- 1Department of Neurology, Secondary Medical Center, National Clinical Research Center for Geriatric Disease, Chinese PLA General Hospital, Beijing, China
- 2Department of Neurology, First Medical Center, Chinese PLA General Hospital, Beijing, China
- 3Department of Medical Psychology, Eighth Medical Center, Chinese PLA General Hospital, Beijing, China
- 4School of Biological Science and Medical Engineering, Beihang University, Beijing, China
- 5Department of Psychology, Beijing Sport University, Beijing, China
Objective: The thalamus is a key node for sleep-wake pathway gate switching during acute sleep deprivation (ASD), and studies have shown that it plays a certain role in emotion changes. However, there are no studies on the association between the thalamus and emotion changes in ASD. In this study, we used resting-state functional magnetic resonance imaging (R-fMRI) to explore whether changes in the functional connections between the thalamus and other brain regions are related to emotion changes and further explored the function of the thalamus under total ASD conditions.
Method: Thirty healthy, right-handed adult men underwent emotional assessment according to the Profile of Mood States Scale and R-fMRI scans before and after ASD. The correlations between changes in functional connectivity between the thalamus and other brain regions and emotion changes were then studied.
Results: Positive emotions and psychomotor performance were reduced, and negative emotions were increased following ASD. The functional connections between the left thalamus and left middle temporal gyrus, left inferior frontal gyrus, right thalamus, right inferior temporal gyrus, left middle temporal pole gyrus, right calcarine, left cuneus, left rectus and left medial superior frontal gyrus were significantly altered. Decreased functional connectivity between left thalamus and left inferior frontal gyrus related to emotion changes following ASD.
Conclusion: This study finds that functional changes in the thalamus are associated with emotion changes during ASD, suggesting that the left thalamus probably plays an essential role in emotion changes under ASD conditions.
Introduction
“Sleep deprivation” can be summarized as less sleep than is usually required. It can be acute or chronic, with the effects of a small amount of sleep deprivation accumulating over days, weeks or longer (1). With the rapid development of social modernization, people often actively or passively suffer from the adverse effects of chronic sleep deprivation (CSD) and acute sleep deprivation (ASD) (2, 3). Sleep deprivation leads to a series of neurological and behavioral changes that can significantly interfere with the brain's cognitive and emotional abilities. The short-term consequences of sleep deprivation include increased stress response, physical pain, decreased quality of life, emotional disorders and performance impairment (4), while the long-term consequences of sleep deprivation in healthy individuals include cardiovascular disease, weight-related problems, metabolic syndrome, dyslipidemia, and colorectal cancer (4). There is also an increase in all-cause mortality among men suffering from sleep deprivation (4). Furthermore, following sleep deprivation, brain function is significantly impaired in terms of attention, decision-making, spatial navigation, working memory and emotional and social processing (5, 6).
It is well known that sleep plays a critical role in emotional processing and regulation (5). Functional magnetic resonance imaging (fMRI) studies have shown that sleep-deprived people have altered emotional brain networks, mainly in the limbic system (5). Compared with healthy people, the volume, activity and functional connections of the amygdala, insula, cingulate area and prefrontal lobe in patients with emotional disorders, such as various types of anxiety and bipolar disorder, are significantly changed, which further confirms that these are the main brain areas responsible for the related emotions (7–9). Correlations between these brain regions and emotion have mainly been identified in studies of people with CSD, but a growing number of studies show that ASD has a wide range of effects on emotion (10, 11). Further research shows that the amygdala, anterior insula, medial prefrontal cortex and anterior cingulate cortex are also significantly altered under ASD, and associated with emotion changes caused by ASD (6, 12). The above research findings provide preliminary evidence that the areas of the brain associated with emotion changes are largely the same in both ASD and CSD.
All brain regions are affected by emotions (13). However, the research on human brain by fMRI mainly focuses on the higher cortical regions, and there are few studies on the involvement of subcortical structures in emotional changes. As an important structure involved in the sleep-wake pathway, the thalamus has been shown to be involved in alert-related brain cognitive functions (6). And in certain chronic progressive diseases such as anxiety disorder and insomnia, the thalamus is involved in the emotional neural networks. Research into the emotional dysregulation circuit shows that the thalamus plays a particular role in emotion changes (14, 15). Furthermore, studies have shown that CSD (insomnia, etc.) can cause functional changes in the thalamic-emotional core region (16, 17). To date, the emotional role of the chronically sleep-deprived thalamus has been documented, but the fact that the thalamus is involved in emotion changes has not been adequately studied. At the same time, there were almost no studies have investigated the emotional role of the thalamus during ASD. While the vast majority of studies show that the thalamus plays a key central role in the sleep-wake pathway and involved in a variety of brain cognitive functions (6, 18–21), and the thalamus is located anatomically in the core area of the brain, Therefore, there is good evidence to speculate that the thalamus may play a unique role in emotion changes caused by ASD. However, ASD and insomnia show extensive changes in the microstructure of gray matter and share common but different neurobiological characteristics in brain morphology (22). It is necessary to provide direct evidence to test this hypothesis.
We hypothesized that the functional connections between the thalamus and brain regions proven to be associated with emotion would be altered following ASD, and the functional changes between the thalamus and other regions would correlate with the emotion changes. To test this hypothesis, we devised a within-subject statistical design of 36 h total ASD, then used f-MRI to assess whether changes in the functional connections between the thalamus and other brain regions were correlated with emotion changes under ASD conditions.
Materials and Methods
Participants
We recruited 30 young male college students (right-handed, age range: 20–30 years) and offered them a financial reward to participate in this study. During the recruitment process, we explained in detail the main purpose of the study and its whole process, as well as the possible risks and countermeasures. All subjects voluntarily signed their informed consent. Prior to the beginning of the experiment, we invited qualified Chinese specialists to conduct standardized physical examinations. The main forms of medical examination were subjective and objective physical examinations, as well as related self-reporting scale tests, to exclude subjects who may have serious diseases or be at risk of accidents during the experiment. The inclusion criteria we set were as follows: ① No disease history of the circulatory system or respiratory system, no nervous system structure or function impairment, and no history of severe infectious disease, mental disorder or sleep disorder; ② No colds or other diseases in progress that may affect the experiment; ③ The subjects had regular daily life and rest habits, and their score was <7 on the Pittsburgh Sleep Quality Index (PSQI) scale; ④ No significant events leading to the possible emotional fluctuation of the subjects had occurred within 1 month before the experiment; ⑤ Subjects were required to carry out their daily activities in accordance with the standard procedures in the week before the start of the experiment, and advised to refrain from consuming stimulating beverages, carbonated beverages, tea, coffee and certain foods, as well as refraining from smoking. The study was approved by the Research Ethics Committee of Beihang University (Beijing, China). We conducted it in strict accordance with the protocol approved by the Ethics Committee, and strictly followed the requirements of the Hellenic Declaration.
Behavioral Measures
In this study, we used the Profile of Mood States Scale (POMS) to assess emotion changes (23). The advantage of this scale is that it can provide a reasonable and timely assessment of emotion changes through changes in the score. It consists of a questionnaire containing 65 items over six sections: anxiety, anger, fatigue, depression, confusion, and vitality. The scores for these items range from 0 (not at all) to 4 (very). According to the scoring principle, the scores for the 65 items are added to obtain the scores for the six sections. The top five sections form the negative emotional state assessment, and the sixth section is the positive emotional state assessment. The total score is the sum of the top five sections minus the score for the sixth section. The higher the negative emotion score, the more serious the emotional disorder, while the higher the positive score, the better the emotional state. In our study, Cronbach's alpha coefficients were satisfactory (Cronbach's alpha is 0.909).
We used the Chinese version of the PSQI, which has good psychometric properties (24). The PSQI is a self-reporting questionnaire which measures sleep quality over the previous month (25). Scores for each sleep item range from 0 (not at all) to 3 (maximum dysfunction). The scores for the sleep items are then added according to the coefficient to obtain the overall sleep quality score. We classified PSQI scores of equal to or >7 as low sleep quality.
Procedures
The design of the experiment is presented in Figure 1.
We experimented in the sleep laboratory of Beihang University and the magnetic resonance room of the General Hospital of the People's Liberation Army of China. All subjects participating in the experiment were divided into four batches to monitor their status more accurately and reduce the bias caused by an excessive number of subjects during the scale assessment and R-fMRI scan. During the experiment, we ensured that there were two experimenters (no fewer than one operator with a medical qualification) to guide the subjects to complete the relevant experiment contents and supervise their physical health status and experiment cooperation degree.
After preparing for the experiment in their living quarters, the subjects arrived at the laboratory at 8:00 on the first day of the experiment. They wore exercise watches to record their activity and sleep during the experiment. In the daytime of Day 1, they familiarized themselves with the experimental site and completed the evaluation. From 22:00 on Day 1 to 8:00 on Day 2, the subjects slept for at least 8 h under the supervision of the operator. After these preparations were completed, ASD started at 8:00 on Day 2 and ended at 20:00 on Day 3, during which time all subjects completed 36 h of continuous sleep deprivation. During the experiment, subjects were allowed to carry out daily activities, including playing games, reading, sitting, eating, and chatting. During the whole experiment stage, especially during the sleep deprivation stage, at least two experimenters were on hand at the same time to monitor the status of the subjects, thereby avoiding the occurrence of influencing factors such as naps during the sleep deprivation period.
We performed two R-fMRI scans during rested wakefulness (RW), and they were carried out at least 3 weeks apart to dull the effects of the exercise. The R-fMRI scan during RW used the same scan sequence as the R-fMRI scan during ASD and was performed within the same period, while the subjects completed their POMS evaluation immediately before each scan. The two scans were performed by a 3T Siemens MAGNETOM Skyra (Siemens Medical Solutions, Germany) located in the General Hospital of the People's Liberation Army. During each scan, the T1 sequence was scanned first to obtain high resolution T1-weighted anatomical images (176 images). Next, R-fMRI data collection was performed for 8 min (240 images per time). At the beginning of the scan, the subjects were asked to lie on their backs on an MRI bed with their heads fixed in a sponge and bandage. During the scan, the subjects were told to close their eyes, think of nothing and try to keep their heads and bodies still. To ensure that the subjects were awake during the scan, the operator communicated with them through a microphone before each scan to remind them to stay awake, and they were again asked if they were awake between scans of different sequences. Throughout each scan, the operator monitored the subject's body movements and other states through the viewing window. After each scan, the subjects were asked if they had been awake during the scan.
Data Processing
We used CONN toolbox software (Version 18a, Neuroimaging Informatics Tools and Resources Clearinghouse; http://www.nitrc.org/projects/conn) and SPM software (Version 12, University College London, http://www.fil.ion.ucl.ac.uk/spm) to preprocess the R-fMRI images. The two types of software are both cross-platform software based on MATLAB (Version R2018a, MathWorks, Inc. United States). First, the T1-weighted images underwent translation, segmentation and MNI normalization. Second, the first 10 volumes of the functional images were disregarded. Third, functional slice-timing correction and subject motion estimation and correction were carried out. We excluded all subjects whose mean head movements were more than 2 mm and 2°. While in the remaining subjects, the images were deleted if the head moved more than 0.5° or 0.5 mm from the adjacent images. Finally, functional indirect segmentation and normalization were carried out. In the space standardization step, the functional images were indirectly normalized to the standard space through the corresponding structural images, and the normalized bias correction is generated. The EPI template was used to normalize the structural images directly into the standard Montreal Neurological Institute (MNI) 152 standard space (voxel size of 3 × 3 × 3 mm). The full width at half maximum in smoothing was 6 mm, and the low frequency filter was 0.01–0.08 Hz. Fisher's transformation was used to normalize the distribution of variables (26, 27).
Functional Connectivity Analysis
A measure of functional connectivity between each pair of seed regions was typically calculated by region of interest to the region of interest (ROI-to-ROI) analysis. The seed regions to be studied were selected using the CONN toolbox's Automated Anatomical Labeling (AAL), and the location of these seed points was based on the details given by Tzourio-Mazoyer (28). A total of 90 seed regions were selected through AAL (18 seeds were removed from the cerebellar region). We then calculated the functional connectivity of the left and right thalami to the other seed regions separately for each subject. Next, Pearson correlation analysis was performed on the time series of each seed region. Finally, the Fisher Z-transformation was used to transform the correlation coefficient of each voxel and smooth the obtained data. After several corrections to the dataset, the inter-condition effect was considered to be significant at p < 0.05, and the group-level false discovery rate (FDR) correction p-value was <0.05.
Behavioral Measures and Correlation Analysis
SPSS (Version 21.0, IBM, Inc., USA) software was used to process the collected POMS data and other demographic data. First, descriptive statistics were carried out on each index, and a normality test was carried out on each item result and total POMS score. The measurement data was presented in the form of mean ± standard deviation. Later, a paired sample t-test was used for each section score and total POMS score before and after ASD, and p < 0.05 was considered statistically significant. Finally, the changes in functional connectivity coefficient between the thalamus and various brain regions (p < 0.05, FDR-corrected) were compared with the difference between POMS scores before and after ASD. Under the premise that the p-value was < 0.05, we defined the correlation coefficients (r) ≤ 0.4 as low correlation, 0.4 < r ≤ 0.6 as moderate correlation, and 0.6 < r as high correlation.
Results
Initial Data Quality Assessment
In the demographic description, we gave a statistical description of 28 subjects with complete data. They were all males with an average age of 24.48 ± 2.57 years, average height of 175.93 ± 5.01 cm, and average Body Mass Index (BMI) of 23.64 ± 1.73. Their PSQI scores were all <7, and their average score was 3.37 ± 1.19.
Behavioral Measures and Correlation Analysis
We used POMS to assess emotion changes before and after ASD. These scores were in line with the normal distribution, and a paired sample t-test was used to measure emotion changes. Following ASD, there were statistically significant increases in scores for anxiety (t = 2.635, p = 0.014), anger (t = 2.066, p = 0.049), fatigue (t = 5.217, p < 0.001) and confusion (t = 4.719, p < 0.001), and there was a statistically significant decrease in scores for vitality (t = −6.464, p < 0.001). The total POMS score also showed a statistically significant increase (t = 6.215, p < 0.001). In addition, decrease in depression was not statistically significant (Table 1).
The effect sizes of functional connectivity between the thalamus and whole-brain ROIs significantly changed (p < 0.05, FDR-corrected) under ASD > RW conditions are shown in Table 2. The ROI-to-ROI analysis demonstrated a decreased functional connectivity between the thalamus and other brain regions mainly distributed in the frontal temporal lobe, including the left middle temporal gyrus (l-MTG), right middle temporal gyrus (r-MTG), left middle temporal pole gyrus (l-MTPG), right inferior temporal gyrus (r-ITG), left orbital inferior frontal gyrus (l-OrbIFG) and left opercular inferior frontal gyrus (l-OperIFG). In contrast, an increase in functional connectivity between the left thalamus (l-Tha), left medial superior frontal gyrus (l-MSFG), right thalamus (r-Tha), left cuneus (l-Cun) and right calcarine (r-Cal) occurred during ASD. Figure 2 shows the ROI-to-ROI functional connectivity of the left thalamus under RW, ASD and ASD > RW conditions. Figure 3 shows the ROI-to-ROI functional connectivity of the r-Tha under the three sets of conditions.
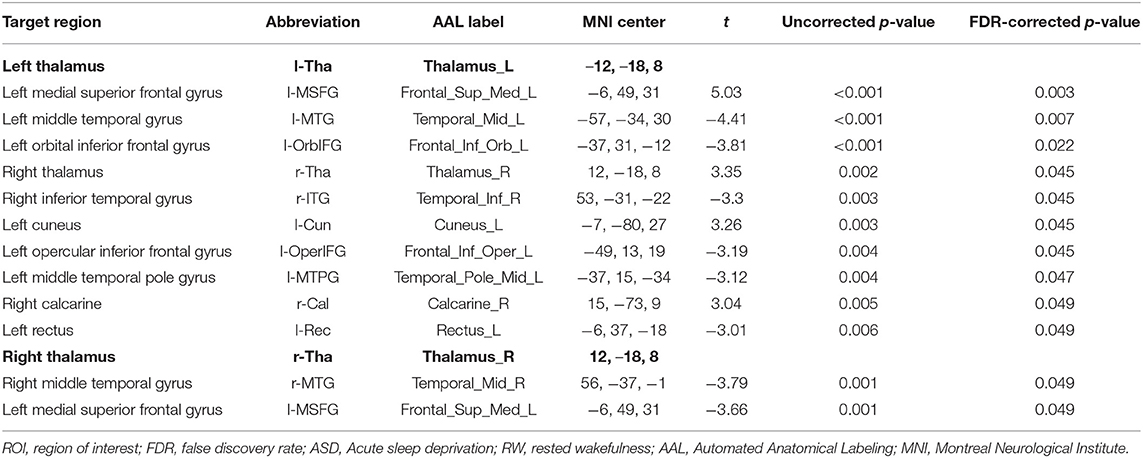
Table 2. ROI-to-ROI functional connectivity statistics: comparisons between RW and ASD scans (ASD > RW, paired t-test, n = 28).
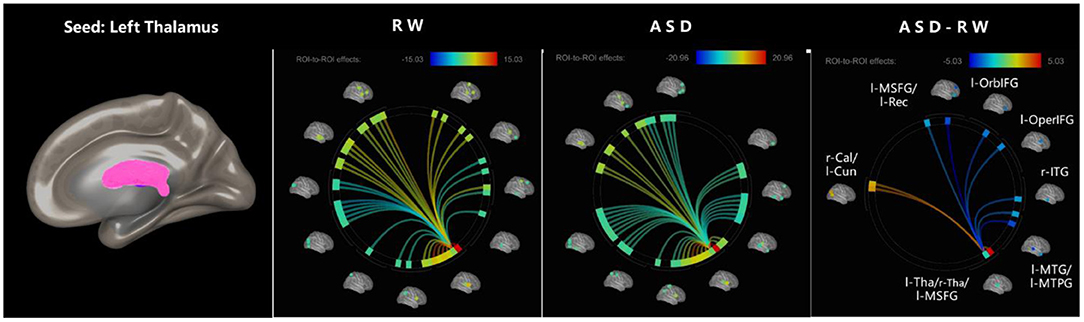
Figure 2. ROI-to-ROI functional connectivity of left thalamus during RW, ASD, and ASD > RW conditions. False discovery rate-corrected (p < 0.05) for ROI-to-ROI tests. The functional connectivity between l-Tha and l-OperIFG, l-OrbIFG, l-MTPG, l-Rec, l-MTG, and r-ITG decreased during ASD. The functional connectivity between l-Tha and l-Cun, r-Cal, l-MSFG, and r-Tha increased during ASD. l-MSFG, left medial superior frontal gyrus; l-MTPG, left middle temporal pole gyrus; l-OrbIFG, left orbital inferior frontal gyrus; l-OperIFG, left opercular inferior frontal gyrus; l-Rec, left rectus; l-MTG, left middle temporal gyrus; r-ITG, right inferior temporal gyrus; r-Tha, right thalamus; l-Cun, left cuneus; r-Cal, right calcarine; ROI, region of interest; ASD, acute sleep deprivation; RW, rested wakefulness.
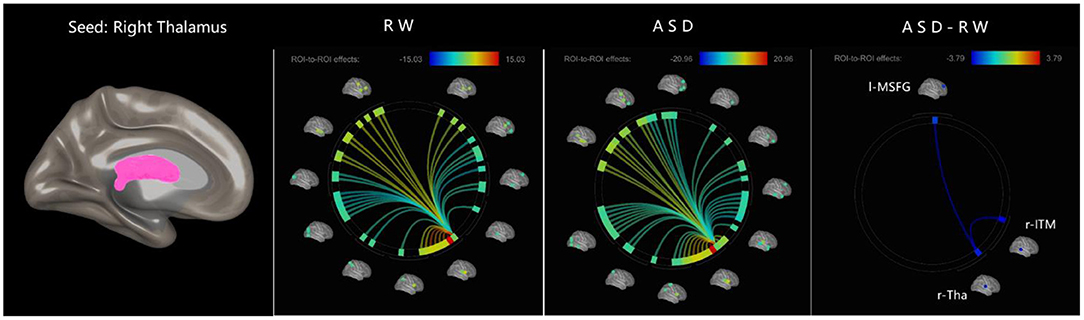
Figure 3. ROI-to-ROI functional connectivity of left thalamus during RW, ASD, and ASD > RW conditions. False discovery rate-corrected (p < 0.05) for ROI-to-ROI tests. The functional connectivity between r-Tha and r-ITM, l-MSFG decreased decreased during ASD. r-Tha, right thalamus; r-ITM, right middle temporal gyrus; l-MSFG, left medial superior frontal gyrus; ROI, region of interest; ASD, acute sleep deprivation; RW, rested wakefulness.
The correlation analyses showed that emotion changes are associated with changes in the functional connections between the thalamus and parts of brain regions following ASD (Figure 4). The decrease in functional connectivity between the left thalamus and left orbital inferior frontal gyrus was correlated with change in emotion: r (anxiety) = −0.446, p = 0.017; r (confusion) = −0.516, p = 0.005; r (fatigue) = −0.420, p = 0.026; r (total score) = −0.500, p = 0.007. The decrease in functional connectivity between the left thalamus and left opercular inferior frontal gyrus was also correlated with emotion changes: r (anxiety) = −0.426, p = 0.024; r (total score) = −0.396, p = 0.037. However, no extra significant correlation was found between the alterations of functional connectivity and emotion changes during ASD (Table 3).
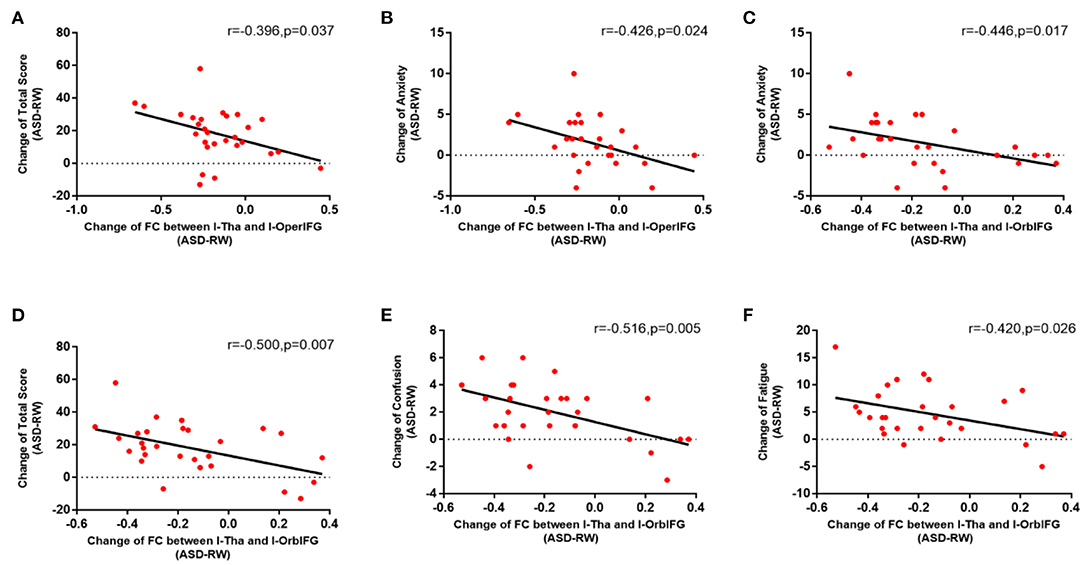
Figure 4. Relation of functional connectivity between left thalamus and other brain regions to emotion changes following acute sleep deprivation (ASD > RW, n = 28). The decrease in functional connectivity between l-Tha and l-OperIFG (A), between l-Tha and l-OrbIFG (D) was significantly negatively correlated with the Change of total score of the POMS. The decrease in functional connectivity between l-Tha and l-OperIFG (B), between l-Tha and l-OrbIFG (C) was significantly negatively correlated with the change of anxiety. The decrease in functional connectivity between l-Tha and l-OrbIFG (E,F) was significantly negatively correlated with the change of confusion and fatigue. FC, functional connectivity; ASD, acute sleep deprivation; RW, rested wakefulness; l-Tha, left thalamus; l-OperIFG, left opercular inferior frontal gyrus; l-OrbIFG, left orbital inferior frontal gyrus.
Discussion
The role played by the thalamus in emotion changes following ASD remains unclear, although ASD can lead to emotion changes (6, 12), and the thalamus has been shown to be involved in emotion changes under CSD conditions. As an essential node in the sleep pathway, the role of the thalamus in sleep is beyond doubt (6). It is of great interest to explore the role of the thalamus in emotion changes following ASD. Our study provides preliminary evidence that the thalamus as a node is associated with emotion changes. In this study, the results of POMS confirmed that negative emotions significantly increased and positive emotions significantly decreased before and after ASD. Next, through R-fMRI analysis, significant changes were found in the functional connections between the thalamus and the brain regions that are mainly responsible for emotional processing. Finally, through correlation analysis, significant changes in the functional connections between the thalamus and related brain regions were found to be closely related to emotion changes.
Emotion Changes Following ASD
Compared to the RW state, there were significant emotion changes following ASD. The total score of the POMS scale and its five parts of anxiety, confusion, anger, vitality and fatigue all changed significantly. This is consistent with other relevant studies (10, 11, 29–31). Furthermore, these results largely support those of Short and Louca (32) and Babson et al. (31), which show that ASD is sensitive to different emotional deficits under different emotional states, and has a more significant impact on confusion, energy and fatigue than on depression, anxiety and anger (31, 32). Moderate sleep deprivation aggravates confusion, vigor and fatigue, and emotional states such as anger and anxiety often worsen with more severe sleep deprivation, especially after complete sleep deprivation (31, 32). We agree with Mischel's reasoning about this changing trend and argue that people with different emotional states are sensitive to sleep deprivation differently. It's important to note that our study found no significant change in depressive mood following ASD. This is consistent with relevant studies to a certain extent, as ASD is an effective treatment for depression, with well-documented efficacy around 50% (33, 34). This may explain the absence of significant changes in depression in healthy subjects following ASD. Besides, our subjects within each group knew each other and did not undergo high-intensity experimental content, which may have reduced the degree of specific negative emotion changes such as depression during the experiment.
Changes in Brain Functional Connectivity
The functional connectivity between the thalamus and other brain regions was significantly changed following ASD, and these regions are mainly located in the frontal and parietal cortex which are involved in almost all functions related to emotion (5–7, 35). We observed significantly changed functional connections between the thalamus and the left inferior frontal gyrus, left middle temporal gyrus, right middle temporal gyrus, right inferior temporal gyrus and left medial superior frontal gyrus, all of which are involved in emotional function to some extent. Actually, almost the entire brain network is involved in emotional functions (36), and sleep deprivation can reliably trigger changes in negative emotional processes including irritability, anxiety, aggression, and mood swings (6). Meanwhile, although the thalamus is an essential node of wakefulness switching in the sleep-wake pathway, we noticed no significant change in the functional connections between the thalamus and the amygdala, an emotion-processing region of the limbic system which is susceptible to ASD (6, 37). These changes in functional connections between the thalamus and other brain regions associated with emotion changes strongly suggest that the thalamus may be involved in emotion changes to some extent. This may support the idea that in emotional experience and the perception of a set of discrete categories of emotion, a group of interactive brain regions is usually involved in emotional and non-emotional basic psychological operations (37).
Relation of Altered Functional Connectivity to Emotion Changes
Our study of the relation of altered functional connectivity to emotion changes further confirms that the thalamus is involved in emotion changes following ASD. It was found that the functional connections between the left thalamus and left orbital inferior frontal gyrus were negatively correlated with the total POMS score and confusion, anxiety and fatigue. The orbital frontal gyrus consists of medial ventral parts of the superior, middle and inferior frontal gyrus. It processes emotional responses to internal cues and modulates emotions and rewards in the decision-making process. In particular, the left orbital inferior frontal gyrus is closely related to emotion changes, and the impairment of the orbital frontal regulation of limbic emotional processing is considered the cause of the bipolar disorder (38, 39).
Our study also observed that the functional connections between the left thalamus and left opercular inferior frontal gyrus were negatively correlated with the total POMS score and anxiety (Table 3). The left opercular inferior frontal gyrus is an essential area for language processing (40), and the relatively few relevant studies have pointed out that there is a specific correlation between the left opercular inferior frontal gyrus and social anxiety disorder (15, 41–43).
The above results show that the functional connections between the left thalamus and left inferior frontal gyrus are correlated with emotion changes following ASD, especially anxiety (Figure 4). The inferior frontal gyrus is comprised of the orbital inferior frontal gyrus, triangular inferior frontal gyrus and opercular inferior frontal gyrus (44). The study found significant changes in frontal lobe function during sleep deprivation (45), and certain studies have suggested that functional changes in the left inferior frontal gyrus during sleep deprivation are associated with anxiety, depression and mood swings (7, 43, 46, 47). Given the various functions of the left inferior frontal gyrus, it is difficult to distinguish whether it is the responsible center or relay station of emotion changes according to the existing research, but it is clear that the left inferior frontal gyrus is an important hub of the emotional pathways.
The left thalamus engages in a variety of emotion changes presented in many diseases such as anxiety disorder, bipolar disorder, posttraumatic stress disorder, major depressive disorder, and hyperalexithymia (8, 48). Patients with high stress are specifically associated with lesions in the left thalamus (49) since both the left thalamus and left inferior frontal gyrus are involved in a variety of emotion changes, and the inferior frontal gyrus interacts closely with the thalamic nuclei (44). Furthermore, studies have suggested that CSD is characterized by structural and functional changes between the thalamus and left inferior frontal gyrus (50–52). Meanwhile, the functional connections between the thalamus and frontal lobe seem sensitive to mild sleep-wake changes (53) which are similar to those observed following ASD in our study. Most importantly, our experiment shows that the functional connections between the left thalamus and left inferior frontal gyrus are strongly associated with emotion changes such as anxiety. Taken together, there is sufficient preliminary evidence to demonstrate the involvement of the thalamus in emotion changes induced by ASD.
Furthermore, although the functional connections between the bilateral thalamus and medial superior frontal gyrus decreased, there was no clear correlation between this decrease and emotion changes. The medial superior frontal gyrus, one of the most important brain regions in the emotional network, showed altered functional connections with the bilateral thalamus during ASD, but this did not seem to be the leading cause of the emotion changes. Meanwhile, our results showed that the functional connections between the thalamus and other brain regions mainly responsible for emotion were not significantly changed following ASD, which further suggests that the brain regions affected by ASD and those affected by CSD may not be entirely consistent.
We further speculate that the emotion changes under ASD related to the left inferior frontal gyrus and thalamus are not entirely consistent with the traditional emotional network. The thalamus has been observed to participate in a variety of functional networks that have different response patterns (54). This suggests that functional tissues allow spatially overlapping networks of resting states of the brain, facilitating the description of various interpersonal relationships between overlapping regions and different functional systems in other regions of the brain (54). This partly explains the decreased functional connections between the left thalamus and left inferior frontal gyrus associated with emotion changes after sleep deprivation. It is also possible that there is a network mechanism for new emotion changes in the context of ASD.
In summary, our study finds that functional changes in the thalamus following 36 h of total ASD are associated with emotion changes. The changes in functional connection between the left thalamus and left inferior frontal gyrus were negatively correlated with emotion changes, and the thalamic-related emotion regulation circuit was affected. This means that the left thalamus plays a vital role in emotion changes following ASD.
Limitations of the Study
First, the subjects of our study were all young male men limited to the experimental conditions, and literature has pointed out that gender may have different effects on emotional state (e.g., depression) during sleep deprivation (55). Gender and age may affect the accuracy of the experimental conclusion, we should take the gender differences into consideration in future fMRI studies, especially the treatment of brain-related diseases. We will build on the present study and refine our study in future studies to cover left-handedness and sleep deprivation in women. Second, the thalamus is a big ROI in AAL, and calculations based on this seed point may be general. However, for the selection of ROI in AAL, we mainly considered that this template was widely used, especially in the previous thalamic research articles. Therefore, we chose this conservative seed point in this exploratory study. Furthermore, we will study the function of the thalamus by using a more detailed method in further studies to further improve this conservative but robust conclusion.
Data Availability Statement
The raw data supporting the conclusions of this article will be made available by the authors, without undue reservation.
Ethics Statement
The studies involving human participants were reviewed and approved by Research Ethics Committee of Beihang University (Beijing, China; BM20180040). The patients/participants provided their written informed consent to participate in this study.
Author Contributions
B-zL contributed to performing the experiments, processing the data and drafting the paper. YCa contributed to processing the data and drafting the paper. YZ and YCh contributed to performing the experiments and acquiring the data. Y-cS, Y-hG, and XZ are the guarantors of the study. All authors made substantial contributions to this work and approved it for publication.
Funding
This research was funded by the National Science Foundation of China, Nos. AWS16J023 and AWS14J011, the NDSTISZP (1716312ZT00211101).
Conflict of Interest
The authors declare that the research was conducted in the absence of any commercial or financial relationships that could be construed as a potential conflict of interest.
References
1. Dorrian J, Centofanti S, Smith A, McDermott KD. Self-regulation and social behavior during sleep deprivation. Prog Brain Res. (2019) 246:73–110. doi: 10.1016/bs.pbr.2019.03.010
2. Itani O, Jike M, Watanabe N, Kaneita Y. Short sleep duration and health outcomes: a systematic review, meta-analysis, and meta-regression. Sleep Med. (2017) 32:246–56. doi: 10.1016/j.sleep.2016.08.006
3. Hisler GC, Muranovic D, Krizan Z. Changes in sleep difficulties among the U.S. population from 2013 to 2017: results from the National Health Interview Survey. Sleep Health. (2019) 5:615–20. doi: 10.1016/j.sleh.2019.08.008
4. Medic G, Wille M, Hemels M. Short- and long-term health consequences of sleep disruption. Nat Sci Sleep. (2017) 9:151–61. doi: 10.2147/NSS.S134864
5. Tempesta D, Socci V, De Gennaro L, Ferrara M. Sleep and emotional processing. Sleep Med Rev. (2018) 40:183–95. doi: 10.1016/j.smrv.2017.12.005
6. Krause AJ, Simon EB, Mander BA, Greer SM, Saletin JM, Goldstein-Piekarski AN, et al. The sleep-deprived human brain. Nat Rev Neurosci. (2017) 18:404–18. doi: 10.1038/nrn.2017.55
7. Goldstein AN, Greer SM, Saletin JM, Harvey AG, Williams LM, Walker MP. Sex, sleep deprivation, and the anxious brain. J Cogn Neurosci. (2018) 30:565–78. doi: 10.1162/jocn_a_01225
8. Chalah MA, Kauv P, Palm U, Lefaucheur J-P, Hodel J, Créange A, et al. Deciphering the neural underpinnings of alexithymia in multiple sclerosis. Neurosci Lett. (2020) 725:134894. doi: 10.1016/j.neulet.2020.134894
9. Jenkins LM, Chiang JJ, Vause K, Hoffer L, Alpert K, Parrish TB, et al. Outward subcortical curvature associated with sub-clinical depression symptoms in adolescents. Neuroimage Clin. (2020) 25:102187. doi: 10.1016/j.nicl.2020.102187
10. Mazzetti C, Staudigl T, Marshall TR, Zumer JM, Fallon SJ, Jensen O. Hemispheric asymmetry of globus pallidus relates to alpha modulation in reward-related attentional tasks. J Neurosci. (2019) 39:9221–36. doi: 10.1523/JNEUROSCI.0610-19.2019
11. Wang X, Zhao X, Xue G, Chen A. Alertness function of thalamus in conflict adaptation. NeuroImage. (2016) 132:274–82. doi: 10.1016/j.neuroimage.2016.02.048
12. Goldstein AN, Walker MP. The role of sleep in emotional brain function. Annu Rev Clin Psychol. (2014) 10:679–708. doi: 10.1146/annurev-clinpsy-032813-153716
13. Aydin S, Demirtaş S, Tunga MA, Ateş K. Comparison of hemispheric asymmetry measurements for emotional recordings from controls. Neural Comput Appl. (2018) 30:1341–51. doi: 10.1007/s00521-017-3006-8
14. Li M, Wang R, Zhao M, Zhai J, Liu B, Yu D, et al. (2018). Abnormalities of thalamus volume and resting state functional connectivity in primary insomnia patients. Brain Imaging Behav. (2019) 13:1193–201. doi: 10.1007/s11682-018-9932-y
15. Sakaki K, Nozawa T, Ikeda S, Kawashima R. Neural correlates of cognitive bias modification for interpretation. Soc Cogn Affect Neurosci. (2020) 15:247–60. doi: 10.1093/scan/nsaa026
16. Qiao J, Li A, Cao C, Wang Z, Sun J, Xu G. Aberrant functional network connectivity as a biomarker of generalized anxiety disorder. Front Hum Neurosci. (2017) 11:626. doi: 10.2174/97816810853261170401
17. Javaheipour N, Shahdipour N, Noori K, Zarei M, Camilleri J, Laird A, et al. Functional brain alterations in acute sleep deprivation: an activation likelihood estimation meta-analysis. Sleep Med Rev. (2019) 46:64–73. doi: 10.1016/j.smrv.2019.03.008
18. Chee MWL, Tan JC, Zheng H, Parimal S, Weissman DH, Zagorodnov V, et al. Lapsing during sleep deprivation is associated with distributed changes in brain activation. J Neurosci. (2008) 28:5519–28. doi: 10.1523/JNEUROSCI.0733-08.2008
19. Chee MWL, Tan JC, Parimal S, Zagorodnov V. Sleep deprivation and its effects on object-selective attention. NeuroImage. (2010) 49:1903–10. doi: 10.1016/j.neuroimage.2009.08.067
20. Gent TC, Bassetti CL, Adamantidis AR. Sleep-wake control and the thalamus. Curr Opin Neurobiol. (2018) 52:188–97. doi: 10.1016/j.conb.2018.08.002
21. Postuma RB, Dagher A. Basal ganglia functional connectivity based on a meta-analysis of 126 positron emission tomography and functional magnetic resonance imaging publications. Cereb Cortex. (2005) 16:1508–21. doi: 10.1093/cercor/bhj088
22. Dai XJ, Jiang J, Zhang Z, Nie X, Liu BX, Pei L, et al. Plasticity and susceptibility of brain morphometry alterations to insufficient sleep. Front Psychiatry. (2018) 9:266. doi: 10.3389/fpsyt.2018.00266
23. McNair D, Lorr M, Droppleman LF. EdITS manual for the Profile of Mood States. San Diego, CA: Educational and Industrial Testing Service. (1992).
24. Tsai P-S, Wang S-Y, Wang M-Y, Su C-T, Yang T-T, Huang C-J, et al. Psychometric evaluation of the Chinese version of the Pittsburgh sleep quality index (CPSQI) in primary insomnia and control subjects. Qual Life Res. (2005) 14:1943–52. doi: 10.1007/s11136-005-4346-x
25. Buysse DJ, Reynolds CF, Monk TH, Berman SR, Kupfer DJ. The Pittsburgh sleep quality index: a new instrument for psychiatric practice and research. Psychiatry Res. (1989) 28:193–213. doi: 10.1016/0165-1781(89)90047-4
26. Behzadi Y, Restom K, Liau J, Liu TT. A component based noise correction method (CompCor) for BOLD and perfusion based fMRI. NeuroImage. (2007) 37:90–101. doi: 10.1016/j.neuroimage.2007.04.042
27. Whitfield-Gabrieli S, Nieto-Castanon A. Conn: a functional connectivity toolbox for correlated and anticorrelated brain networks. Brain Connect. (2012) 2:125–41. doi: 10.1089/brain.2012.0073
28. Tzourio-Mazoyer N, Landeau B, Papathanassiou D, Crivello F, Etard O, Delcroix N, et al. Automated anatomical labeling of activations in SPM using a macroscopic anatomical parcellation of the MNI MRI single-subject brain. NeuroImage. (2002) 15:273–89. doi: 10.1006/nimg.2001.0978
29. Schwarz J, Axelsson J, Gerhardsson A, Tamm S, Fischer H, Kecklund G, et al. Mood impairment is stronger in young than in older adults after sleep deprivation. J Sleep Res. (2018). 28:e12801. doi: 10.1111/jsr.12801
30. Kaida K, Niki K. Total sleep deprivation decreases flow experience and mood status. Neuropsychiatr Dis Treat. (2013) 10:19–25. doi: 10.2147/NDT.S53633
31. Babson KA, Trainor CD, Feldner MT, Blumenthal H. A test of the effects of acute sleep deprivation on general and specific self-reported anxiety and depressive symptoms: an experimental extension. J Behav Ther Exp Psychiatry. (2010) 41:297–303. doi: 10.1016/j.jbtep.2010.02.008
32. Short MA, Louca M. Sleep deprivation leads to mood deficits in healthy adolescents. Sleep Med. (2015) 16:987–93. doi: 10.1016/j.sleep.2015.03.007
33. Boland EM, Rao H, Dinges DF, Smith RV, Goel N, Detre JA, et al. Meta-analysis of the antidepressant effects of acute sleep deprivation. J Clin Psychiatry. (2017) 78:e1020–34. doi: 10.4088/JCP.16r11332
34. Gillin JC. The sleep therapies of depression. Prog Neuropsychopharmacol Biol Psychiatry. (1983) 7:351–64. doi: 10.1016/0278-5846(83)90123-9
35. Saletin JM, Jackvony S, Rodriguez KA, Dickstein DP. A coordinate-based meta-analysis comparing brain activation between attention deficit hyperactivity disorder and total sleep deprivation. Sleep. (2019) 42:zsy251. doi: 10.1093/sleep/zsy251
36. Lindquist KA, Wager TD, Kober H, Bliss-Moreau E, Barrett LF. The brain basis of emotion: a meta-analytic review. Behav Brain Sci. (2012) 35:121–43. doi: 10.1017/S0140525X11000446
37. Yoo SS, Gujar N, Hu P, Jolesz FA, Walker MP. The human emotional brain without sleep–a prefrontal amygdala disconnect. Curr Biol. (2007) 17:R877–8. doi: 10.1016/j.cub.2007.08.007
38. Strakowski SM, Adler CM, Almeida J, Altshuler LL, Blumberg HP, Chang KD, et al. The functional neuroanatomy of bipolar disorder: a consensus model. Bipolar Disord. (2012) 14:313–25. doi: 10.1111/j.1399-5618.2012.01022.x
39. Kandilarova S, Stoyanov D, Sirakov N, Maes M, Specht K. Reduced grey matter volume in frontal and temporal areas in depression: contributions from voxel-based morphometry study. Acta Neuropsychiatr. (2019) 31:252–7. doi: 10.1017/neu.2019.20
40. Bajaj S, Killgore WDS. Vulnerability to mood degradation during sleep deprivation is influenced by white-matter compactness of the triple-network model. NeuroImage. (2019) 202:116123. doi: 10.1016/j.neuroimage.2019.116123
41. Lee M-H, O'Hara NB, Behen ME, Jeong J-W. Altered efficiency of white matter connections for language function in children with language disorder. Brain Lang. (2020) 203:104743. doi: 10.1016/j.bandl.2020.104743
42. Lissek S, Klass A, Tegenthoff M. Left inferior frontal gyrus participates in mediating the renewal effect irrespective of context salience. Front Behav Neurosci. (2020) 14:43. doi: 10.3389/fnbeh.2020.00043
43. Du J, Rolls ET, Cheng W, Li Y, Gong W, Qiu J, et al. Functional connectivity of the orbitofrontal cortex, anterior cingulate cortex, and inferior frontal gyrus in humans. Cortex. (2020) 123:185–99. doi: 10.1016/j.cortex.2019.10.012
44. Binder MD, Hirokawa N, Windhorst U. Inferior Frontal Gyrus (eds) Encyclopedia of Neuroscience. Berlin, Heidelberg: Springer (2009).
45. Gao L, Bai L, Zhang Y, Dai XJ, Netra R, Min Y, et al. Frequency-dependent changes of local resting oscillations in sleep-deprived brain. PLoS ONE. (2015) 10:e0120323. doi: 10.1371/journal.pone.0120323
46. Jabbi M, Keysers C. Inferior frontal gyrus activity triggers anterior insula response to emotional facial expressions. Emotion. (2008) 8:775–80. doi: 10.1037/a0014194
47. Leerssen J, Blanken TF, Pozzi E, Jahanshad N, Aftanas L, Andreassen OA, et al. Brain structural correlates of insomnia severity in 1053 individuals with major depressive disorder: results from the ENIGMA MDD Working Group. Transl Psychiatry. (2020) 10:425. doi: 10.1038/s41398-020-01109-5
48. Janiri D, Moser DA, Doucet GE, Luber MJ, Rasgon A, Lee WH, et al. Shared neural phenotypes for mood and anxiety disorders. JAMA Psychiatry. (2019) 77:172–9. doi: 10.1001/jamapsychiatry.2019.3351
49. Sutoko S, Atsumori H, Obata A, Funane T, Kandori A, Shimonaga K, et al. Lesions in the right Rolandic operculum are associated with self-rating affective and apathetic depressive symptoms for post-stroke patients. Sci Rep. (2020) 10:20264. doi: 10.1038/s41598-020-77136-5
50. Drummond SP, Walker M, Almklov E, Campos M, Anderson DE, Straus LD. Neural correlates of working memory performance in primary insomnia. Sleep. (2013) 36:1307–16. doi: 10.5665/sleep.2952
51. Lu FM, Liu CH, Lu SL, Tang LR, Tie CL, Zhang J, et al. Disrupted topology of frontostriatal circuits is linked to the severity of insomnia. Front Neurosci. (2017) 11:214. doi: 10.3389/fnins.2017.00214
52. Kang J, Joo S, Son Y, Kim HK, Ko K, Lee J, et al. Low white-matter integrity between the left thalamus and inferior frontal gyrus in patients with insomnia disorder. J Psychiatry Neurosci. (2018) 43:366–74. doi: 10.1503/jpn.170195
53. Vallat R, Meunier D, Nicolas A, Ruby P. Hard to wake up? The cerebral correlates of sleep inertia assessed using combined behavioral, EEG and fMRI measures. NeuroImage. (2019) 184:266–78. doi: 10.1016/j.neuroimage.2018.09.033
54. Shen H, Xu H, Wang L, Lei Y, Yang L, Zhang P, et al. Making group inferences using sparse representation of resting-state functional mRI data with application to sleep deprivation. Hum Brain Mapp. (2017) 38:4671–89. doi: 10.1002/hbm.23693
Keywords: mood, functional connectivity, resting-state functional magnetic resonance imaging, thalamus, inferior frontal gyrus, acute sleep deprivation
Citation: Li B-z, Cao Y, Zhang Y, Chen Y, Gao Y-h, Peng J-x, Shao Y-c and Zhang X (2021) Relation of Decreased Functional Connectivity Between Left Thalamus and Left Inferior Frontal Gyrus to Emotion Changes Following Acute Sleep Deprivation. Front. Neurol. 12:642411. doi: 10.3389/fneur.2021.642411
Received: 16 December 2020; Accepted: 01 February 2021;
Published: 26 February 2021.
Edited by:
Xi-jian Dai, The Chinese University of Hong Kong, ChinaReviewed by:
Axel Steiger, Ludwig Maximilian University of Munich, GermanyRoumen Kirov, Bulgarian Academy of Sciences (BAS), Bulgaria
Copyright © 2021 Li, Cao, Zhang, Chen, Gao, Peng, Shao and Zhang. This is an open-access article distributed under the terms of the Creative Commons Attribution License (CC BY). The use, distribution or reproduction in other forums is permitted, provided the original author(s) and the copyright owner(s) are credited and that the original publication in this journal is cited, in accordance with accepted academic practice. No use, distribution or reproduction is permitted which does not comply with these terms.
*Correspondence: Xi Zhang, smrc301@163.com; Yong-cong Shao, budeshao@aliyun.com