G, Quattropani MC, Scibilia A, Conti A, Angileri FF, Esposito F, et al. Surgery of language-eloquent tumors in patients not eligible for awake surgery: the impact of a protocol based on navigated transcranial magnetic stimulation on presurgical planning and language outcome, with evidence of tumor-induced intra-hemispheric plasticity. Clin. Neurol. Neurosurg. (2018) 168:127–39. doi: 10.1016/j.clineuro.2018.03.009
56. Zheng G, Chen X, Xu B, Zhang J, Lv X, Li J, et al. Plasticity of language pathways in patients with low-grade glioma: a diffusion tensor imaging study. Neural Regen Res. (2013) 8:647–54. doi: 10.3969/j.issn.1673-5374.2013.07.009
57. Cirillo S, Caulo M, Pieri V, Falini A, Castellano A. Role of functional imaging techniques to assess motor and language cortical plasticity in glioma patients: a systematic review. Neural Plast. (2019) 2019:4056436. doi: 10.1155/2019/4056436
58. Southwell DG, Hervey-Jumper SL, Perry DW, Berger MS. Intraoperative mapping during repeat awake craniotomy reveals the functional plasticity of adult cortex. J Neurosurg. (2016) 124:1460–9. doi: 10.3171/2015.5.JNS142833
59. Duffau H. Mapping the connectome in awake surgery for gliomas: an update. J Neurosurg Sci. (2017) 61:612–30.
60. van Geemen K, Herbet G, Moritz-Gasser S, Duffau H. Limited plastic potential of the left ventral premotor cortex in speech articulation: evidence from intraoperative awake mapping in glioma patients. Hum Brain Map. (2014) 35:1587–96. doi: 10.1002/hbm.22275
61. Lavrador JP, Ghimire P, Brogna C, Furlanetti L, Patel S, Gullan R, et al. Pre- and intraoperative mapping for tumors in the primary motor cortex: decision-making process in surgical resection. J Neurol Surg A Cent Eur Neurosurg. (2020). doi: 10.1055/s-0040-1709729
62. Mancini M, Casamitjana A, Peter L, Robinson E, Crampsie S, Thomas DL, et al. A multimodal computational pipeline for 3D histology of the human brain. Sci. Rep. (2020) 10:13839. doi: 10.1038/s41598-020-69163-z
63. Tournier JD, Smith R, Raffelt D, Tabbara R, Dhollander T, Pietsch M, et al. MRtrix3: A fast, flexible and open software framework for medical image processing and visualisation. Neuroimage. (2019) 202:116137. doi: 10.1016/j.neuroimage.2019.116137
Keywords: area 55b, language mapping, speech arrest, perioperative mapping, DTI, TMS, language network, nTMS
Citation: Hazem SR, Awan M, Lavrador JP, Patel S, Wren HM, Lucena O, Semedo C, Irzan H, Melbourne A, Ourselin S, Shapey J, Kailaya-Vasan A, Gullan R, Ashkan K, Bhangoo R and Vergani F (2021) Middle Frontal Gyrus and Area 55b: Perioperative Mapping and Language Outcomes. Front. Neurol. 12:646075. doi: 10.3389/fneur.2021.646075
Received: 24 December 2020; Accepted: 29 January 2021;
Published: 10 March 2021.
Edited by:
Giovanni Raffa, University of Messina, ItalyReviewed by:
Cesare Zoia, Fondazione IRCCS Policlinico San Matteo, ItalyCopyright © 2021 Hazem, Awan, Lavrador, Patel, Wren, Lucena, Semedo, Irzan, Melbourne, Ourselin, Shapey, Kailaya-Vasan, Gullan, Ashkan, Bhangoo and Vergani. This is an open-access article distributed under the terms of the Creative Commons Attribution License (CC BY). The use, distribution or reproduction in other forums is permitted, provided the original author(s) and the copyright owner(s) are credited and that the original publication in this journal is cited, in accordance with accepted academic practice. No use, distribution or reproduction is permitted which does not comply with these terms.
*Correspondence: Sally Rosario Hazem, c2FsbHkuaGF6ZW1AbmhzLm5ldA==; Mariam Awan, bS5hd2FuMUBuaHMubmV0
†These authors have contributed equally to this work
Disclaimer: All claims expressed in this article are solely those of the authors and do not necessarily represent those of their affiliated organizations, or those of the publisher, the editors and the reviewers. Any product that may be evaluated in this article or claim that may be made by its manufacturer is not guaranteed or endorsed by the publisher.
Research integrity at Frontiers
Learn more about the work of our research integrity team to safeguard the quality of each article we publish.