- 1Heart and Stroke Foundation Canadian Partnership for Stroke Recovery, Sunnybrook Research Institute, Toronto, ON, Canada
- 2Faculty of Kinesiology and Physical Education, University of Toronto, Toronto, ON, Canada
- 3Rehabilitation Sciences Institute, University of Toronto, Toronto, ON, Canada
- 4Sunnybrook Health Sciences Centre, Toronto, ON, Canada
- 5Department of Pharmacology, University of Toronto, Toronto, ON, Canada
- 6Lady Davis Institute, Jewish General Hospital, Montreal, QC, Canada
- 7Department of Neurology and Neurosurgery, McGill University, Montreal, QC, Canada
Background: During recovery from stroke, the contralesional motor cortex (M1) may undergo maladaptive changes that contribute to impaired interhemispheric inhibition (IHI). Transcranial direct current stimulation (tDCS) with the cathode over contralesional M1 may inhibit this maladaptive plasticity, normalize IHI, and enhance motor recovery.
Objective: The objective of this systematic review and meta-analysis was to evaluate available evidence to determine whether cathodal tDCS on contralesional M1 enhances motor re-learning or recovery post-stroke more than sham tDCS.
Methods: We searched OVID Medline, Embase, and the Cochrane Central Register of Controlled Trials for participants with stroke (>1 week post-onset) with motor impairment and who received cathodal or sham tDCS to contralesional M1 for one or more sessions. The outcomes included a change in any clinically validated assessment of physical function, activity, or participation, or a change in a movement performance variable (e.g., time, accuracy). A meta-analysis was performed by pooling five randomized controlled trials (RCTs) and comparing the change in Fugl–Meyer upper extremity scores between cathodal and sham tDCS groups.
Results: Eleven studies met the inclusion criteria. Qualitatively, four out of five cross-over design studies and three out of six RCTs reported a significant effect of cathodal vs. sham tDCS. In the quantitative synthesis, cathodal tDCS (n = 65) did not significantly reduce motor impairment compared to sham tDCS (n = 67; standardized mean difference = 0.33, z = 1.79, p = 0.07) with a little observed heterogeneity (I2 = 5%).
Conclusions: The effects of cathodal tDCS to contralesional M1 on motor recovery are small and consistent. There may be sub-populations that may respond to this approach; however, further research with larger cohorts is required.
Introduction
More than 60% of stroke survivors have persistent motor deficits for months to years after stroke (1). Innovative approaches to rehabilitation that improve motor recovery are required to reduce the burden of disability post-stroke. Transcranial direct current stimulation (tDCS) is a type of neuromodulatory non-invasive brain stimulation that shifts cortical excitability into a relative state of inhibition or excitation. The pairing of upper extremity rehabilitation therapies with tDCS has potential to facilitate recovery beyond that achieved with rehabilitation alone (2, 3). Many tDCS applications in stroke are based on a model of interhemispheric inhibition (IHI) (4, 5) thought to regulate cortical excitability between left and right motor cortex via transcallosal fibers. Specifically, the IHI model postulates that recovery is hindered because of reduced inhibition from ipsilesional motor cortex (M1) to contralesional M1. This leads to “over-active” contralesional networks that are thought to be less efficient or to even inhibit recruitment of damaged ipsilesional networks. The inhibition of contralesional M1 using tDCS with the cathode over this region may down-regulate the over-activity and promote increased functional recovery (5). Therefore, we performed a systematic review and meta-analysis to determine whether the existing evidence indicates that cathodal tDCS to contralesional M1 enhances motor performance and/or recovery after stroke.
Many studies apply cathodal tDCS to contralesional M1 with the rationale to rebalance impaired interhemispheric interactions and restore function (6). Contralesional neural activity is associated with poor motor outcome (7–13), and IHI is impaired post-stroke (14–16). However, recent discussions have questioned the validity of the impaired IHI model (17). Some studies report no evidence of over-activation in contralesional M1 or impairment in IHI post-stroke (18, 19). A one-size-fits-all model for the use of tDCS to treat stroke motor dysfunction may be insufficient (4, 18–22). For example, individuals with severe motor impairment do not improve with cathodal tDCS to contralesional M1 (23, 24) but improve when anodal tDCS is applied to contralesional M1 (21). This may be because the mechanisms mediating the recovery for individuals with severe impairment differ from those of people who are less impaired (4, 25, 26). In people with severe motor deficits, compensatory activation of contralesional M1 may support motor recovery. Similarly, the stage of stroke may also influence the functional role of the contralesional hemisphere in recovery (13).
Prior systematic reviews and meta-analyses have been published on this topic, however with conflicting conclusions, from no indication (27–30) to indication (31, 32) for clinical use. For example, a group of European experts reviewed current evidence on the therapeutic use of tDCS in stroke motor recovery and concluded based on one class I study and one class II study that there was no effect of tDCS using the cathode on contralesional M1 (30). However, meta-analyses that included more studies either found no significant effect (29) or a significant effect (32) but combined studies that administered multiple and single sessions of tDCS in their analyses. In stroke rehabilitation, individuals typically receive multiple sessions of therapy, and thus evaluating the effects of multiple tDCS sessions is relevant to determine whether these effects are cumulative and enhance efficacy. Multiple sessions of tDCS with the cathode on contralesional M1 improved activities of daily living after stroke but did not reduce motor impairment as measured by Fugl–Meyer (FM) upper extremity assessment (31). This latter study (31), along with other meta-analyses (28, 32), also estimated the effect using outcome data gathered at the end of the intervention and thus did not account for baseline characteristics that may influence the amount of behavioral change possible from an intervention.
The purpose of the systematic review and meta-analysis was to determine whether available evidence indicates that cathodal tDCS to contralesional M1 enhances motor performance or recovery post-stroke more than sham tDCS. This work is an update from prior studies (27–29, 32–34) that also report effects of cathodal vs. sham tDCS on motor performance or recovery. Our meta-analysis represents an incremental advance for the following reasons: First, we only included RCTs that involved multiple sessions of tDCS combined with an intervention, which allows us to evaluate the effects of tDCS on motor recovery. Second, we calculated the pooled effect size using a change score, which represents the difference between baseline and follow-up in the outcome measure. This mitigates issues concerning different baseline characteristics that may confound effect estimation when tDCS is only evaluated cross-sectionally across conditions (27). Third, a follow-up assessment time point was used in the calculation of the change score as opposed to an immediate post-tDCS assessment. This allows us to evaluate the effect of cathodal tDCS on the relative permanence of motor recovery. Fourth, we conducted a meta-analysis that focused on the synthesis of the FM upper extremity assessment of impairment. Ongoing discussions have highlighted the need to understand and distinguish between processes related to “true recovery” or restitution of behaviors vs. adaptation and compensation (35–38). Our meta-analysis evaluates the evidence for whether tDCS reduces motor impairment, which may imply that it involves plasticity mechanisms related to neural repair as opposed to the learning of compensatory behaviors. Our study is an advance over prior work that showed an effect of tDCS on measures of body functions/structures and activity levels of the International Classification of Functioning combined (32, 33). It also builds on a prior meta-analysis of n = 4 studies that evaluated the effect of multiple sessions of tDCS on the change in FM scores (27).
Methods
This systematic review and meta-analysis was conducted in accordance with the PRISMA statement and checklist (39).
Information Sources and Search Strategy
The literature search was performed in collaboration with a medical librarian (HL). We identified studies by systematically searching the following electronic databases, limited from the year 1990: Ovid MEDLINE(R) and Ovid OLDMEDLINE(R) <1946 to October, week 3, 2019; Embase Classic + Embase <1947 to 2019, week 43; and the Cochrane Central Register of Controlled Trials <September 2019 edition>.
We applied a broad search strategy, which we then narrowed down with specific eligibility criteria as outlined in the following “Eligibility Criteria”. We combined the following set of search terms using the Boolean operator “OR”: transcranial direct current stimulation, tDCS, electric stimulation therapy, neuro stimulation, transcranial stimulation, non-invasive brain stimulation, NIBS, direct current stimulation, brain stimulation, cortical stimulation, cranial stimulation. A second set of search terms was also combined using the Boolean operator “OR”: cerebrovascular disorders, intracranial arteriosclerosis, intracranial embolism and thrombosis, stroke, cerebrovascular accident. The Boolean operator “AND” was applied to combine the first two searches, with the limits human and English language. Furthermore, medical sub-headings for each database were used when provided (see Table 1 for the Medline database search strategy and see Supplementary Tables 1, 2 for Embase and Cochrane Central searches, respectively).
Eligibility Criteria
The eligibility criteria were specific in order to address the updates represented by this systematic review and meta-analysis in comparison to prior work reviewed in the “INTRODUCTION”. Articles retrieved from the search were included if they met the following criteria: (1) participants: individuals with hemorrhagic or ischemic stroke (no lesion location limit), >18 years of age and >7 days post-stroke (i.e., subacute and chronic stages), with motor impairment to the upper limb; (2) intervention: tDCS applied online or offline, with any motor rehabilitation intervention or motor training paradigm; the cathode electrode is placed on contralesional M1 and the anode electrode on the contralateral supraorbital area; (3) comparison: the condition of sham tDCS; (4) outcomes: change in any clinically validated assessment of physical function, activity, or participation (e.g., Fugl–Meyer, action research arm test, etc.) or change in a movement performance variable (e.g., movement time, accuracy, smoothness); (5) time: one or more sessions of the intervention; and (6) type of publication: articles published in English and peer-reviewed. The included studies were all clinical in nature.
We excluded the following: review articles, conference abstracts, and single case reports.
Study Selection, Data Extraction, and Quality Assessment
Three investigators (JLC, AS, and CPS) independently screened the titles and abstracts of all articles identified in the search and performed a full-text evaluation of the articles against the inclusion and exclusion criteria to determine eligibility. The following information was extracted for each included paper: participant characteristics, intervention program, tDCS parameters, outcome measures, and sample size. Discrepancies were resolved by consensus between the investigators.
Two investigators (JLC and JDE) independently rated the quality of evidence using the Physiotherapy Evidence Database (PEDro) (40). The PEDro scale includes 11 items, the first of which is not used to calculate the PEDro score. Scores of 9 and 10 reflect studies of excellent quality, 6–8 as good, 4 and 5 as fair, and <4 as poor (41). Cohen's kappa was performed to measure the inter-rater agreement. Discrepancies were resolved by consensus between the two investigators.
Data Synthesis and Analysis
The extracted data were summarized in a table where differences and similarities were noted. A meta-analysis was conducted using Review Manager (version 5.3). The outcome of interest was the standardized mean difference (SMD) of the “change” score on the FM upper extremity assessment between cathodal tDCS and sham tDCS. Change is defined as the difference between baseline (i.e., before tDCS) and follow-up (i.e., after tDCS). As our goal was to assess long-term changes in motor skill retention, follow-up was defined as any assessment time point that did not occur immediately after tDCS (e.g., same day after the last tDCS session). We also performed a sub-group analysis to determine if the effects of cathodal tDCS differ between people in the chronic (>6 months) vs. sub-acute (7 days to 6 months) stage of stroke. A random-effects model was used to account for differences in effect sizes between studies (42). Ninety-five percent confidence intervals (95% CI) were calculated for the overall effect. Heterogeneity was assessed with chi-square, I2, and τ2 statistics.
Results
Search Results
We identified 4,202 records using our search criteria (Figure 1). After removal of duplicates, we screened the titles and abstracts of 2,841 articles, 35 of which further underwent a full-text review. Of these, 24 articles were excluded after the full-text review (see Figure 1 for details). Eleven articles met the inclusion and exclusion criteria and were included in the systematic review (43–53); five of these studies were included in the meta-analysis (45, 47, 48, 50, 53).
Characteristics of Studies
Quality
There was “substantial agreement” (54) between the two raters' initial PEDro scores (κ = 0.612, p < 0.001). Out of 121 ratings (11 items by 11 studies), the two raters disagreed on 14 items. These disagreements were resolved by discussion, and the final results of the PEDro quality assessment are shown in Table 2. The scores ranged from 6 to 10. Six studies are considered excellent in quality (PEDro score 9 and 10), and five studies are considered good in quality (PEDro score 6–8). There were only two PEDro items for which few studies scored points on: (1) concealed allocation (item 3) of participants occurred in five studies and (2) therapist blinding (item 6) occurred in six studies.
Participants
A total of 289 participants with stroke who met study the inclusion and exclusion criteria were included in the analyses (see Table 3 for information on the participants' characteristics). Across studies, the average age of the participants ranged between 50 and 69.9 years. There were 169 male (64%) and 96 female (36%) participants; one study did not report sex. Information about gender was not reported in any study. One hundred one participants (96%) were reported as right-handed, four participants (4%) were left-handed, and three studies did not report handedness.
Four studies tested participants in the early sub-acute stage (i.e., 7 days to 3 months post-stroke), one study tested participants spanning the late sub-acute (3 to 6 months) and chronic stages (>6 months post-stroke), and six studies tested participants in the chronic stage (>6 months post-stroke). Two hundred forty-five participants (95%) had an ischemic stroke, and 13 participants (5%) had a hemorrhagic stroke; three studies did not report stroke sub-type. Stroke lesions were in the right hemisphere in 148 participants (51%) and in the left hemisphere in 141 participants (49%). Stroke lesions were cortical in 117 participants (44%), subcortical in 105 participants (39%), and both cortical and subcortical in 46 (17%) participants; one study did not report lesion location. Individuals with mild, moderate, and severe strokes were tested across the studies. Seven studies assessed baseline severity using the FM assessment: one study tested severely affected individuals (average FM scores <10) (45), one study tested people with mild impairment (average FM score >60) (52), and the other studies tested people with scores in between.
Nine studies reported whether the participants experienced adverse events (AE). Of these studies, two reported the presence of AEs, and the participants withdrew because of headache (n = 2) (44, 47) and dizziness (n = 1) (47). Two studies did not mention whether AEs were experienced (48, 51).
Study Interventions
Table 4 presents information on study characteristics and results.
Study design
There were five studies that employed a cross-over design to evaluate the effects of different types of tDCS on the same participant (i.e., sham vs. cathode and/or anode montages). Four of these studies probed the effects of a single tDCS session, and one study tested the effects of four sessions. The number of participants tested in these studies ranged from four to 24, two studies tested six or less participants, two studies tested 12 to 13 participants, and one study tested 24 participants.
There were six randomized controlled trials (RCTs). Five studies evaluated the effects of five to 12 sessions of tDCS, three studies tested <10 participants per group, and two studies tested 13 to 14 participants per group. One study evaluated the effects of 30 sessions of tDCS on 32 participants per group.
Behavioral paradigm
Five studies that employed a cross-over design asked the participants to perform motor tasks ranging from the Jebsen Taylor Hand Function Test, motor (finger) sequence learning, hand movement in response to cues, and grip force. Of the six RCTs, four employed physical or occupational therapy, one implemented robotics, and one implemented constraint-induced movement therapy.
tDCS parameters
Stimulation intensity was either 1 mA (eight studies) or 2 mA (three studies). Stimulation duration ranged from 20 min (seven studies), 25 min (two studies), or 30 min (one study). One study applied 8 min of stimulation with the cathodal on M1 and 13 min of stimulation with the anode on M1. The electrode size applied was either 25 cm2 (three studies) or 35 cm2 (eight studies).
Nine studies applied tDCS online, concurrent with the behavioral paradigm, and two RCTs applied tDCS offline before the therapy.
Outcome Measures and Outcomes
Studies that employed a cross-over design either administered a clinical test of motor activity, The Jebsen Taylor Hand Function Test (three studies), or study specific measures of response times and accuracy (two studies). In four studies, the outcomes were assessed before and after the tDCS intervention; only one study tested skill retention 24 h post-intervention. Four studies reported a significant effect for cathodal compared to sham tDCS (43, 44, 46, 52). While one study showed a significant difference between cathodal and sham tDCS in the percent response time change from baseline, there was, in fact, no difference in response times between baseline and post-testing for the cathodal tDCS condition (51).
Five of the included RCTs administered the FM assessment of motor impairment, and one trial evaluated several outcomes including the NIHSS and Barthel Index. All RCTs included a follow-up testing session, which ranged from 7 days to 6 months post-intervention. Three of the six RCTs report a significant effect for cathodal compared to sham tDCS (47, 48, 53).
Meta-analysis
We conducted a meta-analysis of RCTs that reported the FM assessment of motor impairment as the primary outcome (n = 5) and compared the effects of cathodal to sham tDCS over multiple days. In one trial, the sham stimulation group comprised of participants who underwent sham tDCS (n = 5) or sham cTBS (n = 8) (49). The follow-up time point ranged from 7 days to 6 months. Three trials tested people with chronic stroke, and two tested people in the early sub-acute stage.
The test for subgroup differences indicated no statistically significant subgroup effect (p = 0.12), suggesting that stage of stroke did not modify the effect of cathodal tDCS in comparison to sham tDCS. The pooled effect estimate favored cathodal tDCS in participants with chronic stroke: cathodal tDCS (n = 19) significantly reduced motor impairment compared to sham tDCS (n = 21) (SMD = 0.77, 95% CI = 0.11 to 1.44, p = 0.02, Figure 2). There was evidence for low heterogeneity (χ2 = 1.74, p = 0.42; I2 = 0%; τ2 = 0.00) across trials. In people in the early sub-acute stroke stage, the effect of cathodal tDCS (n = 46) was not significantly different from the effect of sham tDCS (n = 46) (SMD = 0.15, 95% CI = −0.26 to 0.56, p = 0.48, Figure 2). There was evidence for low heterogeneity (χ2 = 0.02, p = 0.88; I2 = 0%; τ2 = 0.00) across trials.
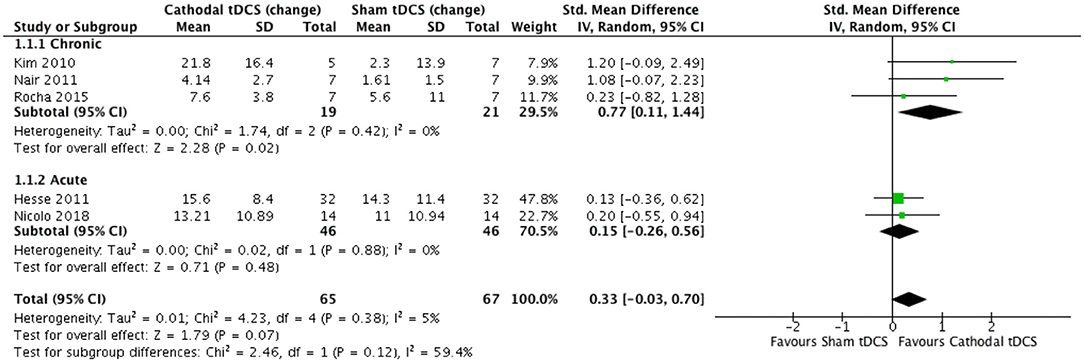
Figure 2. Effect (standardized mean difference) of cathodal transcranial direct current stimulation (tDCS) vs. sham tDCS on the change in Fugl–Meyer upper extremity score.
Combining data from both chronic and early sub-acute stages of stroke, cathodal tDCS (n = 65) did not significantly reduce motor impairment compared to sham tDCS (n = 67) (SMD = 0.33, 95% CI = −0.03 to 0.70, p = 0.07, Figure 2). There was evidence for low heterogeneity (χ2 = 4.23, p = 0.38; I2 = 5%; τ2 = 0.01) across trials. Bias was not formally tested given that there were fewer than 10 studies in the meta-analysis (55).
Discussion
The aim of this systematic review and meta-analysis was to evaluate the evidence for an effect of cathodal tDCS to contralesional M1 relative to sham tDCS in people with stroke motor impairment. The studies evaluated in this systematic review were considered good to excellent in quality. We pooled data from five RCTs to test the effects of multiple tDCS sessions on upper limb motor impairment as measured by the FM assessment. The effect of cathodal tDCS to contralesional M1, relative to sham tDCS, was small to medium (SMD = 0.33) and did not significantly reduce motor impairment (p = 0.07). The heterogeneity across studies was low, and the effect was also not different between people in the early sub-acute vs. chronic phase of stroke. We conclude that, while the effects were small, they were consistent and thus could be clinically meaningful in certain sub-populations. Studies with larger sample sizes selected based on biomarkers may help identify responders from non-responders.
The findings from our meta-analysis are comparable to the effects previously reported that also evaluated the change score. Marquez et al. reported an SMD of 0.39, p = 0.08; they pooled seven studies in their meta-analysis, three of which tested the effects of multiple tDCS sessions (29). Chhatbar et al. reported an SMD of 0.43, p = 0.2, pooling data from four studies testing multiple tDCS sessions (27). Three studies (45, 47, 48) were common across these meta-analyses, and we included an additional two published more recently (49, 50). Taken together, there is modest evidence to support the use of cathodal tDCS to contralesional M1 in reducing motor impairment. To better understand the nature of these improvements at the impairment level, future studies could report changes for each sub-section of the FM upper extremity assessment and/or obtain kinematic measures that enable the precise quantification of movement patterns (38, 56).
We now consider factors that may explain the small-to-medium effect sizes observed with tDCS, which may help us to better target future research studies in this area. First, the notion of an impaired IHI that hinders motor recovery may not apply to all stroke survivors (17). A one-size-fits-all approach of cathodal tDCS to contralesional M1 may lead to a small and non-significant effect since not every individual can benefit. Supporting evidence comes from studies included in our systematic review and meta-analysis. Zimmerman et al. tested well-recovered individuals and found enhanced motor sequence performance that was maintained 24 h after practice (52). In contrast, two RCTs that tested individuals with severe motor impairment (45, 49) showed no difference in motor outcomes between people who received cathodal vs. sham tDCS. RCTs that demonstrated significant findings with large effect sizes tested people who were less impaired (47, 48). Thus, while the findings from our meta-analysis were not significant (p = 0.07), it may be that future studies with larger samples and a set of stratification variables to derive sub-groups are required. Refined participant selection criteria may identify individuals with the greatest capacity to benefit for this intervention (57). Our ongoing work (ClinicalTrials.gov ID: NCT02473549) evaluates whether the amount of damage to the corticospinal tract predicts response to tDCS on contralesional M1. Participant selection based on validated biomarkers may also allow for a way to reduce variance and increase statistical power (58).
A second reason for a small-to-medium effect size may be that the dose of tDCS applied is insufficient. Many studies, including those in this systematic review and meta-analysis, typically employ 1-mA current for 20 min applied over an electrode area of 35 cm2. There is a positive dose–response relationship whereby the application of a higher current density is associated with greater motor recovery (27). Higher current density is also associated with less variability in corticospinal excitability (59). Therefore, higher current densities may be required to sufficiently engage the targeted area for each individual. This may be especially relevant given the inter-individual variability related to, for example, anatomy and lesion characteristics (60). To control for this variability, computational modeling of current flow through the brain may also permit researchers to individualize dose and spatial targeting (61).
One unanticipated observation was that some studies did not directly test whether the effects of tDCS are long lasting. This is important to incorporate for future research because we are concerned if the skills practiced lead to permanent improvements (62) as evaluated by testing motor performance after some delay from the practice period. All RCTs did evaluate motor performance at a delayed follow-up period. However, of the five studies using a cross-over design, only one study evaluated retention, tested 24 h later, and showed that performance was better for the cathodal vs. sham tDCS groups (52). Three studies report immediate improvement on motor performance after cathodal relative to sham tDCS; however, since retention was not tested, one cannot infer if these improvements would have been maintained.
Future research may also need to consider the psychometric properties of assessments used to evaluate improvement from tDCS. The FM upper extremity assessment has excellent psychometric properties (63), is commonly used in clinical practice, and is highly recommended for research practice (38). The assessment demonstrates longitudinal stability in terms of item difficulty order and is thus valid for measuring change in motor state over time (64). However, it has floor and ceiling effects (63), and items are not linearly related as a gain of points at the bottom vs. the top end has a different meaning (65). Therefore, future studies that use the FM should take these factors into account. Some propose using a sliding dichotomous outcome or responder analysis, whereby subgroups are specified before the trial, and response to therapy is defined differently for each subgroup (65). Another approach is to implement a Rasch-rescaled version of the assessment (66).
Another noteworthy observation was that more males (64%) than females (36%) participated in the research studies, which limits the generalizability of findings. Future work should consider sex as a variable of interest to determine if there are biological differences in response to tDCS for stroke motor recovery. To better understand this potential bias, one can also explore social–pragmatic barriers to research participation (67) that may differentially affect females. Otherwise, the characteristics of the participants studied in this review are mostly consistent with those of the general population of people with stroke. The average age ranged from 50 to 70 years, with most participants being right-hand dominant (95%), having had an ischemic stroke (94%), with equal representation of right (49%) or left (51%) hemispheres affected, and with cortical (42%) vs. subcortical (42%) lesions.
The stage of stroke recovery may be an important moderator variable to further explore in future research that targets tDCS to contralesional M1. Corticospinal and intracortical excitability changes occur as a function of time since stroke, which may reflect how motor output is generated (68). Few studies have evaluated excitability changes longitudinally in the same individuals. One study found that cortical M1 excitability in the lesioned hemisphere correlates with assessments of motor activity in the acute stage, but only weakly at 3 and 6 months post-stroke (69). In contrast, intracortical M1 excitability in the unaffected hemisphere correlated strongly with assessments of motor activity at 3 months but not in the acute stage (69). These findings align with those from another longitudinal study showing a time-dependent role of the contralesional M1: activity in contralesional M1 negatively affects motor performance during the early sub-acute phase (1 and 2 weeks) but not in the late sub-acute phase (>3 months) (13). A prior meta-analysis also found that cathodal tDCS improved motor outcomes in people with chronic but not acute or sub-acute stroke (32). However, they evaluated data at the post-test/intervention time point and combined findings from cross-sectional studies and RCTs. Our sub-group analysis suggests that the effect does not differ between individuals in the sub-acute vs. chronic stage of stroke. A caveat with our sub-analysis is that a small number of trials and participants contributed data such that the analysis may not be able to detect subgroup differences.
One major limitation of prior work included in this systematic review with meta-analysis is that many studies tested a small number of participants. Of the five studies that employed a cross-over design, four studies tested 13 or less participants. Similarly, five out of six RCTs tested 14 participants or less per group. Studies in the meta-analysis that reported a significant effect of cathodal vs. sham tDCS tested seven participants or less per group (47, 48), and thus this evidence may be unreliable (70). Based on our findings where there may be a small-to-medium effect in the direction favoring cathodal tDCS, to detect an effect size of 0.33 (α = 0.05, β = 0.80, one-sided independent t-test), a sample size of 115 participants per group is required. This supports recommendations for large RCTs in this field (2). The sample size could also be reduced with better selection of participants using biomarkers (58).
Conclusions
Cathodal tDCS to contralesional M1 did not significantly (p = 0.07) improve motor outcomes relative to sham tDCS. The effect size is small to medium (SMD = 0.33), consistent as very little heterogeneity was observed, and aligns with values reported for other meta-analyses using similar data (27, 29). Taken together, cathodal tDCS to contralesional M1 could still be of clinical significance for subpopulations to be identified in future research with larger samples. To maximally harness the effects of tDCS, future research may need to (1) personalize tDCS using evidence-based rationale for how it may facilitate motor recovery from a neurophysiological perspective and (2) be statistically powered to detect an effect.
Data Availability Statement
The original contributions presented in the study are included in the article/Supplementary Material, further inquiries can be directed to the corresponding author.
Author Contributions
JC, WS, AT, and JE designed the study. JC, AS, CS, and HL designed and performed the search. JC, AS, and CS performed data collection. JC, CS, and JE performed data analysis. JC wrote the manuscript. JC, AS, CS, HL, WS, AT, and JE edited the manuscript. All authors contributed to the article and approved the submitted version.
Funding
JC was supported by the Heart and Stroke Foundation Canadian Partnership for Stroke Recovery.
Conflict of Interest
The authors declare that the research was conducted in the absence of any commercial or financial relationships that could be construed as a potential conflict of interest.
Supplementary Material
The Supplementary Material for this article can be found online at: https://www.frontiersin.org/articles/10.3389/fneur.2021.626021/full#supplementary-material
References
1. Kwakkel G, Kollen BJ, van der Grond J, Prevo AJH. Probability of regaining dexterity in the flaccid upper limb: impact of severity of paresis and time since onset in acute stroke. Stroke. (2003) 34:2181–6. doi: 10.1161/01.STR.0000087172.16305CD
2. Grefkes C, Fink GR. Noninvasive brain stimulation after stroke: it is time for large randomized controlled trials!. Curr Opin Neurol. (2016) 29:714–20. doi: 10.1097/WCO0000000000000395
3. Koch PJ, Hummel FC. Toward precision medicine: tailoring interventional strategies based on noninvasive brain stimulation for motor recovery after stroke. Curr Opin Neurol. (2017) 30:388–97. doi: 10.1097/WCO0000000000000462
4. Pino Di G, Pellegrino G, Assenza G, Capone F, Ferreri F, Formica D, et al. Modulation of brain plasticity in stroke: a novel model for neurorehabilitation. Nat Rev Neurol. (2014) 10:597–608. doi: 10.1038/nrneurol.2014162
5. Nowak DA, Grefkes C, Ameli M, Fink GR. Interhemispheric competition after stroke: brain stimulation to enhance recovery of function of the affected hand. Neurorehabil Neural Repair. (2009) 23:641–56. doi: 10.1177/1545968309336661
6. Lefaucheur JP. A comprehensive database of published tDCS clinical trials (2005-2016). Neurophysiologie Clinique. (2016) 46:319–98. doi: 10.1016/j.neucli.2016.10002
7. Marshall RS, Perera GM, Lazar RM, Krakauer JW, Constantine RC, DeLaPaz RL. Evolution of cortical activation during recovery from corticospinal tract infarction. Stroke. (2000) 31:656–61. doi: 10.1161/01.STR.31.3656
8. Nowak DA, Grefkes C, Dafotakis M, Eickhoff S, Küst J, Karbe H, et al. Effects of low-frequency repetitive transcranial magnetic stimulation of the contralesional primary motor cortex on movement kinematics and neural activity in subcortical stroke. Arch Neurol. (2008) 65:741–7. doi: 10.1001/archneur.65.6741
9. Loubinoux I, Carel C, Pariente J, Dechaumont S, Albucher JF, Marque P, et al. Correlation between cerebral reorganization and motor recovery after subcortical infarcts. Neuroimage. (2003) 20:2166–80. doi: 10.1016/j.neuroimage.2003.08017
10. Ward NS, Newton JM, Swayne OBC, Lee L, Thompson AJ, Greenwood RJ, et al. Motor system activation after subcortical stroke depends on corticospinal system integrity. Brain. (2006) 129:809–19. doi: 10.1093/brain/awl002
11. Rehme AK, Fink GR, Von Cramon DY, Grefkes C. The role of the contralesional motor cortex for motor recovery in the early days after stroke assessed with longitudinal fMRI. Cereb Cortex. (2011) 21:756–68. doi: 10.1093/cercor/bhq140
12. Grefkes C, Nowak DA, Eickhoff SB, Dafotakis M, Küst J, Karbe H, et al. Cortical connectivity after subcortical stroke assessed with functional magnetic resonance imaging. Ann Neurol. (2008) 63:236–46. doi: 10.1002/ana21228
13. Volz LJ, Vollmer M, Michely J, Fink GR, Rothwell JC, Grefkes C. Time-dependent functional role of the contralesional motor cortex after stroke. NeuroImage Clin. (2017) 16:165–74. doi: 10.1016/j.nicl.2017.07024
14. Murase N, Duque J, Mazzocchio R, Cohen LG. Influence of Interhemispheric Interactions on Motor Function in Chronic Stroke. Ann Neurol. (2004) 55:400–9. doi: 10.1002/ana10848
15. Duque J, Hummel F, Celnik P, Murase N, Mazzocchio R, Cohen LG. Transcallosal inhibition in chronic subcortical stroke. Neuroimage. (2005) 28:940–6. doi: 10.1016/j.neuroimage.2005.06033
16. Bütefisch CM, Weβling M, Netz J, Seitz RJ, Hömberg V. Relationship Between Interhemispheric Inhibition and Motor Cortex Excitability in Subacute Stroke Patients. Neurorehabil Neural Repair. (2008) 22:4–21. doi: 10.1177/1545968307301769
17. Xu J, Branscheidt M, Schambra H, Steiner L, Widmer M, Diedrichsen J, et al. Rethinking interhemispheric imbalance as a target for stroke neurorehabilitation. Ann Neurol. (2019) 85:502–13. doi: 10.1002/ana25452
18. McDonnell MN, Stinear CM. TMS measures of motor cortex function after stroke: A meta-analysis. Brain Stimul. (2017) 10:721–34. doi: 10.1016/j.brs.2017.03008
19. Stinear CM, Petoe MA, Byblow WD. Primary motor cortex excitability during recovery after stroke: implications for neuromodulation. Brain Stimul. (2015) 8:1183–90. doi: 10.1016/j.brs.2015.06015
20. Boddington JL, Reynolds JNJ. Targeting interhemispheric inhibition with neuromodulation to enhance stroke rehabilitation. Brain Stimulation. (2017) 10:214–22. doi: 10.1016/j.brs.2017.01006
21. McCambridge AB, Stinear JW, Byblow WD. Revisiting interhemispheric imbalance in chronic stroke: a tDCS study. Clin Neurophysiol. (2018) 129:42–50. doi: 10.1016/j.clinph.2017.10016
22. Plow EB, Sankarasubramanian V, Cunningham DA, Potter-Baker K, Varnerin N, Cohen LG, et al. Models to tailor brain stimulation therapies in stroke. Neural Plast. (2016) 2016:1–17. doi: 10.1155/2016/4071620
23. Yao J, Drogos J, Veltink F, Anderson C, Concha Urday Zaa J, Hanson LI, et al. The effect of transcranial direct current stimulation on the expression of the flexor synergy in the paretic arm in chronic stroke is dependent on shoulder abduction loading. Front Hum Neurosci. (2015) 9. doi: 10.3389/fnhum.201500262
24. Bradnam L. V, Stinear CM, Barber PA, Byblow WD. Contralesional hemisphere control of the proximal paretic upper limb following stroke. Cereb Cortex. (2012) 22:2662–71. doi: 10.1093/cercor/bhr344
25. Bradnam L. V, Stinear CM, Byblow WD. Ipsilateral motor pathways after stroke: implications for non-invasive brain stimulation. Front Hum Neurosci. (2013) 7:184. doi: 10.3389/fnhum.201300184
26. Harris-Love ML, Harrington RM. Non-invasive brain stimulation to enhance upper limb motor practice poststroke: a model for selection of cortical site. Front Neurol. (2017) 8. doi: 10.3389/fneur.201700224
27. Chhatbar PY, Ramakrishnan V, Kautz S, George MS, Adams RJ, Feng W. Transcranial direct current stimulation post-stroke upper extremity motor recovery studies exhibit a dose–response relationship. Brain Stimul. (2016) 9:16–26. doi: 10.1016/j.brs.2015.09002
28. Tedesco Triccas L, Burridge JH, Hughes AM, Pickering RM, Desikan M, Rothwell JC, et al. Multiple sessions of transcranial direct current stimulation and upper extremity rehabilitation in stroke: a review and meta-analysis. Clin Neurophysiol. (2016) 127:946–55. doi: 10.1016/j.clinph.2015.04067
29. Marquez J, van Vliet P, McElduff P, Lagopoulos J, Parsons M. Transcranial Direct Current Stimulation (tDCS): does it have merit in stroke rehabilitation? A systematic review. Int J Stroke. (2015) 10:306–16. doi: 10.1111/ijs12169
30. Lefaucheur J-P, Antal A, Ayache SS, Benninger DH, Brunelin J, Cogiamanian F, et al. Evidence-based guidelines on the therapeutic use of transcranial direct current stimulation (tDCS). Clin Neurophysiol. (2017) 128:56–92. doi: 10.1016/j.clinph.2016.10087
31. Elsner B, Kwakkel G, Kugler J, Mehrholz J. Transcranial direct current stimulation (tDCS) for improving capacity in activities and arm function after stroke: a network meta-analysis of randomised controlled trials. J Neuroeng Rehabil. (2017) 14:95. doi: 10.1186/s12984-017-0301-7
32. Kang N, Weingart A, Cauraugh JH. Transcranial direct current stimulation and suppression of contralesional primary motor cortex post-stroke: a systematic review and meta-analysis. Brain Inj. (2018) 32:1063–70. doi: 10.1080/02699052.20181481526
33. Kang N, Summers JJ, Cauraugh JH. Transcranial direct current stimulation facilitates motor learning post-stroke: a systematic review and meta-analysis. J Neurol Neurosurg Psychiatry. (2016) 87:345–55. doi: 10.1136/jnnp-2015-311242
34. Elsner B, Kugler J, Pohl M, Mehrholz J. Transcranial direct current stimulation (tDCS) for improving activities of daily living, and physical and cognitive functioning, in people after stroke. Cochrane Database Syst Rev. (2016) 3:CD009645. doi: 10.1002/14651858.CD009645pub3
35. Levin MF, Kleim JA, Wolf SL. What do motor “recovery” and “compensation” mean in patients following stroke? Neurorehabil Neural Repair. (2009) 23:313–9. doi: 10.1177/1545968308328727
36. Krakauer JW, Carmichael ST, Corbett D, Wittenberg GF. Getting neurorehabilitation right: what can be learned from animal models? Neurorehabil Neural Repair. (2012) 26:923–31. doi: 10.1177/1545968312440745
37. Bernhardt J, Hayward KS, Kwakkel G, Ward NS, Wolf SL, Borschmann K, et al. Agreed definitions and a shared vision for new standards in stroke recovery research: the stroke recovery and rehabilitation roundtable taskforce. Int J Stroke. (2017) 12:444–50. doi: 10.1177/1747493017711816
38. Kwakkel G, Lannin NA, Borschmann K, English C, Ali M, Churilov L, et al. Standardized measurement of sensorimotor recovery in stroke trials: consensus-based core recommendations from the Stroke Recovery and Rehabilitation Roundtable. Int J Stroke. (2017) 12:451–61. doi: 10.1177/1747493017711813
39. Liberati A, Altman DG, Tetzlaff J, Mulrow C, Gøtzsche PC, Ioannidis JPA, et al. The PRISMA statement for reporting systematic reviews and meta-analyses of studies that evaluate health care interventions: explanation and elaboration. PLoS Med. (2009) 6:e1000100. doi: 10.1371/journal.pmed1000100
40. PEDro, S,.cale. Available onlin at: https://www.pedro.org.au/english/downloads/pedro-scale/ (accessed June 10, 2020).
41. Foley NC, Teasell RW, Bhogal SK, Speechley MR. Stroke rehabilitation evidence-based review: methodology. Top Stroke Rehabil. (2003) 10:1–7. doi: 10.1310/Y6TG-1KQ9-LEDQ-64L8
42. Borenstein M, Hedges L, Higgins J, Rothstein H. Fixed-effect versus random-effect models. In: Introduction to Meta-Analyses. 1st ed. West Sussex: John Wiley & Sons, Ltd. (2009). p. 77–85. doi: 10.1002/jrsm12
43. Boggio PS, Nunes A, Rigonatti SP, Nitsche MA, Pascual-Leone A, Fregni F. Repeated sessions of noninvasive brain DC stimulation is associated with motor function improvement in stroke patients. Restor Neurol Neurosci. (2007) 25:123–9.
44. Fleming MK, Rothwell JC, Sztriha L, Teo JT, Newham DJ. The effect of transcranial direct current stimulation on motor sequence learning and upper limb function after stroke. Clin Neurophysiol. (2017) 128:1389–98. doi: 10.1016/j.clinph.2017.03036
45. Hesse S, Waldner A, Mehrholz J, Tomelleri C, Pohl M, Werner C. Combined transcranial direct current stimulation and robot-assisted arm training in subacute stroke patients. Neurorehabil Neural Repair. (2011) 25:838–46. doi: 10.1177/1545968311413906
46. Fregni F, Boggio PS, Mansur CG, Wagner T, Ferreira MJL, Lima MC, et al. Transcranial direct current stimulation of the unaffected hemisphere in stroke patients. Neuroreport. (2005) 16:1551–5. doi: 10.1097/01.wnr.0000177010.446025e
47. Kim DY, Lim JY, Kang EK, You DS, Oh MK, Oh BM, et al. Effect of transcranial direct current stimulation on motor recovery in patients with subacute stroke. Am J Phys Med Rehabil. (2010) 89:879–86. doi: 10.1097/PHM0b013e3181f70aa7
48. Nair DG, Renga V, Lindenberg R, Zhu L, Schlaug G. Optimizing recovery potential through simultaneous occupational therapy and non-invasive brain-stimulation using tDCS. Restor Neurol Neurosci. (2011) 29:411–20. doi: 10.3233/RNN-2011-0612
49. Nicolo P, Magnin C, Pedrazzini E, Plomp G, Mottaz A, Schnider A, et al. Comparison of neuroplastic responses to cathodal transcranial direct current stimulation and continuous theta burst stimulation in subacute stroke. Arch Phys Med Rehabil. (2018) 99:862–72.e1. doi: 10.1016/j.apmr.2017.10026
50. Rocha S, Silva E, Foerster Á, Wiesiolek C, Chagas AP, Machado G, et al. The impact of transcranial direct current stimulation (tDCS) combined with modified constraint-induced movement therapy (mCIMT) on upper limb function in chronic stroke: a double-blind randomized controlled trial. Disabil Rehabil. (2015) 38:653–60. doi: 10.3109/09638288.20151055382
51. Stagg CJ, Bachtiar V, O'Shea J, Allman C, Bosnell RA, Kischka U, et al. Cortical activation changes underlying stimulation-induced behavioural gains in chronic stroke. Brain. (2012) 135(Pt 1):276–84. doi: 10.1093/brain/awr313
52. Zimerman M, Heise KF, Hoppe J, Cohen LG, Gerloff C, Hummel FC. Modulation of training by single-session transcranial direct current stimulation to the intact motor cortex enhances motor skill acquisition of the paretic hand. Stroke. (2012) 43:2185–91. doi: 10.1161/STROKEAHA.111645382
53. Khedr EM, Shawky O. a, El-Hammady DH, Rothwell JC, Darwish ES, Mostafa OM, et al. Effect of anodal versus cathodal transcranial direct current stimulation on stroke rehabilitation: a pilot randomized controlled trial. Neurorehabil Neural Repair. (2013) 27:592–601. doi: 10.1177/1545968313484808
54. Landis JR, Koch GG. The measurement of observer agreement for categorical data. Biometrics. (1977) 33:159–74. doi: 10.2307/2529310
55. Sterne JAC, Sutton AJ, Ioannidis JPA, Terrin N, Jones DR, Lau J, et al. Recommendations for examining and interpreting funnel plot asymmetry in meta-analyses of randomised controlled trials. BMJ. (2011) 343:d4002. doi: 10.1136/bmjd4002
56. Kwakkel G, van Wegen EEH, Burridge JH, Winstein CJ, van Dokkum LEH, Alt Murphy M, et al. Standardized measurement of quality of upper limb movement after stroke: consensus-based core recommendations from the second stroke recovery and rehabilitation roundtable. Neurorehabil Neural Repair. (2019) 33:951–8. doi: 10.1177/1545968319886477
57. Sankarasubramanian V, Machado AG, Conforto AB, Potter-Baker KA, Cunningham DA, Varnerin NM, et al. Inhibition versus facilitation of contralesional motor cortices in stroke: deriving a model to tailor brain stimulation. Clin Neurophysiol. (2017) 128:892–902. doi: 10.1016/j.clinph.2017.03030
58. Boyd LA, Hayward KS, Ward NS, Stinear CM, Rosso C, Fisher RJ, et al. Biomarkers of stroke recovery: consensus-based core recommendations from the stroke recovery and rehabilitation roundtable. Int J Stroke. (2017) 12:480–93. doi: 10.1177/1747493017714176
59. Ammann C, Lindquist MA, Celnik PA. Response variability of different anodal transcranial direct current stimulation intensities across multiple sessions. Brain Stimul. (2017) 10:757–63. doi: 10.1016/j.brs.2017.04003
60. LM Li, Uehara K, Hanakawa T. The contribution of interindividual factors to variability of response in transcranial direct current stimulation studies. Front Cell Neurosci. (2015) 9. doi: 10.3389/fncel.201500181
61. Bikson M, Brunoni AR, Charvet LE, Clark VP, Cohen LG, Deng Z-D, et al. Rigor and reproducibility in research with transcranial electrical stimulation: an NIMH-sponsored workshop. Brain Stimul. (2018) 11:465–80. doi: 10.1016/j.brs.2017.12008
62. Kantak SS, Winstein CJ. Learning-performance distinction and memory processes for motor skills: a focused review and perspective. Behav Brain Res. (2012) 228:219–31. doi: 10.1016/j.bbr.2011.11028
63. Gladstone DJ, Danells CJ, Black SE. The fugl-meyer assessment of motor recovery after stroke: a critical review of its measurement properties. Neurorehabil Neural Repair. (2002) 16:232–40. doi: 10.1177/154596802401105171
64. Woodbury ML, Velozo CA, Richards LG, Duncan PW, Studenski S, Lai S-M. Longitudinal stability of the fugl-meyer assessment of the upper extremity. Arch Phys Med Rehabil. (2008) 89:1563–9. doi: 10.1016/j.apmr.2007.12041
65. See J, Dodakian L, Chou C, Chan V, McKenzie A, Reinkensmeyer DJ, et al. A Standardized approach to the fugl-meyer assessment and its implications for clinical trials. Neurorehabil Neural Repair. (2013) 27:732–41. doi: 10.1177/1545968313491000
66. Geed S, Lane CJ, Nelsen MA, Wolf SL, Winstein CJ, Dromerick AW. Inaccurate use of the upper extremity fugl-meyer negatively affects upper extremity rehabilitation trial design: findings from the ICARE randomized controlled trial. Arch Phys Med Rehabil. (2021) 102:270–9. doi: 10.1016/j.apmr.2020.08019
67. Cramer SC, Wolf SL, Adams HP, Chen D, Dromerick AW, Dunning K, et al. Stroke recovery and rehabilitation research. Stroke. (2017) 48:813–9. doi: 10.1161/STROKEAHA.116015501
68. Sebastianelli L, Versace V, Martignago S, Brigo F, Trinka E, Saltuari L, et al. Low-frequency rTMS of the unaffected hemisphere in stroke patients: a systematic review. Acta Neurol Scand. (2017) 136:585–605. doi: 10.1111/ane12773
69. Swayne OBC, Rothwell JC, Ward NS, Greenwood RJ. Stages of motor output reorganization after hemispheric stroke suggested by longitudinal studies of cortical physiology. Cereb Cortex. (2008) 18:1909–22. doi: 10.1093/cercor/bhm218
Keywords: stroke, transcranial direct current stimulation, upper limb, motor recovery, systematic review and meta-analysis
Citation: Chen JL, Schipani A, Schuch CP, Lam H, Swardfager W, Thiel A and Edwards JD (2021) Does Cathodal vs. Sham Transcranial Direct Current Stimulation Over Contralesional Motor Cortex Enhance Upper Limb Motor Recovery Post-stroke? A Systematic Review and Meta-analysis. Front. Neurol. 12:626021. doi: 10.3389/fneur.2021.626021
Received: 04 November 2020; Accepted: 03 March 2021;
Published: 15 April 2021.
Edited by:
Ina M. Tarkka, University of Jyväskylä, FinlandReviewed by:
Luca Sebastianelli, Hospital of Vipiteno, ItalyKeith M. McGregor, Emory University, United States
Copyright © 2021 Chen, Schipani, Schuch, Lam, Swardfager, Thiel and Edwards. This is an open-access article distributed under the terms of the Creative Commons Attribution License (CC BY). The use, distribution or reproduction in other forums is permitted, provided the original author(s) and the copyright owner(s) are credited and that the original publication in this journal is cited, in accordance with accepted academic practice. No use, distribution or reproduction is permitted which does not comply with these terms.
*Correspondence: Joyce L. Chen, joycelynn.chen@utoronto.ca
†Present address: Jodi D. Edwards, University of Ottawa Heart Institute, Ottawa, ON, Canada