- 1Defense Health Group, Leidos, San Diego, CA, United States
- 2Health and Behavioral Sciences Department, Naval Health Research Center, San Diego, CA, United States
Although blast exposure has been recognized as a significant source of morbidity and mortality in military populations, our understanding of the effects of blast exposure, particularly low-level blast (LLB) exposure, on health outcomes remains limited. This scoping review provides a comprehensive, accessible review of the peer-reviewed literature that has been published on blast exposure over the past two decades, with specific emphasis on LLB. We conducted a comprehensive scoping review of the scientific literature published between January 2000 and 2019 pertaining to the effects of blast injury and/or exposure on human and animal health. A three-level review process with specific inclusion and exclusion criteria was used. A full-text review of all articles pertaining to LLB exposure was conducted and relevant study characteristics were extracted. The research team identified 3,215 blast-relevant articles, approximately half of which (55.4%) studied live humans, 16% studied animals, and the remainder were non-subjects research (e.g., literature reviews). Nearly all (99.49%) of the included studies were conducted by experts in medicine or epidemiology; approximately half of these articles were categorized into more than one medical specialty. Among the 51 articles identified as pertaining to LLB specifically, 45.1% were conducted on animals and 39.2% focused on human subjects. Animal studies of LLB predominately used shock tubes to induce various blast exposures in rats, assessed a variety of outcomes, and clearly demonstrated that LLB exposure is associated with brain injury. In contrast, the majority of LLB studies on humans were conducted among military and law enforcement personnel in training environments and had remarkable variability in the exposures and outcomes assessed. While findings suggest that there is the potential for LLB to harm human populations, findings are mixed and more research is needed. Although it is clear that more research is needed on this rapidly growing topic, this review highlights the detrimental effects of LLB on the health of both animals and humans. Future research would benefit from multidisciplinary collaboration, larger sample sizes, and standardization of terminology, exposures, and outcomes.
Introduction
Modern explosives have grown increasingly destructive over time and have been identified as the leading cause of injuries in recent conflicts such as Operation Enduring Freedom and Operation Iraqi Freedom (1). In response, advances in personal protective equipment (PPE) and combat casualty care have been prioritized and have resulted in increased rates of survival following blast exposure (2). However, while service members are more likely to survive exposure to blast now than in the past, blast exposure is still associated with significant morbidity and mortality and can result in a variety of adverse health outcomes, including traumatic amputations, traumatic brain injury (TBI), posttraumatic stress disorder, and chronic pain (3, 4). Because estimates suggest that 10–20% of veterans returning from deployment have sustained a TBI, the majority of which are the direct result of blast exposure (5), TBI in particular has received a great deal of attention as a threat to service member health and well-being (6).
When most people think of explosive blasts, the image that comes to mind is a high-level blast (HLB), such as the detonation of an improvised explosive device in combat or a terrorist attack such as the Boston Marathon Bombing in 2013. In such cases, explosives emit a blast wave which causes a transient increase in overpressure, followed by a negative pressure phase, before returning to ambient pressure. During this process, those that are nearby can be subject to injury through five mechanisms: the overpressure wave (i.e., primary blast injury), debris (i.e., secondary blast injury), physical displacement of one's body (i.e., tertiary blast injury), heat and toxins (i.e., quaternary blast injury), and environmental contaminants (i.e., quinary blast injury) (7–9).
One type of blast overpressure that is still insufficiently understood by the scientific community is a commonly underestimated form of blast exposure: low-level blast (LLB). Although there is no clear consensus regarding the distinction between HLB and LLB, subject matter experts (SMEs) agree that an LLB is a form of overpressure that is typically below the strength or intensity of an HLB and can result from firing heavy caliber weapons (e.g., Carl Gustaf bazooka, a Howitzer cannon). Empirical research into this form of overpressure dates back to the early 1980's, when researchers examined the association between LLB and pulmonary injury (10). Research on LLB was reinvigorated in the 2000's, when military operators, particularly instructors of advanced training courses (e.g., breacher training courses), reported that they were experiencing a number of symptoms typically associated with concussion and expressed concerns about their long-term health and well-being following such exposures (11).
With increased recognition of the potential adverse health outcomes associated with both HLB and LLB, our understanding of the effects of blast overpressure has advanced remarkably over the past 20 years. Due to the complexity of explosions themselves, as well as the diverse nature of their potential ramifications for health, a full understanding of blast and its effects requires the utilization of multiple disciplinary perspectives and approaches (8, 9, 12–15). For example, physicists study shock waves and how they differ across environments (16). Engineers develop PPE and gauges to measure blast overpressure (17). Medical providers and researchers investigate the effects of blast on various systems within the human body (e.g., neurological, pulmonary, or auditory systems) (8). Much of this health-focused research in particular is conducted with the ultimate goal of identifying avenues for screening, mitigation, and treatment of blast-induced injuries.
Unfortunately, findings from blast research often fail to cross discipline boundaries and instead remain siloed; most literature reviews on blast exposure tend to focus on a single topic or injury (e.g., blast-induced TBI, blast-induced ocular injury) (12, 18–20). Due to the nature of blast exposure and its potential consequences, multidisciplinary efforts will be required to prevent, mitigate, and treat those exposed to blast. However, developing a truly comprehensive understanding of blast exposure is a near-Herculean effort that requires extensive subject matter expertise across a wide variety of disciplines. Thus, there is a need for a recent, accessible, and comprehensive review of the scientific literature exploring the effects of blast overpressure exposure on health and performance.
The purpose of this review was to identify research on the health-related consequences of overpressure exposure that has been published in peer-reviewed outlets and is publicly accessible to the scientific community. Specifically, we present findings from a scoping review with two complementary foci. The first goal was to provide a broad overview of the interdisciplinary nature of blast research generally; the second was to thoroughly summarize research examining LLB that has been published within the past two decades. We believe this dual-focus approach was necessary due to a lack of consistency in the definition of LLB and related terms, which muddles the distinction between HLB and LLB; this might have resulted in failure to identify relevant literature through database searches if only LLB-related terms had been used. Although there is a critical gap in the literature regarding the distinction between HLB and LLB that desperately needs to be addressed, any such discussion requires a paper of its own and is beyond the scope of this paper.
Due to the particularly broad and complex nature of blast research, which uses a variety of sophisticated designs with diverse populations, we opted to conduct a scoping review rather than a traditional systematic literature review. Briefly, a scoping review is a type of systematic literature review that is designed to map an existing body of diverse literature to characterize the extent, range, and nature of research activities within a topic area (21). Whereas, traditional systematic literature reviews were designed to provide thorough summaries of results bearing on a specific research question addressed with a specific methodology, scoping reviews are used to provide an overview of research on broad topics that may have been addressed with a variety of methodologies. Due to the breadth and diversity of the literature surveyed, scoping reviews are not designed to assess the risk of bias within individual studies or to aggregate principal summary measures or estimates of effect sizes (e.g., odds ratios). However, scoping reviews are more appropriate than traditional systematic literature reviews when the purpose of the review is to characterize large bodies of relevant literature across diverse sources, topic areas, and research designs (22).
Materials and Methods
Established guidelines (PRISMA-ScR) were followed to ensure methodological rigor (23).
Data Sources
In January 2019, we conducted a literature search to identify all existing peer-reviewed journal articles pertaining to blast injury and/or exposure that may have implications for health and well-being. We searched PubMed for articles published between January 2000 and January 2019 using the following comprehensive list of search terms: blast injury(ies), blast exposure(s), blast wave(s), as well as the co-occurrence of blast with each of the following terms: bullets, wounds, low-level, low pressure, low intensity, lung, force, trauma, traumatic, concussion, induced, pressure, overpressure, and over pressure (e.g., “blast and bullets,” “blast and wounds”). All duplicates and non-English language articles were removed prior to evaluation for relevance and data extraction.
Study Selection and Data Extraction
Each identified article from the PubMed search was subject to a progressive three-level review process. Each level was completed by two members of the research team. During the first level of review, one of two independent reviewers examined the title and abstract to determine if the article discussed a topic related to overpressure. If an article was deemed relevant, a level 2 review was performed in which the reviewers extracted information from the abstract regarding the type of overpressure (HLB vs. LLB), study population, scientific discipline of researchers (for human studies only), and the medical specialty discussed (if relevant), using a standardized data extraction form in DistillerSR (Evidence Partners, Ottawa, Canada; see Table 1). Articles deemed relevant to LLB received a full-text level 3 review in which two reviewers independently reviewed and extracted data using a standardized form in Microsoft Word (see Supplementary Material).
Interrater Reliability
A random 10% of articles included in levels 1 and 2 were reviewed by both reviewers and inter-rater reliability (IRR) was calculated via Cohen's kappa (24). There was substantial agreement (κ = 0.72) on whether articles were deemed relevant to blast overpressure at level 1 and greater than substantial agreement for the data extracted at level 2 (κ = 0.82) (25). Discrepancies between reviewers were resolved through negotiated consensus. IRR was not calculated for data extracted by the two reviewers at level 3 because the data were primarily qualitative in nature and a direct comparison of reviewers' responses indicated that disagreements were rare; disagreements were resolved through discussion between reviewers.
Ensuring Comprehensiveness of the Search
After the initial PubMed search was completed, three additional strategies were employed to ensure the comprehensiveness of our search. First, we conducted additional searches in PsycInfo and WebofScience using the search terms previously described. Second, references cited in all papers that received level 3 review were examined for possible relevance. Third, we used Google Scholar to identify papers that referenced the level 3 articles and reviewed their title and abstracts for potential relevance. Data were extracted from all articles identified through this process using the level 3 review process previously described.
Results
Level 1 Results
Literature Search
The initial PubMed search yielded a total of 5,596 peer-reviewed articles, 3,215 of which were identified as relevant to blast overpressure. Forty-three articles from the PubMed search were identified as relevant to LLB, and eight additional articles relevant to LLB were identified through the added literature search methods described previously. This yielded 51 articles that received full-text review and data extraction during level 3. Figure 1 depicts the PRISMA diagram of the literature search process.
Level 2 Results
Blast Overpressure
A summary of the study characteristics extracted from articles during level 2 review are presented in Table 2. Of the 3,215 articles identified as being relevant to blast overpressure, the majority of articles studied live humans (55.37%), while the remainder of the articles were relatively evenly split between those studying animals (16.05%), literature reviews (16.33%), and none of the above (20.16%; e.g., articles on the development of equipment such as helmets that did not involve animals or humans). It is important to note that 6.97% of articles fit into more than one of these categories. Additionally, nearly all research published on blast and identified by our search criteria were conducted by experts working in medicine or epidemiology (99.49%). Only 0.90% of articles were conducted by physicists or engineers and 0.67% were conducted by experts in other disciplines (e.g., education, management, anthropology). Very few articles (n = 19; 1.07%) were coded into more than one disciplinary category. Of the 1,771 articles that studied humans and were conducted by researchers within medical or epidemiological fields, approximately half (49.41%) were coded into more than one medical specialty (M = 1.56, SD = 0.62). Most published papers focused on non-neurological injury (i.e., injuries excluding TBI; 66.06%), followed by neurology (including TBI; 24.56%), mental health (13.33%), and pulmonology (5.99%). Surprisingly, 45.57% of papers were coded into an “other” category, the majority of which were subsequently identified as articles on management (e.g., hospital staffing required during blast-related mass casualties).
LLB
Of the 51 articles identified that addressed LLB, 20 presented empirical data on humans (11, 26–44), 23 presented empirical data on animals (45–66), 1 presented findings from a study developing computational models of LLB exposure (17), and 7 articles were non-empirical (primarily literature reviews or commentaries) (67–73). Table 3 briefly summarizes the essential characteristics of each of the 43 empirical studies, which are further elaborated upon in the Discussion.
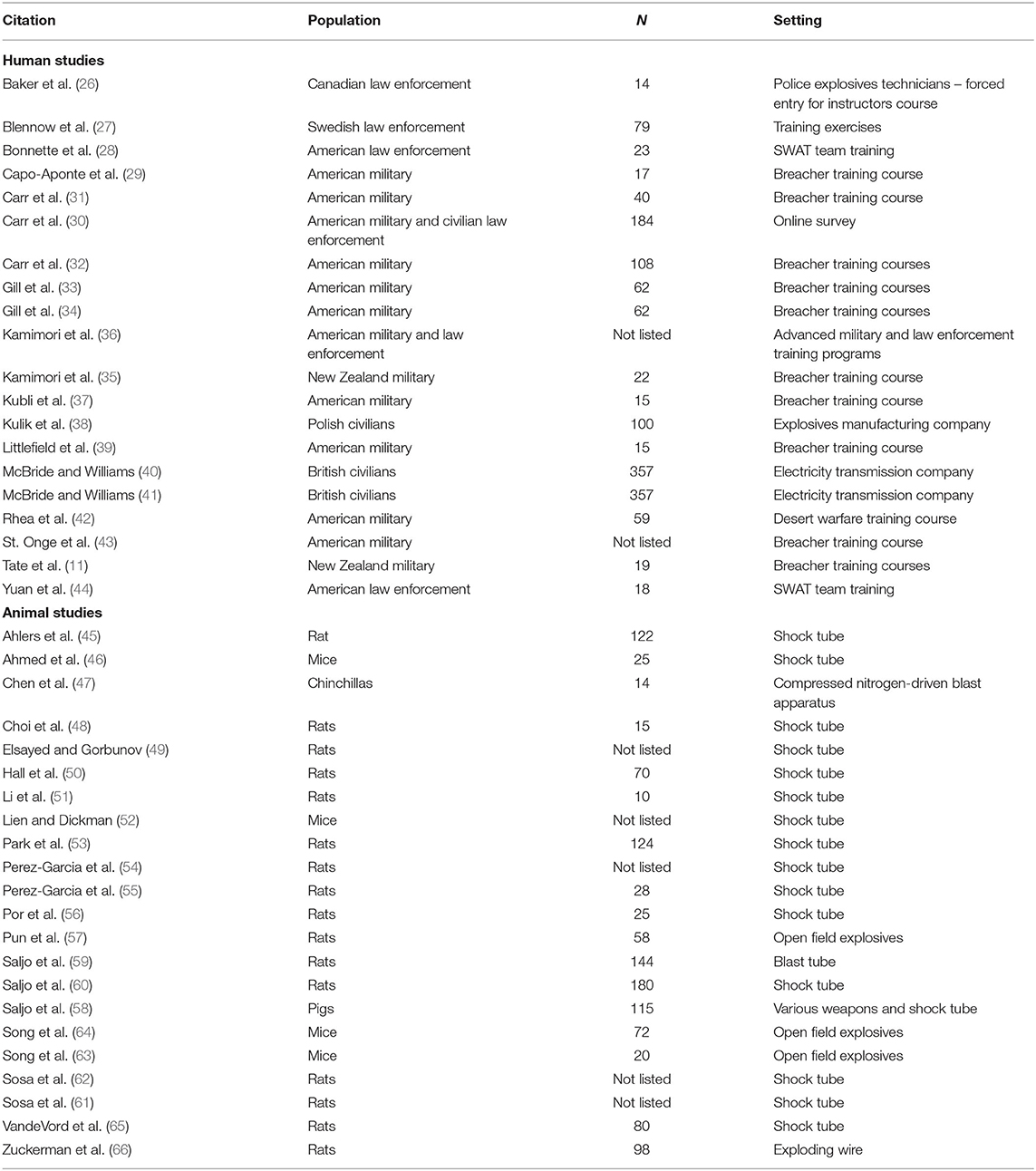
Table 3. Population, sample size, and setting for empirical studies of low-level blast in humans and animals.
Level 3 Results
Overview of HLB
In the past two decades, more than 3,000 articles on the potential health and well-being sequelae associated with blast overpressure have been published, approximately half of which reported data obtained from live human participants. While we anticipated that most of this research would have been published by scholars working in medicine or epidemiology at the outset of this review, we discovered remarkable variability in the medical specialties represented in the literature. Although TBI has been labeled the signature injury of recent conflicts and most deployment-related TBIs are caused by blast (74–76), articles on non-neurological (e.g., traumatic amputation), as opposed to neurological injuries, were published more than twice as often. Articles on mental health emerged frequently, likely because of the comorbidity of TBI and mental health conditions (77–79). It was, however, surprising that nearly half of the medical or epidemiological articles coded were categorized into an “other” category, with hundreds of articles focusing on medical management. These articles tended to focus on the management of healthcare staff and/or facilities (e.g., required trainings, required levels of staffing) as they pertain to treatment of blast-induced injuries (e.g., lessons learned following the Boston Marathon Bombing). Because nearly half of medical/epidemiological studies on humans were coded into more than one subspecialty, our findings demonstrated that health researchers and medical providers from different specialties have begun to collaborate in examining the effects of blast exposure. This contrasts starkly with the general lack of collaboration and cross-talk between members of other scientific disciplines studying the effects of blast exposure. Taken together, evidence from the past two decades clearly shows that HLB exposure can result in injury and/or death, and thus presents a significant threat to warfighter health and well-being.
Overview of LLB
Although more than 3,000 articles on blast overpressure have been published since 2000, fewer than 2% of these articles specifically examined health outcomes that may be associated with LLB. Most articles focused on providing empirical data based on animal and human studies, though a few literature reviews and commentaries have been published as well. Our in-depth review of LLB articles revealed substantial diversity in purpose, study design, and conclusions, which we describe subsequently.
LLB: Animal Studies
Purpose
In general, animal studies of LLB articulated methods to induce blast exposure in well-controlled environments (45–66), examined longitudinal outcomes associated with LLB (46, 64) or repeated exposures to LLB (49), or assessed the impact of environmental characteristics on outcomes associated with LLB (58). Studies frequently examined neurological outcomes, such as neuroinflammation and neurodegeneration (51, 57, 61–63) and damage to specific brain regions or types of cells (53, 65). Animal studies also examined outcomes related to mental health (54, 55) and auditory (47), visual (48, 56), vestibular (52), and vascular (50) systems. Only one study specifically examined an intervention to attenuate adverse outcomes associated with LLB exposure (60).
Subjects and Setting
Animal studies used predominately rats (45, 48–51, 53–57, 59–62, 65, 66). Sample sizes in studies using rats ranged from 10 to 180, with 75% of studies including fewer than 100 subjects. Other species studied included mice (46, 52, 63, 64), pigs (58), and chinchillas (47). Sample size for these species varied from 14 (chinchillas) to 115 (pigs). Five studies failed to report a total sample size (49, 52, 54, 61, 62). As expected, all studies using animal subjects were conducted in laboratories.
Exposures
In general, LLB exposures were most frequently induced via shock tubes (45, 48, 50, 51, 54, 55, 61) with anesthetized animals (48, 49, 51–57, 61, 62). Most of these studies used the Walter Reed Army Institute of Research shock tube (45, 48, 50, 51, 54, 55, 61) though four studies were conducted at other locations (47, 52, 56, 65). Non–shock tube-induced exposures included subjecting animals to actual weapons systems (e.g., Howitzers, bazookas) (58), explosives (e.g., TNT, C4) (57, 63, 64), and impulse noise (59). In a particularly compelling study of LLB exposure meant to mimic real-world LLB, Zuckerman et al. (66) used an exploding wire technique that allowed for some rats to be subjected to both the psychological stressors and overpressure simultaneously, while others were exposed to only the psychological stressors associated with LLB without the corresponding overpressure. In several studies, animals were exposed to blast only once (45, 52, 65). However, many studies involved repeated exposures (47–49, 54–56, 60, 61) or a combination of single and repeated exposures (56), with the 12 as the highest number of exposures (45, 50). The interval between repeated exposures was most commonly 1 day (45, 48, 50, 54–56, 61), although some induced repeated exposures only minutes (46, 49) or hours apart (47).
In most animal studies of LLB, peak overpressure levels were reported in kilopascals (kPa). There was not only tremendous variability in peak pressures induced, but more importantly, almost all of these exposures exceeded the 4 psi (or ~27.58 kPa) threshold that is often used to differentiate LLB from HLB (35, 36, 46, 67). The peak pressures induced in the lab ranged from 10 kPa (~1.45 psi) (58, 60) to 153 kPa (~22.19 psi) (65), and only three studies meeting our inclusion criteria reported inducing peak pressures below the 4 psi threshold (47, 58, 60).
Studies varied in the physical orientation of the animal relative to the blast, as well as whether restraints were used (e.g., to restrict potential damage from tertiary blast exposure) (46, 49, 50, 54, 57, 61, 65). Animals were typically positioned frontally so the blast reached the head first (51, 54, 55, 60, 61) and were often 1 foot or less from the source of the overpressure (51, 53, 60). Some studies varied, depending on whether animals were restrained as part of the experimental design (45, 56).
Outcomes
A wide variety of outcomes were assessed, including measures of physical injury, cognitive and behavioral outcomes, and biomarkers. In terms of general physical outcomes assessed, two studies examined vital signs, such as respiration, heart rate, and body weight, as well as several indicators of neurological injury (e.g., increased intracranial pressure) (46, 58). Additionally, several studies assessed motor dysfunction using rotarod tests (52, 53), balance beam tasks (45), horizontal ladder studies (65), and locomotor activity (55). Further, three studies directly examined injuries in the ocular (56) or auditory systems (47, 52). Behavioral assessments included measures of open-field behavior (53–55, 64), light/dark box behaviors (53, 55, 64), acoustic startle response (55, 66), fear conditioning (55), and a forced swimming test (66). Cognitive outcomes primarily involved assessments of spatial learning and memory, with the Morris water maze test being the most frequently used measure (45, 50, 55, 60, 64–66). There was a remarkable amount of variability in biomarkers assessed (45, 46, 48–53, 56–59, 61–65). including immunohistochemistry assessments for measures of glial fibrillary acidic protein (GFAP), amyloid precursor protein (APP), and more (45, 48, 52, 56, 58, 59, 64). Histopathology was also reported in several studies (45, 51, 57).
Summary of Findings From Animal Studies of LLB
Findings from animal studies of LLB have clearly demonstrated that LLB exposure is associated with unique and complex pathological processes (46, 50, 61, 63). Specifically, several studies have demonstrated brain injury as a result of LLB (45, 53, 56, 57, 59, 65), including that associated with neuroinflammation (51, 62), neurodegeneration (59), and white matter perturbations (57). Furthermore, LLB exposure was associated with injury to the visual (48), auditory (47), and vestibular systems (52). Beyond physical injury, LLB exposure was also associated with cognitive and behavioral changes in animals (64), including those resembling PTSD (54, 55, 66, 70) and deficits in learning and memory (45, 50, 65). The effects of repeated LLB exposure in particular are still somewhat mixed in that some studies found greater risk of injury with repeated (vs. single) exposure [e.g., increased learning deficits (45), enhanced pain responses (56), increased activated caspase 3 in the optic nerve (48)], while others have not [e.g., no significant differences in pulmonary-related outcomes (49)]. This suggests that effects of repeated LLB may vary across systems, although more research is needed to conclusively establish these differences. Even so, animal researchers have articulated a need for protection from and prompt treatment for LLB-induced injuries (49).
LLB: Human Studies
Purpose
The majority of studies examining the effects of LLB on humans attempted to determine if exposure is associated with acute and long-term effects, such as impaired neurological functioning (11, 31, 32, 35, 44); neurochemical evidence of brain damage (27, 32–35); damage to auditory (37, 43), vestibular (26, 39, 43), or visual systems (29); and self-reported symptoms (11, 26, 30, 35). Additionally, one study attempted to quantify and validate the extent of LLB exposure during military and law enforcement training exercises (36). These studies predominantly examined military service members or law enforcement personnel and were primarily observational in nature. Other studies examined outcomes associated with occupational exposure to impulse noise and/or overpressure from explosives (38), exposure to air blast circuit breakers (40, 41), and the effectiveness of specific equipment [e.g., to measure neuromotor function after blast exposure (42), PPE (28, 44)].
Subjects and Setting
Surprisingly, only 11 papers reported studies exclusively on active duty personnel. Three studies reported data from both military and non-military personnel [i.e., civilian law enforcement (27, 30, 36)], while six reported data on purely non-military personnel, including civilian personnel working in law enforcement and corporations or volunteers (26, 28, 38, 40, 41, 44).
Of the 20 published peer-reviewed studies on humans, 16 were conducted in training environments, three were conducted in corporate settings, and one was an online survey. Studies on military personnel have focused predominantly on service members attending Marine Corps or Army breacher training courses (29, 31–34, 37, 39, 43). Samples of law enforcement personnel included American Special Weapons and Tactics (SWAT) teams (28, 44) and Canadian Police (26). Four studies were conducted in non-training environments. These included an online survey of military and civilian law enforcement personnel (30), examination of the association between exposure to blast-induced impulse noise and irritability and anxiety symptoms at an explosives production company (38), and two examinations of noise-induced hearing loss among workers at an electrical transmission company, which the authors argued exposed workers to LLB similar in intensity and mechanism to that produced by firearms (40, 41).
In general, human studies on LLB exposure used relatively small samples in final analysis that ranged in size from 14 to 357, with an average of 83 participants. Of the five studies that included more than 100 participants, three reported data provided by civilian corporate employees (38, 40, 41), one was the web-based survey conducted by Carr et al. (30), and only one involved breachers (32). When only studies of military and civilian law enforcement training programs were examined, the average sample size was substantially smaller (~39 participants).
Exposures
In general, there was a notable amount of variability in the LLB exposures to which humans were subjected, as well as the detail in which it was described by authors, including a lack of information in some studies (35, 43). Several studies included examinations of LLB exposure from various weapons, including Howitzers (27, 36), the Carl Gustaf bazooka (28, 42), rocket-propelled grenades (42), light anti-tank weapons (42), shotgun door-breaching rounds (36), mortars (36), M4 Carbine rifles (36), and C4 (28). Training programs in which these studies were conducted ranged from 1 day (44) to 3 weeks (42), although most training courses were 2 weeks in duration (11, 26, 31–33, 35, 39, 43).
Several studies included measures of frequency of exposure to LLB, although the referent time frame varied across studies. Mean daily frequency of exposures was 10 in one study and 39 in another (38–44). Mean weekly exposures ranged between 40 and 50 per week (31, 38). In some cases, the frequency of exposures for instructors was reported as a calculated yearly metric and suggests that instructors experience hundreds of LLBs per year (29, 37, 39).
Estimated or measured overpressure exposure also varied. Several studies reported that exposures were generally below the 4 psi threshold thought to be safe from risk of tympanic membrane rupture (31, 37, 67). For example, some studies reported mean peak pressures ranging between 1 and 3 psi (28, 44), although one study reported mean peak pressures ranging between 4.8 and 8.5 psi (42). However, several studies reported that at least one subject who was exposed to overpressure that exceeded the 4 psi threshold, including exposure > 5 psi (33), 6 psi (37), 8 psi (32), 12 psi (36), and 13 psi (31). Only one paper reported total impulse pressure, which was measured at 7.5–19.4 psi per ms (42).
Outcomes
Studies of the effects of LLB on humans assessed a wide variety of outcomes (see Figure 2). Measures of self-reported symptoms (e.g., the Neurobehavioral Symptom Inventory, a collection of concussion-related symptoms) were most frequently collected, followed by objective markers of brain health (e.g., GFAP, ubiquitin carboxy-terminal hydrolase L1 [UCH-L1]), and assessments of neurocognitive function (e.g., the Automated Neuropsychological Assessment Metrics [ANAM], the Defense Automated Neurobehavioral Assessment). Five or fewer papers included assessments of the auditory, vestibular, and visual systems, mental health, or other outcomes (i.e., actigraphy to measure sleep health). Only six specific measures were used in three or more peer-reviewed papers: blast gauges (31, 33, 36, 42, 44), concussion-related symptoms (11, 30, 32, 33, 35), the ANAM (11, 31, 32, 35), UCH-L1 (11, 32, 35), GFAP (11, 27, 35), and distortion product otoacoustic emissions (37, 43, 44).
Summary of Findings From Human Studies of LLB
In general, results of studies of LLB suggest that it has potential to cause harm to humans. Specifically, several studies suggested the potential for adverse outcomes for brain perturbation (11), including axonal disruption (28), changes in neuromotor functioning (42), and impairments in working memory (31, 44). Furthermore, a few studies provided suggestive evidence for changes in biomarkers such as UCH-L1 (32), peripheral inflammatory markers (34), or APP (33). However, it is also worth noting that these effects were not necessarily replicated across studies and that Blennow et al. failed to demonstrate any neurochemical evidence of brain damage (27).
Beyond objective assessments of damage to the brain, several studies have also clearly indicated subclinical symptoms experienced because of exposure to LLB (11, 30, 33) and impulse noise generated by explosive blasts (38). However, four studies failed to show significant increases in self-reported symptoms as a function of exposure to LLB (26, 35, 37, 39). Two study teams attributed these failures to differences between instructors and students at baseline (26) and exposures that were generally below the 4 psi threshold and may have thus been considered safe (35).
Other studies have investigated the threat of LLB exposure on visual, auditory, and vestibular health. Taken together, studies to date have demonstrated potential adverse outcomes on the visual system, but not on the auditory or vestibular systems. Although there was only a single study directly examining adverse outcomes in the visual system, this study suggested that LLB can result in acute injury to the eye (29), which has the potential to affect warfighter performance, particularly for special forces operators (72). Hearing loss is often discussed as being a direct result of repeated exposure to loud noises such as those associated with overpressure exposure, but findings on the relationship between LLB and hearing loss were mixed. Studies by St. Onge et al. (43) and McBride and Williams (40, 41) suggest that exposure to LLB may result in hearing loss, but a study by Kubli et al. (37) did not. Regarding vestibular system functioning, although Carr et al. (32) showed deficits on two measures of vestibular system functioning, the other two measures they included did not show similar patterns. Research by St. Onge et al. (43) and Baker et al. (27) also did not show decrements in vestibular system functioning following LLB exposure during military and law enforcement breacher training courses, though the latter did show differences between instructors and students at baseline. In the one study that focused directly on vestibular system functioning of breaching instructors over 17 months and included a large battery of measures, Littlefield et al. (39) concluded that there were no adverse outcomes for vestibular system functioning as a result of LLB exposure. They did, however, demonstrate that there were differences in upbeat positional nystagmus for those with a history of mild TBI, the majority of which were presumably induced by HLB, and suggested that this could be an outcome of acute HLB, but perhaps not LLB (39).
Limitations of Studies on LLB
Animal Studies
Although it appears to be the norm that papers presenting findings of the effects of LLB on animals do not discuss limitations of their work (46–50, 52–57, 59, 60, 62–65), a few articles addressed their limitations. These self-articulated limitations included concerns that the shock tubes used to generate LLB may not approximate the LLB exposure experienced by warfighters (45, 66), that adverse outcomes identified may not be reducible to purely primary blast (45), that pathophysiological processes that are apparent in animals may not necessarily translate to humans (66), and that these studies used small sample sizes (51, 66).
Beyond these self-identified limitations of animal research on LLB, several other limitations warrant further exposition. The first and perhaps most important limitation is that most of the animal studies investigating the effects of LLB exposure actually induced overpressure that exceeds the oft-discussed, yet arbitrary, 4 psi threshold expected to differentiate LLB from more intense blast exposure (28, 30, 32, 35–37, 42, 44, 67), and thus are not necessarily studies of LLB. Indeed, only 3 of the 23 articles on animals used overpressure exposure at or below the 4 psi threshold. This highlights the need for a clear discussion of thresholds for what constitutes LLB vs. what is no longer low-level blast.
Second, although animal models present an excellent opportunity to conduct basic science with the goal of understanding the complex processes resulting from LLB exposure, the animals or methods most frequently used may not be the best approximation for how such injuries or pathological processes may translate to humans (13). For example, there are remarkable anatomical differences between humans and most species used in animal research (80). This limits the ability to directly leverage the knowledge gained through these studies to inform clinical practice guidelines for the treatment of blast-induced injuries in humans at this time. However, this criticism is common in animal research that is aimed at understanding the processes involved in complex injuries and should not be taken as a reason to discount the animal literature entirely.
Third, although research sought to understand the effects of repeated exposure to LLB, the repetition of exposures was still quite limited, with the number of repetitions ranging from 2 to 12 exposures. Although these studies are valuable for understanding the effects of LLB exposure, this is not an appropriate approximation of repeated exposures that warfighters endure. For example, it is estimated that service members assigned to a 2–3 year tour as a breaching instructor will experience hundreds of exposures over the course of that specific tour alone (29, 37, 39). As a result, animal research on repeated blast exposure may not adequately inform our understanding of what our warfighters are truly facing, at least in some occupations.
Human Studies
Unlike animal studies of LLB, studies on humans regularly articulated a variety of limitations. The most articulated limitation was a limited sample size (11, 26, 28, 29, 31, 34, 37, 39, 40, 44), which typically occurred as a result of assessing LLB exposure in training environments. Studies on humans were generally quite small with an average sample size of ~83 for all included studies, and >40 for studies with data collected during military and law enforcement training programs. While data from instructors of such programs is particularly informative because they are suspected of having the largest cumulative exposure throughout their careers, the sample size of this unique subpopulation is meager and is frequently in the single digits in published studies.
Beyond limited sample sizes, there are several unique challenges associated with conducting research in warfighter training environments. First, these studies are primarily observational in nature. Second, such courses have intentionally low exposure over which the researchers have no control; such limited exposure may or may not result in adverse outcomes that are observable or quantifiable using existing measures, even if it has the potential to affect warfighter health and well-being over the course of their military careers (28, 37). Third, there can be remarkable variability in the LLB exposure of individuals within the same environment, and this can be challenging to assess at a person level rather than the overall event level (11). Fourth, the trainees and instructors in such courses that involve routine blast exposure are not randomly selected, and selection bias could limit the generalizability of these findings (30, 33). Furthermore, the study of training courses does not necessarily represent exposures incurred during less structured or regulated field training activities.
Several papers also articulated limitations associated with the study design or execution. For example, four papers noted the lack of a control group and/or appropriate controls (26, 32, 41, 42). Beyond this, several studies also highlighted that there is potential for an individual's personal history of blast exposure, head trauma, and/or extra-occupational activities (e.g., participation in sports) to influence the outcomes examined (26, 30, 35, 44), though such prior history was often not assessed. Additionally, some studies either did not include long-term follow-up or commented that their follow-up periods may not have been sufficient to observe differences in the outcomes of interest (28, 33, 42, 44). Two papers also articulated limitations associated with statistical procedures used (33, 40).
A number of studies described limitations associated with the measures they used. Some of these limitations related to the measures of blast exposure, such as an inability to articulate exposure at the individual person level (37), issues associated with the placement of blast sensors (32), and an inability to compare exposure levels with an analogous population (40). Another commonly mentioned limitation was that outcome measures often relied on self-report, which can be associated with recall bias and narrow ranges of reporting (e.g., for symptom reporting). Some researchers have also called for inclusion of more objective measures (11, 30, 40, 42, 67). A few other limitations, including those associated with missing measures [e.g., measuring certain additional biomarkers (32)] and difficulty in differentiating between commonly comorbid conditions (e.g., postconcussive syndrome and PTSD) when examining symptoms (30), were also discussed.
One additional limitation that was not discussed in the published studies of LLB using humans was a lack of assessment of blast exposure between training courses and follow-up. Although some studies collected follow-up data after the completion of training courses, limited efforts were made to account for additional exposures that may have occurred after the training but during the study window. Despite the plethora of limitations that afflict studies of LLB, our knowledge of the potential adverse outcomes of LLB has grown remarkably over time. Future research is and will continue to be needed to ensure an accurate understanding of the outcomes associated with overpressure exposure.
Discussion
In this research, we sought to conduct a scoping review of peer-reviewed articles that reported investigations of the potentially detrimental effects of overpressure exposure, with a targeted focus on LLB exposure. Because terminology for LLB exposure is inconsistent at best, we used a comprehensive list of search terms regarding both HLB and LLB. After reviewing titles and abstracts from nearly 5,600 articles, we identified 3,215 articles that were relevant to blast (HLB and/or LLB) exposure. Most of these articles were focused on human subjects and often represented more than one subspecialty of medicine. This highlights the complexity of blast effects on human tissues and underscores the importance of interdisciplinary approaches to blast injury research.
Although research on LLB is growing rapidly, it still presents a tiny fraction of research on blast injury. Specifically, our review located only 51 peer-reviewed published articles on LLB across the past 20 years, including both animal and human research. Although animal studies have generally identified several adverse effects on complex physiological processes, conclusions based on human subjects' research are somewhat mixed regarding whether LLB exposure may be a threat to warfighter health and performance. Nonetheless, the results of this review support previous assertions that LLB exposure has the potential to harm warfighters (67, 70). The most well-supported adverse outcomes identified in humans remain self-reported symptomology (e.g., headaches, trouble hearing), particularly among those with greater cumulative exposure (e.g., breacher training instructors) (45, 70). However, given the diversity of the outcomes examined to date, much more research is needed to expand our understanding of the potential effects of LLB exposure, including examining whether adverse outcomes associated with repetitive LLB exposure present a threat to performance of duties and/or increased risk of adverse long-term health outcomes, including Alzheimer's disease (69, 72, 73).
Although this scoping review was conducted according to published guidelines to ensure methodological rigor (23), several limitations of this work should be noted. First, our review intentionally only included peer-reviewed articles published in English and may thus have missed some important ongoing research. For example, research conducted by those affiliated with the Department of Defense (DoD) is often published in the form of white papers, briefs, and/or technical reports outside of peer-reviewed outlets and thus would not have met our inclusion criteria. However, our focus on peer-reviewed publications was intentional and based on the premise that such research has been completed and was found to be of sufficient merit to warrant publication and dissemination among the broader scientific community. The intention of this review was to provide a summary of the literature that had been made publicly available to researchers and medical providers, both within and outside of the DoD, rather than including additional reports that may be limited to only DoD personnel. For further information on such studies (including work currently in progress), interested readers are referred to the summary provided by Simmons et al. (81).
Second, although we used a comprehensive list of search terms, it is still possible that some articles were not captured in our review. To offset this limitation, we intentionally used search terms that were not limited to purely LLB. Unlike other recent reviews of LLB (81, 82), our review evaluated the title and abstract of nearly 5,600 articles investigating blast to identify those that were relevant to LLB. Because of the lack of consistency in the terminology used to describe LLB within the scientific community, this was the only way to ensure that all relevant articles were captured in our review. We also took several additional steps to ensure that the search was as thorough as possible. Even so, we note that it is still possible that our review missed some peer-reviewed articles on LLB (e.g., because they used shock waves that we did not deem to be low-level) (83). Additionally, it should also be noted that because the field of blast research is rapidly evolving, even the most comprehensive literature search will rapidly become outdated as more articles are published. For example, in the year since we conducted our search, several additional papers on LLB been published summarizing epidemiological investigations (84, 85), case studies (86), studies of training courses (87), animal studies (88), and more.
It is important to note that two other literature reviews on LLB were published within the past year. Specifically, one review was prepared by researchers at RAND (81) as part of their efforts to facilitate the Seventh DoD State-of-the-Science Meeting on blast injury research, a conference held in 2018 that focused specifically on the neurological effects of repeated LLB exposure. This report specifically examined all research, including peer-reviewed and gray literature, that received funding from the DoD, was conducted at a DoD laboratory, and/or had a DoD-affiliated author. Our review included fewer articles than the RAND review, due in large part to their inclusion of gray literature and ongoing as well as completed work. While their review highlights more ongoing and newly emerging work, much of it is not accessible to the public and/or has not been peer-reviewed for scientific rigor. Additionally, LLB exposure is more common in military populations, but important research on LLB is being conducted in non-military populations by researchers who are not affiliated with the DoD, including researchers outside of the United States (71), as evidenced by the 17 articles included in our review that were not DoD affiliated in any way.
The second review was prepared by researchers at the Defense and Veterans Brain Injury Center and included only peer-reviewed published literature on the effect of LLB on humans (82). Given the important advances in animal research that is directly relevant to LLB in human populations, we believe the inclusion of these articles in a comprehensive review is essential because animal research allows for more experimental control and more comprehensive investigation than can ethically be conducted with humans. As research on LLB continues to advance, SMEs from these scientific disciplines will need to collaborate to translate findings from animals to human populations and will need to be informed about all relevant literature. Additionally, while Belanger et al. review included two articles that ours did not [both of which were technical reports rather than peer-reviewed publications (89, 90)], our review identified four additional articles (36, 38, 40, 41) investigating the effects of LLB on humans than theirs, likely due to rigorous methods to identify additional studies that were not revealed through our initial literature search.
Conclusion
Due to the complexity of blast overpressure and its potential effects on the human body, a wide range of multidisciplinary expertise is required to identify prevention, mitigation, and treatment strategies for those exposed to blast overpressure. However, ensuring that emerging research is disseminated effectively to scholars from multiple disciplines has historically been a challenge. With this scoping review, we hope we have provided a clear, accessible overview of research on blast exposure that has been published within the past two decades, with a specific focus on LLB. It is intended to provide a detailed overview for researchers and medical providers from a variety of disciplines to increase awareness and understanding of the diverse studies on the topic. Research on the sequelae associated with blast overpressure exposure should and will continue to grow and develop over time, and it presents an important avenue for ensuring the health and well-being of members of the U.S. Armed Forces and others who are routinely exposed to blast.
Data Availability Statement
The raw data supporting the conclusions of this article will be made available by the authors, without undue reservation.
Author Contributions
JB led the research effort. SF and JJ coded data Levels 1 & 2. JB and SF coded data at Level 3. Manuscript was drafted primarily by JB and RE. All authors contributed to the theoretical framework of the paper, interpretation of results, contributed to the development of this manuscript, provided comments, and revisions.
Disclaimer
I am a military service member or employee of the U.S. Government. This work was prepared as part of my official duties. Title 17, U.S.C. §105 provides that copyright protection under this title is not available for any work of the U.S. Government. Title 17, U.S.C. §101 defines a U.S. Government work as work prepared by a military service member or employee of the U.S. Government as part of that person's official duties. Report No. 20-95 was supported by the U.S. Navy Bureau of Medicine and Surgery under work unit no. N1518. The views expressed in this article are those of the authors and do not necessarily reflect the official policy or position of the Department of the Navy, Department of Defense, nor the U.S. Government.
Conflict of Interest
The authors declare that the research was conducted in the absence of any commercial or financial relationships that could be construed as a potential conflict of interest.
Supplementary Material
The Supplementary Material for this article can be found online at: https://www.frontiersin.org/articles/10.3389/fneur.2021.628782/full#supplementary-material
References
1. Owens BD, Kragh JF, Wenke JC, Macaitis J, Wade CE, Holcomb JB. Combat wounds in operation Iraqi freedom and operation enduring freedom. J Trauma. (2008) 64:295–9. doi: 10.1097/TA.0b013e318163b875
2. Magnuson J, Leonessa F, Ling GSF. Neuropathology of explosive blast traumatic brain injury. Curr Neurol Neurosci R. (2012) 12:570–9. doi: 10.1007/s11910-012-0303-6
3. Hoge CW, McGurk D, Thomas JL, Cox AL, Engel CC, Castro CA. Mild traumatic brain injury in U.S. soldiers returning from Iraq. N Engl J Med. (2008) 358:453–63. doi: 10.1056/NEJMoa072972
4. Clark ME, Walker RL, Gironda RJ, Scholten JD. Comparison of pain and emotional symptoms in soldiers with polytrauma: unique aspects of blast exposure. Pain Med. (2009) 10:447–55. doi: 10.1111/j.1526-4637.2009.00590.x
5. Tanielian T, Jaycox LH. Invisible Wounds of War: Psychological and Cognitive Injuries, Their Consequences, and Services to Assist Recovery (MG-720). Santa Monica, CA: RAND Corporation (2008). p. 498.
6. Rosenfeld JV, McFarlane AC, Bragge P, Armonda RA, Grimes JB, Ling GS. Blast-related traumatic brain injury. Lancet Neurol. (2013) 12:882–93. doi: 10.1016/S1474-4422(13)70161-3
7. Kluger Y, Nimrod A, Biderman P, Mayo A, Sorkin P. The quinary pattern of blast injury. J Emergency Manage. (2006) 4:51–5. doi: 10.5055/jem.2006.0009
8. Wolf SJ, Bebarta VS, Bonnett CJ, Pons PT, Cantrill SV. Blast injuries. Lancet. (2009) 374:405–15. doi: 10.1016/S0140-6736(09)60257-9
9. Cernak I, Noble-Haeusslein LJ. Traumatic brain injury: an overview of pathobiology with emphasis on military populations. J Cereb Blood Flow Metab. (2009) 30:255–66. doi: 10.1038/jcbfm.2009.203
10. Clifford CB, Moe JB, Jaeger JJ, Hess JL. Gastrointestinal lesions in lambs due to multiple low-level blast overpressure exposure. Mil Med. (1984) 149:491–5. doi: 10.1093/milmed/149.9.491
11. Tate CM, Wang KKW, Eonta SE, Zhang Y, Carr W, Tortella FC, et al. Serum brain biomarker level, neurocognitive performance, and self-reported symptom changes in soldiers repeatedly exposed to low-level blast: a breacher pilot study. J Neurotrauma. (2013) 30:1620–30. doi: 10.1089/neu.2012.2683
12. Ling G, Bandak F, Armonda R, Grant G, Ecklund J. Explosive blast neurotrauma. J Neurotrauma. (2009) 26:815–25. doi: 10.1089/neu.2007.0484
13. Cernak I, Stein DG, Elder GA, Ahlers S, Curley K, DePalma RG, et al. Preclinical modelling of militarily relevant traumatic brain injuries: challenges and recommendations for future directions. Brain Injury. (2017) 31:1168–76. doi: 10.1080/02699052.2016.1274779
14. Nakagawa A, Manley GT, Gean AD, Ohtani K, Armonda R, Tsukamoto A, et al. Mechanisms of primary blast-induced traumatic brain injury: Insights from shock-wave research. J Neurotrauma. (2011) 28:1101–19. doi: 10.1089/neu.2010.1442
15. Bull AMJ, Clasper J, Mahoney PF. Blast Injury Science and Engineering. A Guide for Clinicians and Researchers. Cham: Springer. (2016). doi: 10.1007/978-3-319-21867-0
16. Stuhmiller JH, Phillips YY III, Richmond DR. The physics and mechanisms of primary blast injury. In: Bellamy RF, Zajtchuk R, editors. Conventional Warfare: Ballistic, Blast, and Burn Injuries. Washington, DC: Walter Reed Army Medical Center (1991). p. 241–70.
17. Wiri S, Ritter AC, Bailie JM, Needham C, Duckworth JL. Computational modeling of blast exposure associated with recoilless weapons combat training. Shock Waves. (2017) 27:849–62. doi: 10.1007/s00193-017-0755-3
18. Bass CR, Panzer MB, Rafaels KA, Wood G, Shridharani J, et al. Brain injuries from blast. Ann Biomed Eng. (2012) 40:185–202. doi: 10.1007/s10439-011-0424-0
19. Chalioulias K, Sim KT, Scott R. Retinal sequelae of primary ocular blast injuries. J R Army Med Corps. (2007) 153:124. doi: 10.1136/jramc-153-02-11
20. Morley MG, Nguyen JK, Heier JS, Shingleton BJ, Pasternak JF, Bower KS. Blast eye injuries: a review for first responders. Disaster Med Public Health Prep. (2010) 4:154–60. doi: 10.1001/dmp.v4n2.hra10003
21. Pham MT, Rajić A, Greig JD, Sargeant JM, Papadopoulos A, McEwen SA. A scoping review of scoping reviews: advancing the approach and enhancing the consistency. Res Synth Methods. (2014) 5:371–85. doi: 10.1002/jrsm.1123
22. Arksey H, O'Malley L. Scoping studies: towards a methodological framework. Int J Soc Res Methodol. (2005) 8:19–32. doi: 10.1080/1364557032000119616
23. Tricco AC, Cogo E, Ashoor H, Perrier L, McKibbon KA, Grimshaw JM, et al. Sustainability of knowledge translation interventions in healthcare decision-making: protocol for a scoping review. BMJ Open. (2013) 3:e002970. doi: 10.1136/bmjopen-2013-002970
24. Cohen J. A coefficient of agreements for nominal scales. Educ Psychol Meas. (1960) 20:37–46. doi: 10.1177/001316446002000104
25. Landis JR, Koch GG. An application of hierachical kappa type statistics in the assessment of majority agreement among multiple observers. Biometrics. (1977) 33:363–74. doi: 10.2307/2529786
26. Baker AJ, Topolovec-Vranic J, Michalak A, Pollmann-Mudryj M-A, Ouchterlony D, Cheung B, et al. Controlled blast exposure during forced explosive entry training and mild traumatic brain injury. J Trauma. (2011) 71:S472–7. doi: 10.1097/TA.0b013e318232e7da
27. Blennow K, Jonsson M, Andreasen N, Rosengren L, Wallin A, Hellström PA, et al. No neurochemical evidence of brain injury after blast overpressure by repeated explosions or firing heavy weapons. Acta Neurol Scand. (2011) 123:245–51. doi: 10.1111/j.1600-0404.2010.01408.x
28. Bonnette S, Diekfuss JA, Kiefer AW, Riley MA, Barber Foss KD, Thomas S, et al. A jugular vein compression collar prevents alterations of endogenous electrocortical dynamics following blast exposure during special weapons and tactical (SWAT) breacher training. Exp Brain Res. (2018) 236:2691–701. doi: 10.1007/s00221-018-5328-x
29. Capo-Aponte JE, Jurek GM, Walsh DV, Temme LA, Ahroon WA, Riggs DW. Effects of repetitive low-level blast exposure on visual system and ocular structures. J Rehabil Res Dev. (2015) 52:273–90. doi: 10.1682/JRRD.2014.09.0204
30. Carr W, Polejaeva E, Grome A, Crandall B, LaValle C, Eonta SE, et al. Relation of repeated low-level blast exposure with symptomology similar to concussion. J Head Trauma Rehabil. (2015) 30:47–55. doi: 10.1097/HTR.0000000000000064
31. Carr W, Stone JR, Walilko T, Young LA, Snook TL, Paggi ME, et al. Repeated low-level blast exposure: a descriptive human subjects study. Mil Med. (2016) 181:28–39. doi: 10.7205/MILMED-D-15-00137
32. Carr W, Yarnell AM, Ong R, Walilko T, Kamimori GH, da Silva U, et al. Ubiquitin carboxy-terminal hydrolase-L1 as a serum neurotrauma biomarker for exposure to occupational low-level blast. Front Neurol. (2015) 6:49. doi: 10.3389/fneur.2015.00049
33. Gill J, Cashion A, Osier N, Arcurio L, Motamedi V, Dell KC, et al. Moderate blast exposure alters gene expression and levels of amyloid precursor protein. Neurol Genet. (2017) 3:e186. doi: 10.1212/NXG.0000000000000186
34. Gill J, Motamedi V, Osier N, Dell K, Arcurio L, Carr W, et al. Moderate blast exposure results in increased IL-6 and TNFα in peripheral blood. Brain Behav Immun. (2017) 65:90–4. doi: 10.1016/j.bbi.2017.02.015
35. Kamimori GH, LaValle CR, Eonta SE, Carr W, Tate C, Wang KKW. Longitudinal investigation of neurotrauma serum biomarkers, behavioral characterization, and brain imaging in soldiers following repeated low-level blast exposure (New Zealand Breacher Study). Mil Med. (2018) 183:28–33. doi: 10.1093/milmed/usx186
36. Kamimori GH, Reilly LA, LaValle CR, Olaghere Da Silva UB. Occupational overpressure exposure of breachers and military personnel. Shock Waves. (2017) 27:837–47. doi: 10.1007/s00193-017-0738-4
37. Kubli LR, Pinto RL, Burrows HL, Littlefield PD, Brungart DS. The effects of repeated low-level blast exposure on hearing in marines. Noise Health. (2017) 19:227–38. doi: 10.4103/nah.NAH_58_16
38. Kulik A, Malinowska-Borowska J. Exposure to impulse noise at an explosives company: a case study. Int J Occup Saf Ergon. (2018) 24:366–72. doi: 10.1080/10803548.2017.1420583
39. Littlefield PD, Pinto RL, Burrows HL, Brungart DS. The vestibular effects. J Neurotrauma. (2016) 33:71–81. doi: 10.1089/neu.2014.3824
40. McBride DI, Williams S. Air blast circuit breaker noise and hearing loss: a multifactorial model for risk assessment. Occup Med. (2000) 50:173–81. doi: 10.1093/occmed/50.3.173
41. McBride DI, Williams S. Audiometric notch as a sign of noise induced hearing loss. Occup Environ Med. (2001) 58:46–51. doi: 10.1136/oem.58.1.46
42. Rhea CK, Kuznetsov NA, Ross SE, Long B, Jakiela JT, et al. Development of a portable tool for screening neuromotor sequelae from repetitive low-level blast exposure. Mil Med. (2017) 182:147–54. doi: 10.7205/MILMED-D-16-00140
43. St Onge P, McIlwain DS, Hill ME, Walilko TJ, Bardolf LB. Marine corps breacher training study: auditory and vestibular findings. US Army Med Dep J. (2011) 97–107.
44. Yuan W, Foss KDB, Dudley J, Thomas S, Galloway R, DiCesare C, et al. Impact of low-level blast exposure on brain function after a one-day tacticle training and the ameliorating effect of a jugular vein compression neck collar device. J Neurotrauma. (2019) 36:721–34. doi: 10.1089/neu.2018.5737
45. Ahlers S, Vasserman-Stokes E, Shaughness M, Hall A, Shear D, Chavko M, et al. Assessment of the effects of acute and repeated exposure to blast overpressure in rodents: toward a greater understanding of blast and the potential ramification for injury in humans exposed to blast. Front Neurol. (2012) 3:32. doi: 10.3389/fneur.2012.00032
46. Ahmed F, Plantman S, Cernak I, Agoston DV. The temporal patttern of changes in serum biomarker levels reveals complex and dynamically changing pathologies after exposure to a single low-intensity blast in mice. Front Neurol. (2015) 6:114. doi: 10.3389/fneur.2015.00114
47. Chen T, Smith K, Jiang S, Zhang T, Gan RZ. Progressive hearing damage after exposure to repeated low-intensity blasts in chinchillas. Hearing Res. (2019) 378:33–42. doi: 10.1016/j.heares.2019.01.010
48. Choi JH, Greene WA, Johnson AJ, Chavko M, Cleland JM, McCarron RM, et al. Pathophysiology of blast-induced ocular trauma in rats after repeated exposure to low-level blast overpressure. Clin Exp Ophthalmol. (2015) 43:239–46. doi: 10.1111/ceo.12407
49. Elsayed NM, Gorbunov NV. Pulmonary biochemical and histological alterations after repeated low-level blast overpressure. Toxicol Sci. (2006) 95:289–96. doi: 10.1093/toxsci/kfl138
50. Hall AA, Mendoza MI, Zhou H, Shaughness M, McCarron RM, Ahlers ST. Repeated low intensity blast exposure is associated with damaged endothelial glycocalyx and downstream behavioral deficits. Front Behav Neurosci. (2017) 11:104. doi: 10.3389/fnbeh.2017.00104
51. Li Y, Yang Z, Liu B, Valdez C, Chavko M, Cancio LC. Low-level primary blast induces neuroinflammation and neurodegeneration in rats. Mil Med. (2019) 184:265–72. doi: 10.1093/milmed/usy330
52. Lien S, Dickman JD. Vestibular injury after low-intensity blast exposure. Front Neurol. (2018) 9:297. doi: 10.3389/fneur.2018.00297
53. Park E, Eisen R, Kinio A, Baker AJ. Electrophysiological white matter dysfunction and association with neurobehavioral deficits following low-level primary blast trauma. Neurobiol Dis. (2013) 52:150–9. doi: 10.1016/j.nbd.2012.12.002
54. Perez-Garcia G, Gama Sosa MA, De Gasperi R, Lashof-Sullivan M, Maudlin-Jeronimo E, Stone JR, et al. Exposure to a predator scent induces chronic behavioral changes in rats previously exposed to low-level blast: implications for the relationship of blast-related TBI to PTSD. Front Neurol. (2016) 7:176. doi: 10.3389/fneur.2016.00176
55. Perez-Garcia G, Gama Sosa MA, De Gasperi R, Lashof-Sullivan M, Maudlin-Jeronimo E, Stone JR, et al. Chronic post-traumatic stress disorder-related traits in a rat model of low-level blast exposure. Behav Brain Res. (2018) 340:117–25. doi: 10.1016/j.bbr.2016.09.061
56. Por ED, Choi J-H, Lund BJ. Low-level blast exposure increases transient receptor potential vanilloid 1 (TRPV1) expression in the rat cornea. Curr Eye Res. (2016) 41:1294–301. doi: 10.3109/02713683.2015.1122812
57. Pun P, Kan EM, Salim A, Li ZH, Ng KC, Moochhala S, et al. Low level primary blast injury in rodent brain. Front Neurol. (2011) 2:19. doi: 10.3389/fneur.2011.00019
58. Saljo A, Arrhen F, Bolouri H, Mayorga M, Hamberger A. Neuropathology and pressure in the pig brain resulting from low-impulse noise exposure. J Neurotrauma. (2008) 25:1397–406. doi: 10.1089/neu.2008.0602
59. Säljö A, Bao F, Hamberger A, Haglid KG, Hansson H-A. Exposure to short-lasting impulse noise causes microglial and astroglial cell activation in the adult rat brain. Pathophysiology. (2001) 8:105–11. doi: 10.1016/S0928-4680(01)00067-0
60. Saljo A, Bolouri H, Mayorga M, Svensson B, Hamberger A. Low-level blast raises intracranial pressure and impairs cognitive function in rats: propylaxis with processed cereal feed. J Neurotrauma. (2010) 27:383–9. doi: 10.1089/neu.2009.1053
61. Gama Sosa MA, De Gasperi R, Perez Garcia GS, Perez GM, Searcy C, Vargas D, et al. Low-level blast exposure disrupts gliovascular and neurovascular connections and induces a chronic vascular pathology in rat brain. Acta Neuropathol Commun. (2019) 7:6. doi: 10.1186/s40478-018-0647-5
62. Gama Sosa MA, De Gasperi R, Perez Garcia GS, Sosa H, Searcy C, Vargas D, et al. Lack of chronic neuroinflammation in the absence of focal hemorrhage in a rat model of low-energy blast-induced TBI. Acta Neuropathol Commun. (2017) 5:80. doi: 10.1186/s40478-017-0483-z
63. Song H, Chen M, Chen C, Cui J, Johnson CE, Cheng J, et al. Proteomic analysis and biochemical correlates of mitochondrial dysfunction after low-intensity primary blast exposure. J Neurotrauma. (2019) 36:1591–605. doi: 10.1089/neu.2018.6114
64. Song H, Konan LM, Cui J, Johnson CE, Langenderfer M, Grant D, et al. Ultrastructural brain abnormalities and associated behavioral changes in mice after low-intensity blast exposure. Behav Brain Res. (2018) 347:148–57. doi: 10.1016/j.bbr.2018.03.007
65. VandeVord PJ, Bolander R, Sajja VSSS, Hay K, Bir CA. Mild neurotrauma indicates a range-specific pressure response to low level shock wave exposure. Ann Biomed Eng. (2012) 40:227–36. doi: 10.1007/s10439-011-0420-4
66. Zuckerman A, Ram O, Ifergane G, Matar MA, Sagi R, Ostfeld I, et al. Controlled low-pressure blast-wave exposure causes distinct behavioral and morphological responses modelling mild traumatic brain injury, post-traumatic stress disorder, and comorbid mild traumatic brain injury–post-traumatic stress disorder. J Neurotrauma. (2017) 34:145–64. doi: 10.1089/neu.2015.4310
67. Carr W, Dell KC, Yanagi MA, Hassan DM, LoPresti ML. Perspectives on repeated low-level blast and the measurement of neurotrauma in humans as an occupational exposure risk. Shock Waves. (2017) 27:829–36. doi: 10.1007/s00193-017-0766-0
68. Conley YP, Diaz-Arrastia R. Genomic links between blast exposure, brain injury, and Alzheimer disease. Neurol Genet. (2017) 3:e196. doi: 10.1212/NXG.0000000000000196
69. Dams-O'Connor K, Tsao JW. Functional decline 5 years after blast traumatic brain injury: sounding the alarm for a wave of disability? JAMA Neurol. (2017) 7:763–4. doi: 10.1001/jamaneurol.2017.0176
70. Elder GA, Stone JR, Ahlers ST. Effects of low-level blast exposure on the nervous system: is there really a controversy? Front Neurol. (2014) 5:269. doi: 10.3389/fneur.2014.00269
71. Säljö A, Mayorga M, Bolouri H, Svensson B, Hamberger A. Mechanisms and pathophysiology of the low-level blast brain injury in animal models. Neuroimage. (2011) 54:S83–8. doi: 10.1016/j.neuroimage.2010.05.050
72. Suttles ST. Potential of visual sensory screening, diagnostic evaluation, and training for treatment of postconcussive symptoms and performance enhancement for special forces qualified personnel. J Spec Oper Med. (2015) 15:54–63.
73. Weiner MW, Friedl KE, Pacifico A, Chapman JC, Jaffee MS, Little DM, et al. Military risk factors for Alzheimer's disease. Alzheimers Dement. (2013) 9:445–51. doi: 10.1016/j.jalz.2013.03.005
74. Galarneau MR, Woodruff SI, Dye JL, Mohrle CR, Wade AL. Traumatic brain injury during operation Iraqi freedom: findings from the United States Navy–marine corps combat trauma registry. J Neurosurg. (2008) 108:950–7. doi: 10.3171/JNS/2008/108/5/0950
75. Okie S. Traumatic brain injury in the war zone. N Engl J Med. (2005) 352:2043–7. doi: 10.1056/NEJMp058102
76. Warden D. Military TBI during the Iraq and Afghanistan wars. J Head Trauma Rehabil. (2006) 21:398–402. doi: 10.1097/00001199-200609000-00004
77. Jaramillo CA, Cooper DB, Wang C-P, Tate DF, Eapen York GE, Pugh MJ. Subgroups of US Iraq and Afghanistan veterans: associations with traumatic brain injury and mental health conditions. Brain Imaging Behav. (2015) 9:445–55. doi: 10.1007/s11682-015-9402-8
78. Pugh MJ, Finley EP, Wang C-P, Copeland LA, Jaramillo CA, Swan AA, et al. A retrospective cohort study of comorbidity trajectories associated with traumatic brain injury in veterans of the Iraq and Afghanistan wars. Brain Inj. (2016) 30:1481–90. doi: 10.1080/02699052.2016.1219055
79. Rogers JM, Read CA. Psychiatric comorbidity following traumatic brain injury. Brain Inj. (2007) 21:1321–33. doi: 10.1080/02699050701765700
80. Bauman RA, Ling G, Tong L, Januszkiewicz A, Agoston D, Delanerolle N, et al. An introductory characterization of a combat-casualty-care relevant swine model of closed head injury resulting from exposure to explosive blast. J Neurotrauma. (2009) 26:841–60. doi: 10.1089/neu.2008.0898
81. Simmons MM, Engel CC, Hoch E, Orr P, Anderson B, Azhar GS. Neurological Effects of Repeated Exposure to Military Occupational Levels of Blast: A Review of Scientific Literature (RR-2350-A). Santa Monica, CA: RAND Corporation. (2020). p. 64. doi: 10.7249/RR2350
82. Belanger HG, Bowling F, Yao EF. Low-level blast exposure in humans: a systematic review of acute and chronic effects. J Spec Oper Med. (2020) 20:87–93.
83. Koliatsos VE, Cernak I, Xu L, Song Y, Savonenko A, Crain BJ, et al. A mouse model of blast injury to brain: Initial pathological, neuropathological, and behavioral characterization. J Neuropathol Exp Neurol. (2011) 70:399–416. doi: 10.1097/NEN.0b013e3182189f06
84. Belding JN, Fitzmaurice S, Englert RM, Koenig HG, Thomsen CJ. Self-reported concussion symptomology during deployment: differences as a function of injury mechanism and low-level blast exposure. J Neurotrauma. (2020) 37:2219–26. doi: 10.1089/neu.2020.6997
85. Belding JN, Fitzmaurice S, Englert RM, Lee I, Kowitz B, Highfill-McRoy RM, et al. Blast exposure and risk of recurrent occupational overpressure exposure predict deployment TBIs. Mil Med. (2020) 185:e538–44. doi: 10.1093/milmed/usz289
86. Eonta SE, Kamimori GH, Wang KKW, Carr W, LaValle CR, Egnoto MJ, et al. Case study of a breacher investigation of neurotrauma biomarker levels, self-reported symptoms, and functional MRI analysis before and after exposure to measured low-level blast. Mil Med. (2019) 185:e513–7. doi: 10.1093/milmed/usz185
87. Sajja VSSS, LaValle C, Salib JE, Misistia AC, Ghebremedhin MY, Ramos AN, et al. The role of very low level blast overpressure in symptomatology. Front Neurol. (2019) 10:891. doi: 10.3389/fneur.2019.00891
88. Heyburn L, Abutarboush R, Goodrich S, Urioste R, Batuure A, Statz J, et al. Repeated low-level blast overpressure leads to endovascular disruption and alterations in TDP-43 and Piezo2 in a rat model of blast TBI. Front Neurol. (2019) 10:766. doi: 10.3389/fneur.2019.00766
89. Kelley A, Athy J, Vasbinder M, Chiaramonte J, Rath E. The Effect of Blast Exposure pn Sleep and Daytime Sleepiness in U.S. Marine Corps Breachers (USAARL 2010-16). Fort Rucker, AL: U.S. Army Aeromedical Research Laboratory (2010).
Keywords: blast, TBI, low-level blast, explosives, overpressure, military, injury, injury - head trauma
Citation: Belding JN, Englert RM, Fitzmaurice S, Jackson JR, Koenig HG, Hunter MA, Thomsen CJ and da Silva UO (2021) Potential Health and Performance Effects of High-Level and Low-Level Blast: A Scoping Review of Two Decades of Research. Front. Neurol. 12:628782. doi: 10.3389/fneur.2021.628782
Received: 12 November 2020; Accepted: 10 February 2021;
Published: 10 March 2021.
Edited by:
Ibolja Cernak, Mercer University School of Medicine, United StatesReviewed by:
Vassilis E. Koliatsos, Johns Hopkins University, United StatesLisa Anne Brenner, University of Colorado Anschutz Medical Campus, United States
Copyright © 2021 Belding, Englert, Fitzmaurice, Jackson, Koenig, Hunter, Thomsen and da Silva. This is an open-access article distributed under the terms of the Creative Commons Attribution License (CC BY). The use, distribution or reproduction in other forums is permitted, provided the original author(s) and the copyright owner(s) are credited and that the original publication in this journal is cited, in accordance with accepted academic practice. No use, distribution or reproduction is permitted which does not comply with these terms.
*Correspondence: Jennifer N. Belding, jennifer.n.belding.ctr@mail.mil