- 1Department of Neurosurgery, Renmin Hospital, Wuhan University, Wuhan, China
- 2Department of Neurology, Ruijin Hospital, Shanghai Jiao Tong University School of Medicine, Shanghai, China
- 3Department of Functional Neurosurgery, Ruijin Hospital, Shanghai Jiao Tong University School of Medicine, Shanghai, China
- 4Fixel Center for Neurological Diseases, University of Florida, Gainesville, FL, United States
Purpose: Deep brain stimulation of the subthalamic nucleus (STN-DBS) is an effective treatment method for advanced Parkinson's disease (PD) and isolated dystonia and provides marked improvement of major motor symptoms. In addition, non-motor effects have been reported including weight gain (WG) in patients with PD after STN-DBS. However, it is still unclear whether patients with isolated dystonia also experience WG.
Methods: Data from 47 patients with isolated dystonia who underwent bilateral STN-DBS surgery between October 2012 and June 2019 were retrospectively collected. The severity of dystonia was assessed via the Burke–Fahn–Marsden Dystonia Rating Scale (BFMDRS). Changes in the body mass index (BMI) and BFMDRS score were analyzed using paired Student's t-tests. Regression analysis was performed to identify factors that affected the BMI after surgery.
Results: Postoperative WG was observed in 78.7% of patients. The percentage of overweight and obese patients increased from 25.5% (before STN-DBS) to 48.9% (at the last follow-up). The mean BMI and mean percentage change in BMI increased by 1.32 ± 1.83 kg/m2 (P < 0.001) and 6.28 ± 8.34%, respectively. BMI increased more in female than in male patients. At the last follow-up, BFMDRS movement and disability scores improved by 69.76 ± 33.23% and 65.66 ± 31.41%, respectively (both P < 0.001). The final regression model analysis revealed that sex and preoperative BMI alone were independently associated with BMI change (P < 0.05).
Conclusions: STN-DBS is associated with postoperative WG with patients with isolated dystonia. WG is more prominent in female patients and is associated with preoperative weight but not with the efficacy of STN-DBS on motor symptoms.
Introduction
In recent decades, deep brain stimulation (DBS) has been applied to treat many disorders, such as advanced stages of Parkinson's disease (PD), intractable essential tremor, complicated segmental and generalized dystonia, and neuropsychiatric diseases (1, 2). DBS may improve patients' quality of life and result in desired therapeutic effects such as reduced tremor, dyskinesia, and stiffness in patients with PD (3, 4). However, DBS can also cause adverse events (5) and side effects (e.g., cognitive and emotional changes) (6–8) in some patients.
Interestingly, many researchers have reported that weight gain (WG) is common in patients with PD after DBS of the subthalamic nucleus (STN-DBS) (9). This is concerning because WG may lead to additional health complications such as obesity and diabetes (6). Some potential mechanisms underlying WG in patients with PD after STN-DBS treatment have been suggested, including the improvement of resting tremor and dyskinesias, reduction in energy expenditure (EE), changes in eating behavior and food intake, perturbations of homeostatic control, changes in hormone and neurotransmitter systems, and improvement in motor function; however, the exact mechanism underlying WG remains unknown (9).
Dystonia is characterized by sustained and involuntary muscular contractions, which result from dysfunction of the cortico-striato-thalamo-cortical circuitry. Dystonia can be classified according to the involved body distribution: focal, segmental, multifocal, generalized, and hemidystonia, or according to the etiology: inherited (proven genetic origin), acquired (known specific cause), and idiopathic (unknown cause) (10). Dystonia is the third most common movement disorder after PD and essential tremor (11). It is highly disabling and can seriously affect patients' quality of life. Recent studies have demonstrated that DBS of either the STN or globus pallidus internus (GPi) is an efficient treatment option for dystonia (12–16). A dual-target, crossover sham-controlled study has directly compared the clinical effects of GPi and STN stimulations in patients with dystonia, which indicated that the STN was a more effective target for dystonia than the GPi (17). STN-DBS is believed to improve the repetitive motions, abnormal posture, and quality of life of refractory patients with isolated dystonia (12, 13, 15, 18–21). The motor outcomes of STN-DBS in the treatment of dystonia have been extensively studied (12–16, 18–21). However, little is known about the effects of STN-DBS on non-motor (e.g., weight changes) symptoms in dystonia.
A thorough review of the available literature revealed that only one study has investigated WG in patients with isolated dystonia after STN-DBS, which only included nine patients (22). Given that there are significant differences in the pathophysiology and clinical manifestations of dystonia and PD (23), we investigated WG in patients with isolated dystonia who underwent STN-DBS at our center. Our center was one of the early explorers to the use of STN as a DBS target to treat isolated dystonia, which allowed us to study weight changes of a larger sample of patients with isolated dystonia after STN-DBS (24). Information on adverse events can help to better define the populations that will most benefit from STN-DBS. Thus, careful attention must be devoted to the investigation of WG after STN-DBS for the treatment of dystonia. To the best of our knowledge, this is the first study to explore the association between weight change and symptomatic improvement after STN-DBS in patients with dystonia.
Materials and Methods
Participants
Patients with isolated dystonia [with normal brain magnetic resonance imaging (MRI) scans] (10) who were treated with bilateral STN-DBS between August 2012 and May 2019 were included in the present study. Patients were diagnosed with isolated dystonia by movement disorder specialists and admitted to the Department of Functional Neurosurgery, Ruijin Hospital, Shanghai Jiao Tong University School of Medicine, Shanghai. All patients, in the absence of secondary causes, presented with marked symptoms, despite optimal pharmacologic treatment. Except for dystonia, all patients presented without other neurological deficiencies. The exclusion criteria were as follows: diabetes and thyroid disease, nutritional intervention received in addition to routine care, unexplained weight change, severe metabolic disease (chronic gastroenteritis, liver dysfunction, malignant tumors, other consumptive diseases, and others), severe dysphagia, history of operations (esophagectomy, bowel resection, gastrectomy, and others), active mental illness (depression, schizophrenia, and others), other brain surgery, and postoperative complications (cerebral hemorrhage, infection, and others). Patients' age ranged from 18 to 75 years. To gain insight into the etiology (idiopathic or inherited) of patients with isolated dystonia, we performed whole-exome sequencing. Thirteen patients underwent genetic testing, whereas 34 declined genetic testing. This study was approved by the ethics committee of Ruijin Hospital. Written informed consent from all patients was obtained for participation in this study.
Data Collection
We used a structured questionnaire (32 questions) about their family and personal history, focusing on metabolic syndrome, diabetes, access to nutrition counseling, and body mass index (BMI) data. Patients with recent (<1 month) Burke–Fahn–Marsden Dystonia Rating Scale (BFMDRS) data received questionnaires via email or express delivery. The remaining patients received clinical routine outpatient follow-ups with this particular additional questionnaire. We retrospectively collected the following pre- and post-DBS data from Ruijin Hospital database of surgical patients: BFMDRS scores, weight, height, age at surgery, disease duration, and sex. Videos of BFMDRS scores were recorded by an evaluator who did not know the patient's neurostimulation status at baseline and the last follow-up postoperatively. Data from the evaluations were obtained by other evaluators (HXL and WBH) who were blinded tothe patients' names and stimulation status, using standardized scoring criteria. According to the Chinese standard “WS/T 428-2013: Criteria of weight for adults,” we classified patients into the four following groups: low weight (BMI < 18.5 kg/m2), normal weight (18.5 ≤ BMI < 24.0 kg/m2), overweight (24.0 ≤ BMI < 28.0 kg/m2), and obese (BMI ≥ 28.0 kg/m2).
Surgical Procedure
Patients underwent standard bilateral stereotactic STN-DBS implantation procedures. MRI (1.5 T SIGNA or 3 T HDx; General Electric Company, Boston, MA, USA) and macrostimulation were used for STN targeting. Quadripolar DBS electrodes (serial number, 3387; Medtronic, Minneapolis, MN, USA or serial number, 3389; Medtronic, Minneapolis, MN, USA or L301; PINS, Beijing, China or L302; PINS, Beijing, China) were implanted under local anesthesia for precise monitoring of motor function and adverse effects using macrostimulation. An implantable pulse generator (Kinetra 7428; Medtronic or 37612; Medtronic or G101A; PINS or G102R; PINS) was implanted subclavicularly under general anesthesia. Electrode placement was validated using postoperative MRI or CT.
Statistical Analyses
IBM SPSS Statistics for Windows version 22.0 (IBM Corp., Armonk, NY, USA) was used for data analysis. We analyzed changes in BMI, BFMDRS movement scale (BFMDRS-MS), and BFMDRS disability scale (BFMDRS-DS) using a paired Student's t-test or independent samples t-test. A one-way analysis of variance (ANOVA) was used to analyze the influence of body fat distribution on the percentage change in BMI. Multivariate linear regression was performed to evaluate the association between clinical/demographic characteristics and percentage change in BMI following surgery. The latter was regarded as dependent variables. Independent variables included sex (binary variable, where male = 0, and female = 1), age at surgery (years), preoperative BMI, time interval after surgery (months), preoperative BFMDRS-MS, and percentage change in BFMDRS-MS following surgery. All continuous data are presented as the means ± standard deviations and ranges. We report two-tailed P-values along with 95% confidence intervals. We selected a significance threshold of P = 0.05 and adjusted the significance levels for multivariate linear regression using the Benjamini–Hochberg procedure to account for multiple testing.
Results
Patient Characteristics
In total, 54 patients with isolated dystonia underwent bilateral STN-DBS surgery within the study period. Seven patients were excluded due to a lack of follow-up, and 47 (87%) were included in the final analysis. The results of the 13 patients who underwent genetic testing were as follows: 10 patients with idiopathic isolated dystonia, 2 patients with TOR1A (DYT1)-positive isolated dystonia, and 1 patient with THAP1 (DYT6)-positive isolated dystonia. Thirty-four patients who refused genetic testing were diagnosed with unknown (inherited or idiopathic) isolated dystonia. The body distribution of 47 patients was as follows: generalized (N = 14), multifocal (N = 17), segmental (N = 5), and focal (N = 11). These 47 patients (23 women and 24 men) were 50.62 ± 15.02 years old at the time of the operation (range, 21 to 73 years), and the average follow-up time after surgery was 39.89 ± 26.25 months (range, 4 to 83 months). The average time from diagnosis to surgery was 11.98 ± 7.68 years. The preoperative medications were as follows: 40 patients were treated with botulinum toxin, 8 with clonazepam, 17 with trihexyphenidyl, 12 with tiapride, 8 with haloperidol, 18 with baclofen, and 2 with levodopa and benserazide hydrochloride. Patient demographics and clinical data are summarized in Table 1.
Changes in Body Weight
Changes in BMI are summarized in Table 2 and Figure 1. Compared with the baseline, 78.7% of the 47 patients exhibited postoperative WG at the final follow-up. The percentage of overweight or obese patients increased from 25.5 to 48.9%. The mean BMI of all patients increased by 1.32 ± 1.83 kg/m2 (22.64 ± 3.09 kg/m2 before surgery vs. 23.95 ± 2.94 kg/m2 after surgery; P < 0.001, n = 47), and the mean increase in BMI increase was 6.28 ± 8.34%. The mean BMI of female patients increased significantly more than that of male patients (2.02 ± 2 and 0.65 ± 1.38 kg/m2 for female and male, respectively; P = 0.009).
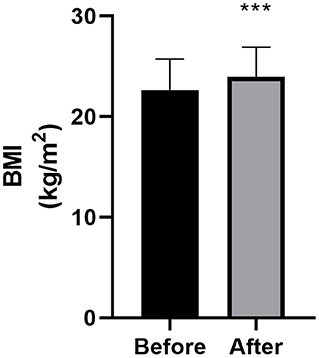
Figure 1. Body mass index (BMI) at baseline and final follow-up (n = 47). Bars represent the mean + standard deviation score. The asterisk indicates that the comparison with the preoperative score was statistically significant (***P < 0.001).
Similarly, the mean percentage change in BMI increased significantly more in female than in male patients (9.54 ± 9.32% and 3.15 ± 5.93%, respectively; P = 0.007). There was no significant difference in clinical characteristics between male and female patients (see Table 3). Patients were divided into three groups according to the age: <40 (N = 13), 40–60 (N = 19), and over 60 years (N = 15). One-way ANOVA showed no difference in percentage change in BMI among the three groups [F2,45 = 0.909, P = 0.410]. Considering the different follow-up times, patients were divided into four groups according to the follow-up time: <1 (N = 13), 1–3 (N = 8), 3–5 (N = 11), and more than 5 years (N = 16). One-way ANOVA showed no difference in the percentage change in BMI [F3,44 = 0.850, P = 0.474], BFMDRS-MS [F3,44 = 0.558, P = 0.646], and BFMDRS-DS [F3,44 = 0.296, P = 0.828] among the three groups.
Changes in the Burke–Fahn–Marsden Dystonia Rating Scale
The changes in the BFMDRS scores are shown in Table 4 and Figure 2. Compared with those at baseline, the BFMDRS-MS and BFMDRS-DS were lower at the final follow-up. The BFMDRS-MS was reduced by 69.76 ± 33.23% (a score of 29.5 ± 21.27 before surgery vs. 8.46 ± 9.87 after surgery; P < 0.001, n = 47); for BFMDRS-DS, this was 65.66 ± 31.41% (a score of 10.28 ± 6.28 before surgery vs. 2.96 ± 2.95 after surgery; P < 0.001, n = 47).
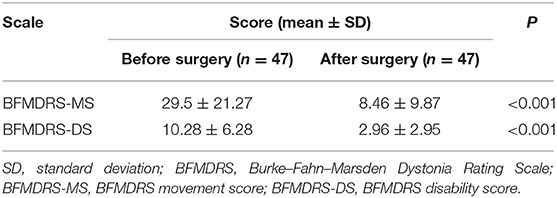
Table 4. Effects of STN stimulation on BFMDRS disability scores at baseline and last follow-up in patients with isolated dystonia.
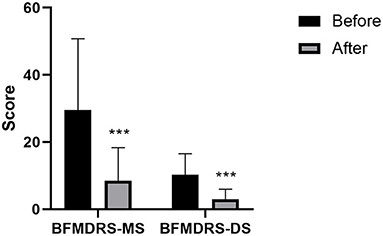
Figure 2. Burke–Fahn–Marsden Dystonia Rating Scale (BFMDRS) movement scores and disability scores at baseline and final follow-up (n = 47). Bars represent the mean + standard deviation score. Asterisks indicate where the comparisons between final follow-up score and preoperative score were statistically significant (***P < 0.001). BFMDRS-MS, BFMDRS movement score; BFMDRS-DS, BFMDRS disability score.
Factors Associated With Body Mass Index Gain
In the univariate linear regression analysis, age at surgery, follow-up period, baseline BFMDRS-MS, and percentage change in BFMDRS-MS were not associated with percentage change in BMI (P > 0.05; see Table 5); thus, we only added sex and preoperative BMI to the multivariate linear regression model. These were both independently associated with an increase in BMI (P < 0.05; see Table 6).
According to the distribution of dystonia, the 47 patients with dystonia were divided into four groups, as follows: focal (n = 11), segmental (n = 5), multifocal (n = 17), and generalized (with or without eyelid involvement) (n = 14). The one-way ANOVA indicated no difference in the percentage change of BMI before and after operation [F3, 43 = 0.465, P = 0.708] or BFMDRS-MS [F3,43 = 0.484, P = 0.695]. Patients were divided into two groups according to their dyskinesias, as follows: group 1, with limb involvement (n = 30); and group 2, without limb involvement (n = 17). The results of the independent samples t-test revealed no difference in the percentage change in BMI (P = 0.346) or BFMDRS-MS (P = 0.477) between the two groups.
Five patients in whom only the eyelids were affected were regarded as one group, and the other patients (with or without eyelid involvement) (n = 42) were regarded as another group. The results of an independent samples t-test revealed no difference in the percentage change in BMI (P = 0.862) or BFMDRS-MS (P = 0.499) between the two groups.
Discussion
In this study, we confirmed that patients with isolated dystonia typically exhibit WG after STN-DBS. Overall, 78.7% of the patients exhibited postoperative WG at the final follow-up. The proportion of patients that were overweight or obese increased from 25.5% before surgery to 48.9% in the final follow-up period.
There was a significant correlation between WG and sex, whereby the mean BMI of female patients increased significantly more than that of male patients after surgery. Considering that the average age of female patients at surgery was 51 years, this association may be associated with changes in menopausal hormones (25). This correlation has been previously identified for patients with PD who underwent STN-DBS (26). There is evidence showing that female patients with PD gain disproportionate amounts of fat mass after STN-DBS, while WG in men was driven by both fat-free mass and fat mass (27, 28). In this study, the percentage of overweight or obese female patients increased by 34.8% (8.7% before surgery vs. 43.5% after surgery), while that of men increased by 12% (42% before vs. 54% after surgery). In this context, it is also important to assess the changes in body composition after STN-DBS.
We found that the average percentage reduction in BFMDRS-MS was 69.76 ± 33.23%. However, the baseline BFMDRS-MS and percentage change in BFMDRS-MS were not associated with WG. There are three possible explanations for this result. First, BFMDRS-MS evaluates not only the limbs and trunk but also the eyes, the mouth, speech, swallowing, and the neck; thus, the affected body part may not be associated with resting EE (REE). There were only three patients with low weight before the operation, which may also indicate that the slight movement disorder before the operation had little effect on the patients' REE. Second, patients were able to perform larger movements and were more mobile after the operation than before the operation, which should theoretically increase EE. This may offset the reduced EE caused by improved motor symptoms. Of course, since we did not evaluate the free-living EE in a calorimetric chamber, the decrease in total daily EE due to the reduction in dyskinesias is uncertain. Third, we regrouped the patients three times (according to the distribution of the dystonia, dyskinesias, and involvement of the eyelids) and analyzed the subgroups separately. There were no differences in the percentage change of postoperative BMI and BFMDRS-MS between subgroups. Initially, we assumed that there would be no difference in postoperative physical activity in patients with involvement of only the eyelids before and after surgery and that there would be no difference in REE or free-living EE before and after surgery in this subgroup. However, the BMI of these patients differed before and after surgery. Therefore, the observed WG may result from changes in the central regulation of energy metabolism caused by STN-DBS. Concerning WG after STN-DBS in patients with PD, various studies have discovered no correlation between WG and changes in the Unified Parkinson Disease Rating Scale III score (28, 29). For future studies, it will be important to measure REE and free-living EE. Moreover, the reduction in EE may be due to the reduction in sustained or intermittent muscle contractions; however, changes in the central regulation of energy metabolism caused by direct STN-DBS may also be involved.
Indeed, previous studies have illustrated that a change in body weight is the direct result of STN-DBS on the regulation of the dietary and metabolic nucleus (in the lateral region of the thalamus and the marginal portion of the STN) (30–34). STN-DBS may have a regional effect on the hypothalamic–pituitary–adrenal homeostatic control of EE by diffusion of the electrical pulse to the hypothalamus (35, 36). Results of the direct effects of STN-DBS on hypothalamic catabolic/anabolic regulators including blood cortisol (36), ghrelin, neuropeptide Y, and leptin peptides (37–39) remain inconsistent and require further study. Therefore, the improvement of motor symptoms in dystonia is insufficient to explain WG after STN-DBS.
The balance between energy intake and EE determines weight change. However, there are no data on the difference in pre- and postoperative energy intake for STD-DBS in patients with isolated dystonia. Notably, a number of researchers have observed that WG after STN-DBS in patients with PD could result from increased sensitivity to food reward cues (40) and changes in eating behavior, including higher food intake, increased appetite, binge eating, or cravings (41–45). However, there have also been reports of no significant changes in the quality or quantity of daily food intake after surgery (41, 46). These inconsistencies could be explained by inaccuracies in food intake measurements. Patients with PD display a large amount of interindividual variation of non-motor symptoms including autonomic dysfunction, olfactory hypoxia, mood disturbances, sleep disorders, and gastrointestinal dysfunction. However, the association of non-motor symptoms with body weight and daily food intake in patients with PD has not been confirmed, and findings have often been contradictory (47, 48). Patients with dystonia also show non-motor symptoms including pain, cognitive, and sleep disturbances (8); therefore, the association between non-motor symptoms and weight change in patients with dystonia deserves further study.
The limitations of the current study include its retrospective study design and the absence of a control group. As this was a retrospective study, relevant preoperative data (hormonal and dietary diaries represent) are not available. We did not prospectively collect the patients' movement scores and BMI information during multiple follow-ups. Despite the follow-up time in our study, ranging from 4 to 83 months, previous studies have revealed that patients with isolated dystonia can achieve near-maximum improvement in motor scores and remain relatively stable for 3 months after STN-DBS (13). Despite a wide age range and different follow-up times, the one-way ANOVA showed no difference in the percentage change in BMI, BFMDRS-MS, and BFMDRS-DS in different subgroups. Nevertheless, the conclusions that can be drawn from this study are limited by the heterogeneity of isolated dystonia, age range of patients, and follow-up time. In the future, a larger sample size is required to study weight change after STN-DBS in patients with isolated dystonia, as well as to explore the mechanisms underlying WG after treatment. Also, due to the heterogeneity of isolated dystonia and the relatively small number of dystonia patients, we do not have data on the GPI-DBS matching STN-DBS group. Therefore, we did not use the GPI-DBS treatment group as the control group. Interestingly, the WG in patients with PD after STN-DBS was more significant than that in patients with PD after GPI-DBS (22).
Large-scale systematic studies are required to determine the reasons for postoperative weight change. Future studies should also focus on changes in non-motor symptoms, food intake, and EE (resting/free-living) after STN-DBS in patients with isolated dystonia. In addition, those patients in whom sustained or intermittent muscle contractions has little to no effect on the range of physical activity (such as those with dystonia involving only the eyelids) warrant further study. Such patients may be better disease models for studying weight change after STN-DBS. Their postoperative REE and free-living EE should be similar before and after STN-DBS, which should help elucidate reasons (such as changes in energy metabolism caused by direct stimulation), other than the improvement of motor symptoms, that lead to postprocedural weight change.
Conclusions
To our knowledge, this is the first report to investigate changes in body weight after STN-DBS in patients with isolated dystonia. We found that STN-DBS is an effective treatment to relieve symptoms of isolated dystonia, but that it may cause postoperative WG, especially in female patients. Such WG was correlated with preoperative weight, but not to DBS treatment efficacy of, for example, motor symptoms. Clinical teams must be more careful in choosing STN as a therapeutic target for DBS in patients with dystonia who are overweight or obese, especially women. Although this is the first case investigating change in body weight after STN-DBS in patients with isolated dystonia, more studies and long-term follow-ups are needed to determine the mechanisms underlying WG after STN-DBS operation.
Data Availability Statement
The original contributions presented in the study are included in the article/supplementary material, further inquiries can be directed to the corresponding author/s.
Ethics Statement
The studies involving human participants were reviewed and approved by the ethical committee of Ruijin Hospital. The patients/participants provided their written informed consent to participate in this study.
Author Contributions
WH: research project execution, statistical analysis design and execution, and manuscript writing of the first draft. HL: research project execution, and statistical analysis execution. YL and YuW: statistical analysis design and execution. YiW: research project conception and organization, statistical analysis design, review, critique, and manuscript review and critique. AR-Z: research project conception and organization, statistical analysis design, review, critique, and manuscript review and critique. WY and CZ: research project conception and organization, statistical analysis design, review, critique, and manuscript review and critique. All authors contributed to the article and approved the submitted version.
Conflict of Interest
The authors declare that the research was conducted in the absence of any commercial or financial relationships that could be construed as a potential conflict of interest.
Acknowledgments
We thank all of the participants who took part in our study.
References
1. Okun MS. Deep-brain stimulation for parkinson's disease. N Engl J Med. (2012) 367:1529–38. doi: 10.1056/NEJMct1208070
2. Koeglsperger T, Palleis C, Hell F, Mehrkens JH, Bötzel K. Deep brain stimulation programming for movement disorders: current concepts and evidence-Based strategies. Front Neurol. (2019) 10:410. doi: 10.3389/fneur.2019.00410
3. Zhou H, Wang L, Zhang C, Qiu X, Hu W, van der Stelt O, et al. Acute effects of subthalamic deep brain stimulation on motor outcomes in parkinson's disease; 13 year follow up. Front Neurol. (2019) 10:689. doi: 10.3389/fneur.2019.00689
4. Ramirez-Zamora A, Ostrem JL. Globus pallidus interna or subthalamic nucleus deep brain stimulation for parkinson disease: a review. JAMA Neurol. (2018) 75:367–72. doi: 10.1001/jamaneurol.2017.4321
5. Zhang J, Wang T, Zhang CC, Zeljic K, Zhan S, Sun BM, et al. The safety issues and hardware-related complications of deep brain stimulation therapy: a single-center retrospective analysis of 478 patients with parkinson's disease. Clin Interv Aging. (2017) 12:923–8. doi: 10.2147/CIA.S130882
6. Guimarães J, Matos E, Rosas MJ, Vieira-Coelho A, Borges N, Correia F, et al. Modulation of nutritional state in parkinsonian patients with bilateral subthalamic nucleus stimulation. J. Neurol. (2009) 256:2072–8. doi: 10.1007/s00415-009-5252-x
7. Fasano A, Daniele A, Albanese A. Treatment of motor and non-motor features of parkinson's disease with deep brain stimulation. Lancet Neurol. (2012) 11:429–42. doi: 10.1016/S1474-4422(12)70049-2
8. Eggink H, Szlufik S, Coenen MA, van Egmond ME, Moro E, Tijssen MAJ. Non-motor effects of deep brain stimulation in dystonia: a systematic review. Parkinsonism Relat Disord. (2018) 55:26–44. doi: 10.1016/j.parkreldis.2018.06.024
9. Steinhardt J, Münte TF, Schmid SM, Wilms B, Brüggemann N. A systematic review of body mass gain after deep brain stimulation of the subthalamic nucleus in patients with parkinson's disease. Obes Rev. (2020) 21:e12955. doi: 10.1111/obr.12955
10. Albanese A, Bhatia K, Bressman SB, Delong MR, Fahn S, Fung VSC, et al. Phenomenology and classification of dystonia: a consensus update. Mov Disord. (2013) 28:863–73. doi: 10.1002/mds.25475
11. Defazio G. The epidemiology of primary dystonia: current evidence and perspectives. Eur J Neurol. (2010) 17 Suppl. 1:9–14. doi: 10.1111/j.1468-1331.2010.03053.x
12. Liu Y, Zhu G, Jiang Y, Wang X, Chen Y, Meng F, et al. Comparison of short-Term stimulation of the globus pallidus interna and subthalamic nucleus for treatment of primary dystonia. World Neurosurg. (2019) 123:e211–e7. doi: 10.1016/j.wneu.2018.11.137
13. Lin S, Wu Y, Li H, Zhang C, Wang T, Pan Y, et al. Deep brain stimulation of the globus pallidus internus versus the subthalamic nucleus in isolated dystonia. J Neurosurg. (2019) 2019:1–12. doi: 10.3171/2018.12.JNS181927
14. Balint B, Mencacci NE, Valente EM, Pisani A, Rothwell J, Jankovic J, et al. Dystonia. Nat Rev. (2018) 4:25. doi: 10.1038/s41572-018-0039-y
15. Deng ZD, Li DY, Zhang CC, Pan YX, Zhang J, Jin H, et al. Long-term follow-up of bilateral subthalamic deep brain stimulation for refractory tardive dystonia. Parkinsonism Relat. Disord. (2017) 41:58–65. doi: 10.1016/j.parkreldis.2017.05.010
16. Ostrem JL, Racine CA, Glass GA, Grace JK, Volz MM, Heath SL, et al. Subthalamic nucleus deep brain stimulation in primary cervical dystonia. Neurology. (2011) 76:870–8. doi: 10.1212/WNL.0b013e31820f2e4f
17. Schjerling L, Hjermind LE, Jespersen B, Madsen FF, Brennum J, Jensen SR, et al. A randomized double-blind crossover trial comparing subthalamic and pallidal deep brain stimulation for dystonia. J Neurosurg. (2013) 119:1537–45. doi: 10.3171/2013.8.JNS13844
18. Cao C, Pan Y, Li D, Zhan S, Zhang J, Sun B. Subthalamus deep brain stimulation for primary dystonia patients: a long-term follow-up study. Mov Disord. (2013) 28:1877–82. doi: 10.1002/mds.25586
19. Ostrem JL, San Luciano M, Dodenhoff KA, Ziman N, Markun LC, Racine CA, et al. Subthalamic nucleus deep brain stimulation in isolated dystonia: a 3-year follow-up study. Neurology. (2017) 88:25–35. doi: 10.1212/WNL.0000000000003451
20. Zhan S, Sun F, Pan Y, Liu W, Huang P, Cao C, et al. Bilateral deep brain stimulation of the subthalamic nucleus in primary meige syndrome. J Neurosurg. (2018) 128:897–902. doi: 10.3171/2016.12.JNS16383
21. Xu W, Li H, Zhang C, Sun B, Wu Y, Li D. Subthalamic nucleus stimulation in pediatric isolated dystonia: a 10-Year follow-up. Can J Neurol Sci. (2020) 47:328–35. doi: 10.1017/cjn.2020.32
22. Mills KA, Scherzer R, Starr PA, Ostrem JL. Weight change after globus pallidus internus or subthalamic nucleus deep brain stimulation in parkinson's disease and dystonia. Stereotact Funct Neurosurg. (2012) 90:386–93. doi: 10.1159/000340071
23. Shetty AS, Bhatia KP, Lang AE. Dystonia and parkinson's disease: what is the relationship? Neurobiol Dis. (2019) 132:104462. doi: 10.1016/j.nbd.2019.05.001
24. Sun B, Chen S, Zhan S, Le W, Krahl SE. Subthalamic nucleus stimulation for primary dystonia and tardive dystonia. Acta Neurochir Suppl. (2007) 97:207–14. doi: 10.1007/978-3-211-33081-4_23
25. Zhu D, Chung HF, Pandeya N, Dobson AJ, Kuh D, Crawford SL, et al. Body mass index and age at natural menopause: an international pooled analysis of 11 prospective studies. Eur. J. Epidemiol. (2018) 33:699–710. doi: 10.1007/s10654-018-0367-y
26. Foubert-Samier A, Maurice S, Hivert S, Guelh D, Rigalleau V, Burbaud P, et al. A long-term follow-up of weight changes in subthalamic nucleus stimulated parkinson's disease patients. Rev Neurol. (2012) 168:173–6. doi: 10.1016/j.neurol.2011.04.006
27. Macia F, Perlemoine C, Coman I, Guehl D, Burbaud P, Cuny E, et al. Parkinson's disease patients with bilateral subthalamic deep brain stimulation gain weight. Mov Disord. (2004) 19:206–12. doi: 10.1002/mds.10630
28. Montaurier C, Morio B, Bannier S, Derost P, Arnaud P, Brandolini-Bunlon M, et al. Mechanisms of body weight gain in patients with parkinson's disease after subthalamic stimulation. Brain. (2007) 130:1808–18. doi: 10.1093/brain/awm113
29. Locke MC, Wu SS, Foote KD, Sassi M, Jacobson CE, Rodriguez RL, et al. Weight changes in subthalamic nucleus vs globus pallidus internus deep brain stimulation: results from the cOMPARE parkinson disease deep brain stimulation cohort. Neurosurgery. (2011) 68:1233–7; discussion 7–8. doi: 10.1227/NEU.0b013e31820b52c5
30. Berthoud HR. Multiple neural systems controlling food intake and body weight. Neurosci Biobehav Rev. (2002) 26:393–428. doi: 10.1016/S0149-7634(02)00014-3
31. Haegelen C, Rouaud T, Darnault P, Morandi X. The subthalamic nucleus is a key-structure of limbic basal ganglia functions. Med Hypotheses. (2009) 72:421–6. doi: 10.1016/j.mehy.2008.07.065
32. Lardeux S, Pernaud R, Paleressompoulle D, Baunez C. Beyond the reward pathway: coding reward magnitude and error in the rat subthalamic nucleus. J Neurophysiol. (2009) 102:2526–37. doi: 10.1152/jn.91009.2008
33. Rouaud T, Lardeux S, Panayotis N, Paleressompoulle D, Cador M, Baunez C. Reducing the desire for cocaine with subthalamic nucleus deep brain stimulation. Proc Natl Acad Sci USA. (2010) 107:1196–200. doi: 10.1073/pnas.0908189107
34. Wise RA. Forebrain substrates of reward and motivation. J Comp Neurol. (2005) 493:115–21. doi: 10.1002/cne.20689
35. Lipp A, Tank J, Trottenberg T, Kupsch A, Arnold G, Jordan J. Sympathetic activation due to deep brain stimulation in the region of the sTN. Neurology. (2005) 65:774–5. doi: 10.1212/01.wnl.0000174436.36399.ca
36. RuŽička E, Nováková L, Jech R, Urgošík D, RuŽička F, Haluzík M. Decrease in blood cortisol corresponds to weight gain following deep brain stimulation of the subthalamic nucleus in parkinson's disease. Stereot Funct Neurosurg. (2012) 90:410–1. doi: 10.1159/000341707
37. Escamilla-Sevilla F, Pérez-Navarro MJ, Muñoz-Pasadas M, Sáez-Zea C, Jouma-Katati M, Piédrola-Maroto G, et al. Change of the melanocortin system caused by bilateral subthalamic nucleus stimulation in parkinson's disease. Acta Neurol Scand. (2011) 124:275–81. doi: 10.1111/j.1600-0404.2010.01469.x
38. Markaki E, Ellul J, Kefalopoulou Z, Trachani E, Theodoropoulou A, Kyriazopoulou V, et al. The role of ghrelin, neuropeptide y and leptin peptides in weight gain after deep brain stimulation for parkinson's disease. Stereotact Funct Neurosurg. (2012) 90:104–12. doi: 10.1159/000335045
39. Novakova L, Haluzik M, Jech R, Urgosik D, Ruzicka F, Ruzicka E. Hormonal regulators of food intake and weight gain in parkinson's disease after subthalamic nucleus stimulation. Neuro Endocrinol Lett. (2011) 32:437–41.
40. Aiello M, Eleopra R, Foroni F, Rinaldo S, Rumiati RI. Weight gain after sTN-DBS: the role of reward sensitivity and impulsivity. Cortex. (2017) 92:150–61. doi: 10.1016/j.cortex.2017.04.005
41. Barichella M, Marczewska AM, Mariani C, Landi A, Vairo A, Pezzoli G. Body weight gain rate in patients with parkinson's disease and deep brain stimulation. Mov Disord. (2003) 18:1337–40. doi: 10.1002/mds.10543
42. Castrioto A, Lhommée E, Moro E, Krack P. Mood and behavioural effects of subthalamic stimulation in parkinson's disease. Lancet. (2014) 13:287–305. doi: 10.1016/S1474-4422(13)70294-1
43. Sauleau P, Le Jeune F, Drapier S, Houvenaghel JF, Dondaine T, Haegelen C, et al. Weight gain following subthalamic nucleus deep brain stimulation: a pET study. Mov Disord. (2014) 29:1781–7. doi: 10.1002/mds.26063
44. Serranová T, Jech R, Dušek P, Sieger T, RuŽička F, Urgošík D, et al. Subthalamic nucleus stimulation affects incentive salience attribution in parkinson's disease. Mov Disord. (2011) 26:2260–6. doi: 10.1002/mds.23880
45. Serranová T, Sieger T, Dušek P, RuŽička F, Urgošík D, RuŽička E, et al. Sex, food and threat: startling changes after subthalamic stimulation in parkinson's disease. Brain Stimul. (2013) 6:740–5. doi: 10.1016/j.brs.2013.03.009
46. Tuite PJ, Maxwell RE, Ikramuddin S, Kotz CM, Kotzd CM, Billington CJ, et al. Weight and body mass index in parkinson's disease patients after deep brain stimulation surgery. Parkinson Rel Disord. (2005) 11:247–52. doi: 10.1016/j.parkreldis.2005.01.006
47. Aiello M, Eleopra R, Rumiati RI. Body weight and food intake in parkinson's disease. A review of the association to non-motor symptoms. Appetite. (2015) 84:204–11. doi: 10.1016/j.appet.2014.10.011
Keywords: isolated dystonia, subthalamic nucleus, deep brain stimulation, Burke-Fahn-Marsden dystonia rating scale, weight gain
Citation: He W, Li H, Lai Y, Wu Y, Wu Y, Ramirez-Zamora A, Yi W and Zhang C (2021) Weight Change After Subthalamic Nucleus Deep Brain Stimulation in Patients With Isolated Dystonia. Front. Neurol. 12:632913. doi: 10.3389/fneur.2021.632913
Received: 24 November 2020; Accepted: 21 January 2021;
Published: 24 February 2021.
Edited by:
Jeremy Micah Crook, University of Wollongong, AustraliaReviewed by:
Aristide Merola, The Ohio State University, United StatesHortensia Gimeno, King's College London, United Kingdom
Copyright © 2021 He, Li, Lai, Wu, Wu, Ramirez-Zamora, Yi and Zhang. This is an open-access article distributed under the terms of the Creative Commons Attribution License (CC BY). The use, distribution or reproduction in other forums is permitted, provided the original author(s) and the copyright owner(s) are credited and that the original publication in this journal is cited, in accordance with accepted academic practice. No use, distribution or reproduction is permitted which does not comply with these terms.
*Correspondence: Wei Yi, d2VpeWkucmVubWluJiN4MDAwNDA7d2h1LmVkdS5jbg==; Chencheng Zhang, aSYjeDAwMDQwO2Njemhhbmcub3Jn