- 1Department of Neurology, The First Affiliated Hospital, Jinan University, Guangzhou, China
- 2Medical Imaging Center, The First Affiliated Hospital, Jinan University, Guangzhou, China
Objective: To investigate the velocity and extent of cortical venous filling (CVF) and its association with clinical manifestations in patients with severe stenosis or occlusion of the middle cerebral artery (MCA) using dynamic computed tomography angiography (CTA).
Methods: Fifty-eight patients (36 symptomatic and 22 asymptomatic) with severe unilateral stenosis (≥70%) or occlusion of the MCA M1 segment who underwent dynamic CTA were included. Collateral status, antegrade flow, and CVF of each patient were observed using dynamic CTA. Three types of cortical veins were selected to observe the extent of CVF, and the absence of CVF (CVF-) was recorded. Based on the appearance of CVF in the superior sagittal sinus, instances of CVF, including early (CVF1), peak (CVF2), and late (CVF3) venous phases, were recorded. The differences in CVF times between the affected and contralateral hemispheres were represented as rCVFs, and CVF velocity was defined compared to the median time of each rCVF.
Results: All CVF times in the affected hemisphere were longer than those in the contralateral hemisphere (p < 0.05). Patients with symptomatic MCA stenosis had more ipsilateral CVF- (p = 0.02) and more delayed CVF at rCVF2 and rCVF21 (rCVF2-rCVF1) (p = 0.03 and 0.001, respectively) compared to those with asymptomatic MCA stenosis. For symptomatic patients, fast CVF at rCVF21 was associated with poor collateral status (odds ratio [OR] 6.42, 95% confidence interval [CI] 1.37–30.05, p = 0.02), and ipsilateral CVF- in two cortical veins was associated with poor 3-month outcomes (adjusted OR 0.025, 95% CI 0.002–0.33, p = 0.005).
Conclusions: Complete and fast CVF is essential for patients with symptomatic MCA stenosis or occlusion. The clinical value of additional CVF assessment should be explored in future studies to identify patients with severe MCA stenosis or occlusion at a higher risk of stroke occurrence and poor recovery.
Introduction
A series of studies have shown that patients with severe intracranial atherosclerotic stenosis (≥70%) or occlusion are at elevated risk of stroke occurrence and recurrence, regardless of whether the best medical therapy is received (1–3). For patients with symptomatic intracranial stenosis, the gradual development of collateral circulation plays a role in protecting perfusion and stabilizing cerebral blood flow (4, 5), including arterial collateral compensation as well as cerebral venous autoregulation (6). The intracranial venous system, a vital component of the vascular neural network, accounts for up to 70% of the total cerebral blood volume (7). However, vascular assessment in intracranial atherosclerosis is mainly based on arterial collateral recruitment, ignoring the significant element of intracranial venous drainage (8).
In recent years, imaging-based venous biomarkers such as cortical veins have been widely reported to play an essential role in acute ischemic events (7, 9–11). The presence of cortical venous filling (CVF) is related to a reduction in infarct volume and decreased severity of hemiparesis (10). Slow or poor CVF of the affected territory probably represents a delayed transmission of cerebral microcirculation, which is more prevalent in strokes in patients with poor collaterals (12–14). Several studies have demonstrated that the asymmetry of CVF can accurately predict clinical prognosis (15–18). In acute stroke patients with severe intracranial arterial stenosis or occlusion, the asymmetrical prominent cortical vein sign is associated with early neurological deterioration (19). However, there have been no reports on the combined assessment of the extent and velocity of CVF in patients with chronic atherosclerosis.
As a non-invasive technique, dynamic computed tomography angiography (CTA)/whole-brain CT perfusion (CTP) is a potential adjunct to traditional digital subtraction angiography (DSA) if time-resolved imaging is required (20). Dynamic CTA/CTP is widely used to evaluate vascular filling from arterial to venous phases because both the velocity and the extent of vessel filling can be considered at the same time, showing high diagnostic accuracy (21–27). To our knowledge, cortical veins, such as the superficial middle cerebral vein (SMCV) and the veins of Trolard (VOT) and Labbe (VOL), receive drainage from most of the arterial supply territories of the middle cerebral artery (MCA) and drain into the superior sagittal sinus (10, 28, 29). This study aimed to investigate the extent and velocity of these key venous fillings and determine whether there is an association between CVF and clinical manifestations in patients with severe unilateral MCA stenosis or occlusion using dynamic CTA/CTP.
Materials and Methods
Subjects
The Ethics Committee of the First Affiliated Hospital of Jinan University approved this study. From January 2018 to March 2020, we prospectively screened consecutive patients in the Department of Neurology of the First Affiliated Hospital of Jinan University, with unilateral MCA M1 segment stenosis (≥70%) or occlusion confirmed by DSA or CTA. These patients were divided into symptomatic and asymptomatic groups. Symptomatic patients were those with ischemic stroke or transient ischemic attack within 2 weeks following the onset of symptoms in the distribution of severe stenotic MCA or occlusion. Asymptomatic patients were considered for inclusion if there was no history of cerebrovascular events related to the internal carotid system but still had unilateral MCA M1 segment stenosis (≥70%) or occlusion detected by DSA or CTA. All patients received antiplatelet medication with aggressive risk factors control after admission. Written informed consent was obtained, and dynamic CTA/CTP examinations were performed for each patient.
Patients with any of the following conditions were excluded: (1) internal carotid artery stenosis (≥50%) or contralateral MCA stenosis (≥50%); (2) previous internal carotid artery or MCA stenting, balloon dilatation, or endarterectomy; (3) non-atherosclerotic vasculopathy, such as dissection, moyamoya disease, or vasculitis; (4) evidence of cardiogenic embolism; (5) poor image quality hindering further image analysis; and (6) CT examination-related contraindications. For symptomatic patients, the National Institutes of Health Stroke Scale (NIHSS) score was assessed at the time of admission, and the modified Rankin score (mRS) was obtained at 3 months by telephone interview or outpatient visit.
CT Protocol
All patients underwent dynamic CTA/CTP examination with a 320-slice multidetector (Aquilion ONE; Cannon Medical Systems, Tokyo, Japan). A total volume of 50 mL of contrast material with an iodine content of 370 mg/mL (Ultravist 370; Bayer, Leverkusen, Germany) was injected at a flow rate of 6 mL/s. The CT scanning parameters were as follows: tube voltage, 80 kV; matrix, 512 × 512; field of view, 320 mm; rotation time, 0.35 s; and collimator, 0.5 mm × 320. A total of 19 whole-brain volume data were obtained for every patient and loaded into a Vitrea Fx 6.3 workstation (Vital Images, Minnetonka, MN). Based on the separation of the arterial and venous time attenuation curves (TACs) using contrast enhancement of the contralateral MCA and the superior sagittal sinus (25), the maximum intensity projection (MIP) images at different phases were reconstructed. We defined the time point with the best contrast opacification of the bilateral MCA, which was less affected by cortical veins and venous sinuses as the arterial phase (A-TAC) and the time point at the peak points of the venous TAC as the venous phase (V-TAC) (Figure 1A). The stenotic degree of the MCA M1 segment was calculated using 3D CTA with dedicated imaging software (Figure 1B) or verified by DSA (30).
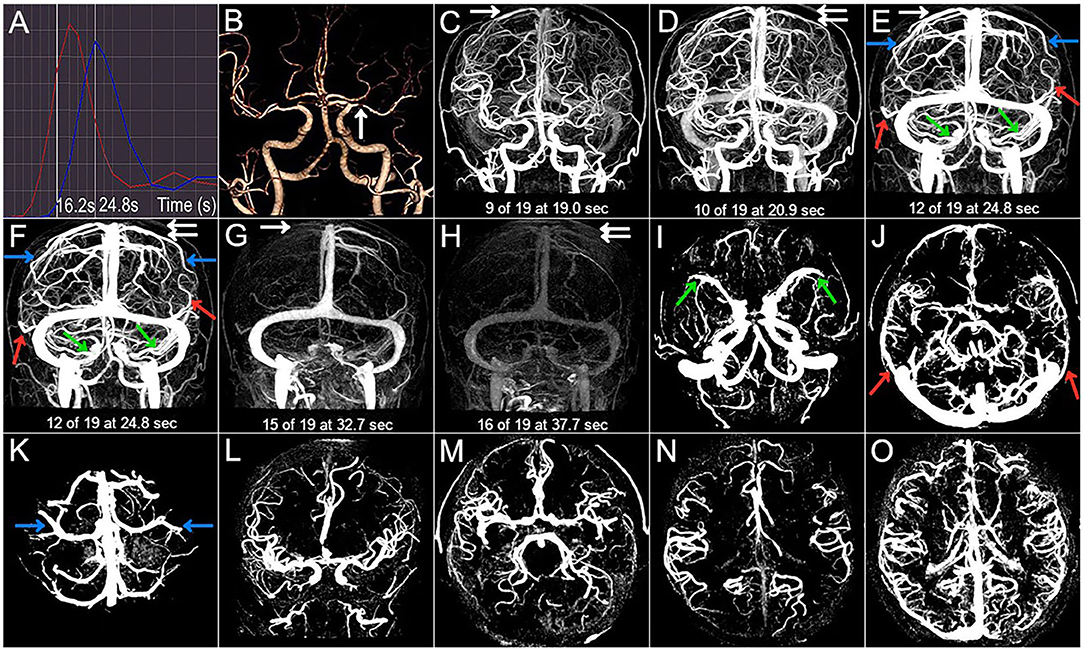
Figure 1. Case 1. A 66-year-old man with a history of hypertension and lipid disorder presented with dizziness for 3 days. (A) The arterial and venous time attenuation curves (TACs; red and blue, respectively). The selected arterial phase on TAC (A-TAC) was 16.2 s, and the time-to-peak on the venous TAC (V-TAC) was 24.8 s. (B) The arrow points to the left MCA M1 severe stenosis at A-TAC on three-dimensional (3D) computed tomographic angiography (CTA). (C–H) The 3D computed tomography venography (CTV) shows cortical venous filling (CVF) draining into the superior sagittal sinus at early (CVF1), peak (CVF2), and late venous phases (CVF3) in the affected (double white arrow) and contralateral (white arrow) hemispheres. Cortical veins begin to be visible in the contralateral (C, CVF1, 19.0 s) and affected hemispheres (D, CVF1, 20.9 s). The maximum contrast opacification of all cortical veins in the contralateral (E, CVF2, 24.8 s) and affected hemispheres (F, CVF2, 24.8 s) appear at the same time, and contrast medium in all cortical veins disappears in the contralateral (G, CVF3, 32.7 s) and affected hemispheres (H, CVF3, 37.7 s). CVF21 and CVF31 of the contralateral hemisphere are 5.8 s and 13.7 s, respectively, while the CVF21 and CVF31 of the affected hemisphere are 3.9 s and 16.8 s, respectively. The mean difference between the affected and contralateral hemispheres is 1.9 s for rCVF1, 0 s for rCVF2, 5 s for rCVF3,−1.9 s for rCVF21, and 3.1 s for rCVF31. The presence (color arrow) and absence (circle) of SMCV (green), VOL (red), and VOT (blue) across all whole venous phases (marked as SMCV+/VOL+/VOT+ and SMCV-/VOL-/VOT-, respectively) are displayed in the 3D CTV and (I–K) axial planes of the V-TAC. SMCV-, VOL-, and VOT- are not found in the bilateral hemispheres. (L,M) Antegrade flow assessment at TAC in the coronal and axial planes. Contrast filling of the MCA M1 segment and its distal branches in the affected hemisphere is more than two-thirds of the contralateral hemisphere. the contralateral hemisphere, and antegrade flow is preserved. (N,O) Collateral status assessment at A-TAC and V-TAC in the axial plane. Complete contrast enhancement of collateral flow at V-TAC in the affected hemisphere with good collateral status.
Image Analysis
Using the reconstructed three-dimensional (3D) CT venography (CTV) MIP images, we observed contrast enhancement of all cortical veins that drained into the superior sagittal sinus. We defined CVF1 as the time point when any cortical vein began to appear, CVF2 as when most cortical veins reached maximum contrast opacification, and CVF3 as the first moment when all cortical veins had completely disappeared (13). In addition, the difference between CVF2 and CVF1 (CVF21) represented the early to peak-venous phase, and the difference between CVF3 and CVF1 (CVF31) represented the whole venous phase. We calculated the above CVF times for both hemispheres according to the timing collection of the 19 volumes (Figures 1C–H). Moreover, the difference in CVF times between the affected and contralateral hemispheres was calculated (rCVFs). To further assess CVF velocity, the fast CVF was defined as a point in time that was less than or equal to the median rCVF, and slow CVF was the opposite (13).
To assess the extent of CVF, we first observed contrast filling of the three cortical veins on 3D CTV, including SMCV, VOT, and VOL. Subsequently, we assessed the MIP reconstruction of the cortical veins above the V-TAC in the axial plane (Figures 1I–K). The presence of CVF at any time point in the venous phase was defined as CVF+ (SMCV+/VOL+/VOT+), whereas the absence of CVF during the whole venous phase was defined as CVF- (SMCV-/VOL-/VOT-) (31). Because CVF- could be seen in the unaffected hemisphere in subjects with anatomical variations (32), we defined the condition of CVF- in the affected hemisphere and CVF+ in the contralateral hemisphere as ipsilateral CVF-. If there was ipsilateral CVF-, the type and number of ipsilateral CVF- were recorded in symptomatic and asymptomatic patients.
The antegrade flow across the stenotic MCA was evaluated in both the coronal and axial planes at A-TAC by referring to the thrombolysis in cerebral infarction scale based on DSA (33, 34) (Figures 1L,M). We reported antegrade flow as preserved or compromised according to whether the vessel filling of the MCA in the affected hemisphere was more than two-thirds of the contralateral hemisphere. Moreover, the collateral status in the affected hemisphere was evaluated at the level of the basal ganglia and thalamus in the axial plane at A-TAC and V-TAC by comparing it with that in the contralateral hemisphere (35) (Figures 1N,O). For our analysis, we reported good collateral status if the collaterals presented complete contrast enhancement at V-TAC or A-TAC and poor collateral status if no contrast enhancement or peripheral contrast enhancement was observed with V-TAC or A-TAC. Two experienced neuroradiologists (Z.Y.C and X.R.C), blinded to all clinical information, independently interpreted and measured the imaging data of all patients. In case of disagreements further judgment was made by consulting a neuroimaging radiologist with higher qualifications.
Statistical Analyses
Statistical analyses were conducted using SPSS version 21.0 (IBM Corp., Armonk, NY). Variables conforming to the contralateral distribution were reported as mean ± standard deviation, and a t-test was conducted for comparison between groups. Categorical variables were expressed as frequencies, and Pearson's chi-square test was used for comparisons between groups. The time from symptom onset or admission to the dynamic CTA/CTP examination, NIHSS score at admission, and CVF times were expressed as the median of the interquartile range (IQR) and were compared using the Mann-Whitney U test between groups. To study the relationship between CVF, collateral status, and clinical outcome in the symptomatic group, univariate and multivariate logistic models were used. Results are expressed as odds ratios (ORs) with 95% CIs. P-values of <0.05 were considered as statistically significant.
Results
Patient Characteristics
A total of 66 consecutive patients underwent dynamic CTA/CTP scanning. Due to poor image quality, eight patients were excluded. Among the 58 patients included in the study, 36 were symptomatic (31 with ischemic stroke in the MCA territory and five with transient ischemic attack) and 22 were asymptomatic. The median time from symptom onset to dynamic CTA/CTP examination of symptomatic patients was 11 days. The traditional risk factors for intracranial atherosclerosis, the stenotic degree of MCA, and the median time from admission to dynamic CTA/CTP scanning were similar between the symptomatic and asymptomatic groups (Table 1). Figure 1 shows a representative asymptomatic patient, and Figures 2, 3 show two representative symptomatic patients.
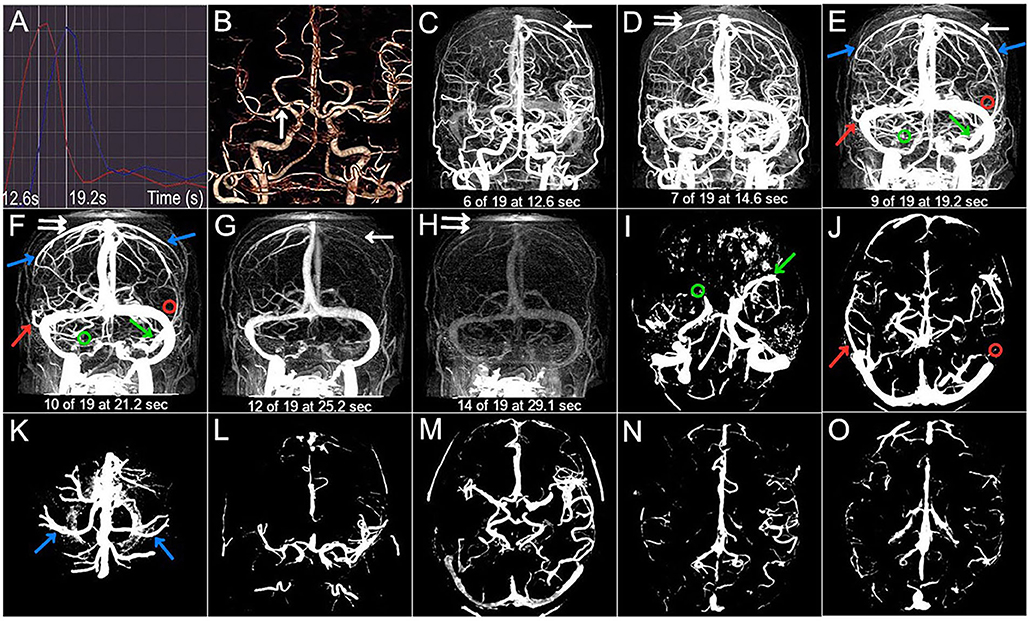
Figure 2. Case 2. A 54-year-old woman with a history of hypertension and diabetes presented with left-sided and left facial droop hemiparesis. The NIHSS score was 5 on admission. 3 months mRS score was 1 (good outcome). (A) The selected arterial and venous phases are 12.6 s and 19.2 s, respectively. (B) The arrow points to the right M1 severe stenosis. (C–H) The CVF1, CVF2, CVF3, CVF21, and CVF31 of the contralateral hemisphere (white arrow) are 12.6 s, 19.2 s, 25.2 s, 6.6 s, and 12.6 s, respectively, while the CVF1, CVF2, CVF3, CVF21, and CVF31 of the affected hemisphere (double white arrow) are 14.6 s, 21.2 s, 29.1 s, 6.6 s, and 14.5 s, respectively. The mean difference between the affected and contralateral hemisphere is 0 s for rCVF21. (C–K) The presence (color arrow) and the absence (circle) of SMCV (green), VOL (red), and VOT (blue). SMCV- is found in the affected hemisphere, and VOL- is found in the contralateral hemisphere. (L–O) Compromised antegrade flow and poor collateral status.
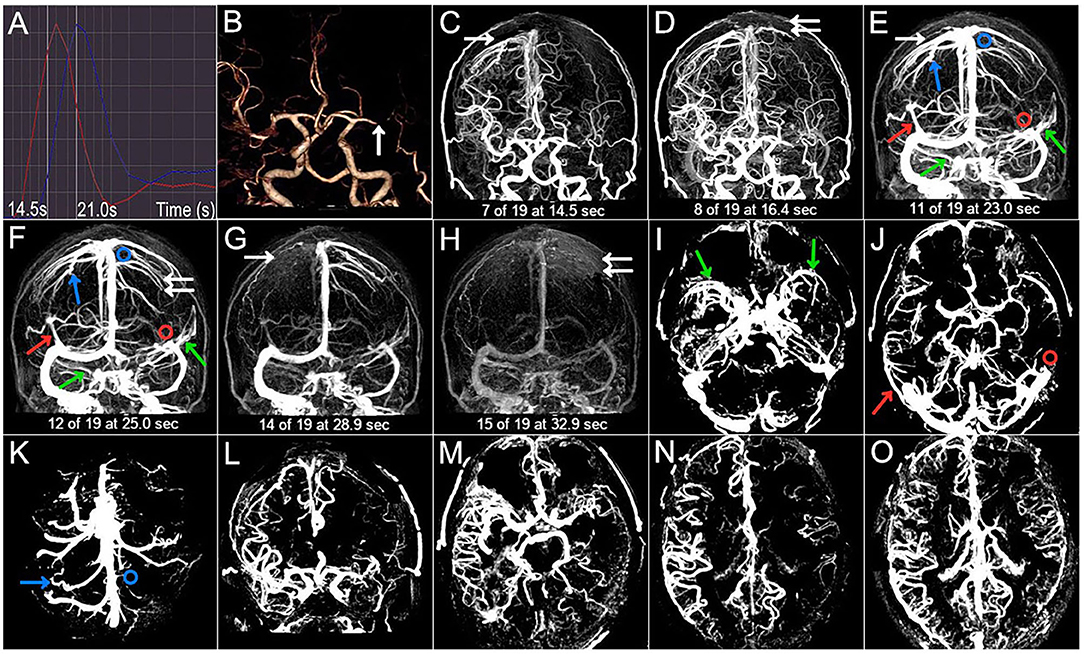
Figure 3. Case 3. A 50-year-old man with a history of hypertension, diabetes, and smoking presented with dysarthria and right-sided hemiparesis. NIHSS score was 6 on admission and 3-month mRS score was 3 (poor outcome). (A) The selected arterial phase and the venous phase are 14.5 s and 21.0 s, respectively. (B) The arrow points to the left M1 occlusion. (C–H) The CVF1, CVF2, CVF3, CVF21, and CVF31 of the contralateral hemisphere (white arrow) are 14.5 s, 23.0 s, 28.9 s, 8.5 s, and 14.4 s, respectively, while CVF1, CVF2, CVF3, CVF21, and CVF31 of the affected hemisphere (double white arrow) are 16.4 s, 25.0 s, 32.9 s, 8.6 s, and 16.5 s, respectively. The mean difference between the affected and contralateral hemisphere is 0.1 s for rCVF21. (C–K) SMCV-, VOL-, and VOT- are not found in the contralateral hemisphere (color arrow), while VOL- (red circle) and VOT- (blue circle) are shown in the affected hemisphere. (L–O) Compromised antegrade flow and poor collateral status.
Comparison of CVF Between Symptomatic and Asymptomatic Patients
The CVF times and instances of CVF- of the affected and contralateral hemispheres in both symptomatic and asymptomatic patients were compared, and the results are listed in Table 2. In symptomatic patients, CVF-, SMCV-, VOT-, and VOL- in the affected hemisphere were more common than in the contralateral hemisphere (p < 0.001, p = 0.02, 0.004, and 0.03, respectively), while there was no significant difference in the proportion and type of CVF- between the affected and contralateral hemispheres in the asymptomatic group. In addition, the CVF times of the affected hemisphere were all significantly longer than those of the contralateral hemisphere in both the symptomatic and asymptomatic groups (p < 0.05 for all CVF times).
Imaging findings of symptomatic and asymptomatic patients are listed in Table 3. Since there was no ipsilateral SMCV-, VOT-, or VOL- at the same time in either group, we divided the number of instances of ipsilateral CVF- into two groups: CVF- = 1 and CVF- = 2. Patients with symptomatic MCA stenosis had longer CVF times at rCVF2 and rCVF21 (p = 0.03 and 0.001, respectively; e.g., 0 s in Figure 1 vs. 2 s in Figures 2, 3 for rCVF2; −1.9 s in Figure 1 vs. 0 s in Figure 2, 0.1 s in Figure 3 for rCVF21) and more ipsilateral CVF- (p = 0.02; e.g., ipsilateral CVF+ in Figure 1 vs. ipsilateral CVF- in Figures 2, 3) in the MCA territory of the affected hemisphere, but were similar in the type and number of ipsilateral CVF- compared to the asymptomatic group. In addition, there was no significant difference in collateral status or antegrade flow between the groups.
CVF Velocity and Collateral Status in Symptomatic Patients
It can be concluded from Table 3 that the mean difference between the affected and contralateral hemisphere was 2.0 s for rCVF1, 2.5 s for rCVF2, 2.7 s for rCVF3, 1.9 s for rCVF21, and 1.7 s for rCVF31 in symptomatic patients. Therefore, we selected fast rCVF21 if the difference in CVF time was ≤1.9 s compared to that in the contralateral hemisphere. In patients with symptomatic MCA stenosis, 22 had good collateral status and 14 had poor collateral status. The relationship between CVF velocity and collateral status at each time point is shown in Table 4. Fast CVF at rCVF21 was present in 8 (36%) patients with good collateral status, whereas it was found in 11 (79%) patients with poor collateral status (p = 0.02). In univariate analysis, fast CVF (only at rCVF21, i.e., early to peak-venous phase) was positively associated with poor collateral status (OR 6.42, 95% CI 1.37–30.05, p = 0.02; e.g., fast CVF at rCVF21 and poor collateral status in Figures 2, 3).
CVF and Neurological Outcomes at 3 Months in Symptomatic Patients
At 3 months after discharge, 25 patients had a favorable prognosis (mRS score 0–2), while 11 had a poor outcome (mRS score >2). Table 5 shows the associations between clinical and imaging variables and clinical outcomes at the 3-month follow-up. There was no significant relationship between baseline characteristics and clinical prognosis. The proportion of patients with poor outcomes was greater in those with higher NIHSS scores after admission (p = 0.04). Four patients underwent elective endovascular angioplasty for severe stenotic MCA within 3 months; however, there was a non-significant trend toward a good prognosis. Ipsilateral CVF-, type of ipsilateral CVF-, absence of filling of one cortical vein, poor collateral status, and compromised antegrade flow were not significantly related to poor clinical outcomes. Among the 11 patients with poor outcomes, the absence of filling of the two cortical veins was found in six cases (56%) (p < 0.001). In univariate analysis, the absence of filling of the two cortical veins was associated with clinical results (OR 0.04, 95% CI 0.003–0.36, p = 0.005). Furthermore, multivariate analysis showed that the absence of filling of the two cortical veins was still related to the poor outcome at the 3-month follow-up (adjusted OR 0.025, 95% CI, 0.002–0.33, p = 0.005) (Figure 2 vs. Figure 3).
Discussion
To the best of our knowledge, this is the first prospective study to describe the velocity and extent of CVF in patients with severe stenosis or occlusion of the MCA responsible or not responsible for recent ischemic stroke or transient ischemic attack. Prolonged CVF times were commonly found at different stages of the venous phase in the affected hemisphere. Patients with symptomatic MCA stenosis also had longer CVF times and more ipsilateral CVF- than those with asymptomatic MCA stenosis. Moreover, our preliminary study demonstrated that fast CVF was associated with poor collateral status, and the absence of filling of the two cortical veins was linked with poor outcome, suggesting the essential and irreplaceable role of cortical veins in patients with symptomatic high-grade MCA stenosis or occlusion.
In this study, we noticed an obvious relationship between delayed filling of the ipsilateral cortical veins and severe stenosis or occlusion of the MCA. Adequate collateral perfusion requires arterial and venous autoregulation to redistribute cerebral blood flow and maintain cerebral perfusion (6, 7), which might indicate a slowdown of venous drainage to varying degrees in response to chronic cerebral hypoperfusion. However, there was a similar proportion of CVF- in the bilateral hemispheres of asymptomatic patients. A possible explanation is that compensatory venous collaterals can extensively communicate at the cortical surface (36, 37), resulting in delayed venous drainage, but the presence of cortical vein collaterals (SMCV, VOT, VOL).
Similar to previous research studies on acute MCA occlusion (10, 38), our study demonstrated that symptomatic patients were more likely to experience slower and asymmetrical CVF in the affected MCA territory. No serial studies have assessed changes in the cortical veins over time after qualifying ischemic events. If ischemic strokes occur under the condition of chronic stenosis, a compensatory hemodynamic function of venous collaterals associated with increased venous blood volume and cerebral vasodilation may be continuously and seriously impaired (39), resulting in slower or even absent ipsilateral CVF for a long time. Additionally, a previous study has shown that VOL and VOT are often seen in a certain hemisphere in contralateral subjects (40), which may partially explain why the type and number of ipsilateral CVF- between the symptomatic and asymptomatic groups were not enough to contribute to a statistical difference.
We also found that fast CVF was closely related to poor arterial collateral in symptomatic patients. This finding was not in line with that of a previous study, which demonstrated a trend toward slow CVF with a worse collateral grade in patients with acute MCA occlusion (10). It is important to mention that the clinical correlations of arterial collaterals in this study were not evident, indicating that the rapid and effective drainage of cortical veins may be beneficial in compensating for potential arterial hemodynamic damage. Moreover, once chronic atherosclerosis reaches a stage with severe stenosis or complete occlusion, it will lead to insufficient and slow venous drainage far beyond the nearby arterial collaterals, even if good collateral flow tends to compensate circulation. Interestingly, delayed drainage of cortical veins in the early to peak-phase, not the late venous phase (41), was related to arterial collateral status and stroke occurrence in this study. The CVF time lag in the affected hemisphere has been proven to be associated with prolonged mean transit time (12), which probably reflects compromised perfusion through microcirculation at an earlier stage of venous drainage due to progressive microvascular obstruction (16).
Our results support the effect of asymmetric CVF on the prognosis of ischemic stroke demonstrated in previous studies (15–18). In contrast to acute occlusion, the number of ipsilateral CVF-, rather than the type of ipsilateral CVF- has superior prognostic value in patients with symptomatic MCA stenosis in this study, which might be explained by hemodynamic mechanisms. First, the lower extent of CVF during chronic stenosis may be explained by the upregulation of vascular endothelial cell adhesion molecules and the downregulation of tight junction proteins to weaken the blood-brain barrier in hypoperfusion (42). Other explanations include active venous contraction (43), leukocyte-platelet aggregation obstruction (44, 45), and passive thin-walled venule compression (46). In addition, the respective collateral pathways of venous drainage are irrevocably impaired when the number of ipsilateral CVF- is high (47). Furthermore, the severely impaired venous drainage pathway around the lesions, accompanied by long-term cerebral hypoperfusion, will ultimately damage the required perfusion and upstream arterial regulation (48), causing subsequent pathophysiological consequences that are difficult to correct.
In conclusion, we used dynamic CTA/CTP to investigate the relationships between various stages of cortical venous flow, symptom occurrence, and clinical prognosis in the present study. An increased proportion of CVF- or prolonged CVF times in the early to peak-phase in the affected hemisphere are more likely to be associated with recent ischemic events in patients with severe MCA stenosis or occlusion. Moreover, a lower extent of CVF is associated with worse short-term clinical outcomes, and fast CVF is likely to be a reaction to poor collateral flow, suggesting the importance of complete and fast cortical venous drainage in symptomatic MCA stenosis. Further prospective studies are warranted to validate the feasibility of CVF assessment in identifying patients with high-grade MCA stenosis or occlusion at a higher risk of stroke occurrence and poor prognosis.
However, this study had several shortcomings. First, the sample size collected in this study was small. Larger sample sizes will be critical for moving the field forward. Second, the period between symptom onset and imaging acquisition could not be determined for asymptomatic patients without clinical symptoms. Third, CVF-related MIP images were acquired in the target subjects with unilateral MCA severe stenosis or occlusion, which may be difficult to rule out patients with multifocal intracranial atherosclerotic stenosis. Therefore, a contralateral MCA with <50% stenosis was used as a control. Fourth, there is a certain proportion of CVF- in healthy individuals. Considering the physiological differences in each patient, our study mainly focused on the asymmetry of CVF in the affected hemisphere and evaluated whether ipsilateral CVF- had any effect on the occurrence and prognosis of stroke. Finally, the time interval of commonly used clinical image acquisition was quite long because of the clinical limitations of dynamic CTA/CTP. Therefore, it is necessary to carefully compare the CVF times at different stages of venous drainage.
Data Availability Statement
The raw data supporting the conclusions of this article will be made available by the authors, without undue reservation.
Ethics Statement
The studies involving human participants were reviewed and approved by The Ethics Committee of the First Affiliated Hospital of Jinan University. The patients/participants provided their written informed consent to participate in this study.
Author Contributions
LH contributed to the conception and design of the study and edited the manuscript. JL performed data analyses and wrote the manuscript. JL and YS contributed toward the patient recruitment. ZC and XC interpreted and measured the imaging data. All authors contributed to the article.
Funding
This work was supported by grants from the Natural Science Foundation of Guangdong Province (Grant Number 2018A0303130264), and the National Natural Science Foundation of China (Grant Number 81971120).
Conflict of Interest
The authors declare that the research was conducted in the absence of any commercial or financial relationships that could be construed as a potential conflict of interest.
Acknowledgments
We would like to thank Editage (www.editage.cn) for English language editing.
References
1. Li H, Wong KS. Racial distribution of intracranial and extracranial atherosclerosis. J Clin Neurosci. (2003) 10:30–4. doi: 10.1016/S0967-5868(02)00264-3
2. Kasner SE, Chimowitz MI, Lynn MJ, Howlett-Smith H, Stern BJ, Hertzberg VS, et al. Predictors of ischemic stroke in the territory of a symptomatic intracranial arterial stenosis. Circulation. (2006) 113:555–63. doi: 10.1161/CIRCULATIONAHA.105.578229
3. Qureshi AI, Caplan LR. Intracranial atherosclerosis. Lancet. (2014) 383:984–98. doi: 10.1016/S0140-6736(13)61088-0
4. Liebeskind DS. Understanding blood flow: the other side of an acute arterial occlusion. Int J Stroke. (2007) 2:118–20. doi: 10.1111/j.1747-4949.2007.00117.x
5. Liebeskind DS, Cotsonis GA, Saver JL, Lynn MJ, Cloft HJ, Chimowitz MI, et al. Collateral circulation in symptomatic intracranial atherosclerosis. J Cereb Blood Flow Metab. (2011) 31:1293–301. doi: 10.1038/jcbfm.2010.224
6. Faber JE, Moore SM, Lucitti JL, Aghajanian A, Zhang H. Sex differences in the cerebral collateral circulation. Transl Stroke Res. (2017) 8:273–83. doi: 10.1007/s12975-016-0508-0
7. Zhang JH, Badaut J, Tang JP, Obenaus A, Hartman R, Pearce WJ. The vascular neural network-a new paradigm in stroke pathophysiology. Nat Rev Neurol. (2012) 8:711–6. doi: 10.1038/nrneurol.2012.210
8. Tomita M, Gotoh F, Amano T, Tanahashi N, Tanaka K. Low perfusion hyperemia' following middle cerebral arterial occlusion in cats of different age groups. Stroke. (1980) 11:629–36. doi: 10.1161/01.STR.11.6.629
9. Ishikawa M, Kusaka G, Yamaguchi N, Sekizuka E, Nakadate H, Minamitani H, et al. Platelet and leukocyte adhesion in the microvasculature at the cerebral surface immediately after subarachnoid hemorrhage. Neurosurgery. (2009) 64:546–53. doi: 10.1227/01.NEU.0000337579.05110.F4
10. Sasaki M, Honmou O, Radtke C, Kocsis JD. Development of a middle cerebral artery occlusion model in the nonhuman primate and a safety study of i.v. infusion of human mesenchymal stem cells. PLoS ONE. (2011) 6:e26577. doi: 10.1371/journal.pone.0026577
11. Garaci FG, Marziali S, Meschini A, Fornari M, Rossi S, Melis M, et al. Brain hemodynamic changes associated with chronic cerebrospinal venous insufficiency are not specific to multiple sclerosis and do not increase its severity. Radiology. (2012) 265:233–9. doi: 10.1148/radiol.12112245
12. Menon BK, Bai HD, Modi J, Demchuk AM, Hudon M, Goyal M, et al. The ICV sign as a marker of increased cerebral blood transit time. Can J Neurol Sci. (2013) 40:187–91. doi: 10.1017/S0317167100013718
13. van den Wijngaard IR, Wermer JHM, Boiten J, Algra A, Holswilder, Meijer JAF, et al. Cortical venous filling on dynamic computed tomographic angiography: a novel predictor of clinical outcome in patients with acute middle cerebral artery stroke. Stroke. (2016) 47:762–7. doi: 10.1161/STROKEAHA.115.012279
14. Munuera J, Blasco G, Hernández-Pérez M, Daunis-I-Estadella P, Dávalos A, Liebeskind DS, et al. Venous imaging-based biomarkers in acute ischemic stroke. J Neurol Neurosurg Psychiatry. (2017) 88:62–9. doi: 10.1136/jnnp-2016-314814
15. Yu WG, Rives J, Welch B, White J, Stehel E, Samson D. Hypoplasia or occlusion of the ipsilateral cranial venous drainage is associated with early fatal edema of middle cerebral artery infarction. Stroke. (2009) 40:3736–9. doi: 10.1161/STROKEAHA.109.563080
16. Parthasarathy R, Kate M, Rempel JL, Liebeskind DS, Jeerakathil T, Butcher KS, et al. Prognostic evaluation based on cortical vein score difference in stroke. Stroke. (2013) 44:2748–54. doi: 10.1161/STROKEAHA.113.001231
17. Mucke J, Möhlenbruch M, Kickingereder P, Kieslich PJ, Bäumer P, Gumbinger C, et al. Asymmetry of deep medullary veins on susceptibility weighted MRI in patients with acute MCA stroke is associated with poor outcome. PLoS ONE. (2015) 10:e0120801. doi: 10.1371/journal.pone.0120801
18. Parthasarathy R, Sohn SI, Jeerakathil T, Kate MP, Mishra SM, Nambiar VK, et al. A combined arterial and venous grading scale to predict outcome in anterior circulation ischemic stroke. J Neuroimag. (2015) 25:969–77. doi: 10.1111/jon.12260
19. Li W, Xiao WM, Luo GP, Liu YL, Qu JF, Fang XW, et al. Asymmetrical cortical vein sign predicts early neurological deterioration in acute ischemic stroke patients with severe intracranial arterial stenosis or occlusion. BMC Neurol. (2020) 20:331. doi: 10.1186/s12883-020-01907-w
20. Snyder KV, Mokin M, Bates VE. Neurologic applications of whole-brain volumetric multidetector computed tomography. Neurol Clin. (2014) 32:237–51. doi: 10.1016/j.ncl.2013.08.001
21. Hassler O. Deep cerebral venous system in man. A microangiographic study on its areas of drainage and its anastomoses with the superficial cerebral veins. Neurology. (1966) 16:505–11. doi: 10.1212/WNL.16.5.505
22. Abud DG, Spelle L, Piotin M, Mounayer C, Vanzin JR, Moret J. Venous phase timing during balloon test occlusion as a criterion for permanent internal carotid artery sacrifice. AJNR. (2005) 26:2602–9. Available online at: http://www.ajnr.org/content/26/10/2602/tab-article-info
23. Förster A, Mürle B, Böhme J, Al-Zghloul M, Kerl HU, Wenz H, et al. Perfusion-weighted imaging and dynamic 4D angiograms for the estimation of collateral blood flow in lacunar infarction. J Cereb Blood Flow Metab. (2016) 36:1744–54. doi: 10.1177/0271678X15606458
24. Frölich AMJ, Wolff SL, Psychogios MN, Klotz E, Schramm R, Wasser K, et al. Time-resolved assessment of collateral flow using 4D CT angiography in large-vessel occlusion stroke. Eur Radiol. (2014) 24:390–6. doi: 10.1007/s00330-013-3024-6
25. Beyer SE, Thierfelder KM, von Baumgarten L, Rottenkolber M, Meinel FG, Janssen H, et al. Strategies of collateral blood flow assessment in ischemic stroke: prediction of the follow-up infarct volume in conventional and dynamic. CTA. AJNR Am J Neuroradiol. (2015) 36:488–94. doi: 10.3174/ajnr.A4131
26. Menon BK, d'Esterre CD, Qazi EM, Almekhlafi M, Hahn L, Demchuk AM, et al. Multiphase CT angiography: a new tool for the imaging triage of patients with acute ischemic stroke. Radiology. (2015) 275:510–20. doi: 10.1148/radiol.15142256
27. van den Wijngaard IR, Boiten J, Holswilder G, Algra A, Dippel WJD, Velthuis BK, et al. Impact of collateral status evaluated by dynamic computed tomographic angiography on clinical outcome in patients with ischemic stroke. Stroke. (2015) 46:3398–404. doi: 10.1161/STROKEAHA.115.010354
28. Meder JF, Chiras J, Roland J, Guinet P, Bracard S, Bargy F. Venous territories of the brain. J Neuroradiol. (1994) 21:118–33.
29. Tong LS, Guo ZN, Ou YB, Yu YN, Zhang XC, Tang JP, et al. Cerebral venous collaterals: A new fort for fighting ischemic stroke? Prog Neurobiol. (2018) 163–164:172–93. doi: 10.1016/j.pneurobio.2017.11.001
30. Samuels OB, Joseph GJ, Lynn MJ, Smith HA, Chimowitz MI. A standardized method for measuring intracranial arterial stenosis. AJNR Am J Neuroradiol. (2000) 21:643–6. Available online at: http://www.ajnr.org/content/21/4/643.long
31. Zhang S, Lai YX, Ding XF, Parsons M, Zhang JH, Lou M. Absent filling of ipsilateral superficial middle cerebral vein is associated with poor outcome after reperfusion therapy. Stroke. (2017) 48:907–14. doi: 10.1161/STROKEAHA.116.016174
32. Rhoton AL Jr. The cerebral veins. Neurosurgery. (2002) 51: S159–205. doi: 10.1097/00006123-200210001-00005
33. Higashida RT, Furlan AJ, Roberts H, Tomsick T, Connors B, Barr J, et al. Trial design and reporting standards for intra-arterial cerebral thrombolysis for acute ischemic stroke. Stroke. (2003) 34:e109–37. doi: 10.1161/01.STR.0000082721.62796.09
34. Jia BX, Liebeskind DS, Song LG, Xu XT, Sun X, Liu L, et al. Performance of computed tomography angiography to determine anterograde and collateral blood flow status in patients with symptomatic middle cerebral artery stenosis. Interv Neuroradiol. (2017) 23:267–73. doi: 10.1177/1591019917694480
35. Xue J, Peng YJ, Zhang YN, Chen WQ, Pan YS, Yu Qi, et al. Preliminary application of CT perfusion source images for evaluating regional collateral circulation in unilateral Moyamoya disease. Quant Imaging Med Surg. (2019) 9:615–24. doi: 10.21037/qims.2019.04.05
36. Guibert R, Fonta C, Risser L, Plouraboué F. Coupling and robustness of intra-cortical vascular territories. Neuroimage. (2012) 62:408–17. doi: 10.1016/j.neuroimage.2012.04.030
37. Feuerstein D, Backes H, Gramer M, Takagaki M, Gabel P, Kumagai T, et al. Regulation of cerebral metabolism during cortical spreading depression. J Cereb Blood Flow Metab. (2016) 36:1965–77. doi: 10.1177/0271678X15612779
38. Sorimachi T, Morita K, Sasaki O, Koike T, Fujii Y. Change in cortical vein appearance on susceptibility-weighted MR imaging before and after carotid artery stenting. Neurol Res. (2011) 33:314–8. doi: 10.1179/016164110X12644252260510
39. Rosso C, Belleville M, Pires C, Dormont D, Crozier S, Chiras J, et al. Clinical usefulness of the visibility of the transcerebral veins at 3T on T2*-weighted sequence in acute stroke patients. Eur J Radiol. (2012) 81:1282–7. doi: 10.1016/j.ejrad.2011.03.025
40. DiChiro G. Angiographic patterns of cerebral convexity veins and superficial dural sinuses. AJR Am J Roentgenol. (1962) 87:308–21.
41. Bhaskar S, Bivard A, Parsons M, Nilsson M, Attia JR, Stanwell P, et al. Delay of late-venous phase cortical vein filling in acute ischemic stroke patients:Associations with collateral status. J Cereb Blood Flow Metab. (2017) 37:671–82. doi: 10.1177/0271678X16637611
42. Beggs CB. Venous hemodynamics in neurological disorders: an analytical review with hydrodynamic analysis. BMC Med. (2013) 11:142. doi: 10.1186/1741-7015-11-142
43. Abels C, Röhrich F, Corvin S, Meyermann R, Baethmann A, Schürer L. Leukocyte-endothelium-interaction in pial vessels following global, cerebral ischaemia. Acta Neurochir (Wien). (2000) 142:333–9. doi: 10.1007/s007010050043
44. Belayev L, Pinard E, Nallet H, Seylaz J, Liu YT, Riyamongkol P, et al. Albumin therapy of transient focal cerebral ischemia: in vivo analysis of dynamic microvascular responses. Stroke. (2002) 33:1077–84. doi: 10.1161/hs0402.105555
45. Lapi D, Vagnani S, Cardaci E, Paterni M, Colantuoni A. Rat pial microvascular responses to melatonin during bilateral common carotid artery occlusion and reperfusion. J Pineal Res. (2011) 51:136–44. doi: 10.3389/fphys.2012.00099
46. Chen S, Chen YJ, Xu L, Matei N, Tang JP, Feng H, et al. Venous system in acute brain injury: mechanisms of pathophysiological change and function. Exp Neurol. (2015) 272:4–10. doi: 10.1016/j.expneurol.2015.03.007
47. van Everdingen KJ, Visser GH, Klijn CJ, Kappelle LJ, van der Grond J. Role of collateral flow on cerebral hemodynamics in patients with unilateral internal carotid artery occlusion. Ann Neurol. (1998) 44:167–76. doi: 10.1002/ana.410440206
Keywords: cortical venous filling, middle cerebral artery, severe stenosis, occlusion, dynamic computed tomography angiography
Citation: Lin J, Cheng Z, Shi Y, Cai X and Huang L (2021) Evaluating the Velocity and Extent of Cortical Venous Filling in Patients With Severe Middle Cerebral Artery Stenosis or Occlusion. Front. Neurol. 12:610658. doi: 10.3389/fneur.2021.610658
Received: 26 September 2020; Accepted: 16 March 2021;
Published: 08 April 2021.
Edited by:
Osama O. Zaidat, Northeast Ohio Medical University, United StatesReviewed by:
Luis Rafael Moscote-Salazar, University of Cartagena, ColombiaNing Ma, Capital Medical University, China
Copyright © 2021 Lin, Cheng, Shi, Cai and Huang. This is an open-access article distributed under the terms of the Creative Commons Attribution License (CC BY). The use, distribution or reproduction in other forums is permitted, provided the original author(s) and the copyright owner(s) are credited and that the original publication in this journal is cited, in accordance with accepted academic practice. No use, distribution or reproduction is permitted which does not comply with these terms.
*Correspondence: Li'An Huang, aHVhbmdsaWFuMTMwNkAxMjYuY29t; Xiang'Ran Cai, Y2FpeHJhbkBqbnUuZWR1LmNu